Abstract
The awareness of the presence and consequences of sarcopenia has significantly increased over the past decade. Sarcopenia is defined as gradual loss of muscle mass and strength and ultimately loss of physical performance associated with aging and chronic disease. The prevalence of sarcopenia is higher in chronic obstructive pulmonary disease (COPD) compared to age-matched controls. Current literature suggests that next to physical inactivity, COPD-specific alterations in physiological processes contribute to accelerated development of sarcopenia. Sarcopenia in COPD can be assessed according to current guidelines, but during physical performance testing, ventilatory limitation should be considered. Treatment of muscle impairment can halt or even reverse sarcopenia, despite respiratory impairment. Exercise training and protein supplementation are currently at the basis of sarcopenia treatment. Furthermore, effective current and new interventions targeting the pulmonary system (eg, smoking cessation, bronchodilators and lung volume reduction surgery) may also facilitate muscle maintenance. Better understanding of disease-specific pathophysiological mechanisms involved in the accelerated development of sarcopenia in COPD will provide new leads to refine nutritional, exercise and physical activity interventions and develop pharmacological co-interventions.
Introduction
Over the past decade, there has been increasing awareness of the prevalence and consequences of sarcopenia. The term sarcopenia, derived from the Greek words “sarx” (flesh) and “penia” (poverty) was introduced in 1989 by Rosenberg to describe generalized and progressive loss of muscle mass and function with advancing age.Citation1 In that same time period, Schols et al showed that low muscle mass is also highly prevalent in patients with advanced Chronic Obstructive Pulmonary Disease (COPD).Citation2 While loss of muscle mass in COPD was initially studied in the context of cachexia, these early studies already showed that low muscle mass may also occur in weight stable normal to overweight COPD patients, adversely affecting physical performance.Citation2,Citation3
More recently, the prevalence of sarcopenia showed to be higher in COPD patients compared to age-matched controls, implying more pronounced contribution of lifestyle factors, for example, inactivity and disease-specific factors to its accelerated development.Citation4,Citation5 Whereas treatment of respiratory impairment in COPD is largely symptomatic, non-pharmacological interventions can halt progression or even reverse the loss of muscle mass and function.Citation6,Citation7 Therefore, treatment of sarcopenia can contribute to alleviate disease burden and improve quality of life in COPD patients.
In this review, we provide a concise overview of the implications of sarcopenia for COPD patients, its clinical assessment and the latest evidence on treatment efficacy and feasibility, while taking limitations and opportunities imposed by COPD pathology into consideration.
Definition of Sarcopenia
Over the past decade, several international committees and working groups have published consensus statements on the definition and criteria of sarcopenia. These different definitions all consider a combination of low muscle mass and function as the core components of sarcopenia; because of difference in ethnicity, genetics and body size, different cut-off points have however been reported, which may contribute to the heterogeneity in reported prevalence of sarcopenia.Citation8–Citation12 In 2019, the European Working Group on Sarcopenia in Older People (EWGSOP) published an updated version of their previous consensus statement,Citation13 providing an operational definition from probable sarcopenia with low muscle strength, to confirmed sarcopenia with low muscle mass and eventually severe sarcopenia with low muscle mass, strength and physical performance present. Furthermore, a distinction between primary sarcopenia (solely age-related) and secondary sarcopenia (caused by additional factors other than aging, including COPD) was proposed.Citation11 In 2019 the Asian Working Group for Sarcopenia (AWGS) also published a consensus update on sarcopenia diagnosis and treatment, but retained their original definition of sarcopenia as “age-related loss of skeletal muscle mass plus loss of muscle strength and/or reduced physical performance”.Citation12 Furthermore, AWGS 2019 introduced the terms “possible sarcopenia”, defined as low muscle strength with or without reduced physical performance, and “severe sarcopenia” defined by low muscle mass, strength and physical performance, allowing a distinction in severity of sarcopenia. Each of these definitions considers muscle mass and function at a certain point in time in comparison to reference values to define a sarcopenic state. In contrast, in the definitions of cachexia, an involuntary loss of lean tissue mass within a set time period is at the core.Citation14–Citation16 Muscle atrophy (state) and muscle mass loss (process) are overlapping features of both conditions.
Prevalence of Sarcopenia in COPD
The reported numbers for sarcopenia in the general population vary from 4.4% to 27.5%,Citation17,Citation18 not only because of the different definitions and cutoff points used, but also depending on the studied population. Sarcopenia prevalence is lowest in community-based populations and the highest in nursing home populations.Citation4,Citation8,Citation19 A meta-analysis from 2017 by Shafiee et alCitation20 reported an overall estimated prevalence of sarcopenia of 10%, whereas the Rotterdam Study by Trajanoska et alCitation17 from 2018 only reported a prevalence of 4.4% in a general community population. The meta-analysis demonstrated an influence of assessment methods (some being addressed below) used on sarcopenia prevalence, which could (partly) explain the heterogeneity of results between these two studies, next to different definitions (based on different parameters and different cut-offs) of sarcopenia over time. To reduce heterogeneity between studies, one universal consensus definition would therefore be preferable. The Rotterdam study showed that the prevalence of COPD in subjects with sarcopenia was higher than in the non-sarcopenic population. In line, a recent meta-analysis by Benz et al reported a higher prevalence of sarcopenia in COPD patients than in non-COPD patients with a 21.6% pooled prevalence of sarcopenia in COPD. They also demonstrated that prevalence depends on the selected population and reported a prevalence range from 8% in community based populations to 63% in nursing home populations.Citation4 Lastly, the most recent meta-analysis reported a pooled prevalence of sarcopenia in COPD patients of 27.5% and a significantly higher prevalence in more severe COPD stages.Citation18 Despite considerable heterogeneity between studies, these results all point towards a higher prevalence of sarcopenia in COPD than in the general population.
Consequences of Sarcopenia
Low fat-free mass (FFM) and sarcopenia proved independent predictors of both all-cause mortality and specific COPD-related mortality.Citation21–Citation23 Reduced muscle strength and impaired physical performance are predictors of poor health-related quality of life, more and longer hospital stays and higher mortality in both the general population as well as in COPD patients specifically.Citation24,Citation25 Compared to the general population, increased incidence of falls and fractures has been reported in COPD patients.Citation26,Citation27 Additionally, in COPD the presence of sarcopenia increased the risk for osteopenia and osteoporosis, which complies with the association of increased risk of falls and fractures in primary sarcopenia.Citation28 Experienced quality of life measured by the Sarcopenia and Quality of Life (SarQOL) questionnaire,Citation29,Citation30 exercise capacity and physical activity levels were limited in both sarcopenic and COPD patients, with dyspnea as an additional impairing factor in COPD.Citation4,Citation5,Citation31 Physical inactivity resulted in further deconditioning and deterioration of muscle function,Citation32–Citation34 and can therefore be considered both a cause and a consequence of sarcopenia. Acute exacerbations of COPD (AECOPD) during which various catabolic triggers converge, may accelerate the development or progress of sarcopenia. Indeed, recently, a sarcopenia prevalence of 48% was demonstrated within a COPD population with frequent AECOPD,Citation35 which is significantly higher than the most recent reported pooled prevalence of 27.5% in COPD patients.Citation18
Pathophysiology of Sarcopenia
The underlying pathophysiologic process of sarcopenia is complex and not yet completely understood. Multiple determinants and risk factors trigger (patho)physiological mechanisms that affect skeletal muscle homeostasis, resulting in reduced muscle mass and strength.Citation36,Citation37 An in-depth review of these exact processes and mechanisms is beyond the scope of this review, but the processes relevant to sarcopenia in COPD are briefly discussed and visualized in .
Figure 1 Pathophysiology of sarcopenia in COPD: Age and COPD can be considered as initial determinants of disease specific and shared triggers (in blue) that initiate independent and interrelated pathophysiological mechanisms through alterations of multiple muscle cell signaling pathways (which have been discussed extensively by other authors such as Langen et alCitation36 and Abdulai et alCitation37). Eventually these converge on and deregulate three key processes (in orange) responsible for muscle homeostasis, leading to sarcopenia.
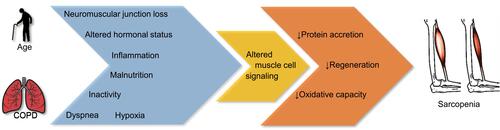
A gradual decline of muscle mass and strength starts around the age of 50 and progresses with advancing age, eventually leading to primary sarcopenia.Citation33 Furthermore, age is one of the major risk factors for chronic diseases such as COPD. With several hallmarks of aging being already present in skeletal muscle of COPD patients, including cellular senescenceCitation38 and impaired proteostasis,Citation39,Citation40 this may suggest that sarcopenia in COPD could be a manifestation of accelerated aging.
With advancing age, there is (progressive) neuromuscular junction loss, ie, a progressive denervation of motor units. The consequential loss of communication between the nervous and muscular system, eventually contributes to loss of muscle mass and strength, particularly in type II muscle fibers.Citation32–Citation34,Citation41 Furthermore, aging is accompanied by alterations in hormonal status. Age-related decreases in anabolic hormones such as growth hormone, testosterone, thyroid hormone and insulin-like growth factor have been well established and are known to contribute to loss of muscle mass and strength.Citation32 Furthermore, increased catabolic signaling through increased inflammation has long been implicated in muscle atrophy and cachexia.Citation5,Citation36 Both aging and COPD are associated with chronically elevated levels of inflammatory cytokines in the circulatory system.Citation5 Particularly in COPD, increased levels of tumor necrosis factor-α (TNF-α), C-reactive protein (CRP) and interleukin (IL)1 and IL-6 have been reported, and these cytokines have been shown to stimulate muscle protein degradation, inhibit protein synthesis as well as myogenesis in preclinical models related to COPD pathology.Citation36,Citation42,Citation43 Recently, it was demonstrated that sarcopenia was also associated with levels of systemic inflammation in COPD.Citation44 Nevertheless, to what extent low-grade systemic inflammation actually contributes to muscle wasting in stable COPD remains contradictory.Citation45–Citation47 In addition, systemic inflammation spikes during acute exacerbations of COPD and may predominantly accelerate muscle loss acutely.Citation5,Citation31,Citation36,Citation42 Moreover, in an attempt to control (mainly) local pulmonary inflammation during acute exacerbations, corticosteroid treatment is an important therapeutic cornerstone in COPD. However, administration of systemic corticosteroids has been demonstrated to affect muscle mass and consequently muscle strength.Citation48–Citation50 The extent of these negative effects vary depending on type of steroid chosenCitation51 and are also dose-dependent.Citation52,Citation53
Furthermore, COPD and aging are both associated with malnutrition, or the risk thereof.Citation54,Citation55 A deficit in energy intake results in (involuntary) weight loss and can contribute to the loss of muscle mass. In addition, specific deficits in vitamins and other micronutrients such as vitamin D are also correlated to muscle mass and function.Citation33,Citation54 Protein intake specifically is relevant in the maintenance of muscle mass. The Health ABC study demonstrated that protein intake relates to lean mass in elderly, with higher protein intake attenuating loss of lean mass over time.Citation56
Physical inactivity at any age is an important trigger loss of muscle loss, as inactivity results in a reduced stimulation of the corresponding motor units, triggering disuse-atrophy.Citation32,Citation34 Aging as well as COPD is associated with a more sedentary lifestyle and a reduced level of physical activity. In COPD (exertional) dyspnea is the main symptom limiting the patients’ activity levels. Reduced chest wall compliance with consequent dynamic hyperinflation and an increased work of breathing are additional, COPD specific, contributors to a limited exercise tolerance and determinants of limited physical activity.Citation4,Citation31 Furthermore, in some, but not all, COPD patients dyspnea is preceded or accompanied by (exertional) hypoxia. Hypoxic exposure is sufficient to induce muscle atrophyCitation57 and at the cellular level, severe hypoxia results in down-regulation of energy-consuming processes, such as protein synthesis.Citation36 Moreover, hypoxia has been shown to induce inflammatory responses and increase oxidative stress which may indirectly impact on muscle protein turnover and contribute to muscle wasting.Citation36 Recently, Debevec et al demonstrated that hypoxemia, independent of age and particularly in combination with inactivity, aggravates muscle wasting.Citation58
Each of these putative triggers of sarcopenia may act indirectly, eg, through altering the levels of key growth factors involved in muscle mass regulation or other signaling pathways, that have been comprehensively described and evaluated by other authors (such as Langen et alCitation36 and Abdulai et alCitation37).
Insulin-like growth factor 1 (IGF1) is considered essential for protein synthesis and inhibitory of protein degradation while myostatin (MSTN) is a powerful negative regulator of protein synthesis and inhibits satellite cell proliferation which is essential for muscle growth and repair, ie, its regenerative capacity.Citation36,Citation59,Citation60 During acute exacerbations, IGF1 levels are decreased and correspond with lower muscle mass in COPD patients.Citation36 Furthermore, MSTN levels are inversely correlated to muscle mass in COPD, and circulating MSTN levels are increased in sarcopenic and non-sarcopenic COPD patients compared to controls, suggesting MSTN elevation may precede sarcopenia.Citation5,Citation59,Citation61 A balance in protein turnover is essential for maintenance of muscle mass and an imbalance towards degradation results in reduced protein accretion. Increased protein turnover on the whole body level has been reported in COPD patients, as well as increased muscle protein degradation and muscle protein synthesis signaling.Citation36,Citation59 Lastly, a reduced oxidative capacity, ie, the rate at which nutrients can be oxidized in mitochondria to produce energy, influences muscle quality and function. Mitochondria are sensitive to protein and DNA damage, which worsens with age and chronic disease, leading to mitochondrial breakdown (mitophagy) and rendering the muscle less energy efficient.Citation60,Citation62 Skeletal muscle of COPD patients is indeed characterized by a loss of oxidative capacity reflected by both a loss of mitochondrial bulk and function as well as by a proportional shift from oxidative type I to glycolytic type II muscle fibers.Citation60,Citation63 This reduced proportion of oxidative type I fibers but also a reduction of oxidative enzyme activity results in a reduced oxidative capacity which has been associated with reduced muscle endurance in COPD patients.Citation5 Interestingly, more recently, Van de Bool et al demonstrated a more pronounced loss of type I fibers in sarcopenic COPD patients than in non-sarcopenic COPD patients.Citation64 Additionally, the remaining type II fibers are generally more sensitive to atrophy related triggers, implying a link between muscle quality and muscle quantity and a possible acceleration of muscle wasting by the loss of oxidative capacity.Citation60,Citation64
Assessment
In clinical practice, sarcopenia may only become obvious when serious exercise impairment develops, whereas early recognition may lead to timely and personalized treatment, resulting in improved clinical outcome. Depending on the setting and the patients’ clinical condition, either a thorough assessment of all sarcopenia domains; muscle mass, strength and physical performance, or a shorter assessment is indicated.Citation11
Screening
The SARC-F is a relatively simple, easily accessible five-item questionnaire that is currently the most popular of four existing screening tools for sarcopenia; it is also recommended by the EWGSOP2 as a screening tool for probable sarcopenia in community health care and other clinical settings.Citation11,Citation65 With its low sensitivity but high specificity, the SARC-F will most likely detect severe cases rather than low-risk patients.Citation66,Citation67 To increase its sensitivity, Barbosa-Silva et alCitation68 added calf circumference to the SARC-F questionnaire, resulting in the SARC-CalF screening tool. A recent comparative study found that the SARC-CalF was the optimal choice for screening sarcopenia in community dwelling-older adults.Citation65 Following this recent literature, the AWGS 2019 recommends the use of the SARC-CalF in their screening protocol.Citation12
Recently the serum creatinine (Cr)/serum cystatin C (CysC) ratio, also called the sarcopenia index, has been introduced.Citation69 Since Cr reflects muscle mass and CysC does not, the Cr/CysC ratio has been proposed as a surrogate marker for sarcopenia. A correlation between Cr/CysC ratio with muscle massCitation69 and adverse COPD-related outcomesCitation70 has been demonstrated. Moreover, Hirai et alCitation71 demonstrated that the Cr/CysC may serve as a surrogate marker for sarcopenia in COPD, with a cut-off of 0.71 in male patients. However, Tang et alCitation72 recently suggested that the Cr/CysC ratio could only serve as a potentially valuable biomarker to predict adverse outcomes in elderly, but not necessarily as a surrogate marker for sarcopenia, since other factors, such as chronic kidney disease,Citation73 a frequent comorbidity in COPD patients,Citation74 might also influence serum Cr levels. Therefore, although serum Cr and CysC can be easily determined in clinical practice, better validation is required before this can be applied in clinical practice.Citation72
In the search for reliable biomarkers to reflect muscle damage, urinary titin, a sarcomeric protein that is excreted in urine, has been investigated and was found to correlate with blood markers of muscle damage.Citation75 More recently, elevated levels of urinary titin were demonstrated to correlate with rectus femoris muscle atrophy in intensive care patients.Citation76 These studies suggest that urinary titin might be a useful, non-invasive potential biomarker for sarcopenia, but more research and validation is still required.
Muscle Mass
Whole body Magnetic Resonance Imaging (MRI) is usually considered as the gold standard for muscle mass assessment. MRI is a non-invasive method that does not require radiation exposure, but is limited by its need for highly trained operating personnel, lack of portability, equipment costs, availability and its long measurement duration.Citation4,Citation77 Recently, however, fat-referenced MRI has been introduced as a method to assess body composition. This six-minute MRI scanning protocol provides quantitative fat-referenced images and is significantly quicker than traditional whole-body MRI scanning. It uses a supervised automated segmentation tool that facilitates efficient segmenting of different body compartments, further reducing the processing time of analyzing a whole body data set.Citation78 Rapid MRI protocols proved to accurately quantify whole body skeletal muscle volume and body composition.Citation78–Citation80 Besides whole-body assessment, single slice MRI at the third lumbar vertebrae (L3) and mid-thigh level have been proposed as a proxy for whole-body muscle mass. Schweitzer et al found that a single slice MRI at the L3 level was the optimal site to assess total skeletal muscle mass and adipose tissue in healthy, middle-aged subjects.Citation81 Furthermore, a single MRI image at the mid-femur level has also shown good estimation of skeletal muscle mass and correlations with clinical sarcopenia criteria.Citation82
Computed Tomography (CT) provides a three-dimensional, high-resolution image of the scanned area. CT scanning is an accurate way to measure muscle mass, but is limited by its consequential radiation exposure, equipment costs, lack of portability and need for trained operating personnel.Citation78 The radiation exposure accompanying CT scans makes whole-body CT to assess muscle mass less favorable. Nevertheless, because chest CT scanning is increasingly considered in staging and follow-up of COPD, it provides the possibility of opportunistic imaging and the use of single-slice analysis to assess muscle mass.Citation83 Cross-sectional imaging of the psoas muscle in the lumbar spine region, all abdominal muscles on the lumbar spine level or at the mid-thigh level are the most commonly used methods to assess muscle mass through CT scanning, with a preference for imaging at the lumbar spine level over thigh muscle imaging.Citation84 When measuring CT-derived skeletal muscle mass, the L3 vertebra level is the most commonly used and considered as reference method of cross-sectional analysis of skeletal muscle.Citation85,Citation86 However, most chest-CTs as obtained in regular COPD care, do not reach beyond the first lumbar level (L1), leaving the question if CT-derived pectoralis muscle area, erector spinae muscle area or muscle area at the L1 level can serve as an alternative. In a study by McDonald et al CT-derived pectoralis muscle area showed to be associated with COPD disease severity and to provide information on COPD morbidity.Citation87 Tanimura et al later demonstrated that CT-derived erector spinae muscle area is correlated with clinical parameters of COPD and all-cause mortality,Citation88 Furthermore, Sanders et al showed that muscle mass at L1 can substitute the L3 level assessment, but pectoralis muscle to a lesser extent.Citation85 Consensus on the exact cut-off points that should be used when determining muscle cross-sectional area are yet to be determined, as current cut-off points vary between authors.Citation86
Dual-energy X-ray absorptiometry (DXA) is another commonly used imaging method to assess body composition as it allows for lean mass, fat mass and bone mineral content measurements.Citation84 This method is non-invasive, quick to perform and relatively less expensive than MRI or CT. It also has significantly lower radiation exposures compared to CT scans.Citation77 Whilst DXA proved to estimate muscle mass reasonably well, it is however also known that different brands and different patient circumstances (like hydration status, height and weight) introduce variability, which complicates comparison of data.Citation11,Citation77,Citation89 In regular care of COPD patients, DXA might be favorable since it not only assesses body composition, but also provides opportunity to screen for osteoporosis.Citation64 Combined screening is convenient, since besides sarcopenia, osteoporosis is a prevalent comorbidity amongst COPD patients.Citation90 Recently, Ofenheimer et al published DXA-specific age and sex-related reference values for body composition parameters.Citation91
Bioelectrical Impedance Analysis (BIA) estimates total body water based on whole-body electrical conductivity and uses a conversion equation to estimate fat mass and fat-free mass.Citation11 Because of its portability and affordability, BIA might have some clinically relevant advantages over DXA scan. However, BIA measurements are highly sensitive to patient conditions such as hydration status and fasting versus fed conditions, which contributes to the reported inconsistent accuracy of the method.Citation89,Citation92 To minimize discrepancies between measurements, specific conversion equations and reference values for specific populations, including COPD patients and elderly, have been developed.Citation11,Citation93 Furthermore, BIA reference values may also need to be corrected for height, weight or BMI as published by Franssen et alCitation94 and Lee et al.Citation95
Anthropometric measures such as calf circumference or mid-arm muscle circumference are possibly the most accessible, affordable and portable manners of muscle mass assessment. Calf circumference (cut-off point <31 cm) has been shown to reflect health and nutritional status and predict performance and survival in older people.Citation96 Training is required to properly assess anthropometrics, but even after training, they have low precision in obese subjects and older subjects.Citation92 Therefore, as advised by EWGSOP2, they can be used as a diagnostic proxy in settings where no other methods are available.Citation11
Muscle Strength
Quadriceps strength, the maximal contraction force that can be produced, measured by a leg dynamometer, is a reliable method to assess lower limb muscle strength.Citation5 Isometric quadriceps strength measurements proved to predict mortalityCitation5 and to have a high test-re-test reliability and to be responsive to exercise training in COPD patients.Citation97 There is no universal cut-off for weak quadriceps strength, but Canavan et al determined clinically relevant cut-off points for isometric maximal voluntary force normalized to heigth squared as 8.30 kg/m2 for men and 5.99 kg/m2 for women, using a hydraulic measurement.Citation98 The hydraulic dynamometers that reliably assess leg muscle strength are not portable and could therefore limit clinical applicability. A hand-held dynamometer is portable and a feasible proxy in clinical settings, however it has been demonstrated that hand-held measurement is less accurate than traditional, hydraulic measurement methods.Citation99
Handgrip strength measurement, measured by a calibrated dynamometer, is a simple, inexpensive and very portable method of assessing muscle strength and therefore a valuable surrogate for more complicated or less portable measures of limb muscle strength such as quadriceps strength.Citation5,Citation11,Citation100 Handgrip strength carries limited to no ventilatory load and is therefore also feasible in more severe COPD disease stages. This easy to use, portable tool is advised for routine use, both in hospital as in community health-care settings and has been validated in COPD patients.Citation101 Exact cut-off values for low handgrip strength vary between studies and are dependent of appropriate reference populations. Gender, age and height specific cut-off values were published by Spruit et al,Citation102 whereas gender specific cut-off points have been proposed by EWGSOP2: <27 kg for men and <16 kg for women, and AWGS 2019: <28 kg for men and <18 kg for women.Citation11,Citation12,Citation103–Citation105
The chair stand test can assess both leg muscle strength and functionality, since getting up from a chair reflects common daily activity. The test measures the amount of time a patient needs to rise five times from seated position, without using their arms. A test-time of more than 17 seconds for five rises was found to identify a high risk of functional limitation within the Health ABC study.Citation106 However, the AWGS 2019 proposes a cut of point of >12 seconds as this correlates with their definition of impaired gait speed.Citation12 Recently, a cut-off of 10.88 seconds was found to have good sensitivity and specificity for sarcopenia screening in COPD patients specifically.Citation107 The chair stand test proved reliable in COPD patients, correlates with exercise capacity, quadriceps strength, and is responsive to rehabilitation in COPD.Citation11,Citation108 Because the test requires limited space and equipment, it is feasible in most health care and research settings.
Physical Performance
Low gait speed is used as a diagnostic measure for sarcopenia, but definition of low gait speed ranges from <0.8m/s (EWGSOP2)Citation11 to <1m/s (AWGS 2019)Citation12 between authors and diagnostic criteria. Low gait speed proved to be prognostic of poor physical performance, adverse outcomes like disability, cognitive impairment, falls and mortality.Citation9,Citation11 Combined with assessment of handgrip strength, it proved to be sensitive and specific as a proxy for the “frailty phenotype”.Citation109 Assessment of gait speed requires a flat floor where a subjects time to walk 4 meters can be timed (either manually or instrumentally with sensors). This makes it feasible for almost all care and research settings. In COPD patients, gait speed is responsive to pulmonary rehabilitation, with greatest responsiveness in poorly functioning, frail patients for whom longer tests are more difficult to perform.Citation110 For standardization purposes, a single cut off point of <0.8m/s, in accordance with the EWGSOP2 is suggested.
The Short Physical Performance Battery (SPPB) consists of gait speed, a balance test and a chair stand test. This short (approximately 10 minute) test battery has a maximum score of 12 points. A meta-analysis by Pavasini et alCitation111 demonstrated SPPB scores of 7–9 to predict increased all-cause mortality. EWGSOP 2 defines a score of <8 poor physical performance whilst AWGS 2019 defines a score of <9 impairedCitation11,Citation77 and even more recently, a score of <10 on the SPPB was proposed as a screening tool for sarcopenia in COPD patients.Citation107
The Timed Up and Go (TUG) test times how long it takes patients getting up from a chair, walk to a 3 m away marker, turn, walk back and sit down again.Citation11 Time needed to complete the test increases with age, cut-off values of 8.1–11.3 seconds, depending on age have been reported.Citation112 However, as an age independent cut-off a time of >10 seconds has also been reported.Citation95 The TUG test proved to be valid and responsive in COPD patients and was not associated with airflow limitation.Citation113 Worse performance on the test is indicative for poor health outcomes (functional dependency, mortality) and increases the chance for COPD patients to present with sarcopenia.Citation95,Citation114–Citation116 The limited space and materials required to perform the test make it feasible in most health-care settings.
The 6-minute walk test is commonly used to objectively assess functional capacity (for rehabilitation) in COPD patients. It requires patients to walk as far as possible along a stretched course for 6 minutes and has a good reliability in COPD patients.Citation117 The 6-min walk test (cut-off <350 m) predicts mortality and morbidity in COPD patients and can therefore be used to stratify patients to functional capacity.Citation118,Citation119 It does not require specialized equipment, but does need a 30 meters long corridor, which might make it more feasible in research settings than in everyday clinical assessment.
Interventions
As previously discussed, the pathological processes of sarcopenia and COPD are interconnected. An integrated approach in the management of both conditions, targeting their underlying pathophysiological mechanisms, would thus be preferable. We will therefore discuss intervention modalities primarily targeting sarcopenia in COPD patients, as well as therapeutic interventions primarily targeting COPD lung pathology that also reported effects on sarcopenia measures.
Interventions Primarily Targeting Sarcopenia in COPD
Exercise Training
Physical activity and exercise training are key in maintaining muscle mass and strength. Therefore, targeted exercise training is the hallmark of sarcopenia treatment as well as in pulmonary rehabilitation (PR) programs offered to COPD patients.Citation120 The efficacy of PR in relieving dyspnea and fatigue in COPD has been convincingly demonstrated over the years, however exact composition of PR programs varies widely and the essential components, optimal intensity and duration of the exercise training remain to be determined and personalized.Citation121 Jones et al demonstrated that the presence of sarcopenia in COPD does not affect the response to PR and in some patients PR might lead to a resolution of sarcopenia.Citation7
General principles of exercise training are no different in COPD patients from healthy controls or even athletes, although the physiological response might be altered in COPD patients.Citation5,Citation122 Aerobic exercise or endurance training in COPD patients specifically improves exercise tolerance, breathlessness, exercise induced hyperinflation and stimulates a muscle fiber shift back to more oxidative type I fibers, improving mitochondrial capacity and thus efficiency.Citation123–Citation125 Interval training is a variation to continuous endurance training, with short periods of high-intensity exercise alternated with periods of lower intensity or rest. This might be a valuable alternative for patients who have difficulty tolerating longer periods of continuous exercise or achieving the targeted intensity because of dyspnea or leg muscle fatigue.Citation5 Interval training compared to continuous exercise resulted in lower levels of breathlessness and less dynamic hyperinflation in COPD patients,Citation121,Citation126 whilst it proved to be just as effective as endurance training in improving exercise tolerance.Citation124 Additionally, progressive strength or resistance training relies on progressively heavy resistance counteracting muscular contraction. It is a well-established method to improve skeletal muscle mass and strength, with similar improvements demonstrated in COPD patients compared to healthy controls.Citation123,Citation127 Resistance training consistently increased muscle mass, and was more effective in counteracting muscle atrophy than endurance training in COPD.Citation122 Therefore, a combination of both endurance or interval training and resistance training, as commonly applied in PR, might be optimal to improve muscle mass, strength and endurance in sarcopenic COPD patients. Theoretically, oxygen supplementation during exercise would enable a higher training intensity and thus would yield better improvements in muscle mass and strength. However, recent studies are heterogeneous in their results; some show improved exercise capacity and breathlessness but not muscle mass, whereas other studies show no difference in training results, irrespective of oxygen supplementation.Citation128,Citation129 Neuromuscular electrical stimulation (NMES) is a passive form of exercise training where electrodes placed on the skin apply electrical stimulation of isolated muscles, evoking involuntary contractions. In a Cochrane review, Jones et al conclude that this passive form of muscle stimulation might be an effective treatment for muscle weakness in adults with advanced progressive disease.Citation130 The fact that this is a portable, non-invasive and passive intervention, which does not evoke dyspnea, makes it suitable for even severely impaired and dyspneic COPD patients, whom might not tolerate active forms of exercise interventions.Citation124 Several studies show that NMES improves exercise tolerance, walking distance, FFM and exercise induced dyspnea, but not peak power in (severely dyspneic) COPD patients. The extent of its efficacy in increasing muscle mass and strength and its possible role as adjuvant or addition to regular exercise warrants further investigation.Citation124,Citation130–Citation132
Nutrition
Poor dietary intake resulting in low intake of essential nutrients is highly prevalent among the elderly population and contributes to weight loss, sarcopenia and frailty.Citation133 Unintended weight loss is also common in COPD patients.Citation54,Citation134 In contrast to primary sarcopenia, malnutrition in COPD is associated with elevated energy- and probably also protein-requirements due to hypermetabolismCitation135 and elevated whole body protein turnover.Citation136 Emphysematous patients with severe airflow obstruction and hyperinflation and those suffering from chronic respiratory failure are at increased risk for weigh loss due to adverse effects on dietary intake. Furthermore during acute exacerbations appetite and dietary intake are often decreased.Citation35,Citation54 Adequate protein intake is of major importance when following an exercise program to enhance protein anabolism and facilitate muscle repair in health and disease. A reduced anabolic response to protein intake and a hypermetabolic state may further enhance protein requirements of sarcopenic elderly and in particular COPD patients.Citation54,Citation133 Furthermore, the Health ABC study demonstrated that high dietary protein intake in elderly was associated with less muscle mass loss over time, providing a strong rationale for adequate protein intake in sarcopenic elderly.Citation56 Consensus on the optimal level of protein intake in sarcopenia management is yet to be determined; current recommendations vary substantially between 1.2 up to 2.0 g/kg.Citation137 When supplementing protein, branched-chain amino acids (BCAAs), such as leucine and its metabolite beta-hydroxy-beta-methylbutyrate (HMB), seem to be of particular importance to increasing FFM, muscle mass and strength.Citation138
Polyunsaturated fatty-acids (PUFA’s), more specifically omega-3 PUFA’s have been implicated to modulate (systemic) inflammatory processes, to boost muscle mitochondrial metabolism and to positively affect protein synthesis and exercise capacity.Citation5,Citation54,Citation138–Citation141 Positive effects of PUFA supplementation on exercise capacity in COPD has indeed been demonstrated,Citation142 adding to the rationale for supplementation of PUFA’s next to sufficient protein intake to prevent or treat sarcopenia in COPD, but further evidence from clinical trials is needed.Citation143
Vitamin D deficiency is common in COPD patients, especially in elderly patients.Citation54,Citation144 Vitamin D influences muscle metabolism and current literature does support the hypothesis that vitamin D supplementation might is beneficial in sarcopenia treatment. However, according to the recent review by Remelli et al, the exact role of vitamin D supplementation in the prevention of sarcopenia remains to be determined.Citation145 Vitamin D supplementation was demonstrated to reduce the rate of COPD exacerbations, which indirectly might slow down the course of sarcopenia in these patients.Citation146
Studies are diverse in their exact strategies, but a combination of exercise training with additional supplementation of different combinations of dietary protein (whey), PUFA’s and vitamin D are reported to maintain or improve FFM in both primary sarcopenia and sarcopenic COPD patients.Citation56,Citation141,Citation147,Citation148
Pharmacology
A combination of exercise therapy and nutritional interventions might not always be feasible in COPD patients with severe respiratory impairment or sarcopenia. Pharmacologic approaches could therefore be an important third party in a multimodal treatment plan for sarcopenia.
Anabolics
Both in young healthy adults, as in sarcopenic patients, testosterone or anabolic steroid treatment proved to increase muscle mass and reduce fat mass.Citation124 Testosterone administration in COPD patients, alone or in conjunction with exercise, was associated with reduced hospitalization, increased FFM and muscle strength, but did not enhance exercise capacity,Citation122,Citation149,Citation150 Similarly, intramuscular anabolic steroid injection in the form of nandrolone decanoate (ND) increased FFM without further improvement of exercise capacity compared to control when provided during PR, although exercise capacity was reported to increase by ND in the subgroup of patients on glucocorticoid medication.Citation151 Although testosterone induced an increase in FFM, whether or not effects can be sustained after cessation of supplementation remains to be determined.Citation152 Significant, usually dose dependent, side effects of testosterone administration (such as cardiovascular, gastro-intestinal and endocrine) have been well-documented and warrant caution when administering testosterone.Citation149
Growth hormone (GH) promotes muscle growth through several pathways. Despite contradicting results, studies over the years have shown potential benefit of GH supplementation as it increased body weight, muscle mass and respiratory muscle strength in COPD patients.Citation5,Citation153 Importantly, as with testosterone, GH supplementation is associated with important, negative side effects (such as cardiovascular complications and insulin resistance) that raise serious concern for clinical practice.Citation154
Ghrelin stimulates appetite and feeding. Preventing weight loss by stimulating appetite could be a desired effect in sarcopenia treatment and in those COPD patients with reduced appetite and insufficient calorie intake.Citation154 In COPD patients, improvement of exercise capacity following ghrelin supplementation has been reported,Citation124,Citation155 although not all studies demonstrated an increase in muscle strength, or functionality.Citation124
Myostatin Inhibition
As previously discussed, elevated levels of MSTN, a potent negative regulator of muscle mass, have been found in COPD. Inhibition of myostatin or antagonizing its receptor (activinIIR) consequently leads to muscle growth.Citation124,Citation154 Indeed Bimagrumab, an activinIIR antagonist proved to be of particular interest in sarcopenia treatment, as its administration to sarcopenic, community dwelling elderly improved muscle mass, strength and mobility.Citation156 Strikingly, however, in COPD patients an increase in muscle mass, but no improvement in functional capacity after Bimagrumab treatment has been demonstrated.Citation157 This suggests that improvement in muscle mass alone (through anabolic agents) may not be sufficient to improve physical performance, but also that COPD specific factors might be impacting the physical performance in parallel to affecting muscle mass.
Interventions Primarily Targeting the Respiratory System with Potential Benefits for Muscle in COPD
Smoking Cessation
Smoking cessation is the most effective intervention that can halt or slow down the progression of COPD.Citation158,Citation159 Besides its detrimental effects on lung tissue and function, evidence indicates cigarette smoke also impairs muscle mitochondrial function and muscle mass,Citation160 implying that smoking cessation could have beneficial effects on muscle function. Indeed, recently a study in mice demonstrated that short-term smoking cessation reversed smoking-induced mitochondrial dysfunction, limb muscle mass loss and diaphragm muscle atrophy.Citation161
Bronchodilators
Optimizing dyspnea treatment and reducing symptoms with bronchodilators can enhance exercise capacity.Citation162,Citation163 In addition, beta2-agonists, have been demonstrated to also exert anabolic effects on skeletal muscle.Citation164,Citation165 A recent study indicated increased skeletal muscle protein synthesis and breakdown after resistance exercise when administering oral salbutamol in young men, suggesting that these agents may contribute to muscle growth through direct anabolic actions and indirectly by facilitating increased muscle workload during PR by relieving dyspnea.Citation166
Lung Volume Reduction Interventions
Lung volume reduction surgery (LVRS) and bronchoscopic lung volume reduction (BLVR) through endobronchial valve (EBV) placement not only improve respiratory mechanics and efficiency, but also proved to enhance exercise capacity and body composition in terms of increased FFM.Citation167,Citation168 It is yet unclear if this effect reflects direct modulation of muscle, or whether this is secondary to a reduction in dyspnea symptoms, resulting in increased physical activity and subsequent preservation or recovery of muscle mass.
Conclusion and Perspective
The current literature clearly shows that both age-related and disease-specific determinants contribute to alterations in physiological processes involved in muscle maintenance leading to sarcopenia in COPD. Not only screening for sarcopenia itself, using validated tools, but also determining the presence of the above-mentioned triggers of sarcopenia is needed as an integral part of the diagnostic work-up of COPD patients at risk, to allow a timely and more personalized sarcopenia management approach. The choice of sarcopenia (severity) assessment methods may depend on available resources of the care setting (eg, general practice, hospital or rehabilitation centre). Furthermore, when determining physical performance for sarcopenia assessment, ventilatory impairment needs to be considered as confounding limiting factor to ensure one really measures the sarcopenia-related physical performance. In COPD, quadriceps strength has been strongly correlated to mortality and pulmonary function,Citation5,Citation169 but since the devices to reliably assess leg muscle strength are not portable, systematically assessing handgrip strength in all COPD patients, as proposed by Benz et al, can be a first step in screening for sarcopenia in COPD patients.Citation4 The TUG test, providing information on both strength and functionality is easy to perform in all settings and proved not to be associated with airflow limitation.Citation113 Next to available body composition assessment tools such as BIA, methods that may already be incorporated in standard care, such as chest CT-scans for pulmonary screening or a DEXA scan for osteoporosis screening can be optimized in a cost effective way for simultaneous determination of muscle mass.
Guided by objective assessment of physical performance, lung function and exercise induced symptoms, most prescribed exercise programs as part of PR are nowadays already personalized to the individual. More attention is however needed towards feasibility and efficacy of maintaining adequate exercise and physical activity interventions in daily life and to overcome challenges such as motivation, access to, and availability of those interventions.Citation170 Current generic recommendations for adequate nutrition to combat sarcopenia are total daily energy intake of 25–30 kcal/kg body weight, protein intake 1.0–1.2 g/kg per day and vitamin D supplementation if lower than 30 ng/mL.Citation170 Energy and protein requirements probably are even higher in hypermetabolic sarcopenic COPD patients prone to cachexia. Longitudinal assessment of body weight and muscle mass as an integrated part of the regular COPD care visits or telemonitoring allows timely incorporation of personalized dietary counseling and deployment of nutritional supplements. In the meantime ongoing research will reveal if additional pharmacological interventions or specific nutritional modulation may enhance feasibility, efficacy and sustainability of personalized sarcopenia management in a cost-effective manner. Combined, this is expected to provide personalized strategies for both prevention and treatment of sarcopenia for a large proportion of COPD patients.
Abbreviations
COPD, chronic obstructive pulmonary disease; EWGSOP, European Working Group on Sarcopenia in Older People; AWGS, Asian Working Group for Sarcopenia; FFM, fat-free mass; TNF- α, tumor necrosis factor-α; IGF1, insulin-like growth factor1; MSTN, myostatin; Cr/CysC, creatinine (Cr)/serum cystatin C (CysC) ratio; MRI, magnetic resonance imaging; L3, third lumbar vertebrae; CT, computed tomography; DXA, dual-energy X-ray absorptiometry; BIA, bioelectrical impedance analysis; SPPB, short physical performance battery; TUG, timed up and go; PR, pulmonary rehabilitation; NMES, neuromuscular electrical stimulation; BCAAs, branched-chain amino acids; HMB, beta-hydroxy-beta-methylbutyrate; PUFA’s, polyunsaturated fatty-acids; ND, nandrolone decanoate; GH, growth hormone; LVRS, lung volume reduction surgery; BLVR, bronchoscopic lung volume reduction; EBV, endobronchial valve.
Disclosure
The authors report no conflicts of interest in this work.
References
- Rosenberg IH. Sarcopenia: origins and clinical relevance. J Nutr. 1997;127(5 Suppl):990S–991S. doi:10.1093/jn/127.5.990S
- Schols AM, Mostert R, Soeters PB, Wouters EF. Body composition and exercise performance in patients with chronic obstructive pulmonary disease. Thorax. 1991;46(10):695–699. doi:10.1136/thx.46.10.695
- Schols AM, Mostert R, Soeters PB, Greve LH, Wouters EF. Nutritional state and exercise performance in patients with chronic obstructive lung disease. Thorax. 1989;44(11):937–941. doi:10.1136/thx.44.11.937
- Benz E, Trajanoska K, Lahousse L, et al. Sarcopenia in COPD: a systematic review and meta-analysis. Eur Respir Rev. 2019;28(154):190049. doi:10.1183/16000617.0049-2019
- Maltais F, Decramer M, Casaburi R, et al. An official American thoracic society/European respiratory society statement: update on limb muscle dysfunction in chronic obstructive pulmonary disease. Am J Respir Crit Care Med. 2014;189(9):e15–e62. doi:10.1164/rccm.201402-0373ST
- Patel AR, Patel AR, Singh S, Singh S, Khawaja I. Global initiative for chronic obstructive lung disease: the changes made. Cureus. 2019;11(6):e4985.
- Jones SE, Maddocks M, Kon SSC, et al. Sarcopenia in COPD: prevalence, clinical correlates and response to pulmonary rehabilitation. Thorax. 2015;70(3):213–218. doi:10.1136/thoraxjnl-2014-206440
- Papadopoulou SK, Tsintavis P, Potsaki P, Papandreou D. Differences in the prevalence of sarcopenia in community-dwelling, nursing home and hospitalized individuals. a systematic review and meta-analysis. J Nutr Health Aging. 2020;24(1):83–90. doi:10.1007/s12603-019-1267-x
- Fielding RA, Vellas B, Evans WJ, et al. Sarcopenia: an undiagnosed condition in older adults. Current consensus definition: prevalence, etiology, and consequences. International working group on sarcopenia. J Am Med Dir Assoc. 2011;12(4):249–256. doi:10.1016/j.jamda.2011.01.003
- Studenski SA, Peters KW, Alley DE, et al. The FNIH sarcopenia project: rationale, study description, conference recommendations, and final estimates. J Gerontol a Biol Sci Med Sci. 2014;69(5):547–558. doi:10.1093/gerona/glu010
- Cruz-Jentoft AJ, Bahat G, Bauer J, et al. Sarcopenia: revised European consensus on definition and diagnosis. Age Ageing. 2019;48(1):16–31. doi:10.1093/ageing/afy169
- Chen LK, Woo J, Assantachai P, et al. Asian working group for sarcopenia: 2019 consensus update on sarcopenia diagnosis and treatment. J Am Med Dir Assoc. 2020;21(3):300–307e302. doi:10.1016/j.jamda.2019.12.012
- Cruz-Jentoft AJ, Baeyens JP, Bauer JM, et al. Sarcopenia: European consensus on definition and diagnosis: report of the European working group on sarcopenia in older people. Age Ageing. 2010;39(4):412–423. doi:10.1093/ageing/afq034
- Evans WJ, Morley JE, Argiles J, et al. Cachexia: a new definition. Clin Nutr. 2008;27(6):793–799. doi:10.1016/j.clnu.2008.06.013
- Fearon K, Strasser F, Anker SD, et al. Definition and classification of cancer cachexia: an international consensus. Lancet Oncol. 2011;12(5):489–495. doi:10.1016/S1470-2045(10)70218-7
- Rolland Y, Van Kan GA, Gillette-Guyonnet S, Vellas B. Cachexia versus sarcopenia. Curr Opin Clin Nutr Metab Care. 2011;14(1):15–21. doi:10.1097/MCO.0b013e328340c2c2
- Trajanoska K, Schoufour JD, Darweesh SK, et al. Sarcopenia and its clinical correlates in the general population: the rotterdam study. J Bone Miner Res. 2018;33(7):1209–1218. doi:10.1002/jbmr.3416
- Sepulveda-Loyola W, Osadnik C, Phu S, Morita AA, Duque G, Probst VS. Diagnosis, prevalence, and clinical impact of sarcopenia in COPD: a systematic review and meta-analysis. J Cachexia Sarcopenia Muscle. 2020. doi:10.1002/jcsm.12600
- Cruz-Jentoft AJ, Landi F, Schneider SM, et al. Prevalence of and interventions for sarcopenia in ageing adults: a systematic review. Report of the international sarcopenia initiative (EWGSOP and IWGS). Age Ageing. 2014;43(6):748–759. doi:10.1093/ageing/afu115
- Shafiee G, Keshtkar A, Soltani A, Ahadi Z, Larijani B, Heshmat R. Prevalence of sarcopenia in the world: a systematic review and meta- analysis of general population studies. J Diabetes Metab Disord. 2017;16(1):21. doi:10.1186/s40200-017-0302-x
- Vestbo J, Prescott E, Almdal T, et al. Body mass, fat-free body mass, and prognosis in patients with chronic obstructive pulmonary disease from a random population sample: findings from the copenhagen city heart study. Am J Respir Crit Care Med. 2006;173(1):79–83. doi:10.1164/rccm.200506-969OC
- Davalos-Yerovi V, Marco E, Sanchez-Rodriguez D, et al. Sarcopenia according to the revised European consensus on definition and diagnosis (EWGSOP2) criteria predicts hospitalizations and long-term mortality in rehabilitation patients with stable chronic obstructive pulmonary disease. J Am Med Dir Assoc. 2019;20(8):1047–1049. doi:10.1016/j.jamda.2019.03.019
- Schols AM, Broekhuizen R, Weling-Scheepers CA, Wouters EF. Body composition and mortality in chronic obstructive pulmonary disease. Am J Clin Nutr. 2005;82(1):53–59. doi:10.1093/ajcn/82.1.53
- Puhan MA, Siebeling L, Zoller M, Muggensturm P, Ter Riet G. Simple functional performance tests and mortality in COPD. Eur Respir J. 2013;42(4):956–963. doi:10.1183/09031936.00131612
- Leong DP, Teo KK, Rangarajan S, et al. Prognostic value of grip strength: findings from the prospective urban rural epidemiology (PURE) study. Lancet. 2015;386(9990):266–273. doi:10.1016/S0140-6736(14)62000-6
- Hakamy A, Bolton CE, Gibson JE, McKeever TM. Risk of fall in patients with COPD. Thorax. 2018;73(11):1079–1080. doi:10.1136/thoraxjnl-2017-211008
- Adas-Okuma MG, Maeda SS, Gazzotti MR, et al. COPD as an independent risk factor for osteoporosis and fractures. Osteoporos Int. 2020;31(4):687–697. doi:10.1007/s00198-019-05235-9
- Lee DW, Choi EY, Wu Q. Sarcopenia as an independent risk factor for decreased BMD in COPD patients: Korean national health and nutrition examination surveys IV and V (2008–2011). PLoS One. 2016;11(10):e0164303. doi:10.1371/journal.pone.0164303
- Beaudart C, Biver E, Reginster J-Y, et al. Validation of the SarQoL®, a specific health-related quality of life questionnaire for Sarcopenia. J Cachexia Sarcopenia Muscle. 2017;8(2):238–244. doi:10.1002/jcsm.12149
- Suleymanova A, Baranova I, Brodskaya O, et al. Quality of life in COPD patients with sarcopenia. Eur Respir J. 2019;54.
- Jaitovich A, Barreiro E. Skeletal muscle dysfunction in chronic obstructive pulmonary disease. what we know and can do for our patients. Am J Respir Crit Care Med. 2018;198(2):175–186. doi:10.1164/rccm.201710-2140CI
- Dhillon RJ, Hasni S. Pathogenesis and management of sarcopenia. Clin Geriatr Med. 2017;33(1):17–26. doi:10.1016/j.cger.2016.08.002
- Rolland Y, Czerwinski S. Sarcopenia: its assessment, etiology, pathogenesis, consequences and future perspectives. J Nutr Health Aging. 2008;12(7):433–450.
- Kwan P. Sarcopenia, a neurogenic syndrome? J Aging Res. 2013;2013:791679. doi:10.1155/2013/791679
- Perrot L, Greil A, Boirie Y, et al. Prevalence of sarcopenia and malnutrition during acute exacerbation of COPD and after 6 months recovery. Eur J Clin Nutr. 2020;74(11):1556–1564. doi:10.1038/s41430-020-0623-6
- Langen RC, Gosker HR, Remels AH, Schols AM. Triggers and mechanisms of skeletal muscle wasting in chronic obstructive pulmonary disease. Int J Biochem Cell Biol. 2013;45(10):2245–2256. doi:10.1016/j.biocel.2013.06.015
- Abdulai RM, Jensen TJ, Patel NR, et al. Deterioration of limb muscle function during acute exacerbation of chronic obstructive pulmonary disease. Am J Respir Crit Care Med. 2018;197(4):433–449. doi:10.1164/rccm.201703-0615CI
- Theriault ME, Pare ME, Maltais F, Debigare R. Satellite cells senescence in limb muscle of severe patients with COPD. PLoS One. 2012;7(6):e39124. doi:10.1371/journal.pone.0039124
- Barreiro E, Salazar-Degracia A, Sancho-Munoz A, Gea J. Endoplasmic reticulum stress and unfolded protein response profile in quadriceps of sarcopenic patients with respiratory diseases. J Cell Physiol. 2019;234(7):11315–11329. doi:10.1002/jcp.27789
- Guo Y, Gosker HR, Schols AM, et al. Autophagy in locomotor muscles of patients with chronic obstructive pulmonary disease. Am J Respir Crit Care Med. 2013;188(11):1313–1320. doi:10.1164/rccm.201304-0732OC
- Gonzalez-Freire M, de Cabo R, Studenski SA, Ferrucci L. The neuromuscular junction: aging at the crossroad between nerves and muscle. Front Aging Neurosci. 2014;6:208. doi:10.3389/fnagi.2014.00208
- Remels AH, Gosker HR, Langen RC, Schols AM. The mechanisms of cachexia underlying muscle dysfunction in COPD. J Appl Physiol (1985). 2013;114(9):1253–1262. doi:10.1152/japplphysiol.00790.2012
- Eid AA, Ionescu AA, Nixon LS, et al. Inflammatory response and body composition in chronic obstructive pulmonary disease. Am J Respir Crit Care Med. 2001;164(8 Pt 1):1414–1418. doi:10.1164/ajrccm.164.8.2008109
- Byun MK, Cho EN, Chang J, Ahn CM, Kim HJ. Sarcopenia correlates with systemic inflammation in COPD. Int J Chron Obstruct Pulmon Dis. 2017;12:669–675. doi:10.2147/COPD.S130790
- Crul T, Spruit MA, Gayan-Ramirez G, et al. Markers of inflammation and disuse in vastus lateralis of chronic obstructive pulmonary disease patients. Eur J Clin Invest. 2007;37(11):897–904. doi:10.1111/j.1365-2362.2007.01867.x
- Barreiro E, Schols AM, Polkey MI, et al. Cytokine profile in quadriceps muscles of patients with severe COPD. Thorax. 2008;63(2):100–107. doi:10.1136/thx.2007.078030
- Larsson L, Ansved T. Effects of long-term physical training and detraining on enzyme histochemical and functional skeletal muscle characteristic in man. Muscle Nerve. 1985;8(8):714–722. doi:10.1002/mus.880080815
- Decramer M, Lacquet LM, Fagard R, Rogiers P. Corticosteroids contribute to muscle weakness in chronic airflow obstruction. Am J Respir Crit Care Med. 1994;150(1):11–16. doi:10.1164/ajrccm.150.1.8025735
- Hanada M, Sakamoto N, Ishimatsu Y, et al. Effect of long-term treatment with corticosteroids on skeletal muscle strength, functional exercise capacity and health status in patients with interstitial lung disease. Respirology. 2016;21(6):1088–1093. doi:10.1111/resp.12807
- Minetto MA, Qaisar R, Agoni V, et al. Quantitative and qualitative adaptations of muscle fibers to glucocorticoids. Muscle Nerve. 2015;52(4):631–639. doi:10.1002/mus.24572
- Fappi A, Neves JC, Sanches LN, et al. Skeletal muscle response to deflazacort, dexamethasone and methylprednisolone. Cells. 2019;8(5):406. doi:10.3390/cells8050406
- Koerts-de Lang E, Schols AM, Rooyackers OE, Gayan-Ramirez G, Decramer M, Wouters EF. Different effects of corticosteroid-induced muscle wasting compared with undernutrition on rat diaphragm energy metabolism. Eur J Appl Physiol. 2000;82(5–6):493–498. doi:10.1007/s004210000231
- Pouw EM, Koerts-de Lang E, Gosker HR, et al. Muscle metabolic status in patients with severe COPD with and without long-term prednisolone. Eur Respir J. 2000;16(2):247–252. doi:10.1034/j.1399-3003.2000.16b11.x
- Schols AM, Ferreira IM, Franssen FM, et al. Nutritional assessment and therapy in COPD: a European respiratory society statement. Eur Respir J. 2014;44(6):1504–1520. doi:10.1183/09031936.00070914
- Beaudart C, Sanchez-Rodriguez D, Locquet M, Reginster JY, Lengele L, Bruyere O. Malnutrition as a strong predictor of the onset of sarcopenia. Nutrients. 2019;11(12):2883. doi:10.3390/nu11122883
- Houston DK, Nicklas BJ, Ding J, et al. Dietary protein intake is associated with lean mass change in older, community-dwelling adults: the health, aging, and body composition (health ABC) study. Am J Clin Nutr. 2008;87(1):150–155.
- de Theije CC, Langen RC, Lamers WH, Schols AM, Kohler SE. Distinct responses of protein turnover regulatory pathways in hypoxia- and semistarvation-induced muscle atrophy. Am J Physiol Lung Cell Mol Physiol. 2013;305(1):L82–91. doi:10.1152/ajplung.00354.2012
- Debevec T, Ganse B, Mittag U, Eiken O, Mekjavic IB, Rittweger J. Hypoxia aggravates inactivity-related muscle wasting. Front Physiol. 2018;9:494. doi:10.3389/fphys.2018.00494
- Kneppers AEM, Langen RCJ, Gosker HR, et al. Increased myogenic and protein turnover signaling in skeletal muscle of chronic obstructive pulmonary disease patients with sarcopenia. J Am Med Dir Assoc. 2017;18(7):637 e631–637 e611. doi:10.1016/j.jamda.2017.04.016
- Sanders KJ, Kneppers AE, van de Bool C, Langen RC, Schols AM. Cachexia in chronic obstructive pulmonary disease: new insights and therapeutic perspective. J Cachexia Sarcopenia Muscle. 2016;7(1):5–22. doi:10.1002/jcsm.12062
- Ju C-R, Chen R-C. Serum myostatin levels and skeletal muscle wasting in chronic obstructive pulmonary disease. Respir Med. 2012;106(1):102–108. doi:10.1016/j.rmed.2011.07.016
- Leermakers PA, Gosker HR. Skeletal muscle mitophagy in chronic disease: implications for muscle oxidative capacity? Curr Opin Clin Nutr Metab Care. 2016;19(6):427–433. doi:10.1097/MCO.0000000000000319
- Gosker HR, Zeegers MP, Wouters EF, Schols AM. Muscle fibre type shifting in the vastus lateralis of patients with COPD is associated with disease severity: a systematic review and meta-analysis. Thorax. 2007;62(11):944–949. doi:10.1136/thx.2007.078980
- van de Bool C, Gosker HR, van den Borst B, Op den Kamp CM, Slot IGM, Schols AMWJ. Muscle quality is more impaired in sarcopenic patients with chronic obstructive pulmonary disease. J Am Med Dir Assoc. 2016;17(5):415–420. doi:10.1016/j.jamda.2015.12.094
- Krzyminska-Siemaszko R, Tobis S, Lewandowicz M, Wieczorowska-Tobis K. Comparison of four sarcopenia screening questionnaires in community-dwelling older adults from Poland using six sets of international diagnostic criteria of sarcopenia. PLoS One. 2020;15(4):e0231847. doi:10.1371/journal.pone.0231847
- Hajaoui M, Locquet M, Beaudart C, Reginster J-Y, Petermans J, Bruyère O. Sarcopenia: performance of the SARC-F questionnaire according to the european consensus criteria, EWGSOP1 and EWGSOP2. J Am Med Dir Assoc. 2019;20(9):1182–1183. doi:10.1016/j.jamda.2019.05.021
- Bahat G, Yilmaz O, Kılıç C, Oren MM, Karan MA. Performance of SARC-F in regard to sarcopenia definitions, muscle mass and functional measures. J Nutr Health Aging. 2018;22(8):898–903. doi:10.1007/s12603-018-1067-8
- Barbosa-Silva TG, Menezes AMB, Bielemann RM, Malmstrom TK, Gonzalez MC. Enhancing SARC-F: improving sarcopenia screening in the clinical practice. J Am Med Dir Assoc. 2016;17(12):1136–1141.
- Kashani KB, Frazee EN, Kukralova L, et al. Evaluating muscle mass by using markers of kidney function: development of the sarcopenia index. Crit Care Med. 2017;45(1):e23–e29. doi:10.1097/CCM.0000000000002013
- Amado CA, Garcia-Unzueta MT, Lavin BA, et al. The ratio serum creatinine/serum cystatin C (a surrogate marker of muscle mass) as a predictor of hospitalization in chronic obstructive pulmonary disease outpatients. Respiration. 2019;97(4):302–309. doi:10.1159/000494296
- Hirai K, Tanaka A, Homma T, et al. Serum creatinine/cystatin C ratio as a surrogate marker for sarcopenia in patients with chronic obstructive pulmonary disease. Clin Nutr. 2020. doi:10.1016/j.clnu.2020.08.010
- Tang T, Zhuo Y, Xie L, Wang H, Yang M. Sarcopenia index based on serum creatinine and cystatin C is associated with 3-year mortality in hospitalized older patients. Sci Rep. 2020;10(1):1260. doi:10.1038/s41598-020-58304-z
- Lien YH. Looking for sarcopenia biomarkers. Am J Med. 2017;130(5):502–503. doi:10.1016/j.amjmed.2017.01.018
- Trudzinski FC, Alqudrah M, Omlor A, et al. Consequences of chronic kidney disease in chronic obstructive pulmonary disease. Respir Res. 2019;20(1):151. doi:10.1186/s12931-019-1107-x
- Kanda K, Sakuma J, Akimoto T, Kawakami Y, Suzuki K. Detection of titin fragments in urine in response to exercise-induced muscle damage. PLoS One. 2017;12(7):e0181623. doi:10.1371/journal.pone.0181623
- Nakanishi N, Tsutsumi R, Hara K, et al. Urinary titin is a novel biomarker for muscle atrophy in nonsurgical critically ill patients: a two-center, prospective observational study. Crit Care Med. 2020;48(9):1327–1333. doi:10.1097/CCM.0000000000004486
- Beaudart C, McCloskey E, Bruyère O, et al. Sarcopenia in daily practice: assessment and management. BMC Geriatr. 2016;16(1):170. doi:10.1186/s12877-016-0349-4
- Borga M, West J, Bell JD, et al. Advanced body composition assessment: from body mass index to body composition profiling. J Investig Med. 2018;66(5):1–9. doi:10.1136/jim-2018-000722
- Karlsson A, Rosander J, Romu T, et al. Automatic and quantitative assessment of regional muscle volume by multi-atlas segmentation using whole-body water-fat MRI. J Magn Reson Imaging. 2015;41(6):1558–1569. doi:10.1002/jmri.24726
- West J, Dahlqvist Leinhard O, Romu T, et al. Feasibility of MR-based body composition analysis in large scale population studies. PLoS One. 2016;11(9):e0163332. doi:10.1371/journal.pone.0163332
- Schweitzer L, Geisler C, Pourhassan M, et al. What is the best reference site for a single MRI slice to assess whole-body skeletal muscle and adipose tissue volumes in healthy adults? Am J Clin Nutr. 2015;102(1):58–65. doi:10.3945/ajcn.115.111203
- Lee K, Shin Y, Huh J, et al. Recent issues on body composition imaging for sarcopenia evaluation. Korean J Radiol. 2019;20(2):205–217. doi:10.3348/kjr.2018.0479
- Engelke K, Museyko O, Wang L, Laredo JD. Quantitative analysis of skeletal muscle by computed tomography imaging-State of the art. J Orthop Translat. 2018;15:91–103. doi:10.1016/j.jot.2018.10.004
- Albano D, Messina C, Vitale J, Sconfienza LM. Imaging of sarcopenia: old evidence and new insights. Eur Radiol. 2020;30(4):2199–2208. doi:10.1007/s00330-019-06573-2
- Sanders KJC, Degens J, Dingemans AC, Schols A. Cross-sectional and longitudinal assessment of muscle from regular chest computed tomography scans: L1 and pectoralis muscle compared to L3 as reference in non-small cell lung cancer. Int J Chron Obstruct Pulmon Dis. 2019;14:781–789. doi:10.2147/COPD.S194003
- Amini B, Boyle SP, Boutin RD, Lenchik L. Approaches to assessment of muscle mass and myosteatosis on computed tomography: a systematic review. J Gerontol a Biol Sci Med Sci. 2019;74(10):1671–1678. doi:10.1093/gerona/glz034
- McDonald ML, Diaz AA, Ross JC, et al. Quantitative computed tomography measures of pectoralis muscle area and disease severity in chronic obstructive pulmonary disease. A cross-sectional study. Ann Am Thorac Soc. 2014;11(3):326–334. doi:10.1513/AnnalsATS.201307-229OC
- Tanimura K, Sato S, Fuseya Y, et al. Quantitative assessment of erector spinae muscles in patients with chronic obstructive pulmonary disease. novel chest computed tomography-derived index for prognosis. Ann Am Thorac Soc. 2016;13(3):334–341. doi:10.1513/AnnalsATS.201507-446OC
- Buckinx F, Landi F, Cesari M, et al. Pitfalls in the measurement of muscle mass: a need for a reference standard. J Cachexia Sarcopenia Muscle. 2018;9(2):269–278. doi:10.1002/jcsm.12268
- Chen YW, Ramsook AH, Coxson HO, Bon J, Reid WD. Prevalence and risk factors for osteoporosis in individuals with COPD: a systematic review and meta-analysis. Chest. 2019;156(6):1092–1110. doi:10.1016/j.chest.2019.06.036
- Ofenheimer A, Breyer-Kohansal R, Hartl S, et al. Reference values of body composition parameters and visceral adipose tissue (VAT) by DXA in adults aged 18–81 years-results from the LEAD cohort. Eur J Clin Nutr. 2020;74(8):1181–1191. doi:10.1038/s41430-020-0596-5
- Heymsfield SB, Gonzalez MC, Lu J, et al. Skeletal muscle mass and quality: evolution of modern measurement concepts in the context of sarcopenia. Proc Nutr Soc. 2015;74(4):355–366. doi:10.1017/S0029665115000129
- Rutten EP, Spruit MA, Wouters EF. Critical view on diagnosing muscle wasting by single-frequency bio-electrical impedance in COPD. Respir Med. 2010;104(1):91–98. doi:10.1016/j.rmed.2009.07.004
- Franssen FM, Rutten EP, Groenen MT, Vanfleteren LE, Wouters EF, Spruit MA. New reference values for body composition by bioelectrical impedance analysis in the general population: results from the UK Biobank. J Am Med Dir Assoc. 2014;15(6):448 e441–446. doi:10.1016/j.jamda.2014.03.012
- Lee JE, Chun H, Kim YS, et al. Association between timed up and go test and subsequent functional dependency. J Korean Med Sci. 2020;35(3):e25–e25. doi:10.3346/jkms.2020.35.e25
- Landi F, Onder G, Russo A, et al. Calf circumference, frailty and physical performance among older adults living in the community. Clin Nutr. 2014;33(3):539–544. doi:10.1016/j.clnu.2013.07.013
- Marklund S, Bui KL, Nyberg A. Measuring and monitoring skeletal muscle function in COPD: current perspectives. Int J Chron Obstruct Pulmon Dis. 2019;14:1825–1838. doi:10.2147/COPD.S178948
- Canavan JL, Maddocks M, Nolan CM, et al. Functionally relevant cut point for isometric quadriceps muscle strength in chronic respiratory disease. Am J Respir Crit Care Med. 2015;192(3):395–397. doi:10.1164/rccm.201501-0082LE
- Martin HJ, Yule V, Syddall HE, Dennison EM, Cooper C, Aihie Sayer A. Is hand-held dynamometry useful for the measurement of quadriceps strength in older people? A comparison with the gold standard Bodex dynamometry. Gerontology. 2006;52(3):154–159. doi:10.1159/000091824
- Nyberg A, Saey D, Maltais F. Why and how limb muscle mass and function should be measured in patients with chronic obstructive pulmonary disease. Ann Am Thorac Soc. 2015;12(9):1269–1277. doi:10.1513/AnnalsATS.201505-278PS
- Karagiannis C, Savva C, Korakakis V, et al. Test-retest reliability of handgrip strength in patients with chronic obstructive pulmonary disease. COPD. 2020;1–7.
- Spruit MA, Sillen MJ, Groenen MT, Wouters EF, Franssen FM. New normative values for handgrip strength: results from the UK Biobank. J Am Med Dir Assoc. 2013;14(10):775 e775–711. doi:10.1016/j.jamda.2013.06.013
- Dodds RM, Syddall HE, Cooper R, et al. Grip strength across the life course: normative data from twelve British studies. PLoS One. 2014;9(12):e113637–e113637. doi:10.1371/journal.pone.0113637
- Alley DE, Shardell MD, Peters KW, et al. Grip strength cutpoints for the identification of clinically relevant weakness. J Gerontol a Biol Sci Med Sci. 2014;69(5):559–566. doi:10.1093/gerona/glu011
- Vasconcelos K, Dias JMD, Bastone A, et al. Handgrip strength cutoff points to identify mobility limitation in community-dwelling older people and associated factors. J Nutr Health Aging. 2016;20(3):306–315. doi:10.1007/s12603-015-0584-y
- Cesari M, Kritchevsky SB, Newman AB, et al. Added value of physical performance measures in predicting adverse health-related events: results from the health, aging and body composition study. J Am Geriatr Soc. 2009;57(2):251–259. doi:10.1111/j.1532-5415.2008.02126.x
- Lage V, Silva GPD, Lacerda ACR, et al. Functional tests associated with sarcopenia in moderate chronic obstructive pulmonary disease. Expert Rev Respir Med. 2020. doi:10.1080/17476348.2021.1850276
- Jones SE, Kon SSC, Canavan JL, et al. The five-repetition sit-to-stand test as a functional outcome measure in COPD. Thorax. 2013;68(11):1015–1020. doi:10.1136/thoraxjnl-2013-203576
- Lee L, Patel T, Costa A, et al. Screening for frailty in primary care: accuracy of gait speed and hand-grip strength. Can Fam Physician. 2017;63(1):e51–e57.
- Kon SS, Canavan JL, Nolan CM, et al. The 4-metre gait speed in COPD: responsiveness and minimal clinically important difference. Eur Respir J. 2014;43(5):1298–1305. doi:10.1183/09031936.00088113
- Pavasini R, Guralnik J, Brown JC, et al. Short physical performance battery and all-cause mortality: systematic review and meta-analysis. BMC Med. 2016;14(1):215. doi:10.1186/s12916-016-0763-7
- Bohannon RW. Reference values for the timed up and go test: a descriptive meta-analysis. J Geriatr Phys Ther. 2006;29(2):64–68. doi:10.1519/00139143-200608000-00004
- Al Haddad MA, John M, Hussain S, Bolton CE. Role of the timed up and go test in patients with chronic obstructive pulmonary disease. J Cardiopulm Rehabil Prev. 2016;36(1):49–55. doi:10.1097/HCR.0000000000000143
- Kovelis D, Gomes ARS, Mazzarin C, de Miranda A, Valderramas S. Association between the results of the timed up-and-go test adjusted for disease severity and sarcopenia in patients with chronic obstructive pulmonary disease: a pilot study. Clinics (Sao Paulo). 2019;74:e930–e930. doi:10.6061/clinics/2019/e930
- Mesquita R, Wilke S, Smid DE, et al. Measurement properties of the timed up & go test in patients with COPD. Chron Respir Dis. 2016;13(4):344–352. doi:10.1177/1479972316647178
- Bergland A, Jørgensen L, Emaus N, Strand BH. Mobility as a predictor of all-cause mortality in older men and women: 11.8 year follow-up in the tromsø study. BMC Health Serv Res. 2017;17(1):22. doi:10.1186/s12913-016-1950-0
- Hansen H, Beyer N, Frølich A, Godtfredsen N, Bieler T. Intra- and inter-rater reproducibility of the 6-minute walk test and the 30-second sit-to-stand test in patients with severe and very severe COPD. Int J Chron Obstruct Pulmon Dis. 2018;13:3447–3457. doi:10.2147/COPD.S174248
- Agarwala P, Salzman SH. Six-minute walk test: clinical role, technique, coding, and reimbursement. Chest. 2020;157(3):603–611. doi:10.1016/j.chest.2019.10.014
- Celli B, Tetzlaff K, Criner G, et al. The 6-minute-walk distance test as a chronic obstructive pulmonary disease stratification tool. insights from the COPD biomarker qualification consortium. Am J Respir Crit Care Med. 2016;194(12):1483–1493. doi:10.1164/rccm.201508-1653OC
- Kim SH, Shin MJ, Shin YB, Kim KU. Sarcopenia associated with chronic obstructive pulmonary disease. J Bone Metab. 2019;26(2):65–74. doi:10.11005/jbm.2019.26.2.65
- McCarthy B, Casey D, Devane D, Murphy K, Murphy E, Lacasse Y. Pulmonary rehabilitation for chronic obstructive pulmonary disease. Cochrane Database Syst Rev. 2015;(2):CD003793.
- Marillier M, Bernard AC, Verges S, Neder JA. Locomotor muscles in COPD: the rationale for rehabilitative exercise training. Front Physiol. 2019;10:1590. doi:10.3389/fphys.2019.01590
- Spruit MA, Singh SJ, Garvey C, et al. An official American thoracic society/European respiratory society statement: key concepts and advances in pulmonary rehabilitation. Am J Respir Crit Care Med. 2013;188(8):e13–64. doi:10.1164/rccm.201309-1634ST
- Passey SL, Hansen MJ, Bozinovski S, McDonald CF, Holland AE, Vlahos R. Emerging therapies for the treatment of skeletal muscle wasting in chronic obstructive pulmonary disease. Pharmacol Ther. 2016;166:56–70. doi:10.1016/j.pharmthera.2016.06.013
- Zeng Y, Jiang F, Chen Y, Chen P, Cai S. Exercise assessments and trainings of pulmonary rehabilitation in COPD: a literature review. Int J Chron Obstruct Pulmon Dis. 2018;13:2013–2023. doi:10.2147/COPD.S167098
- Morris NR, Walsh J, Adams L, Alision J. Exercise training in COPD: what is it about intensity? Respirology. 2016;21(7):1185–1192. doi:10.1111/resp.12864
- Hunter GR, Singh H, Carter SJ, Bryan DR, Fisher G. Sarcopenia and its implications for metabolic health. J Obes. 2019;2019:8031705. doi:10.1155/2019/8031705
- Dilektasli AG, Porszasz J, Stringer WW, Casaburi R. Physiologic effects of oxygen supplementation during exercise in chronic obstructive pulmonary disease. Clin Chest Med. 2019;40(2):385–395. doi:10.1016/j.ccm.2019.02.004
- Alison JA, McKeough ZJ, Leung RWM, et al. Oxygen compared to air during exercise training in COPD with exercise-induced desaturation. Eur Respir J. 2019;53(5):1802429. doi:10.1183/13993003.02429-2018
- Jones S, Man WD, Gao W, Higginson IJ, Wilcock A, Maddocks M. Neuromuscular electrical stimulation for muscle weakness in adults with advanced disease. Cochrane Database Syst Rev. 2016;10:CD009419. doi:10.1002/14651858.CD009419.pub3
- Wu X, Hu X, Hu W, Xiang G, Li S. Effects of neuromuscular electrical stimulation on exercise capacity and quality of life in COPD patients: a systematic review and meta-analysis. Biosci Rep. 2020;40(5). doi:10.1042/BSR20191912
- Sillen MJ, Franssen FM, Delbressine JM, Vaes AW, Wouters EF, Spruit MA. Efficacy of lower-limb muscle training modalities in severely dyspnoeic individuals with COPD and quadriceps muscle weakness: results from the DICES trial. Thorax. 2014;69(6):525–531. doi:10.1136/thoraxjnl-2013-204388
- Landi F, Sieber C, Fielding RA, Rolland Y, Guralnik J. Nutritional intervention in sarcopenia: report from the international conference on frailty and sarcopenia research task force. J Frailty Aging. 2018;7(4):247–252. doi:10.14283/jfa.2017.26
- Marco E, Sanchez-Rodriguez D, Davalos-Yerovi VN, et al. Malnutrition according to ESPEN consensus predicts hospitalizations and long-term mortality in rehabilitation patients with stable chronic obstructive pulmonary disease. Clin Nutr. 2019;38(5):2180–2186. doi:10.1016/j.clnu.2018.09.014
- Yoneda T, Yoshikawa M, Fu A, Tsukaguchi K, Okamoto Y, Takenaka H. Plasma levels of amino acids and hypermetabolism in patients with chronic obstructive pulmonary disease. Nutrition. 2001;17(2):95–99. doi:10.1016/S0899-9007(00)00509-8
- Sergi G, Coin A, Marin S, et al. Body composition and resting energy expenditure in elderly male patients with chronic obstructive pulmonary disease. Respir Med. 2006;100(11):1918–1924. doi:10.1016/j.rmed.2006.03.008
- Baum JI, Kim IY, Wolfe RR. Protein consumption and the elderly: what is the optimal level of intake? Nutrients. 2016;8(6):359. doi:10.3390/nu8060359
- Rondanelli M, Klersy C, Terracol G, et al. Whey protein, amino acids, and vitamin D supplementation with physical activity increases fat-free mass and strength, functionality, and quality of life and decreases inflammation in sarcopenic elderly. Am J Clin Nutr. 2016;103(3):830–840. doi:10.3945/ajcn.115.113357
- Wood LG. Omega-3 polyunsaturated fatty acids and chronic obstructive pulmonary disease. Curr Opin Clin Nutr Metab Care. 2015;18(2):128–132. doi:10.1097/MCO.0000000000000142
- Fulton AS, Hill AM, Williams MT, Howe PRC, Coates AM. Paucity of evidence for a relationship between long-chain omega-3 fatty acid intake and chronic obstructive pulmonary disease: a systematic review. Nutr Rev. 2015;73(9):612–623. doi:10.1093/nutrit/nuv017
- Collins PF, Yang IA. Nutritional support in chronic obstructive pulmonary disease (COPD): an evidence update. J Thorac Dis. 2019;11(Suppl 17):S2230–S2237. doi:10.21037/jtd.2019.10.41
- Broekhuizen R, Wouters EF, Creutzberg EC, Weling-Scheepers CA, Schols AM. Polyunsaturated fatty acids improve exercise capacity in chronic obstructive pulmonary disease. Thorax. 2005;60(5):376–382. doi:10.1136/thx.2004.030858
- Calder PC, Laviano A, Lonnqvist F, Muscaritoli M, Öhlander M, Schols A. Targeted medical nutrition for cachexia in chronic obstructive pulmonary disease: a randomized, controlled trial. J Cachexia Sarcopenia Muscle. 2018;9(1):28–40. doi:10.1002/jcsm.12228
- Zhu M, Wang T, Wang C, Ji Y. The association between vitamin D and COPD risk, severity, and exacerbation: an updated systematic review and meta-analysis. Int J Chron Obstruct Pulmon Dis. 2016;11:2597–2607. doi:10.2147/COPD.S101382
- Remelli F, Vitali A, Zurlo A, Volpato S. Vitamin D deficiency and sarcopenia in older persons. Nutrients. 2019;11(12):2861. doi:10.3390/nu11122861
- Jolliffe DA, Greenberg L, Hooper RL, et al. Vitamin D to prevent exacerbations of COPD: systematic review and meta-analysis of individual participant data from randomised controlled trials. Thorax. 2019;74(4):337–345. doi:10.1136/thoraxjnl-2018-212092
- Calder PC, Laviano A, Lonnqvist F, Muscaritoli M, Ohlander M, Schols A. Targeted medical nutrition for cachexia in chronic obstructive pulmonary disease: a randomized, controlled trial. J Cachexia Sarcopenia Muscle. 2018;9(1):28–40.
- Bauer J, Biolo G, Cederholm T, et al. Evidence-based recommendations for optimal dietary protein intake in older people: a position paper from the PROT-AGE study group. J Am Med Dir Assoc. 2013;14(8):542–559. doi:10.1016/j.jamda.2013.05.021
- Pan L, Wang M, Xie X, Du C, Guo Y, Aggarwal AN. Effects of anabolic steroids on chronic obstructive pulmonary disease: a meta-analysis of randomised controlled trials. PLoS One. 2014;9(1):e84855. doi:10.1371/journal.pone.0084855
- Baillargeon J, Urban RJ, Zhang W, et al. Testosterone replacement therapy and hospitalization rates in men with COPD. Chron Respir Dis. 2019;16:1479972318793004. doi:10.1177/1479972318793004
- Creutzberg EC, Wouters EF, Mostert R, Pluymers RJ, Schols AM. A role for anabolic steroids in the rehabilitation of patients with COPD? A double-blind, placebo-controlled, randomized trial. Chest. 2003;124(5):1733–1742. doi:10.1378/chest.124.5.1733
- Casaburi R, Bhasin S, Cosentino L, et al. Effects of testosterone and resistance training in men with chronic obstructive pulmonary disease. Am J Respir Crit Care Med. 2004;170(8):870–878. doi:10.1164/rccm.200305-617OC
- Pape GS, Friedman M, Underwood LE, Clemmons DR. The effect of growth hormone on weight gain and pulmonary function in patients with chronic obstructive lung disease. Chest. 1991;99(6):1495–1500. doi:10.1378/chest.99.6.1495
- Rolland Y, Onder G, Morley JE, Gillette-Guyonet S, Abellan van Kan G, Vellas B. Current and future pharmacologic treatment of sarcopenia. Clin Geriatr Med. 2011;27(3):423–447. doi:10.1016/j.cger.2011.03.008
- Collden G, Tschop MH, Muller TD. Therapeutic potential of targeting the ghrelin pathway. Int J Mol Sci. 2017;18(4):798. doi:10.3390/ijms18040798
- Rooks D, Praestgaard J, Hariry S, et al. Treatment of sarcopenia with bimagrumab: results from a phase II, randomized, controlled, proof-of-concept study. J Am Geriatr Soc. 2017;65(9):1988–1995. doi:10.1111/jgs.14927
- Polkey MI, Praestgaard J, Berwick A, et al. Activin type II receptor blockade for treatment of muscle depletion in chronic obstructive pulmonary disease. a randomized trial. Am J Respir Crit Care Med. 2019;199(3):313–320. doi:10.1164/rccm.201802-0286OC
- Tonnesen P. Smoking cessation and COPD. Eur Respir Rev. 2013;22(127):37–43. doi:10.1183/09059180.00007212
- Tashkin DP. Smoking cessation in chronic obstructive pulmonary disease. Semin Respir Crit Care Med. 2015;36(4):491–507. doi:10.1055/s-0035-1555610
- Degens H, Gayan-Ramirez G, van Hees HW. Smoking-induced skeletal muscle dysfunction: from evidence to mechanisms. Am J Respir Crit Care Med. 2015;191(6):620–625. doi:10.1164/rccm.201410-1830PP
- Ajime TT, Serre J, Wust RCI, et al. Two weeks smoking cessation reverses cigarette smoke-induced skeletal muscle atrophy and mitochondrial dysfunction in mice. Nicotine Tob Res. 2020. doi:10.1093/ntr/ntaa016
- Troosters T, Maltais F, Leidy N, et al. Effect of bronchodilation, exercise training, and behavior modification on symptoms and physical activity in chronic obstructive pulmonary disease. Am J Respir Crit Care Med. 2018;198(8):1021–1032. doi:10.1164/rccm.201706-1288OC
- Di Marco F, Sotgiu G, Santus P, et al. Long-acting bronchodilators improve exercise capacity in COPD patients: a systematic review and meta-analysis. Respir Res. 2018;19(1):18. doi:10.1186/s12931-018-0721-3
- Joassard OR, Durieux AC, Freyssenet DG. Beta2-adrenergic agonists and the treatment of skeletal muscle wasting disorders. Int J Biochem Cell Biol. 2013;45(10):2309–2321. doi:10.1016/j.biocel.2013.06.025
- Koopman R, Ryall JG, Church JE, Lynch GS. The role of beta-adrenoceptor signaling in skeletal muscle: therapeutic implications for muscle wasting disorders. Curr Opin Clin Nutr Metab Care. 2009;12(6):601–606. doi:10.1097/MCO.0b013e3283318a25
- Hostrup M, Reitelseder S, Jessen S, et al. Beta2-adrenoceptor agonist salbutamol increases protein turnover rates and alters signalling in skeletal muscle after resistance exercise in young men. J Physiol. 2018;596(17):4121–4139. doi:10.1113/JP275560
- Mineo D, Ambrogi V, Lauriola V, Pompeo E, Mineo TC. Recovery of body composition improves long-term outcomes after lung volume reduction surgery for emphysema. Eur Respir J. 2010;36(2):408–416. doi:10.1183/09031936.00142309
- Sanders KJC, Klooster K, Vanfleteren L, Slebos DJ, Schols A. CT-derived muscle remodelling after bronchoscopic lung volume reduction in advanced emphysema. Thorax. 2019;74(2):206–207. doi:10.1136/thoraxjnl-2018-211931
- Shah S, Darekar B, Salvi S, Kowale A. Quadriceps strength in patients with chronic obstructive pulmonary disease. Lung India. 2019;36(5):417–421. doi:10.4103/lungindia.lungindia_27_19
- Billot M, Calvani R, Urtamo A, et al. Preserving mobility in older adults with physical frailty and sarcopenia: opportunities, challenges, and recommendations for physical activity interventions. Clin Interv Aging. 2020;15:1675–1690. doi:10.2147/CIA.S253535