Abstract
Purpose
This study reported the efficacy and underlying mechanism of agrimonolide (AM) in treating colon cancer.
Methods
Colon cancer-AM-related targets were screened from online database. AM targets for colon cancer were identified by Venn diagram. Main molecular function, biological process, cellular component and pathways associated with AM targets for colon cancer were analyzed by GO and KEGG enrichment analysis. Relationship of the 10 core targets of AM for colon cancer with the top 15 BP and KEGG pathways was analyzed by Cytoscape software. A “component-target-pathway” network was constructed to select the hub genes of AM for colon cancer. AM effects on colon cancer cell viability, proliferation, invasion, migration and apoptosis were researched by CCK-8, colony formation, Transwell invasion, wound healing and flow cytometry assays. Tumor-bearing nude mice models were constructed and given AM treatment. Hub gene expression in cells/tissues was detected by Western blot.
Results
A total of 107 targets were selected as AM targets for colon cancer. The 10 core targets were related to the top 15 biological process terms and KEGG pathways. PI3K, AKT and mTOR were selected as the hub genes of AM for colon cancer. AM weakened colon cell proliferation, invasion, migration and apoptosis inhibition, and suppressed colon cell in vivo growth. AM up-regulated Caspase-3 and BAX proteins, down-regulated C-Myc, Cyclin D1 and BCL-2 proteins, and inactivated the PI3K/AKT/mTOR pathway both in vitro and in vivo.
Conclusion
AM suppressed colon cancer progression through inactivating the PI3K/AKT/mTOR pathway. It may be useful for colon cancer treatment.
Introduction
Colon cancer is a leading malignancy associated with high recurrence, metastasis and mortality rates within 5 years.Citation1 About 20% of colon cancer cases lose surgery treatment opportunity because of the distant metastases at the first time diagnosis.Citation2 Pharmacological treatment is a common strategy for those metastasis patients. However, the 5-year survival rate is as low as 10% for the metastasis patients.Citation3 Some drugs used for colon cancer treatment have limited efficacy, and at the same time, treatment failure caused by adverse side effects and low patient compliance are common clinical adverse events.Citation4 Therefore, novel effective drugs are particularly needed to be discovered when it comes to prolonging the survival of colon cancer patients, especially those with metastases.
Agrimony is a traditional Chinese edible herb belonging to the Rosaceae family, which possesses multiple functions, such as antioxidant, anticancer and hepatoprotective activities.Citation5 Agrimonolide (AM) is abundantly contained in Agrimony, and it has been discovered to have potent antioxidant activity and strong potential against diabetes.Citation6,Citation7 Besides, AM are able to counteract oxidative stress in hepatocytes and protect them from damage, possibly by scavenging free radical activity and increasing antioxidant enzyme activity.Citation8 Recently, AM has been found to exert anti-cancer activity, as demonstrated by its inhibitory effect on the proliferation and induction effect of the apoptosis of gastric cancer cells.Citation9 Similarly, Liu et alCitation10 demonstrated the suppression role of AM on the proliferation, invasion and migration of ovarian cancer cells; meanwhile, AM induced the apoptosis of ovarian cancer cells, and blocked the oxidative stress in ovarian cancer cells. It was thus proposed to be a novel agent for the treatment of ovarian cancer. However, the effect of AM on colon cancer and the underlying mechanism have not yet been elucidated.
Network pharmacology, proposed by Hopkins et al in 2007, has been developed to be a promising approach for the discovery of effective drugs, as it analyzes drugs and potential targets in treating disease based on the system biology.Citation11,Citation12 Thus, in this study, we predicted the targets of AM for colon cancer based on network pharmacology, and then further validated it by in vivo and in vitro experiments. This study may provide novel drug for colon cancer treatment.
Materials and Methods
Screening for Colon Cancer-Related Targets
By the Genecards database, the targets of colon cancer-related diseases were searched with a score >4-fold median and a score of >2.9521 as the screening criteria. A total of 807 colon cancer diseases-related targets were retrieved. Besides, a total of 724 colon cancer diseases-related targets were screened from the Disgenet database, with a score >2-fold median and a score of >0.02 as the screening criteria. Moreover, a total of 805 colon cancer diseases-related targets were obtained based on the searching of the Online Mendelian Inheritance in Man (OMIM) database. Then these targets screened from the three above databases were standardized by the GENE module of National Center for Biotechnology Information (NCBI). After removing the duplicate targets, 1081 colon cancer-related targets were finally screened. Our study only involved human data in the public databases, not the use of human clinical data in Shanghai Seventh People’s Hospital. Since the above database is a public database, registered users can view and use the data in the database. Based on this, this study does not need to pass the ethical approval of human-related biomedical research of the Ethics Committee of Shanghai Seventh People’s Hospital.
Screening for AM-Related Targets
AM-related targets were predicted by the Pharmmapper database with the Human Protein Targets Only (v2010, 2241) as the target set. The top 300 Reserved Matched Targets with a median Norm Fit ≥0.5253 were selected. These targets were standardized by the Uniprot and the GENE module of NCBI, and then a total of 146 AM-related targets were screened. Further, AM-related targets were predicted by the SwissTarget Prediction database with a score of >0.1 as the screening criteria. After being standardized by the Uniprot and the GENE module of NCBI, a total of 111 AM-related targets were screened. Additionally, AM-related targets were predicted by the Super-PRED database with P ≥ 50% as the screening criteria. After being standardized by the Uniprot and the GENE module of NCBI, and a total of 105 AM-related targets were obtained. All of these AM-related targets collected from the above three databases were standardized by the Uniprot and the GENE module of NCBI. The duplicate targets were excluded. Finally, a total of 315 AM-related targets were screened.
Identification of AM Targets for Colon Cancer
The identification of AM targets for colon cancer was screened. Briefly, the 1081 colon cancer-related targets and the 315 AM-related targets were intersected by Venn diagram. The overlapped targets were considered as the AM targets for colon cancer.
Protein–Protein Interaction (PPI) Network Analysis
PPI network analysis of AM targets for colon cancer was performed by using the String database with the Organism of “Homo sapiens” and a confidence score ≥0.4.
Gene Ontology (GO) and Kyoto Encyclopedia of Genes and Genomes (KEGG) Analysis
Enrichment analysis including GO functional enrichment analysis and KEGG pathway enrichment analysis was performed on the AM targets for colon cancer and the core network targets of PPI network. GO analysis included molecular function (MF), biological process (BP) and cellular component (CC), and data were obtained from the GO database. Data of pathway enrichment analysis were acquired from the KEGG pathway database. The Hypergeometric Distribution Model was utilized to assess whether the target gene set was significantly associated with particular GO and biological pathway. The Hypergeometric Distribution Model was shown below (Note: “N” is the number of total genes; “M” is the number of genes annotated in pathway, GO or subject term; “n” is the number of AM targets; “k” is the number of shared genes):
P-value adjusted by the Bonferroni method reflected the association strength of AM target for colon cancer with GO or pathway. In this research, the “clusterProfiler package version 3.15.4” of the R platform was utilized to perform GO and KEGG analysis.
Screening of Core Targets and Core Hub Genes of AM
This study used the Cytoscape software to build a “component-target-disease” network in order to screen the core targets of AM for colon cancer. The cytoHubba plug-in of Cytoscape software was utilized to construct the core targets network to assess the relationship between these core targets and the top 15 BP terms or the top 15 KEGG pathways. Additionally, a “component-target-pathway” network was constructed by using the Cytoscape software in order to explain the mechanism of AM in treating colon cancer.
Cell Culture and AM Treatment
HCT-116 cells (YB-H197, Yubo Biotechnology Co., Shanghai, China) were maintained in Dulbecco’s modified Eagle’s medium (DMEM) with 10% fetal bovine serum (FBS) at a condition of 37°C and 5% CO2. For AM treatment, DMEM with 10% FBS and different concentration (0–100 μM) of AM (B30385, Shanghai Yuanye Biotechnology Co., Ltd, Shanghai, China) was utilized to treat HCT-116 cells at 37°C and 5% CO2.
Cell Counting Kit-8 (CCK-8) Assay
HCT-116 cells (5 × 103 cells) were grown into 96-well plates with 100 μL DMEM containing 10% FBS and different concentration of AM (0–100 μM) at 37°C and 5% CO2. After 48 h of incubation, CCK8 solution (Solar Biotechnology Ltd., Beijing, China) with 10 μL per well was utilized to treated cells for 2 h at 37°C. The absorbance values of wells were read at 450 nm by microplate reader (Bio-Tek Instruments Inc., Winooski, VT, USA). The “AM concentration-cell viability” curve was made to calculate the inhibitory concentration (IC50) value. Similarly, HCT-116 cells were treated for 48 h by DMEM containing 10% FBS and AM (0, 10, 20 and 40 μM) to detect the cell viability. Five replicates were set for each group.
Colony Formation Assay
HCT-116 cells were prepared into single suspension (1 × 103 cells/mL) by DMEM containing 10% FBS and AM (0, 10, 20 and 40 μM). The single suspension with a volume of 1 mL was inoculated into 6-well plates for 2 weeks culture. The culture environment was at 37°C and 5% CO2. The medium was refreshed at a 3-day interval. At the end, HCT-116 cells were immobilized by 4% paraformaldehyde (for 15 min at room temperature) and stained by 0.1% crystal violet (for 10 min at room temperature). The colony number was counted under an inverted microscope (IX73, Olympus, Tokyo, Japan). A community of more than 50 cells was defined as a clone.
Transwell Invasion Assay
Matrigel (BD Biosciences, San Jose, CA, USA) was diluted into serum-free DMEM at a ratio of 1:3 and then 50 μL was collected to pre-coat the Transwell chambers. After 48 h of AM treatment, HCT-116 cells were collected and dispersed into serum-free DMEM (1 × 105 cells/mL). Then, 500 μL cell suspension was added into the upper chambers, while 600 μL DMEM containing 10% FBS was plated into the lower chambers. HCT-116 cells were placed for 24 h at 37°C and 5% CO2 for invasion. The invasion cells were immobilized by 4% paraformaldehyde (for 15 min at room temperature) and stained by 0.1% crystal violet (for 10 min at room temperature). Finally, the invasion cells were photographed and counter under an inverted microscope (IX73, Olympus, Tokyo, Japan). Five non-overlapping fields were selected for invasion cell counting.
Wound Healing Assay
After 48 h of AM treatment, HCT-116 cells were harvested for the preparation of cell suspension by serum-free DMEM (1 × 106 cells/mL). The cell suspension was plated into 6-well plates with 1 mL per well, and incubated for 24 h at a condition of 37°C and 5% CO2. A wound was then made at the bottom by utilizing a 10 μL sterile pipette. The original wound was photographed and measured under an inverted microscope (IX73, Olympus, Tokyo, Japan). Then, 1 mL fresh serum-free DMEM was added to incubate HCT-116 cells for another 24 h. Similarly, the final wound was photographed and measured. By calculating relative wound width (final wound width/original wound width), the migration ability of HCT-116 cells was evaluated.
Flow Cytometry Analysis of Apoptosis
After 48 h of AM treatment, HCT-116 cells were collected and underwent the apoptosis analysis in accordance with the Annexin V-fluorescein isothiocyanate (FITC)/propidium iodide (PI) Apoptosis Detection Kit (Yubo Biotechnology Co., Shanghai, China) instruction. In short, HCT-116 cells were washed by pre-cooled phosphate buffered saline (PBS) for two times, and then dispersed into 100 μL 1 × binding buffer. Annexin V-FITC (5 μL) and PI (10 μL) were utilized sequentially to stain cells in the dark (for 10 min at room temperature). At last, apoptotic cells were detected by FACSort flow cytometer (Becton Dickinson, San Jose, CA, USA) and analyzed by CellQuest software system (Becton Dickinson, San Jose, CA, USA).
In vivo Experiment
Animal study has been ratified by Animal Ethics Committee of Seventh People’s Hospital of Shanghai University of Traditional Chinese Medicine (IRB number: HB25a89). BALB/c nude mice (n = 12, 4–5 weeks old) were commercially supplied by Junke Biological Engineering Co. (Nanjing, China), and were housed in a specific pathogen-free condition with food and water freely available. Tumor-bearing nude mouse model was constructed by subcutaneous injecting HCT-116 cells. All mice underwent subcutaneous injection of HCT-116 cells (1 × 106 cells in 100 μL PBS) on the right side of the back. After injection, six mice were randomly selected and given AM for 28 days at a dose of 50 mg/kg body weight every day. The other six mice without AM treatment were served as control. During the 28-day, the length and width of tumor tissues were measured at a 7-day interval and the tumor volume was determined by 0.5 × length × width2. After 28 days, mice were deeply anaesthetized with 5% isoflurane and executed by rapid dislocation of the neck. The xenograft tumor tissues were stripped and weighted. Then, protein expression in these xenograft tumor tissues was detected by Western blot.
Western Blot
Radio-immuno precipitation assay (RIPA) lysis buffer (Absin Biotechnology Ltd., Shanghai, China) was applied to extract the total proteins in HCT-116 cells and xenograft tumor tissues in line with the reagent instructions. Then this study used BCA kit (Beyotime, Shanghai, China) to determine the concentration of total protein samples. The detection procedure was carried out based on the instructions. A total of 50 μg total proteins was collected and separated by 10% sodium dodecyl sulfate-polyacrylamide gel electrophoresis. Followed by this, the proteins were transferred onto polyvinylidene fluoride (PVDF, Millipore, Bedford, MA, USA) membranes for the blockage by 5% skimmed milk (for 1 h at room temperature). Next, the proteins were probed by primary antibody (overnight at 4°C) and then secondary antibody (for 2 h at room temperature). Enhanced chemiluminescent kit (AmyJet Scientific, Wuhan, China) was responsible for blot visualization and Image J software (National Institutes of Health, Bethesda, Maryland, USA) for blot qualification.
The primary antibodies used in this experiment were: anti-C-Myc (ab152146, Abcam, Shanghai, China), anti-Cyclin D1 (YT706-YMC, Biolab Technology Co., Beijing, China), anti-Caspase-3 (YT691-QRP, Biolab Technology Co., Beijing, China), anti-BCL-2 (ab59348, Abcam, Shanghai, China), anti-BAX (ab53154, Abcam, Shanghai, China), anti-p-PI3K (BY-3332R, Kibo Biotechnology Co., Shanghai, China), anti-PI3K (K22576-PEC, Biolab Technology Co., Beijing, China), anti-p-AKT (YT-638, Biolab Technology Co., Beijing, China), anti-AKT (YT636, Biolab Technology Co., Beijing, China), anti-p-mTOR (K24032, Biolab Technology Co., Beijing, China), anti-mTOR (YT823, Biolab Technology Co., Beijing, China) and anti-GAPDH (YT778, Biolab Technology Co., Beijing, China). The dilution for primary antibody was 1:1000. Goat anti-rabbit secondary antibody (abs20040, Absin Biotechnology Ltd., Shanghai, China) was diluted to 1:5000 before using.
Statistical Analysis
Data were shown in the form of mean ± standard deviation, and compared by either two-tailed paired Student’s t-test (between two groups) or one-way analysis of variance with Tukey’s post hoc test (among three or more groups). GraphPad Prism 6.0 software (GraphPad Software, San Diego, CA, USA) was used for statistical analysis and chart making. Statistical significance was defined as P < 0.05.
Results
Targets of AM for Colon Cancer
Based on the analysis of the Genecards, OMIM and Disgenet databases, a total of 1801 targets for colon cancer were screened. Besides, 315 relevant targets of AM were screened according to the prediction of the Pharmmapper, SwissTargetPrediction and Super-PRED databases. Then, targets of AM for colon cancer were screened by Venn diagram. Finally, a total of 107 AM targets for colon cancer were identified (, ). Subsequently, PPI network analysis was performed on these 107 targets using the String database, and the results are shown in . As a result, 106 targets shared common nodes, and the green nodes in the middle represented the larger degree values, such as AKT1, CCND1, SRC, etc. These genes might be important targets of AM in treating colon cancer.
Table 1 The 107 Targets of AM for Colon Cancer
GO and KEGG Analysis of AM Targets for Colon Cancer
This study adjusted P < 0.01 as the screening criterion in GO functional enrichment analysis. The AM targets for colon cancer have 75 associated terms at MF level, 1218 associated terms at BP level and 39 associated terms at CC level. The top 15 significant terms at MF level, BP level and CC level were, respectively, shown in . Further, in KEGG pathway enrichment analysis, we adjusted P < 0.05 as the screening criterion. There were 125 pathways associated with AM targets for colon cancer. The top 15 significant KEGG pathways are exhibited in .
Relationship of AM Core Targets for Colon Cancer with the Top 15 BP and KEGG Pathways
The core network of the top 10 core targets of AM for colon cancer is shown in . These core targets included AKT1, CCND1, SRC, EGFR, Caspase-3, ERBB2, ESR1, HIFIA, ALB and HSP90AA1. Moreover, the relationship of the 10 core targets to the top 15 BP terms and the top 15 KEGG pathways is exhibited in and . It could be found that the 10 core targets were involved in the top 15 BP terms and the top 15 KEGG pathways.
Figure 3 Relationship of AM core targets for colon cancer with the top 15 BP and KEGG pathways. (A) Core network of the top 10 core targets of AM for colon cancer. (B) Relationship of the core network with the top 15 BP terms in GO enrichment analysis. (C) Relationship of the core network with the top 15 KEGG pathways.
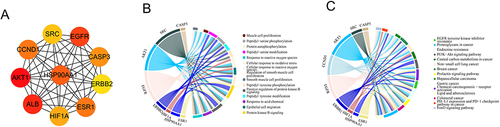
Hub Genes of AM for Colon Cancer
In order to screen the hub genes of AM for colon cancer, this study investigates the relationship between the AM targets for colon cancer and the top 15 KEGG pathways/the top 15 BP-related terms by constructing a “component-target-pathway” network. As shown in , PI3K, AKT and mTOR were finally selected as the hub gene as they possessed higher degree values. Thus, in the following study, the effect of AM on the malignant behavior of colon cells and the PI3K/AKT/mTOR pathway was explored by performing in vitro and in vivo study.
AM Inhibited the Proliferation, Invasion and Migration but Induced the Apoptosis of Colon Cells
AM at doses of 0–100 μM was utilized to treat HCT-116 cells in order to determine the IC50 value. As presented in , the IC50 value was 29.05 μM. In the following, AM at doses of 10, 20 and 40 μM were selected to treat HCT-116 cells. CCK-8 assay, colony formation assay, Transwell invasion assay, wound healing assay and flow cytometry were implemented to evaluate the effect of AM on the viability, proliferation, invasion, migration and apoptosis of HCT-116 cells. The results are exhibited in . AM at doses of 10, 20 and 40 μM distinctly weakened the viability, proliferation, invasion and migration, but induced the apoptosis of HCT-116 cells (P < 0.05 and P < 0.01). AM at a dose of 10 μM had no obvious effect on the invasion of HCT-116 cells. The expression of proteins related to proliferation and apoptosis was detected by Western blot, including C-Myc, Cyclin D1, Caspase-3, BCL2 and BAX. As supplied in , AM at doses of 10, 20 and 40 μM markedly increased the expression of Caspase-3 and BAX proteins, but decreased the expression of BCL-2, C-Myc and Cyclin D1 proteins in HCT-116 cells (P < 0.05 and P < 0.01). The promotion effect of AM at dose of 10 μM on Caspase-3 protein was insignificant. Collectively, AM possessed an inhibition effect on the proliferation, invasion and migration, but an induction effect on the apoptosis of colon cells.
Figure 5 AM inhibited the proliferation, invasion and migration but induced the apoptosis of colon cells. (A) CCK-8 assay revealed that the IC50 value of HCT-116 cells to AM was 29.05 μM. (B-F) CCK-8 assay, colony formation assay, Transwell invasion assay, wound healing assay and flow cytometry implied the suppression role of AM on the viability, proliferation, invasion and migration, but the induction role on the apoptosis of HCT-116 cells. (G) Western blot implied the promotion of AM on the expression of Caspase-3 and BAX proteins, but the inhibition on the expression of C-Myc, Cyclin D1 and BCL-2 proteins in HCT-116 cells. *P < 0.05 and **P < 0.01 vs HCT-116 cells treated by 0 μM AM.
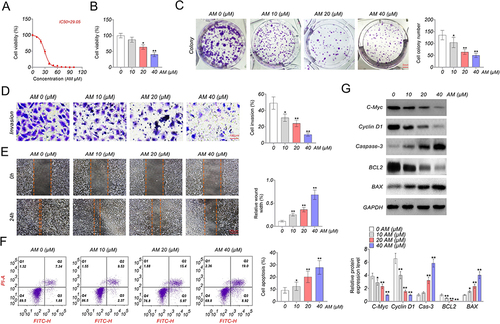
AM Inactivated the PI3K/AKT/mTOR Pathway in Colon Cells
This study investigated the effect of AM on the activity of the PI3K/AKT/mTOR pathway in HCT-116 cells by performing Western blot. The results are presented in . It could be discovered that, AM at doses of 10, 20 and 40 μM pronouncedly reduced the expression of p-PI3K/PI3K, p-AKT/AKT and p-mTOR/mTOR proteins in HCT-116 cells (P < 0.05 and P < 0.01). Meanwhile, AM at a dose of 10 μM did not obviously suppress the expression of p-mTOR/mTOR protein. Thereby, AM could inactivate the PI3K/AKT/mTOR pathway in colon cells.
AM Weakened Colon Cell Growth and Inactivated the PI3K/AKT/mTOR Pathway in vivo
After 28 days, mice were euthanized to harvest the xenograft tumor tissues. As revealed by and , mice with AM treatment had pronouncedly lower tumor volume and tumor weight, when relative to those without AM treatment (P < 0.05 and P < 0.01). Meanwhile, significantly higher levels of Caspase-3 and BAX proteins, but lower levels of C-Myc, Cyclin D1, BCL-2, p-PI3K/PI3K, p-AKT/AKT and p-mTOR/mTOR proteins were found in tumor tissues harvested from the AM treated mice, when compared to mice without AM treatment (P < 0.01) ( and ). Taken together, AM could suppress the in vivo growth of colon cells, and inactivated the PI3K/AKT/mTOR pathway in vivo. The work flow of the present study is shown in .
Figure 7 AM weakened colon cell growth and inactivated the PI3K/AKT/mTOR pathway in vivo. (A and B) Tumor-bearing nude mice were subjected to AM treatment (AM group) or not (Control group). AM treatment suppressed the in vivo growth of HCT-116 cells, as evidenced by the lower tumor volume and weight in the AM group. (C and D) AM treatment increased the expression of Caspase-3 and BAX proteins, reduced the expression of C-Myc, Cyclin D1 and BCL-2 proteins, and inactivated the PI3K /AKT/mTOR pathway in xenograft tumor tissues. *P < 0.05 and **P < 0.01 vs Control group.
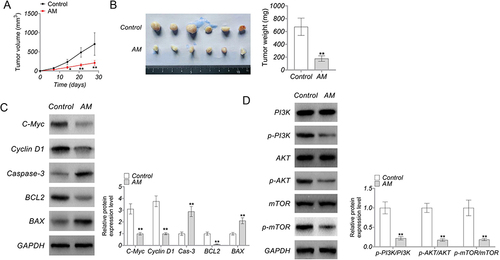
Discussion
In addition to surgical resection, the treatment of colon cancer includes post-operative adjuvant chemotherapy. The common chemotherapy drugs of colon cancer include fluoropyrimidine, oxaliplatin, capecitabine, folinic acid; besides, cetuximab, bevacizumab, pembrolizumab and panitumumab are the common immunotherapy drugs for colon cancer.Citation13 Recently, nanomedicines have shown their advantages in the treatment of colon cancer; for instance, resveratrol nanoformulations can greatly improve the solubility, stability and bioavailability of resveratrol, and control the release of resveratrol to enhance its anticancer activity.Citation14 As a kind of traditional Chinese medicine, AM has recently been discovered to have anti-inflammatory and antioxidant properties,Citation8,Citation15 and recently found to have anti-cancer activity in some human cancers.Citation9,Citation10 However, its effects and underlying mechanisms in colon cancer remains uncovered. This article firstly reported the role and mechanisms of AM for colon cancer via network pharmacology. Through the analysis of the online database, a total of 107 common targets of AM for colon cancer were found, and 10 core targets were discovered by establishing the PPI network, including AKT1, CCND1, SRC, EGFR, Caspase-3, ERBB2, ESR1, HIFIA, ALB and HSP90AA1. Previous reports have revealed that the up-regulated AKT1, CCND1, SRC and EGFR can drive the metastasis and drug-resistance of colon cancer.Citation16–19 The up-regulated HSP90AA1 in colorectal polyps has been suggested to be linked with the malignant lesions.Citation20 On the contrary, low ALB level has been discovered to predict a shorter survival of colon cancer patients.Citation21 Caspase-3 is a well-known pro-apoptotic protein. Interestingly, a recent study has found that, it not only helps to increase the sensitivity of colon cancer cells to chemotherapy, but also inhibits the invasion and metastasis of colon cancer cells.Citation22 ERBB2, ESR1 and HIFIA are also discovered to be associated with colon cancer.Citation23–25 Therefore, the 10 core targets exert important role in the progression of colon cancer, and they may be potential targets for AM in treating colon cancer.
By GO and KEGG enrichment analysis, this study identified the top 15 MF terms, BP terms, CC terms, and the top 15 significant KEGG pathways of the common targets of AM for colon cancer. The 10 core targets were found to be associated with the top 15 BP terms and KEGG pathways. In order to screen the hub genes, the relationship between the AM targets for colon cancer and the top 15 BP terms and KEGG pathway were explored by the construction of “component-target-pathway” network, and PI3K, AKT and mTOR were finally selected as the hub genes. The PI3K/AKT/mTOR pathway is one of the most frequently dysregulated cancer-related intracellular pathway whose abnormal activation can induce the malignant development of human cancers, including colon cancer.Citation26,Citation27 Some natural drugs have been researched to exert their anti-cancer activity in colon cancer by inactivating the PI3K/AKT/mTOR pathway.Citation28,Citation29 Thus, this study verified whether AM acts on the PI3K/AKT/mTOR pathway to treat colon cancer. Our data implied that AM treatment suppressed the in vitro malignant behavior and in vivo growth of colon cancer cells, and inhibited the activation of the PI3K/AKT/mTOR pathway both in vitro and in vivo. The activation of the PI3K/AKT/mTOR pathway can initiate the expression of CyclinD1 and C-Myc, and then CyclinD1 and C-Myc lead to the malignant development of cancer by accelerating the cell cycle progression.Citation30–33 This study suggested that AM possessed inhibition effect on the PI3K/AKT/mTOR pathway activity and the expression of CyclinD1 and C-Myc. Previous study has revealed that the anti-cancer function of AM is linked to its activation of pro-apoptotic factors (such as Caspase-3 and BAX) and inhibition of anti-apoptotic protein BCL-2.Citation9 Similarly, in this study, AM was proved to increase the expression of Caspase-3 and BAX, but reduced BCL-2 expression. Thus, these discoveries in this paper were consistent with the previous findings. At present, some natural substances have been found to exert the inhibition role in multiple cancers by suppressing the PI3K/AKT/mTOR pathway activity, and the suppression of the PI3K/AKT/mTOR pathway activity is thus considered to be an effective strategy for the treatment of cancers.Citation34,Citation35 According to the available literatures, AM can mitigate the H2O2-induced HepG2 cell damage by activating the ERK pathway;Citation8 besides, it can inactivate the JNK and p38 MAPK pathways to attenuate the lipopolysaccharide-induced inflammation in macrophages.Citation15 In terms of tumor, AM can activate the MAPK pathway to suppress the progression of gastric cancer.Citation9 However, the effect of AM on the PI3K/AKT/mTOR pathway activity has yet to be revealed. This was the first time that AM was demonstrated to block the progression of colon cancer by inactivating the PI3K/AKT/mTOR pathway. This finding provided an important clue for the application of AM in the clinical treatment of colon cancer.
Conclusion
In this work, the pharmacological mechanism of AM in colon cancer was investigated by network pharmacology prediction and in vivo and in vitro study validation. It was proved that AM might suppress the progression of colon cancer by inactivating the PI3K/AKT/mTOR pathway. Thus, AM may be a potential antitumor agent in colon cancer treatment.
Data Sharing Statement
All data in the manuscript are available through the responsible corresponding author.
Ethical Approval and Consent to Participate
All methods are reported in accordance with ARRIVE guidelines for the reporting of animal experiments.
The animal experiments conformed to the Guide for the Care and Use of Laboratory Animals. Animal study has been approved by the Animal Ethics Committee of Seventh People’s Hospital of Shanghai University of Traditional Chinese Medicine. (No. HB25a89)
All methods were performed in accordance with Declaration of Helsinki.
Author Contributions
All authors made a significant contribution to the work reported, whether that is in the conception, study design, execution, acquisition of data, analysis and interpretation, or in all these areas; took part in drafting, revising or critically reviewing the article; gave final approval of the version to be published; have agreed on the journal to which the article has been submitted; and agree to be accountable for all aspects of the work.
Disclosure
The authors declare that they have no conflicts of interest in this work.
Additional information
Funding
References
- Chong W, Shang L, Liu J, et al. m6 A regulator-based methylation modification patterns characterized by distinct tumor microenvironment immune profiles in colon cancer. Theranostics. 2021;11(5):2201–2217. doi:10.7150/thno.52717
- Hou W, Yi C, Zhu H. Predictive biomarkers of colon cancer immunotherapy: present and future. Front Immunol. 2022;13:1032314. doi:10.3389/fimmu.2022.1032314
- Liu M, Fu X, Jiang L, et al. Colon cancer cells secreted CXCL11 via RBP-Jκ to facilitated tumour-associated macrophage-induced cancer metastasis. J Cell Mol Med. 2021;25(22):10575–10590. doi:10.1111/jcmm.16989
- Khan H, Alam W, Alsharif KF, et al. Alkaloids and colon cancer: molecular mechanisms and therapeutic implications for cell cycle arrest. Molecules. 2022;27(3):920.
- Park MJ, Kang YH. Isolation of Isocoumarins and Flavonoids as α-Glucosidase Inhibitors from Agrimonia pilosa L. Molecules. 2020;25(11):567.
- Huang Q, Chen L, Teng H, et al. Phenolic compounds ameliorate the glucose uptake in HepG2 cells’ insulin resistance via activating AMPK. J Functional Foods. 2015;19:487–494.
- Lei E. Antioxidant Activities of Agrimonia pilosa Ledeb. J Plant Resources. 2014;27:420.
- Chen L, Teng H, Zhang KY, et al. Agrimonolide and Desmethylagrimonolide induced HO-1 expression in HepG2 Cells through Nrf2-transduction and p38 inactivation. Front Pharmacol. 2016;7:513. doi:10.3389/fphar.2016.00513
- Teng H, Huang Q, Chen L. Inhibition of cell proliferation and triggering of apoptosis by agrimonolide through MAP kinase (ERK and p38) pathways in human gastric cancer AGS cells. Food Funct. 2016;7(11):4605–4613. doi:10.1039/C6FO00715E
- Liu Y, Liu X, Wang H, et al. Agrimonolide inhibits cancer progression and induces ferroptosis and apoptosis by targeting SCD1 in ovarian cancer cells. Phytomedicine. 2022;101:154102. doi:10.1016/j.phymed.2022.154102
- Dong Y, Tao B, Xue X, et al. Molecular mechanism of Epicedium treatment for depression based on network pharmacology and molecular docking technology. BMC Complement Med Ther. 2021;21(1):222. doi:10.1186/s12906-021-03389-w
- Hopkins AL. Network pharmacology. Nat Biotechnol. 2007;25(10):1110–1111. doi:10.1038/nbt1007-1110
- Alfaro A, Murillo Castillo B, Cordero García E, et al. Colon cancer pharmacogenetics: a narrative review. . Pharmacy. 2022;10(4):95.
- Annaji M, Poudel I, Boddu SHS, et al. Resveratrol-loaded nanomedicines for cancer applications. Cancer Rep. 2021;4(3):e1353. doi:10.1002/cnr2.1353
- Chen L, Teng H, Fang T, et al. Agrimonolide from Agrimonia pilosa suppresses inflammatory responses through down-regulation of COX-2/iNOS and inactivation of NF-κB in lipopolysaccharide-stimulated macrophages. Phytomedicine. 2016;23(8):846–855. doi:10.1016/j.phymed.2016.03.016
- Yuan L, Zhou M, Huang D, et al. Resveratrol inhibits the invasion and metastasis of colon cancer through reversal of epithelial‑ mesenchymal transition via the AKT/GSK‑3β/Snail signaling pathway. Mol Med Rep. 2019;20(3):2783–2795. doi:10.3892/mmr.2019.10528
- Li Z, Zhu Z, Wang Y, et al. HSA‑miR‑15a‑5p inhibits colon cell carcinoma via targeting CCND1. Mol Med Rep. 2021;24(4). doi:10.3892/mmr.2021.12375
- Chen J, Elfiky A, Han M, et al. The role of Src in colon cancer and its therapeutic implications. Clin Colorectal Cancer. 2014;13(1):5–13. doi:10.1016/j.clcc.2013.10.003
- Cheng WL, Feng P-H, Lee K-Y, et al. The role of EREG/EGFR pathway in tumor progression. Int J Mol Sci. 2021;22(23):12828. doi:10.3390/ijms222312828
- Szczuka I, Wierzbicki J, Serek P, et al. Heat shock proteins HSPA1 and HSP90AA1 are upregulated in colorectal polyps and can be targeted in cancer cells by anti-inflammatory oxicams with Arylpiperazine Pharmacophore and Benzoyl Moiety Substitutions at Thiazine Ring. Biomolecules. 2021;11(11):1588. doi:10.3390/biom11111588
- Boonpipattanapong T, Chewatanakornkul S. Preoperative carcinoembryonic antigen and albumin in predicting survival in patients with colon and rectal carcinomas. J Clin Gastroenterol. 2006;40(7):592–595. doi:10.1097/00004836-200608000-00006
- Zhou M, Liu X, Li Z, et al. Caspase-3 regulates the migration, invasion and metastasis of colon cancer cells. Int J Cancer. 2018;143(4):921–930. doi:10.1002/ijc.31374
- Zhang S, Wang Y, Sun Y, et al. Hinokiflavone, as a MDM2 inhibitor, activates p53 signaling pathway to induce apoptosis in human colon cancer HCT116 cells. Biochem Biophys Res Commun. 2022;594:93–100. doi:10.1016/j.bbrc.2022.01.032
- Strickler JH, Yoshino T, Graham RP, et al. Diagnosis and treatment of ERBB2-positive metastatic colorectal cancer: a review. JAMA Oncol. 2022;8(5):760–769. doi:10.1001/jamaoncol.2021.8196
- Slattery ML, Lundgreen A, Welbourn B, et al. Oxidative balance and colon and rectal cancer: interaction of lifestyle factors and genes. Mutat Res. 2012;734(1–2):30–40. doi:10.1016/j.mrfmmm.2012.04.002
- Zhu ML, Zhang P-M, Jiang M, et al. Myricetin induces apoptosis and autophagy by inhibiting PI3K/Akt/mTOR signalling in human colon cancer cells. BMC Complement Med Ther. 2020;20(1):209. doi:10.1186/s12906-020-02965-w
- Yu L, Wei J, Liu P. Attacking the PI3K/Akt/mTOR signaling pathway for targeted therapeutic treatment in human cancer. Semin Cancer Biol. 2022;85:69–94. doi:10.1016/j.semcancer.2021.06.019
- Dai W, Dai YG, Ren DF, et al. Dieckol, a natural polyphenolic drug, inhibits the proliferation and migration of colon cancer cells by inhibiting PI3K, AKT, and mTOR phosphorylation. J Biochem Mol Toxicol. 2023;37(5):e23313. doi:10.1002/jbt.23313
- Zhang Y, Lin L, Wang Y, et al. Saikosaponin B4 suppression cancer progression by inhibiting SW480 and SW620 cells proliferation via the PI3K/AKT/mTOR pathway in colon cancer. Curr Cancer Drug Targets. 2022;22(11):889–903. doi:10.2174/1568009622666220627160834
- Liang H, Li Y, Wang F, et al. Combining network pharmacology and experimental validation to study the action and mechanism of water extract of asparagus against colorectal cancer. Front Pharmacol. 2022;13:862966. doi:10.3389/fphar.2022.862966
- Xie Y, Liu C, Zhang Y, et al. PKI-587 enhances radiosensitization of hepatocellular carcinoma by inhibiting the PI3K/AKT/mTOR pathways and DNA damage repair. PLoS One. 2021;16(10):e0258817. doi:10.1371/journal.pone.0258817
- Elbadawy M, Usui T, Yamawaki H, et al. Emerging roles of C-Myc in cancer stem cell-related signaling and resistance to cancer chemotherapy: a potential therapeutic target against colorectal cancer. Int J Mol Sci. 2019;20(9):2340. doi:10.3390/ijms20092340
- García-Gutiérrez L, Delgado MD, León J. MYC oncogene contributions to release of cell cycle brakes. Genes. 2019;10(3):4346.
- Narayanankutty A. PI3K/Akt/mTOR pathway as a therapeutic target for colorectal cancer: a review of preclinical and clinical evidence. Curr Drug Targets. 2019;20(12):1217–1226.
- Janku F, Yap TA, Meric-Bernstam F. Targeting the PI3K pathway in cancer: are we making headway? Nat Rev Clin Oncol. 2018;15(5):273–291.