Abstract
From a clinical perspective, local anesthetics have rather widespread application in regional blockade for surgery, postoperative analgesia, acute/chronic pain control, and even cancer treatments. However, a number of disadvantages are associated with traditional local anesthetic agents as well as routine drug delivery administration ways, such as neurotoxicity, short half-time, and non-sustained release, thereby limiting their application in clinical practice. Successful characterization of drug delivery systems (DDSs) for individual local anesthetic agents can support to achieve more efficient drug release and prolonged duration of action with reduced systemic toxicity. Different types of DDSs involving various carriers have been examined, including micromaterials, nanomaterials, and cyclodextrin. Among them, nanotechnology-based delivery approaches have significantly developed in the last decade due to the low systemic toxicity and the greater efficacy of non-conventional local anesthetics. Multiple nanosized materials, including polymeric, lipid (solid lipid nanoparticles, nanostructured lipid carriers, and nanoemulsions), metallic, inorganic non-metallic, and hybrid nanoparticles, offer a safe, localized, and long-acting solution for pain management and tumor therapy. This review provides a brief synopsis of different nano-based DDSs for local anesthetics with variable sizes and structural morphology, such as nanocapsules and nanospheres. Recent original research utilizing nanotechnology-based delivery systems is particularly discussed, and the progress and strengths of these DDSs are highlighted. A specific focus of this review is the comparison of various nano-based DDSs for local anesthetics, which can offer additional indications for their further improvement. All in all, nano-based DDSs with unique advantages provide a novel direction for the development of safer and more effective local anesthetic formulations.
Introduction
Local anesthetics serve as one of the most common strategies for anesthesia and analgesia during and after surgery, and induce temporary loss of pain through inhibiting transmission of nerve impulses.Citation1–3 In combination with immunotherapy, local anesthetics have been shown to exert a synergistic effect on cancer, which is similar to oncolytic treatments when targeted to lesional areas, thereby extending their traditional indications.Citation4,Citation5 While local anesthetics have been extensively applied in the clinic over the past century, two major disadvantages, specifically, neurotoxicity and short half-time, significantly limit their clinical utility.Citation6,Citation7 The majority of drugs, such as bupivacaine, lidocaine, and ropivacaine, have considerable clinical impacts but are associated with relatively short duration of action, and toxicity-induced neurological and cardiovascular manifestations.Citation8 Of note, many local anesthetics can induce hypertensive events and even serious outcomes, including refractory cardiac dysfunction, dysrhythmias, and prearrest situations due to their systemic toxicity.Citation9 In addition to toxicity, short duration of action is the other drawback, which needs to be improved for some particular conditions, as exemplified by intravertebral anesthesia. To eliminate or minimize these side-effects, drug delivery systems (DDSs) encapsulating local anesthetic agents have been developed for continuous release, which ensure that drug release is considerably slower and analgesia is maintained for a prolonged period with lower associated neurotoxicity.Citation10,Citation11
In general, an effective DDS requires the following features: reduced tissue reaction, efficient drug release capability, good stability, and steady degradation rates of a biodegradable carrier.Citation12,Citation13 A number of DDSs for local anesthetics have been developed for patient use due to promising biomedical advantages, as exemplified by Exparel™ (DepoFoam bupivacaine), the first formulation approved by the US Food and Drug Administration (FDA) since mid-2012.Citation14,Citation15 Exparel™, ie, bupivacaine encapsulated within multivesicular liposomes, is released extremely slowly over a prolonged duration of up to 72 hours. This unique commercial drug was primarily designed for surgical wound infiltration. However, special safety concerns were addressed by Burbridge and Jaffe,Citation16 highlighting the need to obtain further preclinical and clinical evidence of its safety and efficiency. Optimized DDSs for local anesthetics have attracted significant research interest and are under active experimental investigation. More recently, biomedical researchers have focused on the design and development of new DDSs to achieve efficient drug targeting for pain management and cancer treatment.
With the increasing application of nanotechnology in medicine, nano-based DDSs have been rapidly developed that can deliver local anesthetic agents to target sites with high specificity.Citation17,Citation18 Nanoparticles, referring to nanoscale (~10–200 nm in diameter) particles, devices, or systems, such as micelles, nanobrushes, or liposomes,Citation19–21 are the key components of nanomedicine and have received widespread interest as promising DDSs for cancer treatment. In a similar vein, nanoparticles have been explored as DDSs for local anesthetics.Citation17,Citation18 Notably, nano-based DDSs have been shown to provide a safe and effective alternative in perioperative anesthesia treatment.Citation22,Citation23 Their small molecular size enables drug delivery to previously inaccessible body sites. However, particle aggregation and toxicity restrict their translation from animal models to human studies. Among the various nanosized materials investigated to date, lipid nanoparticles are the first nanomedicine delivery system to advance from the laboratory concept to clinical application with high clinical acceptance. Furthermore, the diversity of nanomaterials greatly reduces the cost and expands the potential applications of nanoscale DDS.Citation24
Based on the background literature, a comprehensive overview of recent studies on local anesthetics using different types of nano-based DDSs is provided and their benefits and disadvantages are compared, with a view to providing meaningful indications for future research. With specific focus on the application of nanosized materials as drug delivery carriers, the latest literature in this field is summarized (). To our knowledge, the majority of current studies have validated the potential of nano-based DDSs in improving local anesthetic effects. However, considerable efforts are required to further optimize their properties. The existing limitations further highlight promising research directions in the clinical development of novel nano-based DDSs for therapeutic purposes.
Table 1 Recent Studies Using Different Nano-Based Drug Delivery Systems for Local Anesthetics
Current Drug Delivery Systems for Local Anesthetics
The major goal for clinical application of technological innovations in DDSs is improvement of the drug therapeutic index. The proposed formulations are designed to decrease the dosage of the anesthetic drug while increasing its absorption and permeability, maintain the anesthetic at the target site for a long duration and reduce its clearance. DDSs are generally classified into three categories according to application, specifically, quick performing, long-term and high effective DDSs, which are critical for selecting DDS-based local anesthetics for different types of pain management. The most commonly applied anesthetics for administration via DDS are bupivacaine and lidocaine.Citation48,Citation49 Encapsulation of these drugs in advanced DDSs, such as nano- and microparticles, should facilitate improvement of retention time in the body and prolonged drug release rates at the target site.Citation50 Local anesthetic delivery systems developed using a variety of inorganic materials (including microparticles, lipid nanoparticles, polymeric nanoparticles, or chitosan) have been comprehensively summarized in a recent review.Citation11 Nano-based DDSs for local anesthetics are discussed in detail in subsequent sections in the current review. In this section, we have mainly focused on several other DDS types, specifically, microparticles, and microneedles.
Microparticles range in size between 1 and 1000 μm and nanoparticle structures vary from 1 to 1000 nm. Compared to microparticle-based DDS, nanoparticle-based DDS is more compatible with the nanostructured biological environment, facilitating cellular penetration, bioavailability, and retention times.Citation22,Citation51 However, microparticles have undergone further development than nanoparticles due to technical limitations. The earliest study on application of microparticles for delivery of local anesthetics dates back to around 1981. Wakiyama et alCitation52,Citation53 encapsulated butamben, tetracaine, and dibucaine in polymeric systems and showed that the smaller sizes of microspheres promote faster drug release in vitro and in vivo. Horie et alCitation54 subsequently examined a lidocaine-loaded poly(lactic-co-glycolic) acid (PLGA) microparticle-based DDS in guinea pigs, which achieved 42% encapsulation efficiency and prolonged drug duration up to 3 days, supporting their potential for sustained release of lidocaine. Dai et al further reported a pronounced effect of carbamazepine-loaded microparticle-based DDS on pain relief, even up to two weeks, using a neuropathic pain rat model. Importantly, no toxicological or other side-effects were observed in behavioral and histochemical analyses.Citation55 Thus, microparticles continue to be a focus of research interest for the development of new DDSs for current anesthetics.
Microneedles, representing arrays of micron-sized needles, serve as an effective carrier in DDSs due to their minimally invasive and self-administrable properties, especially for transdermal delivery of local anesthetics. These molecules have been systematically investigated for their practicability as an alternative to traditional delivery methods for local anesthetics.Citation56,Citation57 A study by Baek et alCitation58 on lidocaine-coated poly(L-lactide) (PLLA) microneedle arrays revealed rapid local anesthesia in a painless manner. In their experiments, lidocaine was released into phosphate-buffered saline (PBS) within two minutes and remained stable for up to three weeks at different temperatures. In addition to local anesthetics, analgesics such as fentanyl have also been tested. Maurya et alCitation59 evaluated the effectiveness of soluble fentanyl microneedles in loco-regional anti-nociceptive activity. In their study, microneedle patches induced the onset of analgesic activity more rapidly than the adhesive dermal patch, but drug stability was not evaluated. Microneedles differ from most drug delivery carriers in terms of morphology and consequent effects. Combination of various nanocarriers with current microneedle delivery systems is proposed to significantly extend microneedle applications.Citation60,Citation61
Overall, several drug delivery carriers for local anesthetics have been developed, which remains a hot research topic. However, current explorations in this field are still limited to the experimental phase. Rapid progress in the development of DDSs has allowed manipulation of several biopharmaceutical and pharmacological properties of local anesthetics leading to improved therapeutic effects, highlighting their utility as promising alternatives for delivery and clinical application of local anesthetic agents.Citation62,Citation63
Current Nano-Based Drug Delivery Systems for Local Anesthetics
While nanomedicine is a rapidly growing field, its application for delivery of local anesthetics and pain management remains limited in terms of the complexity of pain physiology as well as the intractable nature of chronic pain.Citation64,Citation65 Nanotechnology involving the study and development of structures and/or devices within the nanometer size range plays a critical role in delivery of local anesthetics due to the advantages of smaller sized drug carriers.Citation66,Citation67 This strategy offers numerous benefits, especially for targeted delivery of anesthetic drugs at the required dose without affecting adjacent tissues.Citation68 Encapsulated local anesthetics by liposomes, as a classical example, bring great technical advances to achieve targeted delivery.Citation69 Nanoparticles have been further verified as an effective tool for postoperative pain relief with fewer side-effects, suggesting a promising future as DDSs for local anesthetics.Citation70,Citation71 Nanoparticles have multiple beneficial characteristics, such as small size, customizable surface, and improved solubility as well as multi-functionality,Citation72,Citation73 which are helpful in the development of new biomedical applications due to improved stability, solubility, bioavailability, and controlled release of drugs.
Over the years, researchers have gradually engineered a number of nanoparticle-based carriers, most of which are combined with different anesthetics for more efficient and targeted drug delivery. The latest literature focusing on nanoparticles for local anesthetic delivery is classified into polymeric, lipid and inorganic nanoparticle categories in the sections below (). In addition, a summary of lipid-polymer hybrid nanoparticle-based DDSs is provided, encompassing eight recent published studies in this field (). Some metallic nanoparticles display special intrinsic analgesic properties, such as zinc oxide, magnesium oxide and magnetite nanoparticles, as verified in mouse models and/or humans.Citation43,Citation44 Several nanoliposomal analgesic formulations have been commercialized, such as Exparel™ for local anesthetic delivery. In addition to the improvement and development of novel nanosized materials as well as manufacturing processes, combination of several nanocarriers and/or other materials provides an alternative strategy to improve therapeutic weaknesses and boost strengths. More recently, several experimental studies have focused on developing hybrid nanoparticle-hydrogel delivery systems for local anesthetics. For instance, Muniz et alCitation74 developed a hybrid hydrogel composed of polymeric nanocapsules for topical intraoral anesthesia in Wistar rats that was co-loaded with lidocaine and prilocaine to achieve effective and longer-lasting superficial anesthesia. Interestingly, stability of up to 6 months was reported for this nano-structured hydrogel formulation. Moreover, Campos et alCitation27 evaluated the potential of chitosan-coated polymeric nanoparticle-thermosensitive hydrogels for benzocaine delivery in prolonging local anesthetic therapy in both keratinocytes and fibroblasts. The collective studies highlight the advantages of anesthetic-loaded nanoparticle-hydrogel hybrid systems for drug delivery purposes.
Table 2 Lipid-Polymer Hybrid Nanoparticle-Based Drug Delivery Systems for Local Anesthetics
As mentioned above, nanosized materials show high efficiency in release of encapsulated drugs and significant value in pain control for patients. Similar to microneedles described in the previous section, nanoneedles have been engineered to provide new opportunities for pain management.Citation82 However, nanoscale DDSs are associated with a number of drawbacks, such as poor structural stability of liposomes, high surface-volume ratio-induced burst release of nanoparticles, and foreign body response of synthetic polymers.Citation22,Citation83–85 These limitations have encouraged increased efforts to further optimize nanoscale DDSs. In this review, we provide a summary of current nano-drug delivery systems with utility in delivery of local anesthetics, with particular emphasis on polymeric, lipid, inorganic non-metallic, metallic, and hybrid nanoparticles, which are described in detail below (see for details). Although these formulations have been tested in cell lines, animal models and even human specimens, the majority of studies are currently limited to the preclinical stage. Therefore, future research should focus on in-depth verification and clinical translation of mature nanoscale DDSs.
Figure 1 Currently applied nanoparticle-based drug delivery systems for local anesthetics. The four types of nanoparticle-based DDSs for local anesthetics are depicted, including polymeric nanoparticle-based, lipid nanoparticle-based, inorganic nanoparticle-based and hybrid nanoparticle-based DDSs. Created with BioRender.com.
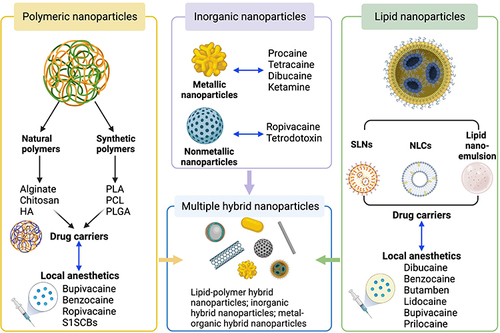
Polymeric Nanoparticle-Based Drug Delivery Systems for Local Anesthetics
Given their excellent applicability, polymeric nanoparticles are the most extensively studied drug delivery carriers to date, not only for local anesthetics,Citation11 but also anticancer agents.Citation86,Citation87 Wang et al recently presented a comprehensive review on polymer-based delivery platforms for local anesthetics, which addressed their advantages and key future challenges.Citation11 In the current review, we have focused on the different types of polymer-based nanoparticles and the relevant data obtained in this context. Polymer-based nanoparticles based on alginate, chitosan, poly-lactic acid (PLA), poly-ε-caprolactone (PCL) and poly(lactic-co-glycolic acid) (PLGA) have been tested in cell lines as well as animal models. Both natural and synthetic polymer systems are discussed in subsequent sections ( and ).
Natural Polymeric Nanoparticle-Based Delivery Systems
Alginate and chitosan, natural marine polysaccharides fabricated into different forms, have been utilized in biological applications for decades owing to their excellent mucosal adhesion, biodegradability, and biocompatibility.Citation88,Citation89 Due to the charge differences of the polymeric chains, alginate has strong electrostatic interactions with chitosan through ionotropic gelification, which protects drugs from the gastrointestinal tract and gastric environment.Citation90–92 Furthermore, alginate/chitosan nanoparticles have been successfully formulated as oral drug carriers utilizing alginate and/or chitosan as shells based on the ionic gel method.Citation92–94
Alginate/chitosan nanoparticles have also been evaluated for their utility in local anesthetic delivery, both separately and in conjunction. Grillo et alCitation25 prepared bupivacaine-loaded polymeric alginate nanoparticles using two different techniques and showed stability of both formulations for up to 30 days. Furthermore, low cytotoxicity, enhanced intensity, and prolonged duration were achieved by alginate nanoparticles in cytotoxic and pharmacological assays, supporting the potential of alginate nanoparticles as efficient vehicles for bupivacaine delivery. Cereda and coworkers conducted a preclinical evaluation of alginate/chitosan nanoparticle-based DDSs in loading of bupivacaine. Their data showed that this encapsulation prolonged the local anesthetic effect after infraorbital nerve blockade and altered the pharmacokinetic profile of the drug in New Zealand white rabbits in vivo.Citation26 Notably, bupivacaine-loaded alginate/chitosan nanoparticles significantly improved the total analgesic effect (by 1.4-fold) compared with bupivacaine solution, as demonstrated using the infraorbital nerve blockade test.Citation26 Moreover, Deng et alCitation18 constructed chitosan (CS) with genipin (GP) hydrogels as the hydrophilic lipid shell-loaded PCL nanocapsules as hydrophobic polymeric core composites (CS-GP/PCL) to deliver bupivacaine with the aim of prolonging anesthesia and pain relief ( and ). There was no obvious tissue damage in any of the test groups. Their other data demonstrated that the CS-GP/PCL polymeric hydrogel loaded with bupivacaine can improve the response to pain for at least 7 days compared to the control group. Overall, the clinical value of alginate/chitosan nanoparticle-based DDSs for local anesthetics has been preliminarily validated in vitro and in vivo. However, the safety issue requires further evaluation. Overall, alginate/chitosan nanoparticles dramatically improve the drug loading efficiency relative to the single component systems.
Figure 2 Representative study shows the potential of polymeric nanoparticle-based drug delivery systems for local anesthetics. (A) SEM micrographs of PCL nanocapsules (a) and CS-GP/PCL polymeric hydrogel (b). a. scale bar, 500 nm; b. scale bar, 2 μm. (B) Histopathological evaluation of the anti-inflammatory effects of bupivacaine-loaded CS-GP hydrogel and CS-GP/PCL polymeric hydrogel on 7, 14, and 21 days were stained with Masson’s trichrome stain. Cytoplasm and muscle fibers stain red, nuclei stains black, and collagen displays blue coloration. There was no obvious tissue damage in any of the test groups. Scale bar, 100 μm. Reproduced from Deng W, Yan Y, Zhuang P, et al. Synthesis of nanocapsules blended polymeric hydrogel loaded with bupivacaine drug delivery system for local anesthetics and pain management. Drug Deliv. 2022;29(1):399–412. Creative Commons.Citation18
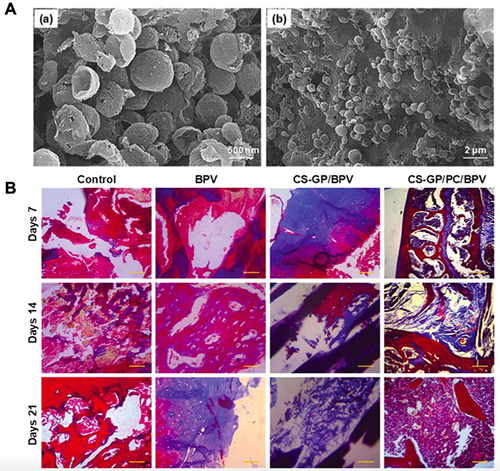
Synthetic Polymeric Nanoparticle-Based Delivery Systems
In addition to natural polymers, synthetic biodegradable polymers, such as PLA, PCL, PLGA, and peptide-drug conjugates, have been extensively studied in the context of tissue engineering and drug delivery.Citation95–97 In terms of pain management, PLGA remains the most attractive polymer for target-specific and controlled delivery of local anesthetics owing to its excellent biocompatibility, biodegradability and mechanical properties.Citation27–29,Citation98 Moreover, in combination with hydrogels, lipids, and polyethylene glycol (PEG), hybrid PLGA nanoparticle systems present a promising delivery strategy for prolonged local anesthetic therapy.
Campos and coworkers examined the utility of chitosan-coated PLGA polymeric nanoparticle-thermosensitive hydrogels as a benzocaine delivery strategy. Compared to nonencapsulated drug, both nanoparticles and hydrogels could regulate the delivery of benzocaine and increase its permeation rates. Furthermore, this effect was enhanced by the incorporation of limonene. The collective results support the efficacy of hydrogel-PLGA nanoparticle hybrids as an efficient delivery system to prolong the local anesthetic effects of benzocaine.Citation27 In 2007, Moraes et alCitation29 initially formulated PLGA nanospheres containing ropivacaine via the nanoprecipitation method and examined their physicochemical properties, cytotoxicity and stability.Citation29 Afterwards, the same research team characterized the functional properties of bupivacaine-loaded PLGA nanospheres, showing that the formulation decreased the toxicity of bupivacaine in 3T3 fibroblasts in vitro. Compared with microspheres, incorporation of bupivacaine into nanospheres can additionally generate monodisperse particles, which is regarded as its main advantage.Citation28
The final type of synthetic polymer for targeted drug delivery briefly discussed in this subchapter is peptide-drug conjugates,Citation99,Citation100 which offers a good choice for long-term accumulation of local anesthetics at the lesion site. Recently, Ji et alCitation17 reported a self-assembling delivery system for site-1 sodium channel blockers (S1SCBs), as exemplified by tetrodotoxin and saxitoxin, based on the specific interactions with two peptide sequences on the sodium channel. These targeted peptides were modified with hydrophobic domains and assembled into nanofibers. In their study, S1SCB-carrying nanofibers were shown to prolong nerve blockade and reduce systemic toxicity in the sciatic nerves of rats. In a similar vein, Zhao et alCitation101 showed that polymer-tetrodotoxin conjugates prolong the duration of nerve block, concomitant with minimal side-effects, in the same rat model. Thus, PLGA and peptide-drug conjugates serve as useful biocompatible polymers with broad applications for local anesthetic delivery.
Lipid Nanoparticle-Based Drug Delivery Systems for Local Anesthetics
Several comprehensive reviews have summarized lipid-based formulations for local anesthetics with extensive applications in skin, mucosa or parenteral injection, as well as potential treatment of pain control.Citation102–104 Here, we focused on the utility of lipid nanoparticle-based DDSs. Currently applied lipid nanoparticles for local anesthetics mainly include solid lipid nanoparticles (SLN), nanostructured lipid carriers (NLC), and lipid nanoemulsions, with evidence of promising prospects over the past decade. In addition, their morphology has been shown in . Original studies on the different types of lipid nanoparticle-based DDSs for local anesthetics are discussed in this section, with a view to providing an update on recent advances in this field.
Figure 3 Morphology of different types of lipid nanoparticles for local anesthetic delivery. (A) TEM micrographs of the SLN formulations SLNCPDBC, prepared by high-pressure homogenization, at two different magnifications: 100,000x (left) and 60,000x (right). Scale bar, 200 nm. Reproduced from de M Barbosa R, Ribeiro LNM, Casadei BR, et al. Solid lipid nanoparticles for dibucaine sustained release. Pharmaceutics. 2018;10(4):231. Creative Commons.Citation30 Licensee MDPI, Basel, Switzerland. (B) TEM images of optimised NLC formulation without (a) and with bupivacaine (b). Magnification, 60,000x. Scale bar, 200 nm. Reprinted from Int J Pharm, 529(1-2), Rodrigues da Silva GH, Ribeiro LNM, Mitsutake H, et al. Optimised NLC: a nanotechnological approach to improve the anaesthetic effect of bupivacaine. 253–263, Copyright 2017, with permission from Elsevier.Citation35
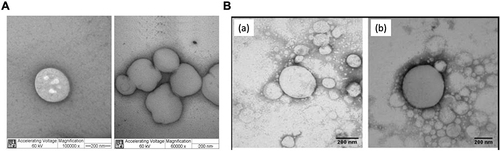
Solid lipid particulate systems encompassing SLNs, lipid microparticles and lipospheres are considered alternative carriers for therapeutic peptides, proteins and antigens. Among these, SLNs, the first generation of lipid nanoparticles and the most extensively studied drug carriers, are prepared using biodegradable and biocompatible lipids or lipid molecules.Citation102,Citation105,Citation106 Optimized pharmacokinetic and biological properties of local anesthetic-loaded SLNs have been determined for lidocaine, bupivacaine, prilocaine, dibucaine, and articaine. Basha et alCitation31 characterized benzocaine-loaded SLNs as a fast-acting and long-lasting topical formulation, both in vitro and in vivo. Using a tail-flick analgesiometer, more potent anesthetic effects were observed with benzocaine-loaded SLN hydrogels relative to benzocaine-loaded hydrogels, suggesting a remarkable improvement in both the intensity and duration of anesthetic effects with application of SLN. In addition, SLNs achieved sustained release of dibucaine with reduced intrinsic cytotoxicity and prolonged anesthetic effects, as established via a cytotoxicity test using fibroblasts and tail flick experiments on rats by Barbosa et alCitation30 These results collectively suggest that SLNs are safe carriers with significant potential in extending the applications of various local anesthetics via enhancing bioavailability.
NLCs are well-characterized second-generation lipid nanoparticles that serve as robust systems for the sustained release of local anesthetics. The major difference between SLNs and NLCs is the lipid core composition. SLNs contain solid lipids, whereas NLCs comprise a blend of solid and liquid lipids at room temperature, which can effectively prevent drug expulsion over time.Citation107,Citation108 Wang et alCitation34 constructed transcriptional transactivator peptide-modified lidocaine-loaded NLCs (TAT-NLCs-LID) that promoted the transdermal delivery of lidocaine by decreasing the pain threshold in mice, indicating their potential as a topical anesthetic system. Transdermal local anesthetic delivery, has several advantages, such as unaffected drug absorption, elimination of hepatic first-pass metabolism, and prevention of other adverse reactions caused by excessive accumulation in blood. Consistent with the findings of Wang et al, the group of Ribeiro reported NLCs as a promising system for topical lidocaine-prilocaine release, particularly in dental applications. This NLC formulation showed the best sustained release profile in studies on Franz diffusion cells, with release of 59% lidocaine and 66% prilocaine after 20 h.Citation36 Of interest, Franz diffusion cells are usually utilised to perform the drug diffusion tests. Rodrigues et alCitation35 reported a formulation of NLCs containing bupivacaine (BVC) S75:R25 with the introduction of products with enantiomeric excess (NLCBVC) that showed good stability for 12 months. Importantly, in vivo analgesia tests revealed a reduced dose of anesthetic and decreased systemic toxicity in rats administered NLCBVC. One year later, Zhao and coworkers engineered tocopheryl polyethylene glycol 1000 succinate (TPGS)-modified cationic NLC (TPGS-NLC) as a transdermal delivery system for lidocaine and investigated its effects in rats. TPGS-NLC induced higher rates and longer times of anesthesia compared to free lidocaine, inducing the maximum possible therapeutic effect.Citation33 More recently, da Silva et alCitation32 developed butamben-loaded NLCs to improve anesthesia in inflamed tissues, ie the sciatic nerve and gastrocnemius muscle of Wistar rats. This formula prolonged the release of butamben and reduced its cytotoxicity in vitro, with no toxic alterations in vivo. These findings validate the utility of NLCs in local anesthetic delivery.
Lipid-based nanoemulsions or nanoscale-sized emulsions were additionally examined as a thermodynamically stable system for drug delivery.Citation109,Citation110 Rachmawati et al explored the utility of castor oil-based nanoemulsions for the sustained delivery of bupivacaine hydrochloric acid (HCl),Citation37 which could present a new potential avenue for pain treatment. In addition, Natalini and coworkers have displayed that 15% isoflurane-loaded nanoemulsion showed an effective property to maintain general anesthesia in dogs.Citation40 And Kang et al demonstrated the self-nanoemulsifying effect of glycerol monostearate (GMS), leading to the increased release of lidocaine from the ointment.Citation39 Meanwhile, Maghbool et al investigated the local anesthetic effect of eugenol, a major ingredient of clove, in hemodialysis patients through a controlled cross-over trial, and revealed that eugenol nanoemulsion may obviously relieve pain.Citation38 These above-mentioned studies together stress the potential of lipid-based nanoemulsions in local anesthetic delivery.
Inorganic Nanoparticle-Based Drug Delivery Systems for Local Anesthetics
Since their introduction as drug carriers, inorganic nanoparticles have been extensively investigated for local anesthetic delivery, including metallic,Citation41–45 and non-metallicCitation46,Citation47 nanoparticles. In addition to their activity as drug carriers, inorganic nanoparticles have emerged as a promising strategy for cancer therapy with the capacity for combination with other therapeutic agents.Citation111,Citation112 Due to a number of important traits, for example, particle size distribution, higher affinity for pathologic cells, and controlled delivery properties, inorganic nanoparticles are considered an indispensable strategy for targeted local anesthetic delivery.Citation111,Citation113
Metallic nanoparticles have several advantages, such as high biocompatibility, non-toxicity, simple synthesis, and effective functionality, supporting the potential of this innovative technology for local anesthetic delivery. Gold and silver nanoparticles are considered superior to other types of metal nanoparticles. Mocanu et alCitation41,Citation42 gradually generated gold and silver nanoparticles and analyzed their interactions with procaine, tetracaine, and dibucaine. Initially, the group demonstrated that gold nanoparticles confer specific interactions to generate different self-assembly patterns, displaying remarkable differences in their behavior with the three anesthetics.Citation41 Subsequently, interactions between silver nanoparticles and these three anesthetics were evaluated,Citation42 providing a theoretical basis for the application of metal nanoparticles in local anesthetic delivery.Citation41,Citation42 However, no in vitro and/or in vivo functional experiments were conducted in this regard, which is the main limitation of their study. Subsequently, Lueshen et alCitation45 explored the value of gold-coated magnetite nanoparticles in a human spine model. Their results suggest that intrathecal magnetic drug targeting is a promising technique for concentrating and localizing drugs at target sites to achieve enhanced therapy for chronic pain and other diseases. Further experimental studies are needed to validate the efficiency of gold and silver nanoparticles for local anesthetic delivery, which could accelerate clinical applications of these formulations.
Metal oxide nanoparticles, such as zinc oxide (ZnO) and magnesium oxide (MgO) nanoparticles, have additionally been directly or indirectly examined in the context of local anesthetic delivery.Citation43,Citation44 Jahangiri et al demonstrated that MgO nanoparticles elicit greater analgesic and anti-inflammatory effects in mice via central and peripheral mechanisms compared to conventional MgO. Moreover, MgO nanoparticles potentiated the effects of ketamine, which could be mechanistically linked to the effect of magnesium on ketamine activity as an N-methyl-D-aspartate (NMDA) receptor blocker.Citation44 On the other hand, Wang et alCitation43 demonstrated that ZnO nanoparticles reduced neurogenic and inflammatory pain induced by chemicals and heat in Swiss Albino mice. This study highlighted the pharmacological properties of ZnO nanoparticles in different models of mice with nociceptive pain. ZnO nanoparticles have several advantages, such as inexpensive synthesis, lower toxicity, and multifunctional properties, supporting their application for targeted drug delivery.Citation114,Citation115 While metal oxide nanoparticles have not been assembled with local anesthetics in studies to date, these nanomaterials may serve as a promising alternative for local anesthetic delivery.
Non-metallic nanoparticles have additionally been introduced as safe drug delivery vehicles. Among inorganic non-metallic nanoparticles, hollow mesoporous organosilica nanoparticles (HMONs) have been extensively explored with regard to delivery of both local anesthetics,Citation46,Citation47 and anticancer drugs.Citation116,Citation117 Gao et alCitation46 further constructed ropivacaine-loaded HMONs, which promoted more controlled and sustained release of ropivacaine compared to single free ropivacaine injection in a mouse model of incision pain (). Tetrodotoxin encapsulation in hollow silica nanoparticles promoted tetrodotoxin-induced nerve blockade ( and ), as shown by Liu et al.Citation47 Fluorescent staining of frozen sections of rat nerves disclosed that hollow silica nanoparticles penetrate nerves in a size-dependent manner. In addition, low neurotoxicity and high biocompatibility of HMONs were observed in nanoparticle-mediated analgesia, providing a safe strategy for pain management.
Figure 4 In vivo imaging of inorganic nanoparticles for local anesthetic delivery. (A) In vivo fluorescence imaging of cy5.5@HMONs with or without two cycles of ultrasound irradiation around the sciatic nerve. Reproduced with permission from Gao X, Zhu P, Yu L, Yang L, Chen Y. Ultrasound/acidity-triggered and nanoparticle-enabled analgesia. Adv Healthc Mater. 2019;8(9):e1801350. Copyright © 2019, WILEY‐VCH Verlag GmbH & Co. KGaA, Weinheim.Citation46 (B) Representative fluorescence images of sciatic nerves and surrounding tissues 4 h after injection of FITC-SN10 (~10 nm) (a, b) and FITC-SN70 (~70 nm) (c). The red dotted line indicates the nerve perimeter. Scale bars, 200 μm. (C) Histology of rat tissues injected with free TTX and TTX-HSN30. Mild myotoxicity and inflammation was observed at 4 and 14 days after injection in animals administrated with free TTX and TTX-HSN30. (a−d) Representative H&E stained sections of muscles at the site of injection 4 and 14 days after injection. Scale bars, 200 μm (left), 50 μm (right). (e−g) Representative toluidine blue-stained sections of sciatic nerves from animals without (e) and with (f and g) injection of TTX-HSN30. Harvested 4 days after injection (f) and 14 days after injection (g). Scale bars, 100 μm. Reprinted with permission from Liu Q, Santamaria CM, Wei T, et al. Hollow silica nanoparticles penetrate the peripheral nerve and enhance the nerve blockade from tetrodotoxin. Nano Lett. 2018;18(1):32–37. Copyright © 2018, American Chemical Society.Citation47
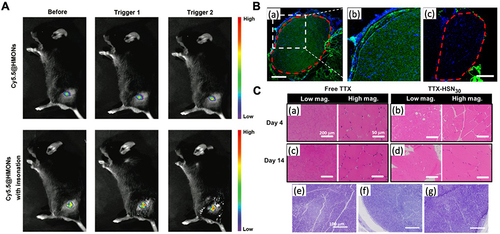
Hybrid Nanoparticle-Based Drug Delivery Systems for Local Anesthetics
Multiple hybrid nanocarriers, such as lipid-polymer hybrid nanoparticles (LPNs), inorganic hybrid nanoparticles, and metal-organic hybrid nanoparticles, have been comprehensively investigated.Citation118–120 Compared to non-hybrid nanocarriers, these hybrid counterparts have the capability of encapsulating multiple cargos and/or targeting agents.Citation121–123 Therefore, it is necessary to discuss about these hybrid nanoparticle-based DDSs for local anesthetics in this section, with a special emphasis on LPN-based DDSs.
Lipid-Polymer Hybrid Nanoparticle-Based Drug Delivery Systems
As one of the most common hybrid nanoparticles, LPNs consisting of polymer cores and single or multiple lipid shells have been extensively applied in the delivery of local anesthetics ( and ). LPNs effectively combine the advantages of polymeric and lipid nanoparticles to achieve enhanced therapeutic efficacy.Citation118,Citation124
Wang et alCitation75 showed efficient skin permeation ability and anesthetic effects of lidocaine-loaded LPNs, as confirmed from skin permeation experiments utilizing Franz diffusion cells and the tail flick latency test. In their study, the scheme () and the transmission electron microscope (TEM) images () of lidocaine-loaded LPNs were displayed. In a similar vein, Ma et al evaluated the local anesthetic effects of bupivacaine-loaded LPNs using fibroblasts in vitro and mice in vivo. In addition to prolonging the duration of anesthetic, LPNs showed good stability and slow drug leakage, concomitant with lower toxicity.Citation76 Subsequently, Khanal et al used chitosan as the nanoparticle core coated by a lipid nanocapsule to load ropivacaine and dexamethasone for musculoskeletal pain management. A significant improvement in pain response was observed with these nanocomposite carriers, supporting their clinical potential in controlling post-operative and neuropathic pain.Citation79
Figure 5 The application of lipid–polymer hybrid nanoparticles for local anesthetic delivery. (A) Scheme of the lidocaine-LPs and lidocaine-LPNs. (B) The TEM images of lidocaine-LPs and lidocaine-LPNs. Scale bar, 100 nm. Reproduced with permission from Wang J, Zhang L, Chi H, Wang S. An alternative choice of lidocaine-loaded liposomes: lidocaine-loaded lipid-polymer hybrid nanoparticles for local anesthetic therapy. Drug Deliv. 2016;23(4):1254–1260. Copyright 2016, Drug delivery. Journal website: http://www.tandfonline.com.Citation75
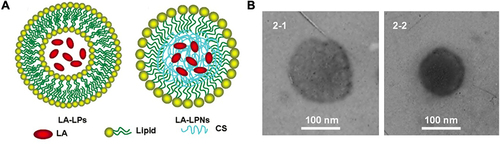
In the same year, Melo et alCitation78 characterized a hybrid formulation of articaine-loaded polymeric nanocapsules and SLNs in hydrogels as potential nanoparticulate systems for skin delivery, which significantly improved drug efficacy. In addition, a prolonged antinociceptive effect was mediated by hyaluronic acid (HA)-modified NLCs developed for transdermal bupivacaine delivery by Yue and coworkers compared with free bupivacaine.Citation77 Rodrigues et alCitation48 constructed a hybrid alginate-NLC formulation, which successfully extended the anesthetic effect of bupivacaine. Interestingly, a long-lasting anesthetic effect elicited by ropivacaine-loaded LPNs has been reported in both rats and mice, providing robust evidence of the clinical benefits of LPNs.Citation107 A recent comparison by Li et alCitation81 of the effects of NLCs and LPNs on the targeted delivery and efficacy of local anesthetics validated the superiority of the two systems in some respects. In summary, LPNs present a promising prospect for local anesthetic delivery.
Other Hybrid Nanoparticle-Based Drug Delivery Systems
In the last paragraph, we would like to briefly mention other hybrid nanoparticles used for local anesthetic delivery, mainly including inorganic hybrid nanoparticles as well as metal-organic hybrid nanoparticles. Even though these two kinds of hybrid nanoparticles have been rarely studied so far, they show a certain potential to improve structural and biological properties further, which is worth investigating.
Conclusions and Perspectives
For the design of novel DDSs for local anesthetics, a desirable system with a controllable and sustainable drug-releasing performance for an adequate analgesic effect is vital. The safety concern is particularly important, since effective delivery of anesthetic drugs is essential for patient safety. Tremendous breakthroughs have been made in the controlled release of local anesthetics to achieve longer duration of analgesic effects and better safety profiles, leading to the successful approval of Exparel™ in a clinical setting. The application of polymeric, lipid and inorganic nanoparticles, as well as various hybrid nanoparticles, has provided a wide range of choices for carrier selection and optimization. These personalized delivery systems endow both an extended range and opportunity to prolong the duration of action for each agent, with consequent maximization of local anesthetic effects.
Author Contributions
All authors made a significant contribution to the work reported; took part in drafting, revising or critically reviewing the article; gave final approval of the version to be published; have agreed on the journal to which the article has been submitted; and agree to be accountable for all aspects of the work.
Disclosure
All the authors declare no competing interests in this work.
Acknowledgments
This work was supported by the project of Jilin Provincial Finance Department (No. 2020SCZT015), and the project of the National Health Commission of Jilin Province (No. 2022LC109) to Z.P, and by the project of Jilin Provincial Finance Department (No. 2020SCZT070), and the project of the Jilin Science and Technology Department (No. YDZJ202201ZYTS613) to H.M.
References
- Moore PA, Hersh EV. Local anesthetics: pharmacology and toxicity. Dent Clin North Am. 2010;54(4):587–599. doi:10.1016/j.cden.2010.06.015
- Barletta M, Reed R. Local anesthetics: pharmacology and special preparations. Vet Clin North Am Small Anim Pract. 2019;49(6):1109–1125. doi:10.1016/j.cvsm.2019.07.004
- Körner J, Albani S, Sudha Bhagavath Eswaran V, Roehl AB, Rossetti G, Lampert A. Sodium channels and local anesthetics-old friends with new perspectives. Front Pharmacol. 2022;13:837088. doi:10.3389/fphar.2022.837088
- Bezu L, Kepp O, Kroemer G. Local anesthetics and immunotherapy: a novel combination to fight cancer. Semin Immunopathol. 2022;2022. doi:10.1007/s00281-022-00960-6
- Wu Chuang A, Kepp O, Kroemer G, Bezu L. Direct cytotoxic and indirect, immune-mediated effects of local anesthetics against cancer. Front Oncol. 2022;11:821785. doi:10.3389/fonc.2021.821785
- Wei Y, Wu Y, Wen K, Bazybek N, Ma G. Recent research and development of local anesthetic-loaded microspheres. J Mater Chem B. 2020;8(30):6322–6332. doi:10.1039/d0tb01129k
- Byram SC, Bialek SE, Husak VA, et al. Distinct neurotoxic effects of select local anesthetics on facial nerve injury and recovery. Restor Neurol Neurosci. 2020;38(2):173–183. doi:10.3233/RNN-190987
- Long B, Chavez S, Gottlieb M, Montrief T, Brady WJ. Local anesthetic systemic toxicity: a narrative review for emergency clinicians. Am J Emerg Med. 2022;59:42–48. doi:10.1016/j.ajem.2022.06.017
- On’Gele MO, Weintraub S, Qi V, Kim J. Local Anesthetics, Local Anesthetic Systemic Toxicity (LAST), and liposomal bupivacaine. Clin Sports Med. 2022;41(2):303–315. doi:10.1016/j.csm.2021.12.001
- de Paula E, Cereda CM, Tofoli GR, Franz-Montan M, Fraceto LF, de Araújo DR. Drug delivery systems for local anesthetics. Recent Pat Drug Deliv Formul. 2010;4(1):23–34. doi:10.2174/187221110789957228
- Wang B, Wang S, Zhang Q, et al. Recent advances in polymer-based drug delivery systems for local anesthetics. Acta Biomater. 2019;96:55–67. doi:10.1016/j.actbio.2019.05.044
- El-Hammadi MM, Arias JL. An update on liposomes in drug delivery: a patent review (2014–2018). Expert Opin Ther Pat. 2019;29(11):891–907. doi:10.1080/13543776.2019.1679767
- Tabakoglu S, Kołbuk D, Sajkiewicz P. Multifluid electrospinning for multi-drug delivery systems: pros and cons, challenges, and future directions. Biomater Sci. 2022;2022. doi:10.1039/d2bm01513g
- McAlvin JB, Padera RF, Shankarappa SA, et al. Multivesicular liposomal bupivacaine at the sciatic nerve. Biomaterials. 2014;35(15):4557–4564. doi:10.1016/j.biomaterials.2014.02.015
- Gorfine SR, Onel E, Patou G, Krivokapic ZV. Bupivacaine extended-release liposome injection for prolonged postsurgical analgesia in patients undergoing hemorrhoidectomy: a multicenter, randomized, double-blind, placebo-controlled trial. Dis Colon Rectum. 2011;54(12):1552–1559. doi:10.1097/DCR.0b013e318232d4c1
- Burbridge M, Jaffe RA. Exparel®: a new local anesthetic with special safety concerns. Anesth Analg. 2015;121(4):1113–1114. doi:10.1213/ANE.0000000000000822
- Ji T, Li Y, Deng X, et al. Delivery of local anaesthetics by a self-assembled supramolecular system mimicking their interactions with a sodium channel. Nat Biomed Eng. 2021;5(9):1099–1109. doi:10.1038/s41551-021-00793-y
- Deng W, Yan Y, Zhuang P, et al. Synthesis of nanocapsules blended polymeric hydrogel loaded with bupivacaine drug delivery system for local anesthetics and pain management. Drug Deliv. 2022;29(1):399–412. doi:10.1080/10717544.2021.2023702
- Bauer TA, Horvat NK, Marques O, et al. Core cross-linked polymeric micelles for specific iron delivery: inducing sterile inflammation in macrophages. Adv Healthc Mater. 2021;10(19):e2100385. doi:10.1002/adhm.202100385
- Kappel C, Seidl C, Medina-Montano C, et al. Density of conjugated antibody determines the extent of Fc receptor dependent capture of nanoparticles by liver sinusoidal endothelial cells. ACS Nano. 2021;15(9):15191–15209. doi:10.1021/acsnano.1c05713
- Zhang H, Bussmann J, Huhnke FH, et al. Together is better: mRNA co-encapsulation in lipoplexes is required to obtain ratiometric co-delivery and protein expression on the single cell level. Adv Sci. 2022;9(4):e2102072. doi:10.1002/advs.202102072
- Zorzetto L, Brambilla P, Marcello E, et al. From micro- to nanostructured implantable device for local anesthetic delivery. Int J Nanomedicine. 2016;11:2695–2709. doi:10.2147/IJN.S99028
- de Paula E, Cereda CM, Fraceto LF, et al. Micro and nanosystems for delivering local anesthetics. Expert Opin Drug Deliv. 2012;9(12):1505–1524. doi:10.1517/17425247.2012.738664
- Santamaria CM, Woodruff A, Yang R, Kohane DS. Drug delivery systems for prolonged duration local anesthesia. Mater Today. 2017;20(1):22–31. doi:10.1016/j.mattod.2016.11.019
- Grillo R, de Melo NF, de Araújo DR, de Paula E, Rosa AH, Fraceto LF. Polymeric alginate nanoparticles containing the local anesthetic bupivacaine. J Drug Target. 2010;18(9):688–699. doi:10.3109/10611861003649738
- Cereda CMS, Mecatti DS, Papini JZB, et al. Bupivacaine in alginate and chitosan nanoparticles: an in vivo evaluation of efficacy, pharmacokinetics, and local toxicity. J Pain Res. 2018;11:683–691. doi:10.2147/JPR.S158695
- Campos EVR, Proença PLF, da Costa TG, De lima R, Fraceto LF, de Araujo DR. Using chitosan-coated polymeric nanoparticles-thermosensitive hydrogels in association with limonene as skin drug delivery strategy. Biomed Res Int. 2022;2022:9165443. doi:10.1155/2022/9165443
- Moraes CM, de Lima R, Rosa AH, de Paula E, Fraceto LF. Encapsulation of local anesthetic bupivacaine in biodegradable Poly(DL-lactide-co-glycolide) nanospheres: factorial design, characterization and cytotoxicity studies. Macromol Symp. 2009;281(1):106–112.
- Moraes CM, de Matos AP, de Lima R, Rosa AH, de Paula E, Fraceto LF. Initial development and characterization of PLGA nanospheres containing ropivacaine. J Biol Phys. 2007;33(5–6):455–461. doi:10.1007/s10867-008-9094-z
- de M Barbosa R, Ribeiro LNM, Casadei BR, et al. Solid lipid nanoparticles for dibucaine sustained release. Pharmaceutics. 2018;10(4):231. doi:10.3390/pharmaceutics10040231
- Basha M, El-Alim SH A, Kassem AA, El Awdan S, Awad G. Benzocaine loaded solid lipid nanoparticles: formulation design, in vitro and in vivo evaluation of local anesthetic effect. Curr Drug Deliv. 2015;12(6):680–692. doi:10.2174/1567201812666150703115126
- Rodrigues da Silva GH, Lemes JBP, Geronimo G, et al. Lipid nanoparticles loaded with butamben and designed to improve anesthesia at inflamed tissues. Biomater Sci. 2021;9(9):3378–3389. doi:10.1039/d1bm00077b
- Zhao X, Sun Y, Li Z. Topical anesthesia therapy using lidocaine-loaded nanostructured lipid carriers: tocopheryl polyethylene glycol 1000 succinate-modified transdermal delivery system. Drug Des Devel Ther. 2018;12:4231–4240. doi:10.2147/DDDT.S187177
- Wang Y, Wang S, Shi P. Transcriptional transactivator peptide modified lidocaine-loaded nanoparticulate drug delivery system for topical anesthetic therapy. Drug Deliv. 2016;23(9):3193–3199. doi:10.3109/10717544.2016.1160459
- Rodrigues da Silva GH, Ribeiro LNM, Mitsutake H, et al. Optimised NLC: a nanotechnological approach to improve the anaesthetic effect of bupivacaine. Int J Pharm. 2017;529(1–2):253–263. doi:10.1016/j.ijpharm.2017.06.066
- Ribeiro LN, Franz-Montan M, Breitkreitz MC, et al. Nanostructured lipid carriers as robust systems for topical lidocaine-prilocaine release in dentistry. Eur J Pharm Sci. 2016;93:192–202. doi:10.1016/j.ejps.2016.08.030
- Rachmawati H, Arvin YA, Asyarie S, Anggadiredja K, Tjandrawinata RR, Storm G. Local sustained delivery of bupivacaine HCl from a new castor oil-based nanoemulsion system. Drug Deliv Transl Res. 2018;8(3):515–524. doi:10.1007/s13346-018-0497-5
- Maghbool M, Khosravi T, Vojdani S, et al. The effects of eugenol nanoemulsion on pain caused by arteriovenous fistula cannulation in hemodialysis patients: a randomized double-blinded controlled cross-over trial. Complement Ther Med. 2020;52:102440. doi:10.1016/j.ctim.2020.102440
- Kang JH, Yoo KH, Park HY, et al. Preparation and in vivo evaluation of a lidocaine self-nanoemulsifying ointment with glycerol monostearate for local delivery. Pharmaceutics. 2021;13(9):1468. doi:10.3390/pharmaceutics13091468
- Natalini CC, Krahn CL, Serpa PBS, Griffith JE, de Almeida RM. Intravenous 15% isoflurane lipid nanoemulsion for general anesthesia in dogs. Vet Anaesth Analg. 2017;44(2):219–227. doi:10.1016/j.vaa.2016.02.004
- Mocanu A, Pasca RD, Tomoaia G, Avranas A, Horovitz O, Tomoaia-Cotisel M. Selective effect of procaine, tetracaine and dibucaine on gold nanoparticles. J Nanosci Nanotechnol. 2012;12(12):8935–8939. doi:10.1166/jnn.2012.6707
- Mocanu A, Pasca RD, Tomoaia G, et al. New procedure to synthesize silver nanoparticles and their interaction with local anesthetics. Int J Nanomedicine. 2013;8:3867–3874. doi:10.2147/IJN.S51063
- Wang Z, Que B, Gan J, et al. Zinc oxide nanoparticles synthesized from Fraxinus rhynchophylla extract by green route method attenuates the chemical and heat induced neurogenic and inflammatory pain models in mice. J Photochem Photobiol B. 2020;202:111668. doi:10.1016/j.jphotobiol.2019.111668
- Jahangiri L, Kesmati M, Najafzadeh H. Evaluation of analgesic and anti-inflammatory effect of nanoparticles of magnesium oxide in mice with and without ketamine. Eur Rev Med Pharmacol Sci. 2013;17(20):2706–2710.
- Lueshen E, Venugopal I, Kanikunnel J, Soni T, Alaraj A, Linninger A. Intrathecal magnetic drug targeting using gold-coated magnetite nanoparticles in a human spine model. Nanomedicine. 2014;9(8):1155–1169. doi:10.2217/nnm.13.69
- Gao X, Zhu P, Yu L, Yang L, Chen Y. Ultrasound/acidity-triggered and nanoparticle-enabled analgesia. Adv Healthc Mater. 2019;8(9):e1801350. doi:10.1002/adhm.201801350
- Liu Q, Santamaria CM, Wei T, et al. Hollow silica nanoparticles penetrate the peripheral nerve and enhance the nerve blockade from tetrodotoxin. Nano Lett. 2018;18(1):32–37. doi:10.1021/acs.nanolett.7b02461
- Rodrigues da Silva GH, Geronimo G, Ribeiro LNM, et al. Injectable in situ forming nanogel: a hybrid Alginate-NLC formulation extends bupivacaine anesthetic effect. Mater Sci Eng C Mater Biol Appl. 2020;109:110608. doi:10.1016/j.msec.2019.110608
- Li Y, Zhao E, Li L, Bai L, Zhang W. Facile design of lidocaine-loaded polymeric hydrogel to persuade effects of local anesthesia drug delivery system: complete in vitro and in vivo toxicity analyses. Drug Deliv. 2021;28(1):1080–1092. doi:10.1080/10717544.2021.1931558
- de Souza MPC, de Camargo BAF, Spósito L, et al. Highlighting the use of micro and nanoparticles based-drug delivery systems for the treatment of Helicobacter pylori infections. Crit Rev Microbiol. 2021;47(4):435–460. doi:10.1080/1040841X.2021.1895721
- He Y, Qin L, Huang Y, Ma C. Advances of nano-structured extended-release local anesthetics. Nanoscale Res Lett. 2020;15(1):13. doi:10.1186/s11671-019-3241-2
- Wakiyama N, Juni K, Nakano M. Preparation and evaluation in vitro of polylactic acid microspheres containing local anesthetics. Chem Pharm Bull. 1981;29(11):3363–3368. doi:10.1248/cpb.29.3363
- Wakiyama N, Juni K, Nakano M. Preparation and evaluation in vitro and in vivo of polylactic acid microspheres containing dibucaine. Chem Pharm Bull. 1982;30(10):3719–3727. doi:10.1248/cpb.30.3719
- Horie RT, Sakamoto T, Nakagawa T, et al. Sustained delivery of lidocaine into the cochlea using poly lactic/glycolic acid microparticles. Laryngoscope. 2010;120(2):377–383. doi:10.1002/lary.20713
- Dai H, Tilley DM, Mercedes G, et al. Opiate-free pain therapy using carbamazepine-loaded microparticles provides up to 2 weeks of pain relief in a neuropathic pain model. Pain Pract. 2018;18(8):1024–1035. doi:10.1111/papr.12705
- Priya S, Singhvi G. Microneedles-based drug delivery strategies: a breakthrough approach for the management of pain. Biomed Pharmacother. 2022;155:113717. doi:10.1016/j.biopha.2022.113717
- Castilla-Casadiego DA, Carlton H, Gonzalez-Nino D, et al. Design, characterization, and modeling of a chitosan microneedle patch for transdermal delivery of meloxicam as a pain management strategy for use in cattle. Mater Sci Eng C Mater Biol Appl. 2021;118:111544. doi:10.1016/j.msec.2020.111544
- Baek SH, Shin JH, Kim YC. Drug-coated microneedles for rapid and painless local anesthesia. Biomed Microdevices. 2017;19(1):2. doi:10.1007/s10544-016-0144-1
- Maurya A, Rangappa S, Bae J, Dhawan T, Ajjarapu SS, Murthy SN. Evaluation of soluble fentanyl microneedles for loco-regional anti-nociceptive activity. Int J Pharm. 2019;564:485–491. doi:10.1016/j.ijpharm.2019.04.066
- Khan NH, Mir M, Qian L, et al. Skin cancer biology and barriers to treatment: recent applications of polymeric micro/nanostructures. J Adv Res. 2021;36:223–247. doi:10.1016/j.jare.2021.06.014
- Hao Y, Li W, Zhou X, Yang F, Qian Z. Microneedles-based transdermal drug delivery systems: a review. J Biomed Nanotechnol. 2017;13(12):1581–1597. doi:10.1166/jbn.2017.2474
- Hou Y, Meng X, Zhang S, Sun F, Liu W. Near-infrared triggered ropivacaine liposomal gel for adjustable and prolonged local anaesthesia. Int J Pharm. 2022;611:121315. doi:10.1016/j.ijpharm.2021.121315
- Cordeiro Lima Fernandes P, David de Moura L, Freitas de Lima F, Henrique Rodrigues da Silva G, Isaias Carvalho Souza R, de Paula E. Lipid nanocapsules loaded with prilocaine and lidocaine and incorporated in gel for topical application. Int J Pharm. 2021;602:120675. doi:10.1016/j.ijpharm.2021.120675
- Yin S, Gao P, Yu L, et al. Engineering 2D silicene-based mesoporous nanomedicine for in vivo near-infrared-triggered analgesia. Adv Sci. 2022;9(25):e2202735. doi:10.1002/advs.202202735
- Saleem M, Deal B, Nehl E, Janjic JM, Pollock JA. Nanomedicine-driven neuropathic pain relief in a rat model is associated with macrophage polarity and mast cell activation. Acta Neuropathol Commun. 2019;7(1):108. doi:10.1186/s40478-019-0762-y
- Peptu C, Rotaru R, Ignat L, et al. Nanotechnology approaches for pain therapy through transdermal drug delivery. Curr Pharm Des. 2015;21(42):6125–6139. doi:10.2174/1381612821666151027152752
- Moradkhani MR, Karimi A, Negahdari B. Nanotechnology application to local anaesthesia (LA). Artif Cells Nanomed Biotechnol. 2018;46(2):355–360. doi:10.1080/21691401.2017.1313263
- Liu GL, Bian WC, Zhao P, Sun LH. Delivery of local anesthesia: current strategies, safety, and future prospects. Curr Drug Metab. 2019;20(6):533–539. doi:10.2174/1389200220666190610155049
- Fatima MT, Islam Z, Ahmad E, Barreto GE, Md Ashraf G. Ionic gradient liposomes: recent advances in the stable entrapment and prolonged released of local anesthetics and anticancer drugs. Biomed Pharmacother. 2018;107:34–43. doi:10.1016/j.biopha.2018.07.138
- Babaie S, Taghvimi A, Hong JH, Hamishehkar H, An S, Kim KH. Recent advances in pain management based on nanoparticle technologies. J Nanobiotechnology. 2022;20(1):290. doi:10.1186/s12951-022-01473-y
- Bhansali D, Teng SL, Lee CS, Schmidt BL, Bunnett NW, Leong KW. Nanotechnology for pain management: current and future therapeutic interventions. Nano Today. 2021;39:101223. doi:10.1016/j.nantod.2021.101223
- Severino P, da Silva CF, Andrade LN, de Lima Oliveira D, Campos J, Souto EB. Alginate nanoparticles for drug delivery and targeting. Curr Pharm Des. 2019;25(11):1312–1334. doi:10.2174/1381612825666190425163424
- Singh R, Lillard JW. Nanoparticle-based targeted drug delivery. Exp Mol Pathol. 2009;86(3):215–223. doi:10.1016/j.yexmp.2008.12.004
- Muniz BV, Baratelli D, Di Carla S, et al. Hybrid hydrogel composed of polymeric nanocapsules co-loading lidocaine and prilocaine for topical intraoral anesthesia. Sci Rep. 2018;8(1):17972. doi:10.1038/s41598-018-36382-4
- Wang J, Zhang L, Chi H, Wang S. An alternative choice of lidocaine-loaded liposomes: lidocaine-loaded lipid-polymer hybrid nanoparticles for local anesthetic therapy. Drug Deliv. 2016;23(4):1254–1260. doi:10.3109/10717544.2016.1141259
- Ma P, Li T, Xing H, et al. Local anesthetic effects of bupivacaine loaded lipid-polymer hybrid nanoparticles: in vitro and in vivo evaluation. Biomed Pharmacother. 2017;89:689–695. doi:10.1016/j.biopha.2017.01.175
- Yue Y, Zhao D, Yin Q. Hyaluronic acid modified nanostructured lipid carriers for transdermal bupivacaine delivery: in vitro and in vivo anesthesia evaluation. Biomed Pharmacother. 2018;98:813–820. doi:10.1016/j.biopha.2017.12.103
- Melo NFS, Campos EVR, Franz-Montan M, et al. Characterization of articaine-loaded poly(ε-caprolactone) nanocapsules and solid lipid nanoparticles in hydrogels for topical formulations. J Nanosci Nanotechnol. 2018;18(6):4428–4438. doi:10.1166/jnn.2018.15235
- Khanal M, Gohil SV, Kuyinu E, et al. Injectable nanocomposite analgesic delivery system for musculoskeletal pain management. Acta Biomater. 2018;74:280–290. doi:10.1016/j.actbio.2018.05.038
- Li A, Yang F, Xin J, Bai X. An efficient and long-acting local anesthetic: ropivacaine-loaded lipid-polymer hybrid nanoparticles for the control of pain. Int J Nanomedicine. 2019;14:913–920. doi:10.2147/IJN.S190164
- Li M, Feng S, Xing H, Sun Y. Dexmedetomidine and levobupivacaine co-loaded, transcriptional transactivator peptide modified nanostructured lipid carriers or lipid-polymer hybrid nanoparticles, which performed better for local anesthetic therapy? Drug Deliv. 2020;27(1):1452–1460. doi:10.1080/10717544.2020.1831105
- Nitayavardhana S, Wanitphakdeedecha R, Ng JNC, Eimpunth S, Manuskiatti W. The efficacy and safety of fractional radiofrequency nanoneedle system in the treatment of atrophic acne scars in Asians. J Cosmet Dermatol. 2020;19(7):1636–1641. doi:10.1111/jocd.13484
- Kyriakides TR, Kim HJ, Zheng C, Harkins L, Tao W, Deschenes E. Foreign body response to synthetic polymer biomaterials and the role of adaptive immunity. Biomed Mater. 2022;17(2):10.1088/1748-605X/ac5574.
- Antimisiaris SG, Marazioti A, Kannavou M, et al. Overcoming barriers by local drug delivery with liposomes. Adv Drug Deliv Rev. 2021;174:53–86. doi:10.1016/j.addr.2021.01.019
- Alhowyan AA, Altamimi MA, Kalam MA, et al. Antifungal efficacy of Itraconazole loaded PLGA-nanoparticles stabilized by vitamin-E TPGS: in vitro and ex vivo studies. J Microbiol Methods. 2019;161:87–95. doi:10.1016/j.mimet.2019.01.020
- Zhao Y, Liu K, Li J, Liao J, Ma L. Engineering of hybrid anticancer drug-loaded polymeric nanoparticles delivery system for the treatment and care of lung cancer therapy. Drug Deliv. 2021;28(1):1539–1547. doi:10.1080/10717544.2021.1934187
- Ferrari R, Sponchioni M, Morbidelli M, Moscatelli D. Polymer nanoparticles for the intravenous delivery of anticancer drugs: the checkpoints on the road from the synthesis to clinical translation. Nanoscale. 2018;10(48):22701–22719. doi:10.1039/c8nr05933k
- Sudhakar S, Chandran SV, Selvamurugan N, Nazeer RA. Biodistribution and pharmacokinetics of thiolated chitosan nanoparticles for oral delivery of insulin in vivo. Int J Biol Macromol. 2020;150:281–288. doi:10.1016/j.ijbiomac.2020.02.079
- Taemeh MA, Shiravandi A, Korayem MA, Daemi H. Fabrication challenges and trends in biomedical applications of alginate electrospun nanofibers. Carbohydr Polym. 2020;228:115419. doi:10.1016/j.carbpol.2019.115419
- Liu L, Yao W, Xie X, Gao J, Lu X. pH-sensitive dual drug loaded janus nanoparticles by oral delivery for multimodal analgesia. J Nanobiotechnology. 2021;19(1):235. doi:10.1186/s12951-021-00974-6
- Della Torre C, Maggioni D, Nigro L, et al. Alginate coating modifies the biological effects of cerium oxide nanoparticles to the freshwater bivalve Dreissena polymorpha. Sci Total Environ. 2021;773:145612. doi:10.1016/j.scitotenv.2021.145612
- Li S, Zhang H, Chen K, et al. Application of chitosan/alginate nanoparticle in oral drug delivery systems: prospects and challenges. Drug Deliv. 2022;29(1):1142–1149. doi:10.1080/10717544.2022.2058646
- Hagras NA, Mogahed NMFH, Sheta E, et al. The powerful synergistic effect of spiramycin/propolis loaded chitosan/alginate nanoparticles on acute murine toxoplasmosis. PLoS Negl Trop Dis. 2022;16(3):e0010268. doi:10.1371/journal.pntd.0010268
- Li W, Li W, Wan Y, Wang L, Zhou T. Preparation, characterization and releasing property of antibacterial nano-capsules composed of ε-PL-EGCG and sodium alginate-chitosan. Int J Biol Macromol. 2022;204:652–660. doi:10.1016/j.ijbiomac.2022.01.123
- Duceac IA, Coseri S. Biopolymers and their derivatives: key components of advanced biomedical technologies. Biotechnol Adv. 2022;61:108056. doi:10.1016/j.biotechadv.2022.108056
- Maadani AM, Salahinejad E. Performance comparison of PLA- and PLGA-coated porous bioceramic scaffolds: mechanical, biodegradability, bioactivity, delivery and biocompatibility assessments. J Control Release. 2022;351:1–7. doi:10.1016/j.jconrel.2022.09.022
- Silva Favacho HA, Oliveira Do Couto R, Ferreira Duarte MP, et al. Synergy between surfactants and mucoadhesive polymers enhances the transbuccal permeation of local anesthetics from freeze-dried tablets. Mater Sci Eng C Mater Biol Appl. 2020;108:110373. doi:10.1016/j.msec.2019.110373
- Mir M, Ahmed N, Rehman AU. Recent applications of PLGA based nanostructures in drug delivery. Colloids Surf B. 2017;159:217–231. doi:10.1016/j.colsurfb.2017.07.038
- Hu Y, Wang Y, Deng J, et al. Enzyme-instructed self-assembly of peptide-drug conjugates in tear fluids for ocular drug delivery. J Control Release. 2022;344:261–271. doi:10.1016/j.jconrel.2022.03.011
- Vrettos EI, Mező G, Tzakos AG. On the design principles of peptide-drug conjugates for targeted drug delivery to the malignant tumor site. Beilstein J Org Chem. 2018;14:930–954. doi:10.3762/bjoc.14.80
- Zhao C, Liu A, Santamaria CM, et al. Polymer-tetrodotoxin conjugates to induce prolonged duration local anesthesia with minimal toxicity. Nat Commun. 2019;10(1):2566. doi:10.1038/s41467-019-10296-9
- de Araújo DR, Ribeiro LNM, de Paula E. Lipid-based carriers for the delivery of local anesthetics. Expert Opin Drug Deliv. 2019;16(7):701–714. doi:10.1080/17425247.2019.1629415
- Liu Y, Zhang J, Yu P, Niu J, Yu S. Mechanisms and efficacy of intravenous lipid emulsion treatment for systemic toxicity from local anesthetics. Front Med. 2021;8:756866. doi:10.3389/fmed.2021.756866
- Ok SH, Hong JM, Lee SH, Sohn JT. Lipid emulsion for treating local anesthetic systemic toxicity. Int J Med Sci. 2018;15(7):713–722. doi:10.7150/ijms.22643
- Almeida AJ, Souto E. Solid lipid nanoparticles as a drug delivery system for peptides and proteins. Adv Drug Deliv Rev. 2007;59(6):478–490. doi:10.1016/j.addr.2007.04.007
- de Araújo DR, da Silva DC, Barbosa RM, et al. Strategies for delivering local anesthetics to the skin: focus on liposomes, solid lipid nanoparticles, hydrogels and patches. Expert Opin Drug Deliv. 2013;10(11):1551–1563. doi:10.1517/17425247.2013.828031
- Agrawal M, Saraf S, Saraf S, et al. Recent strategies and advances in the fabrication of nano lipid carriers and their application towards brain targeting. J Control Release. 2020;321:372–415. doi:10.1016/j.jconrel.2020.02.020
- Müller RH, Ulrike A, Sinambela P, Keck CM. Nanostructured Lipid Carriers (NLC): the second generation of solid lipid nanoparticles. In: Percutaneous Penetration Enhancers Chemical Methods in Penetration Enhancement. Springer; 2016.
- Kadukkattil Ramanunny A, Singh SK, Wadhwa S, et al. Overcoming hydrolytic degradation challenges in topical delivery: non-aqueous nano-emulsions. Expert Opin Drug Deliv. 2022;19(1):23–45. doi:10.1080/17425247.2022.2019218
- Anand K, Rahman M, Ray S, Karmakar S. Insights into the approach, fabrication, application, and lacunae of nanoemulsions in drug delivery systems. Crit Rev Ther Drug Carrier Syst. 2020;37(6):511–551. doi:10.1615/CritRevTherDrugCarrierSyst.2020030291
- Amaldoss MJN, Yang J-L, Koshy P, Unnikrishnan A, Sorrell CC. Inorganic nanoparticle-based advanced cancer therapies: promising combination strategies. Drug Discov Today. 2022;27(12):103386. doi:10.1016/j.drudis.2022.103386
- Khorsandi K, Hosseinzadeh R, Sadat Esfahani H, Keyvani-Ghamsari S, Ur Rahman S. Nanomaterials as drug delivery systems with antibacterial properties: current trends and future priorities. Expert Rev Anti Infect Ther. 2021;19(10):1299–1323. doi:10.1080/14787210.2021.1908125
- Li W, Cao Z, Liu R, et al. AuNPs as an important inorganic nanoparticle applied in drug carrier systems. Artif Cells Nanomed Biotechnol. 2019;47(1):4222–4233. doi:10.1080/21691401.2019.1687501
- Alhujaily M, Albukhaty S, Yusuf M, et al. Recent advances in plant-mediated zinc oxide nanoparticles with their significant biomedical properties. Bioengineering. 2022;9(10):541. doi:10.3390/bioengineering9100541
- Liu M, Peng Y, Nie Y, et al. Co-delivery of doxorubicin and DNAzyme using ZnO@polydopamine core-shell nanocomposites for chemo/gene/photothermal therapy. Acta Biomater. 2020;110:242–253. doi:10.1016/j.actbio.2020.04.041
- Zhang H, Song F, Dong C, Yu L, Chang C, Chen Y. Co-delivery of nanoparticle and molecular drug by hollow mesoporous organosilica for tumor-activated and photothermal-augmented chemotherapy of breast cancer. J Nanobiotechnology. 2021;19(1):290. doi:10.1186/s12951-021-01025-w
- Yang Z, Fan W, Zou J, et al. Precision cancer theranostic platform by in situ polymerization in perylene diimide-hybridized hollow mesoporous organosilica nanoparticles. J Am Chem Soc. 2019;141(37):14687–14698. doi:10.1021/jacs.9b06086
- Khan MA, Khan S, Kazi M, et al. Norfloxacin loaded lipid polymer hybrid nanoparticles for oral administration: fabrication, characterization, in silico modelling and toxicity evaluation. Pharmaceutics. 2021;13(10):1632. doi:10.3390/pharmaceutics13101632
- Li P, Jia X, Zhang J, et al. The roles of gold and silver nanoparticles on ZnIn2S4/silver (gold)/tetra(4-carboxyphenyl)porphyrin iron(III) chloride hybrids in carbon dioxide photoreduction. J Colloid Interface Sci. 2022;628(12):831–839. doi:10.1016/j.drudis.2022.103386
- Coluccia M, Parisse V, Guglielmi P, Giannini G, Secci D. Metal-organic frameworks (MOFs) as biomolecules drug delivery systems for anticancer purposes. Eur J Med Chem. 2022;244:114801. doi:10.1016/j.ejmech.2022.114801
- Rajana N, Mounika A, Chary PS, et al. Multifunctional hybrid nanoparticles in diagnosis and therapy of breast cancer. J Control Release. 2022;352:1024–1047. doi:10.1016/j.jconrel.2022.11.009
- Elhassan E, Devnarain N, Mohammed M, Govender T, Omolo CA. Engineering hybrid nanosystems for efficient and targeted delivery against bacterial infections. J Control Release. 2022;351:598–622. doi:10.1016/j.jconrel.2022.09.052
- Khalili L, Dehghan G, Sheibani N, Khataee A. Smart active-targeting of lipid-polymer hybrid nanoparticles for therapeutic applications: recent advances and challenges. Int J Biol Macromol. 2022;213:166–194. doi:10.1016/j.ijbiomac.2022.05.156
- Fernandes RS, Arribada RG, Silva JO, et al. In vitro and in vivo effect of pH-sensitive PLGA-TPGS-based hybrid nanoparticles loaded with doxorubicin for breast cancer therapy. Pharmaceutics. 2022;14(11):2394. doi:10.3390/pharmaceutics14112394