Abstract
Background
The most common cause of myocardial infarction (MI) is stenotic atherosclerotic lesions in subepicardial coronary arteries. Artery disease progression induces clinical signs and symptoms, among which MI is the leader in mortality and morbidity. Recent studies have been trying to find new biochemical markers that could predict the evolution of clinical complications; among those markers, free fatty acids (FFA) and oxidative modification of low-density lipoproteins (oxidized LDL) have a special place.
Materials and methods
Seventy-nine ST-elevation MI patients were enrolled. The first group included MI patients without the signs of acute heart failure (Killip class I) while MI patients with Killip classes II–IV made up the second group. Thirty-three individuals with no cardiovascular disease were the controls. The lipid profile, serum oxidized LDL, and their antibodies, C-peptide and insulin were measured at days 1 and 12. The level of insulin resistance was assessed with the quantitative insulin sensitivity check index (QUICKI).
Results
MI patients had atherogenic dyslipidemia; however, the Killip II–IV group had the most pronounced and prolonged increase in FFA, oxidized LDL, and their antibodies. Additionally, positive correlations between FFA levels and creatine kinase activity (12 days, R = 0.301; P = 0.001) and negative correlations between the QUICKI index and FFA levels (R = −0.46; P = 0.0013 and R = −0.5; P = 0.01) were observed in the both groups.
Conclusion
The development of MI complications is accompanied by a significant increase in FFA levels, which not only demonstrate myocardial injury, but also take part in development of insulin resistance. Measuring FFA levels can have a great prognostic potential for risk stratification of both acute and recurrent coronary events and choice of treatment strategy.
Introduction
Nowadays, ischemic heart disease (IHD) remains one of the most relevant and unaddressed issues of contemporary medicine.Citation1,Citation2 Moreover, in all its manifestations, IHD is the first leading cause of mortality worldwide. Coronary atherosclerosis underlying IHD usually occurs and progresses silently, so CHD symptoms occur in the later stages of the disease when full recovery cannot be achieved. Recent studies have been trying to detect new biochemical markers that could show the evolution of clinical atherosclerosis complications; among them free fatty acids (FFA) have a special place.Citation1
Cell FFA carry out some key functions, including adenosine triphosphate (ATP) production. They act as cell-signal mediators (activation of various protein kinase C isoforms, apoptosis initiation), ligand transcription factors, and basic components of biological membranes.Citation2 FFA oxidation provides up to 70% of ATP to the heart; the other energy needs are satisfied by glucose oxidation. The intensity of FFA uptake by myocardial cells is defined, first of all, by their plasma concentrations.
Excess products and metabolites of fatty acid oxidation (acetyl-coenzyme A, reduced NADPH, FAD2) are natural inhibitors of pyruvate dehydrogenase complex enzymes, responsible for aerobic glucose oxidation, which leads to a decreased glucose myocardial utilization.Citation3 In ischemia, the main metabolic pathway providing energy to cardiomyocytes is anaerobic glycolysis because fatty acid oxidation requires more oxygen and altered myocardial utilization of fatty acids due to myocardial ischemia and necrosis results in fatty acid build-up in the blood.Citation4 Some researchers think that increased FFA concentrations are the earliest ischemia predictor, and more sensitive to the degree of ischemia than electrocardiography.Citation5 The prospective trial results of the Québec Cardiovascular Study show a strong relationship between increased plasma FFA concentrations and the risk of IHD development.Citation1 Moreover, according to Opie and Knuuti, FFA can be regarded as a potential biochemical marker of postinfarct myocardial remodeling.Citation6
Increased blood FFA concentrations are known to be strongly related to insulin resistance (IR), which is a metabolic risk factor for development of arterial diseases.Citation7 Recent publication highlighted the association between IR, atherosclerotic signs, and cardiovascular risk both in women and in men.Citation8 One of the suggested pathophysiologic mechanisms of such a phenomenon is the extensive production of activated oxygen metabolites in endothelial cells due to high levels of FFA and IR, which are able to induce oxidative modification of low-density lipoproteins (oxidized LDL) and synthesis of their antibodies, inducing atherosclerotic changes in the vessel wall, which eventually causes myocardial ischemia.Citation9,Citation10
A number of researchers suggested that FFA play an important role in atherosclerosis manifestation. Monitoring blood FFA levels in acute coronary events can play an important role in diagnostics and has prognostic value for choosing a strategy from a perspective of risk assessment in this category of patients.
This study was aimed at examining the dynamics of FFA levels and their relationship with IR markers and oxidized LDL and their antibodies in patients with acute myocardial infarction (MI).
Material and methods
Study subjects and design
Seventy-nine patients (50 males and 29 females, aged 63.8 ± 1.69 years) with ST elevation myocardial infarction (STEMI) were examined. The study design complied with the tenets of the Declaration of Helsinki and was approved by the Institutional Review Board of the Research Institute for Complex Issues of Cardiovascular Diseases, and all the patients signed the informed consent form before taking part in the study. MI was diagnosed according to the 2007 guidelines of the Russian National Cardiology Society. The inclusion criteria were chest pain (with no effect of nitroglycerin), signs of ischemia, ST segment elevation on electrocardiography and increased levels of cardiac enzymes: serum creatine kinase MB fraction (CK-MB) and troponin T. The patients were divided into two groups. Group I included 47 MI patients with no clinical signs of acute heart failure (Killip I) and group II enrolled 32 MI patients with acute heart failure (Killip II–IV) developed at day 1 from the onset of the disease. Coronary angiography showed that 58 patients (84.1%) had coronary lesions with >50% stenosis. The exclusion criteria were type 2 diabetes mellitus and laboratory signs of inflammation. The end diastolic (EDV, mL) and end systolic volumes (ESV, mL) reflecting structural and functional myocardial remodeling, which developed during MI, were 179.96 ± 11.04 mL and 157.19 ± 3.91 mL in Group I; and 78.52 ± 3.45 and 106.57 ± 10.46 in Group II, respectively. The echocardiography parameters were measured with the Aloka 5500 (SSD-5500 ProSound PHD; Hitachi Aloka Medical Ltd, Tokyo, Japan) in one- and two-dimensional modes with an ultrasound frequency of 3.5 MHz, according to a standard technique. Thirty-three age and sex-matched subjects without any cardiovascular disease were the control group.
Assays
Blood serum and plasma were tested. The tests were done at day 1 from MI and acute heart failure onset and at day 12 after the condition had been stabilized. Serum FFA, total cholesterol (TC), triglycerides (TG), LDL, very-low-density lipoproteins (VLDL), apolipoprotein B (apo B) as well as high-density lipoproteins (HDL) and apolipoprotein A (apo A) concentrations were measured with the standard Thermo Fisher Scientific test systems (Thermo Fisher Scientific Oy, Vantaa, Finland) on a Konelab 30i biochemistry analyzer (Thermo Fisher Scientific Oy) Serum oxidized LDL, their antibodies, C-peptide and insulin concentrations were measured by ELISA with BioMedica (Waterloo, NSW, Australia) and Diagnostic Systems Laboratories (Webster, TX, USA) lab kits respectively. A structured mathematical model was used to assess IR level based on fasting insulin, glucose values and calculating the quantitative insulin sensitivity check index (QUICKI) valuesCitation11 QUICKI = 1/[log (I0) + log (G0), where I0 was the baseline glycemia (mg/dL) and G0 was the baseline insulinemia (mMU/mL). According to Katz et al, the mean QUICKI index of 0.382 ± 0.007 correlates with normal tissue sensitivity to insulin while the QUICKI indexes of 0.331 ± 0.010 and 0.304 ± 0.007 correlate with moderate and intensive IR.Citation11
Statistical analysis
The results were statistically processed using nonparametric criteria; differences were considered to be statistically significant if P < 0.05. To determine the association between variables, Spearman’s correlation coefficient was calculated.
Results
Both groups had atherogenic dyslipidemia with higher TC, TG, LDL, VLDL, and apoB concentrations, greater apoB/apoA coefficient, and lower antiatherogenic HDL and apoA in healthy subjects than in the control group. (). There were no statistically significant differences in the lipid profiles of Killip I and II–IV patients.
Table 1 Variables of blood lipid-transport function in patients with myocardial infarction on the first day of the disease
FFA concentrations significantly differed in MI patients and healthy subjects as well as at different stages of the disease (). Indeed, at day 1 MI patients both with and without acute heart failure had, on average, sevenfold increased FFA levels than those in the control group. By day 12, FFA levels decreased, but were still 3.0 and 4.5 times (complicated and noncomplicated MI) higher than those in healthy subjects ().
Table 2 Free fatty acids (FFA) in patients with myocardial infarction and in healthy individuals
Both groups had a significant rise in glucose, insulin, and C-peptide concentrations in the acute stage of the disease compared to the control group. Meanwhile, Killip I MI patients still had a tendency towards increased concentrations of the above-mentioned parameters up to day 12. On the contrary, Killip II–IV patients had much more different concentrations of these parameters, but by day 12 insulin and C-peptide levels decreased significantly, even lower than in healthy subjects (), with glucose levels being consistently high. The QUICKI index in both groups significantly differed from that in the controls; in the Killip I group it correlated with moderate IR (according to Katz et alCitation11) and in the Killip II–IV group it correlated with intensive IR (). When the patients’ condition stabilized, the parameters under study did not change significantly.
Table 3 Markers of insulin resistance in patients with myocardial infarction and in healthy individuals
The correlation analysis showed a positive correlation between FFA and CK-MB activity at day 12, which demonstrated the size of myocardial necrosis (R = 0.301; P = 0.001) (). Besides, at day 1, the Killip II–IV group was found to have positive correlations between FFA levels and EDV (R = 0.34; P = 0.01) (), which proved a strong association between increased FFA and postinfarct myocardial remodeling. A negative correlation between the QUICKI index and FFA levels (R = −0.31; P = 0.0067) ( and ) was found in both groups.
Figure 1 Correlation between FFA and CK-MB activity at day 12 for the Killip II–IV group.
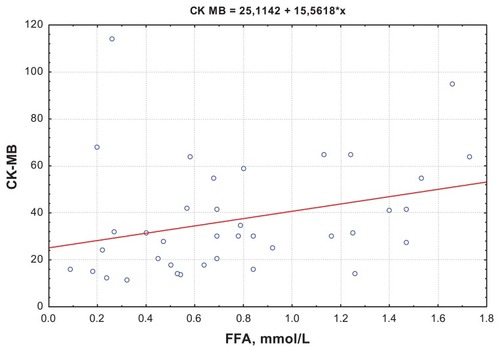
Figure 2 Correlation between FFA levels and EDV at day 1 for the Killip II–IV group.
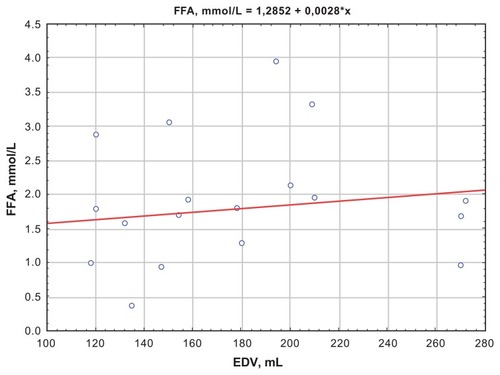
Figure 3 Correlations between the QUICKI index and FFA levels at day 12 for the Killip I group.
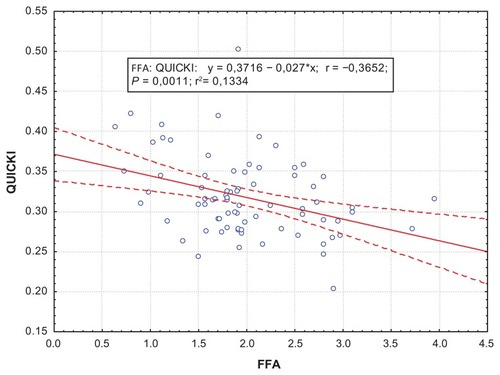
Figure 4 Correlations between the QUICKI index and FFA levels at day 12 for the Killip II–IV group.
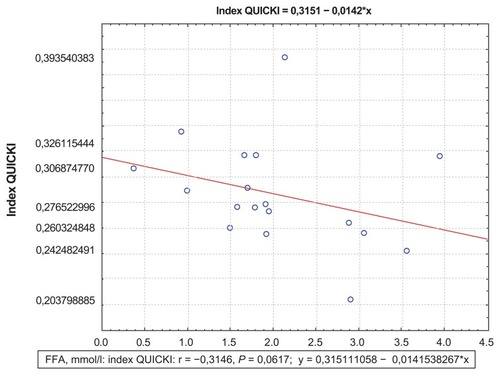
Oxidized LDL concentrations in MI patients changed according to the Killip class (). Both groups had higher oxidized LDL levels compared to those in the control group. Moreover, Killip II–IV patients had significantly higher oxidized LDL levels already at day 1 compared to those in Killip I patients. At day 12, both groups had a tendency toward increased oxidized LDL concentrations.
Table 4 Oxidized low-density lipoprotein content, their antibodies in the serum of patients with myocardial infarction and in normal subjects
Both MI patients with and without acute heart failure were found to have positive correlations between oxidized LDL and FFA concentrations (R = 0.49; P = 0.0009) (), as well as negative correlations between the QUICKI index and oxidized LDL concentrations (R = −0.64; P = 0.0002) Killip II–IV(). There was an increase in oxidized LDL antibodies in both groups compared to healthy subjects (). Moreover, in the complicated MI group, oxidized LDL antibody concentrations were significantly higher at day 1 than in the noncomplicated MI group. At day 12, antibody concentrations were still high in both groups.
Discussion
The pathogenetic role of lipid metabolism disorders in cardiovascular diseases is well known. The results of this study correlate with the medical literature; indeed, MI patients were observed to have atherogenic dyslipidemia and decreased antiatherogenic cholesterol. Besides, according to large epidemiology studies, there is a strong correlation between blood TC, LDL, and apoprotein concentrations and IHD mortality.Citation12 However, it was demonstrated that up to 20% of all cardiovascular events occurred with no altered lipid parameters of cardiovascular risk and about 40% of coronary accidents occurred with normal levels of TC and HDL.Citation12 People with normal HDL levels are known to have only 30% less coronary events than those with decreased HDL; the same situation is observed in normal LDL levels.Citation13
The results of the study demonstrate the increased apoprotein A in MI patients without acute heart failure. Apoprotein A-1 is known to serve as the main HDL component and makes up about 30% of the entire HDL particle. The primary function of this protein is to act as a cofactor for the lecithin cholesterol acyltransferase reaction and ensure cholesterol release from the peripheral tissue cells to the liver. Apparently, the increase in blood apo A in MI is due to the enhanced apoprotein production by the liver cells, which is aimed at the activation of cholesterol transport from the peripheral tissues. We found increased apoprotein B in MI patients with acute heart failure compared to healthy subjects. Apo B is known to be the key component of LDL and VLDL. It takes part in cholesterol transport from the liver to the tissues. The increase in blood apo B in MI is strongly associated with the increased LDL-cholesterol levels. These facts represent the basis for further research on lipid metabolism disorders and searching for new biochemical markers of coronary atherosclerosis and MI development. The measurement of FFA, which are considered to be signal molecules that play an important role in carbohydrate and lipid metabolism, can have a certain diagnostic value in assessing the risk of acute coronary accidents.
The obtained results show increased FFA both in patients without and with acute HF with the latter having a more marked FFA increase. This can be regarded as a result of metabolic derangements and disordered myocardial cellular energy homeostasis in this category of patients and the FFA levels as a prognostic indicator reflecting the intensity of those derangements. Previous studies showed that myocardial ischemia was accompanied by a sharp decrease in FFA uptake by cardiomyocytes with FFA, if there is sufficient oxygen, being the main energy substrate providing this ‘fuel’ to the beating heart muscle.
A decrease in coronary blood flow is accompanied by less energy being produced in the myocardium. If MI and its complications develop, the intensity of aerobic metabolism decreases and anaerobic glycolysis becomes the main mechanism providing energy to cardiomyocytes with lactate being its by-product.Citation4 Developing lactoacidosis causes the dissociation of tissue respiration and oxidative phosphorylation and cardiomyocyte overload with calcium ions, activating phospholipase A2 with further membrane injury by lipid peroxidation products. If there is no early reperfusion, at first reversible metabolic changes inevitably become irreversible and result in cell death.Citation7 Obviously, if MI or its complications develop, FFA increase does not only reflect myocardial ischemia, but may also act as a marker of the extent of myocardial necrosis. The results of the correlation analysis conducted in this study support this suggestion. Indeed, observed positive correlations between FFA levels at day 1 from the MI onset and CK-MB activity, showing the size of myocardial necrosis, as well as between FFA levels, ESV and EDV evidence show strong association between FFA and postinfarct myocardial remodeling.
It is suggested that FFA build-up in the blood during MI development can also be the cause of IR, which leads to a range of metabolic changes, contributing to development of MI complications. Hepatocytes intensively produce atherogenic lipoproteins slowing down antiatherogenic HDL synthesis. Besides, hepatocytes collect lipid metabolism by-products (ceramides, diacylglycerol), changing the transport of glucose into the cells and, consequently, increasing its concentrations in the blood.Citation7 Hepatic IR leads to decrease in glycogen production, glycogenolysis and gluconeogenesis activation, which together worsen hyperglycemia being, according to clinical studies, an independent risk factor for inhospital complications, adverse outcome, and mortality.Citation7
We found general common patterns in the dynamics of IR and some differences in their changes depending on the presence or absence of MI complications (). Indeed, this study demonstrated a significant increase in glucose, insulin, and C-peptide concentrations in both groups in the acute stage of the disease compared to those in the control group. The presence of IR in patients enrolled in the study was further confirmed by a low QUICKI index. An increase in the QUICKI index on day 12 in the complicated MI patients seems to be due to the decreased blood insulin. Apparently, FFA reduction results in a better insulin clearance, reduced hyperinsulinemia, and increase in QUICKI index.Citation14
Experimental IR, due to excess FFA, alters normal function of mitochondrial enzymes engaged in tissue respiration, which can potentially lead to reactive oxygen species production, result in lipoprotein oxidative modification, induce inflammation in vascular endothelium, and promote atherosclerotic plaque and ischemia progression.Citation14 Our study showed that oxidized lipoprotein levels increased both in the acute stage of the disease and during the whole hospital stay. In order to confirm the association between IR and atherogenic oxidized LDL presence in the blood, a correlation analysis was conducted. It demonstrated positive correlations between oxidized LDL, FFA concentrations as well as a negative correlation between the QUICKI index and oxidized LDL levels, which confirmed the presence of a pathogenetic relationship between the parameters under study.
LDL oxidation is not only accompanied by changes in their chemical properties, but also leads to immunocompetent cell activation, producing antibodies to a ‘hostile’ agent, and oxidized LDL elimination from the blood flow.
Antibodies to oxidized LDLs can be also found in healthy subjects; however, their concentrations are significantly increased in patients with other cardiovascular diseases.Citation15 This can be explained by the potential pathogenicity of these antibodies; increased levels of oxidized LDL and their antibodies form immune complexes and, binding with vascular intima, have additional damaging effects on the endothelium.Citation16 In this study, we found increased levels of oxidized LDL antibodies; moreover, patients with complicated MI had higher levels of these antibodies during the whole hospital stay.
The results obtained in this study correlate with the data of previous research, which demonstrated that increased levels of oxidized LDL antibodies did not only reflect the presence of IHD and were a marker of plaque instability, but also acted as a precursor of acute MI. Besides, based on the obtained data, measuring oxidized LDL antibody levels can help predict MI complications.
Conclusion
The results of this study show that MI and its clinical complications are accompanied by a significant increase in the level of FFA, which do not only reflect myocardial injury, but also take part in IR development and are a confounding factor for oxidized LDL. Furthermore, their antibodies build up in the blood flow, which has a powerful atherogenic potential.
Measurement of FFA levels can have a great prognostic value for risk stratification of both acute and recurrent coronary events and further choice of treatment strategy. High levels of FFA in MI, which still persist when the patients’ condition has been stabilized, may theoretically be considered a prerequisite for the use of this marker as one of the criteria to assess the risk of complications.
Disclosure
The authors report no conflicts of interest in this work.
References
- Pirro M Mauriege P Tchernof A Plasma free fatty acid levels and the risk of ischemic heart disease in men: prospective results from the Quebec cardiovascular study Atherosclerosis 2002 160 377 384 11849661
- Taegtmeyer H McNulty P Young ME Adaptation and maladaptation of the heart in diabetes: part I: general concepts Circulation 2002 105 1727 1733 11940554
- van der Vusse GJ van Bilsen M Glatz JF Hasselbaink DM Luiken J Critical steps in cellular fatty acid uptake and utilization Mol Cell Biochem 2002 239 9 15 12479563
- Lopaschuk GD Ussher JR Folmes CDL Myocardial fatty acid metabolism in health and disease Physiol Rev 2010 90 207 258 20086077
- Kleinfeld AM Prothro D Brown DL Davis RC Richieri GV DeMaria A Increases in serum unbound free fatty acid levels following coronary angioplasty Am J Cardiol 1996 78 1350 1354 8970405
- Opie LH Knuuti J The adrenergic-fatty acid load in heart failure J Am Coll Cardiol 2009 54 1637 1646 19850204
- Leclercq IA Da Silva Morais A Schroyen B Van Hul N Geerts A Insulin resistance in hepatocytes and sinusoidal liver cells: mechanisms and consequences J Hepatol 2007 47 142 156 17512085
- Huxley R Barzi F Woodward M Excess risk of fatal coronary heart disease associated with diabetes in men and women: meta-analysis of 37 prospective cohort studies BMJ 2006 332 73 78 16371403
- Lee RT Libby P The unstable atheroma J Arterioscler Thromb Vase Biol 1997 17 1859 1867
- Naruko T Ueda M Ehara S Persistent high levels of plasma oxidized low-density lipoprotein after acute myocardial infarction predict stent restenosis Arterioscler Thromb Vasc Biol 2006 26 877 883 16469945
- Katz A Nambi SS Mather K Quantitative insulin sensitivity check index: a simple, accurate method for assessing insulin sensitivity in humans J Clin Endocrinol Metab 2000 85 2402 2410 10902785
- Khot UN Khot MB Bajzer CT Prevalence of conventional risk factors in patients with coronary heart disease JAMA 2003 290 898 904 12928466
- Ansell BJ Navab M Hama S Inflammatory/antiinflammatory properties of high-density lipoprotein distinguish patients from control subjects better than high-density lipoprotein cholesterol levels and are favorably affected by simvastatin treatment Circulation 2003 108 2751 2756 14638544
- Balent B Goswami G Goodloe G Acute elevation of NEFA causes hyperinsulinemia without effect on insulin secretion rate in healthy human subjects Ann N Y Acad Sci 2002 967 535 543 12079885
- Wu R Lefvert AK Autoantibodies against oxidized low density lipoproteins (oxLDL): characterization of antibody isotype, subclass, affinity and effect on the macrophage uptake of oxLDL Clin Exp Immunol 1995 102 174 180 7554386
- Virella G Lopes-Virella MF Lipoprotein autoantibodies: measurement and significance Clin Diagn Lab Immunol 2003 10 499 505 12853376