Abstract
Biomaterials with high osteogenic activity are desirable for sufficient healing of bone defects resulting from trauma, tumor, infection, and congenital abnormalities. Synthetic materials mimicking the structure and composition of human trabecular bone are of considerable potential in bone augmentation. In the present study, a zinc (Zn)-doped mesoporous hydroxyapatite microspheres (Zn-MHMs)/collagen scaffold (Zn-MHMs/Coll) was developed through a lyophilization fabrication process and designed to mimic the trabecular bone. The Zn-MHMs were synthesized through a microwave-hydrothermal method by using creatine phosphate as an organic phosphorus source. Zn-MHMs that consist of hydroxyapatite nanosheets showed relatively uniform spherical morphology, mesoporous hollow structure, high specific surface area, and homogeneous Zn distribution. They were additionally investigated as a drug nanocarrier, which was efficient in drug delivery and presented a pH-responsive drug release behavior. Furthermore, they were incorporated into the collagen matrix to construct a biomimetic scaffold optimized for bone tissue regeneration. The Zn-MHMs/Coll scaffolds showed an interconnected pore structure in the range of 100–300 μm and a sustained release of Zn ions. More importantly, the Zn-MHMs/Coll scaffolds could enhance the osteogenic differentiation of rat bone marrow-derived mesenchymal stem cells. Finally, the bone defect repair results of critical-sized femoral condyle defect rat model demonstrated that the Zn-MHMs/Coll scaffolds could enhance bone regeneration compared with the Coll or MHMs/Coll scaffolds. The results suggest that the biomimetic Zn-MHMs/Coll scaffolds may be of enormous potential in bone repair and regeneration.
Introduction
Despite rapid developments in biotechnology and biomaterials, reconstruction of critical-sized bone defects resulting from tumor, infection, trauma, and congenital skeletal abnormalities remains a great challenge.Citation1–Citation3 For better restoration of large bone defects, bone tissue engineering scaffolds with both excellent osteoconductivity and osteoinductivity are desirable. Ideally, the scaffolds should not only act as physical template for the guidance of new bone ingrowth but also offer a stimulatory microenvironment to direct cellular differentiation. Autologous trabecular bone is widely regarded as the gold standard for bone defect repair. It serves as an ideal scaffold that provides not only growth factors and cells but also mechanical support after implantation into the defects.Citation4,Citation5 However, problems associated with its use include tissue availability, donor morbidity, and high care cost.Citation5 Hence, the development of biomimetic scaffolds resembling the composition and structure of autologous bone is hopeful to overcome these limitations.
So far, biomimicry has been demonstrated to be a powerful strategy to develop bone tissue engineering scaffolds that could sustain cellular proliferation, differentiation, and functionality similar to those normally occurring in living organisms.Citation6,Citation7 The hydroxyapatite/collagen composite scaffolds mimicking the structure and composition of trabecular bone were extensively investigated, and they have been shown to have great potential in bone repair and regeneration.Citation8–Citation10 The hydroxyapatite–collagen pairing naturally occurs in bone, whereby the collagen matrix provides strength and structural stability, whereas hydroxyapatite reinforces the organic matrix.Citation11 The organic matrix containing collagen and noncollagenous proteins plays an important role in mineralization, during which the organic matrix not only directs mineral deposition but also guides its growth.Citation12,Citation13 The hydroxyapatite/collagen composite scaffolds mimicking the composition of trabecular bone have been demonstrated to be biocompatible, biodegradable, osteoconductive, and osteoinductive in previous studies.Citation11,Citation14 However, compared with the hydroxyapatite/collagen composite scaffolds, the hydroxyapatite within human bone is characterized by a multitude of doping elements that substitute either phosphate or calcium in the crystal lattice. The apatite mineral in human bone mainly consists of non-stoichiometric apatite crystals, and CO32− is one of the most abundant doping ions.Citation15 Bone mineral also contains a series of trace elements such as zinc, magnesium, strontium, silica, and manganese.Citation16 Although they represent only a small fraction of mineral, they play a crucial role in bone metabolism.Citation17 Inspired by the idea that hydroxyapatite/collagen composite scaffolds mimic bone mineral chemistry and that trace elements regulate bone metabolism, the introduction of trace elements into hydroxyapatite/collagen composite scaffolds can theoretically improve their osteoinductive potential.
One of essential trace elements Zn plays an important role in the development and normal growth of skeletal system. Approximately 30% of total Zn in the body is stored in the bone tissue. Zn deficiency impairs the development of skeletal system and associates with the pathogenesis of osteoporosis.Citation18,Citation19 It is well accepted that Zn can stimulate bone formation and enhance osteogenic response in osteoblasts by increasing cell proliferation, osteogenesis-related gene expression, and extracellular matrix synthesis.Citation20–Citation22 Therefore, Zn has been introduced into various biomaterials with a view to improve their bone-forming ability. Indeed, Zn-incorporated bioactive glasses,Citation23 coatings,Citation24,Citation25 and calcium phosphate (CaP)Citation26 showed enhanced osteoinductive potential. However, the development of Zn-incorporated hydroxyapatite/collagen composite scaffolds has not yet been reported, and the effects of Zn on the biological responses of the composite scaffolds remain to be investigated.
CaP such as hydroxyapatite, as the main component of bone mineral, is widely used for the construction of biomimetic bone tissue engineering scaffolds. Among the CaP-based materials, nanostructured CaP porous microspheres attract increasing attention because of their high specific surface area and three-dimensional (3D) hierarchical structure, which make them favorable in the applications of drug delivery and tissue engineering. Recently, a series of nanostructured CaP porous microspheres has been developed in our laboratory through a microwave-hydrothermal method by using different organic phosphorus sources.Citation27–Citation29 These CaP porous microspheres were efficient in drug delivery because of their hierarchically porous nanostructure and high specific surface area. Compared with the CaP particles with a relatively large size (>10 μm) and the monoblock CaP bioceramic scaffolds, the nanostructured CaP microspheres with a smaller size (~1 μm) and a relatively high specific surface area are easier to degrade.Citation11 Moreover, the nanostructured CaP porous microspheres have a much larger surface area to volume ratio, and consequently, a composite scaffold may exhibit improved mechanical properties arising from strong interactions at interfaces. In view of these advantages, the nanostructured CaP porous microspheres may be good candidates for the fabrication of hydroxyapatite/collagen composite scaffolds.
In the present work, the (Zn)-doped mesoporous hydroxyapatite microspheres (Zn-MHMs) with 2 and 5 mol% of Zn substitution were synthesized through a microwave-hydrothermal method using creatine phosphate as an organic phosphorus source. The Zn-MHMs were first explored for drug delivery using doxorubicin hydrochloride (DOX) as a model drug. Furthermore, a novel biomimetic Zn-MHMs/Coll composite scaffold was constructed by incorporating the Zn-MHMs into collagen matrix through a lyophilization fabrication process. The cytocompatibility and osteoinductive potential of the Zn-MHMs/Coll scaffolds were evaluated in vitro by using the rat bone marrow-derived mesenchymal stem cells (rBMSCs), and the capacity to stimulate bone regeneration in vivo was assessed in a critical-sized femoral condyle defect rat model by micro-computed tomography (micro-CT) measurement and histological assay.
Materials and methods
Synthesis and characterization of Zn-MHMs
The Zn-MHMs with designed Zn/(Zn + Ca) molar ratios of 0, 0.02, and 0.05 were synthesized through a microwave-hydrothermal method by using creatine phosphate (Sangon Biotech, Shanghai, China) as the organic phosphorus source. The obtained products were labeled as MHMs, Zn2-MHMs, and Zn5-MHMs. In a typical synthesis of Zn5-MHMs, 0.2109 g CaCl2 and 0.0136 g ZnCl2 were first dissolved in 25 mL of deionized water. Then, an aqueous solution (15 mL) containing creatine phosphate (0.3926 g) was added dropwise to the above solution under constant stirring. The resulting solution was transferred into an autoclave (60 mL) and heated in a microwave oven (MDS-6; Sineo, Shanghai, China) at 100°C for 10 min. After cooling to the ambient temperature, the products were collected by centrifugation. The products of MHMs and Zn2-MHMs were synthesized under the same conditions as described earlier but by varying the Zn/(Zn + Ca) molar ratios of 0 and 0.02.
The as-obtained products were characterized by powder X-ray diffraction (XRD, Rigaku D/max 2550 V [Rigaku, Tokyto, Japan], CuKα radiation, λ=1.54178 Å), scanning electron microscopy (SEM, FEI Magellan 400; FEI, Hillsboro, OR, USA), transmission electron microscopy (TEM, Hitachi H-800; Hitachi, Tokyto, Japan), Brunauer–Emmett–Teller (BET) specific surface area analysis (V-sorb 2800P; Gold APP, Beijing, China), and inductively coupled plasma optical emission spectrometer (ICP-OES, JY 2000-2; Horiba, Paris, France).
In vitro drug loading and release
For drug loading, MHMs or Zn5-MHMs (100 mg) were dispersed into 15 mL of DOX solution (Aladdin, 2 mg mL−1) followed by ultrasonic treatment for 10 min. Then, the suspension was shaken with constant speed (120 rpm) in a sealed vessel at 37°C for 24 h. Finally, the DOX-loaded MHMs or Zn5-MHMs were collected by centrifugation and freeze-dried at −20°C. The DOX-loading capacities of MHMs and Zn5-MHMs were determined by measuring the DOX concentrations in the adsorbed supernatants using an ultraviolet–visible (UV-Vis) spectrophotometer (UV-2300; Techcomp, Shanghai, China) at 480 nm wavelength.
For the drug release assay, 20 mg of DOX-loaded MHMs or Zn5-MHMs was immersed into 15 mL of phosphate-buffered solution (PBS) at different pH values (7.4, 6.0, and 4.5) and shaken with constant speed (120 rpm) at 37°C. At given time intervals, the release medium (0.5 mL) was withdrawn for analysis with UV-Vis absorption spectroscopy at 480 nm wavelength and replaced with the same volume of fresh PBS.
rBMSCs isolation and culture
The rBMSCs were obtained from the femur and tibia of 4-week-old Sprague-Dawley (SD) rats. Briefly, the bone marrow was flushed out from the diaphysis with complete medium (CM, Dulbecco’s Modified Eagle’s Medium; Thermo Fisher Scientific, Waltham, MA, USA) supplemented with 10% fetal bovine serum (Thermo Fisher Scientific) and 1% (v/v) penicillin/streptomycin (Thermo Fisher Scientific). Then the cells were cultured at 37°C in a humidified atmosphere of 5% CO2. The nonadherent cells were removed after 48 h of culture. When they reached 80%–90% confluence, cells were passaged. The rBMSCs from passage 2 to 6 were used for the following experiments.
Scaffold fabrication
Four types of scaffolds corresponding to four groups were prepared: 1) Coll scaffold, 2) MHMs/Coll scaffold, 3) Zn2-MHMs/Coll scaffold, and 4) Zn5-MHMs/Coll scaffold. In a typical experiment, a collagen slurry (40 mg g−1) was prepared by mixing the collagen sponge (Kele Biological Technology Co. Ltd, Chengdu, China) with deionized water under magnetic stirring. Then, the as-prepared microspheres were added to the collagen slurry under vigorous stirring, and the weight ratio of microspheres to collagen was 3:7. For the latter process, the as-prepared slurries were added into a 24-well plate, frozen at −20°C for 24 h and freeze-dried at −20°C for 48 h. The freeze-dried scaffolds were chemically cross-linked using 20 mM N-(3-dimethylaminopropyl)-N′-ethylcarbodiimide hydrochloride (Sigma-Aldrich Co., St Louis, MO, USA) and 8 mM N-hydroxysuccimide (Sigma-Aldrich) in 80:20 ethanol/deionized water as described previously.Citation30 Finally, the scaffolds were rinsed with deionized water and ethanol three times, followed by sterilization with 29 kGy of 60Co radiation.
SEM observation of the scaffolds
Scaffolds were imaged both cell-free and following culturing with rBMSCs for 7 days. The rBMSCs were seeded on the scaffolds (Φ=10×2 mm, n=3) at a density of 1×105 cells/scaffold and cultured for 7 days, followed by fixation in 2.5% gluteraldehyde for 2 h and dehydration through a series of ethanol baths. After freeze-drying, the scaffolds were coated with platinum and observed using SEM.
Zn ions release test
The Zn ions release test was performed as follows: 20 mg of scaffolds (n=3) were immersed in 15 mL of PBS at 37°C under constant shaking (120 rpm) in a desk-type constant-temperature oscillator (THI-92A; Peiying, Suzhou, China). At given time intervals, 3 mL of release medium was collected to measure the concentration of Zn ions using ICP-OES and replaced with the same volume of fresh PBS.
Cell viability on scaffolds
The viability of rBMSCs on the scaffolds was evaluated using the Cell Counting Kit-8 assay (CCK-8; Dojindo Molecular Technologies Inc., Kumamoto, Japan). Briefly, the scaffolds (Φ=10×2 mm, n=3) were placed into a 48-well plate and seeded with rBMSCs at a density of 1×105 cells/scaffold. At days 1, 3, and 7, the medium was removed, and 500 μL of fresh medium containing 10% CCK-8 solution was added to each well. After incubation for 2 h, aliquots (100 μL) from each well were transferred to a 96-well plate for measurement. The absorbance was measured using a microplate reader (Bio-Rad 680; Bio-Rad Laboratories Inc., Hercules, CA, USA) at 450 nm wavelength.
Cytoskeleton staining
The rBMSCs were seeded on the scaffolds (Φ=10×2 mm, n=3) at a density of 1×105 cells/scaffold and cultured for 7 days. Then, the samples were rinsed and fixed in 4% paraformaldehyde for 30 min, followed by a pretreatment with Triton X-100 (0.5% v/v) for 5 min. Subsequently, the samples were stained with phalloidin-fluorescein isothiocyanate (FITC) (Sigma-Aldrich) and 4′,6-diamidino-2-phenylindole (DAPI) (Sigma-Aldrich) successively in darkness for 30 and 5 min, respectively, and observed using a confocal laser scanning microscope (LSM 510 meta; Zeiss, Jena, Germany).
Gene expression analysis
The effects of the scaffolds on the osteogenesis-related gene expression of rBMSCs were assessed by real-time quantitative polymerase chain reaction (RT-qPCR). Briefly, the rBMSCs were seeded on the scaffolds (Φ=10×2 mm, n=3) at a density of 2×105 cells/scaffold. At days 7 and 14, total RNA was isolated from cells on the scaffolds by homogenization in 1 mL of Trizol reagent (Thermo Fisher Scientific). Then, 500 ng of RNA was reversed transcribed into complementary DNA (cDNA) using PrimeScript 1st Strand cDNA Synthesis kit (Takara, Shiga, Japan) according to the manufacturer’s instructions. Quantification of cDNA was performed on an ABI7500 Thermal Cycler (Applied Biosystems, Carlsbad, CA, USA) by using a RT-PCR kit (SYBR Premix EX Taq; Takara). All assays were run in triplicate in three independent experiments. The primers for the selected genes were as follows: Alp (forward: TTCATAATTCCAGGCCGAAC; reverse: GGTTCACTCATGGAGGGTGT), Ocn (forward: AGGACCCTCTCTCTGCTCACT; reverse: ACCTTAC TGCCCTCCTGCTT), Runx2 (forward: CCACCACTCACTACCACACG; reverse: GGACGCTGACGAAGTACCAT), Gapdh (forward: GACATGCCGCCTGGAGAAAC; reverse: AGCCCAGGATGCCCTTTAGT).
Results were normalized to the level of housekeeping gene Gapdh. The expression of the selected genes of rBM-SCs on the MHMs/Coll and Zn-MHMs/Coll scaffolds was compared with that of cells on Coll scaffolds. The relative expression was calculated using the 2−ΔΔCt method.
Animal surgical procedures
All animal procedures were conducted in accordance with guidelines established by the Animal Research Committee of Sixth People’s Hospital, Shanghai Jiao Tong University School of Medicine, and this study was approved by this committee. Eighteen male SD (200–250 g) rats were randomly allocated into the following study groups: 1) Coll scaffold (n=6), 2) MHMs/Coll scaffold (n=6), and 3) Zn5-MHMs/Coll scaffold (n=6). After anesthesia, a lateral linear incision of ~1.5 cm was made on the lateral side of knee joint, followed by blunt dissection of the muscle to expose the femoral condyle. A bone tunnel 3.5 mm in diameter and 4 mm in depth was created perpendicular to the femoral condyle using a trephine under constant irrigation with normal saline. Then, a plug bone defect was created in the femoral condyle after removing the bone fragments. Finally, the defects were implanted with the Coll, MHMs/Coll, or Zn5-MHMs/Coll scaffolds (Φ=3.5×4 mm), and the incisions were closed layer by layer by using absorbable suture.
Micro-CT analysis
Eight weeks following operation, the rats were sacrificed. The femoral condyles were collected and fixed in 10% formaldehyde solution for 48 h. Subsequently, the specimens were scanned using micro-CT (SkyScan 1176; SkyScan, Kontich, Belgium) at a resolution of 18 μm. The following experimental settings were used: an X-ray voltage of 65 kVp, an anode current of 278 μA, and an exposure time of 520 ms for each of the 180 rotational steps. 3D images were reconstructed by using CTVox program (SkyScan). Bone volume to total volume ratio (BV/TV), trabecular number (Tb.N), trabecular spacing (Tb.Sp), and trabecular thickness (Tb.Th) in the defects were measured by using the CTAn program (SkyScan).
Histological observation
Following micro-CT scanning, the specimens were decalcified, dehydrated in ascending concentrations of ethanol solutions, and subsequently embedded in paraffin. Then, 5 μm-thick coronal sections of each specimen were prepared at the central region of the defects and stained with hematoxylin and eosin (HE), the dense and wax-like collagen distributed with nuclei was identified as the newly formed bone. Histomorphometry analysis was performed on representative images (n=5) of sections from each group by using an ImageJ image analysis software (National Institutes of Health [NIH], Bethesda, MD, USA). New bone area (%) = new bone area in each section/total area.
Statistical analysis
The data were presented as mean ± standard deviation. One-way analysis of variance and Tukey’s post hoc tests were performed to determine the level of significance. Significant differences were accepted at P<0.05.
Results
Characterization of the Zn-MHMs
shows the morphologies of the MHMs, Zn2-MHMs, and Zn5-MHMs observed by using SEM and TEM. It can be seen that all the products consisted of hydroxyapatite nanosheets that were hierarchically assembled into mesoporous hollow microspheres. These microspheres exhibited a relatively uniform size with an average diameter of ~1.5 μm. Inset in shows the energy dispersive spectroscopy (EDS) element mapping for the distribution of Zn, Ca, P, and O within Zn5-MHMs, indicating that Zn was homogeneously distributed within the Zn5-MHMs. As shown in , the Zn/(Zn + Ca) molar ratios in the MHMs, Zn2-MHMs, and Zn5-MHMs determined by ICP-OES were 0%, 3.03%, and 7.52%, respectively, which was higher than the nominal Zn/(Zn + Ca) molar ratios.
Table 1 Elemental composition of different samples measured by ICP-OES
Figure 1 SEM (top and middle rows) and TEM (bottom row) images: (A–C) MHMs, (D–F) Zn2-MHMs, (G–I) Zn5-MHMs. Inset in (H) shows the EDS element mapping for the distribution of Zn, Ca, P, and O in Zn5-MHMs.
Abbreviations: SEM, scanning electron microscopy; TEM, transmission electron microscopy; MHM, mesoporous hydroxyapatite microsphere; EDS, energy dispersive spectroscopy.
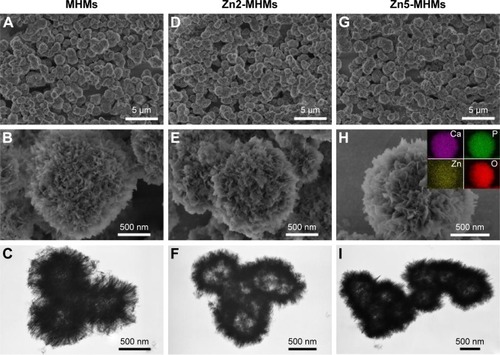
shows the XRD patterns of the MHMs, Zn2-MHMs, and Zn5-MHMs. It was clear that all the products were identified as a single phase of hydroxyapatite (JCPDS 09-0432). The BET specific surface area (SBET) of the MHMs, Zn2-MHMs, and Zn5-MHMs were 83.5, 105.7, and 131.0 m2 g−1, respectively, and the Barrett-Joyner-Halenda (BJH) desorption cumulative pore volumes (Vp) of the MHMs, Zn2-MHMs, and Zn5-MHMs were 0.59, 0.60, and 0.70 cm3 g−1, respectively (). The average BJH desorption pore sizes of MHMs, Zn2-MHMs, and Zn5-MHMs were 16.26, 12.81, and 8.49 nm, respectively ().
Figure 2 Physical characteristics of the MHMs, Zn2-MHMs, and Zn5-MHMs. (A) XRD patterns. (B) Nitrogen adsorption-desorption isotherms. (C) BJH pore size distributions.
Abbreviations: MHM, mesoporous hydroxyapatite microsphere; XRD, X-ray diffraction; BJH, Barrett-Joyner-Halenda; dV/dD, d(volume adsorbed)/d(diameter).
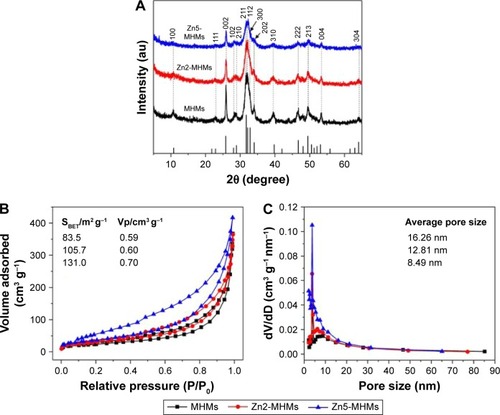
In vitro study of drug loading and release properties
The DOX-loading capacities of MHMs and Zn5-MHMs were 70.4 and 148.0 mg g−1, respectively. As shown in , both MHMs and Zn5-MHMs drug delivery systems exhibited a pH-responsive drug release behavior. As the pH of the medium decreased, the release rates of DOX became faster at the early stage of the drug release process. As time extends, the drug release rates of the MHMs and Zn5-MHMs drug delivery systems decreased gradually and reached a plateau. The cumulative release of DOX from the MHMs at pH values of 7.4, 6.0, and 4.5 was ~308 μg (23.45%), 781 μg (59.39%), and 1,057 μg (80.36%), respectively. And the cumulative release of DOX from the Zn5-MHMs at pH values of 7.4, 6.0, and 4.5 waŝ546 μg (21.19%), 1,361 μg (52.79%), and 2,032 μg (78.81%), respectively.
Figure 3 Drug release curves of (A) DOX-loaded MHMs and (B) DOX-loaded Zn5-MHMs in PBS solutions at different pH values. Cumulative release of DOX from (C) MHMs and (D) Zn5-MHMs as a function of the square root of the release time.
Abbreviations: DOX, doxorubicin hydrochloride; MHM, mesoporous hydroxyapatite microsphere; PBS, phosphate-buffered solution.
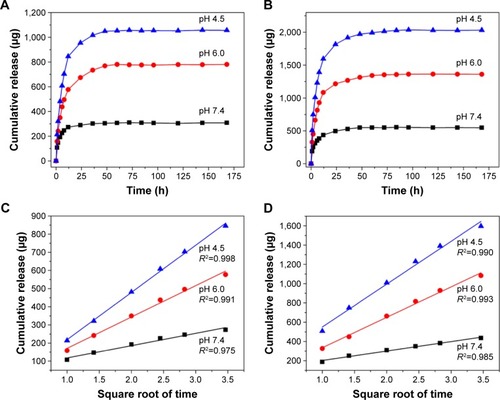
shows the relationships between the cumulative amount of released DOX and the square root of the release time for the MHMs and Zn5-MHMs drug delivery systems at different pH values, both exhibiting good linear relationships with high regression factors of >0.95.
Characterization of the scaffolds
As shown in , all the four types of scaffolds were highly porous with an interconnected pore structure in the range of 100–300 μm. Higher magnification images show that the surfaces of the MHMs/Coll, Zn2-MHMs/Coll, and Zn5-MHMs/Coll scaffolds were rough and homogeneously embedded with spherical particles, whereas the surface of the Coll scaffolds was quite smooth.
Figure 4 Physical characteristics of the Coll, MHMs/Coll, Zn2-MHMs/Coll, and Zn5-MHMs/Coll scaffolds. (A) SEM micrographs of the Coll (a–c), MHMs/Coll (d–f), Zn2-MHMs/Coll (g–i), and Zn5-MHMs/Coll scaffolds (j–l). (B) Release of Zn ions from the MHMs/Coll, Zn2-MHMs/Coll, and Zn5-MHMs/Coll scaffolds.
Abbreviations: MHMs/Coll, mesoporous hydroxyapatite microspheres/collagen scaffold; SEM, scanning electron microscopy.
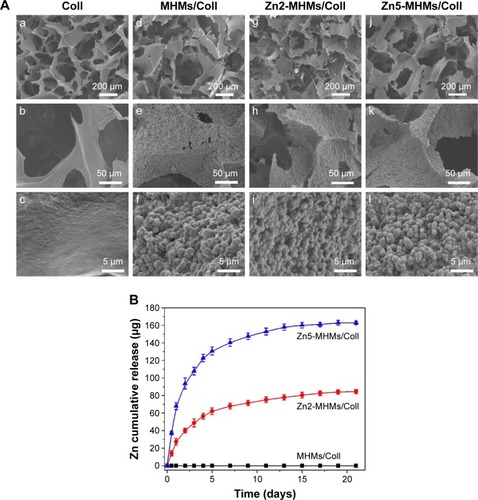
As shown in , both the Zn2-MHMs/Coll and Zn5-MHMs/Coll scaffolds exhibited sustained release of Zn ions in PBS. The initial rapid release of Zn ions from the Zn2-MHMs/Coll and Zn5-MHMs/Coll scaffolds in the first 4 days was 56.4 and 122.8 μg, respectively. As time extends, the release of Zn ions from the Zn2-MHMs/Coll and Zn5-MHMs/Coll scaffolds became slower and trended toward an equilibrium release stage, and the cumulative release amounts of Zn ions from the Zn2-MHMs/Coll and Zn5-MHMs/Coll scaffolds for 21 days were ~84.7 and 163.0 μg, respectively.
Cell adhesion and viability on the scaffolds
The cytoskeleton staining showed that the rBMSCs attached and spread well on all the four types of collagen-based scaffolds (). The SEM images further showed that the rBMSCs presented a well-spreading morphology with clear and prominent filopodia on the scaffolds ().
Figure 5 Cell morphology observation and viability assay on the Coll, MHMs/Coll, Zn2-MHMs/Coll, and Zn5-MHMs/Coll scaffolds. (A and B) CLSM and SEM images of rBMSCs on each type of scaffold after 7 days of culture. (C) Quantitative evaluation of the viability of rBMSCs on the four types of scaffolds at days 1, 3, and 7. *P<0.05 compared to Coll scaffolds.
Abbreviations: MHMs/Coll, mesoporous hydroxyapatite microspheres/collagen scaffold; CLSM, confocal laser scanning microscope; SEM, scanning electron microscopy; rBMSC, rat bone marrow–derived mesenchymal stem cell.
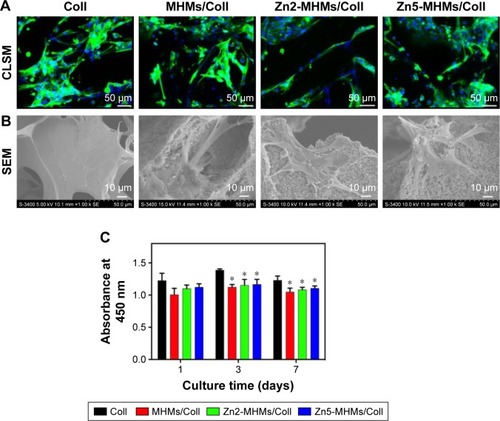
As determined by CCK-8 assay (), there was no apparent drop in cell number for all the four types of scaffolds across the culture period. However, compared to the Coll scaffolds, the MHMs/Coll, Zn2-MHMs/Coll, and Zn5-MHMs/Coll scaffolds showed lower cell viability at days 3 and 7.
Osteogenic induction in vitro
The rBMSCs were cultured directly on the scaffolds to assess the expression of osteogenesis-related genes (). Compared to those cultured on the Coll scaffolds, the rBMSCs cultured on the MHMs/Coll, Zn2-MHMs/Coll and Zn5-MHMs/Coll composite scaffolds showed increased expression levels of Runx2, Alp, and Ocn at days 7 and 14. Moreover, the expression levels of these genes increased with increasing Zn content in the Zn-MHMs/Coll scaffolds. However, the expression of Runx2 was not significantly elevated (P>0.05) for the cells cultured on the MHMs/Coll and Zn2-MHMs/Coll scaffolds at day 14, and the expression of Ocn was not significantly elevated (P>0.05) for the cells cultured on the MHMs/Coll and Zn2-MHMs/Coll scaffolds at day 7 compared with those cultured on the Coll scaffolds.
Figure 6 RT-qPCR analysis of the expression of osteogenesis-related genes (Runx2, Alp, and Ocn) in rBMSCs cultured on the Coll, MHMs/Coll, Zn2-MHMs/Coll, and Zn5-MHMs/Coll scaffolds for 7 and 14 days. *P<0.05 compared to Coll scaffolds; #P<0.05 compared to MHMs/Coll scaffolds; %P<0.05 compared to Zn2-MHMs/Coll scaffolds.
Abbreviations: RT-qPCR, real-time quantitative polymerase chain reaction; MHMs/Coll, mesoporous hydroxyapatite microspheres/collagen scaffold; rBMSC, rat bone marrow–derived mesenchymal stem cell.
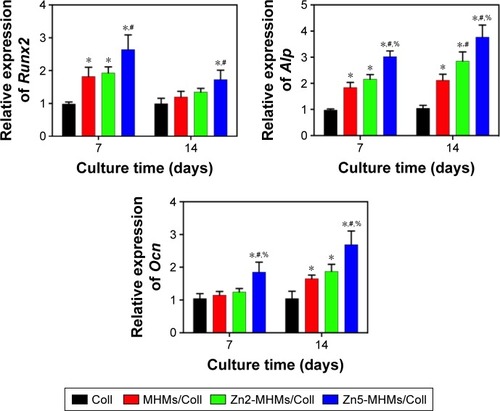
Micro-CT measurement
To evaluate new bone formation in the rat femoral condyle bone defects, micro-CT was performed after 8 weeks of implantation. As shown in , no new bone was formed in the central area of the defects implanted with the Coll scaffolds, and more new bones were formed in the Zn5-MHMs/Coll group compared with the MHMs/Coll group. Furthermore, the morphometric analysis showed that the BV/TV in Zn5-MHMs/Coll group (56.76%±4.46%) was significantly higher (P<0.05) than that in the MHMs/Coll group (40.18%±4.41%) or Coll group (22.87%±4.46%) (), and the Tb.N in Zn5-MHMs/Coll group (2.18±0.33/mm) was significantly greater (P<0.05) than that in the MHMs/Coll group (1.65±0.07/mm) or Coll group (1.10±0.12/mm) (). The Tb.Sp in Zn5-MHMs/Coll group (0.31±0.05 mm) and MHMs/Coll group (0.56±0.10 mm) was significantly lower (P<0.05) than that in the Coll group (1.63±0.37 mm) (), and no significant difference (P>0.05) was observed in Tb.Th among the three groups ().
Figure 7 Micro-CT analysis of bone regeneration for the Coll, MHMs/Coll, and Zn5-MHMs/Coll groups at 8 weeks postimplantation. (A) 3D reconstructed superficial and interior images of femoral condyle defects implanted with different scaffolds. Morphometric analysis of the BV/TV (B), Tb.N (C), Tb.Sp (D), and Tb.Th (E) for each group. *P<0.05 compared to Coll group; #P<0.05 compared to MHMs/Coll group.
Abbreviations: micro-CT, micro-computed tomography; MHMs/Coll, mesoporous hydroxyapatite microspheres/collagen scaffold; 3D, three dimensional; BV/TV, bone volume to total volume ratio; Tb.N, trabecular number; Tb.Sp, trabecular spacing; Tb.Th, trabecular thickness.
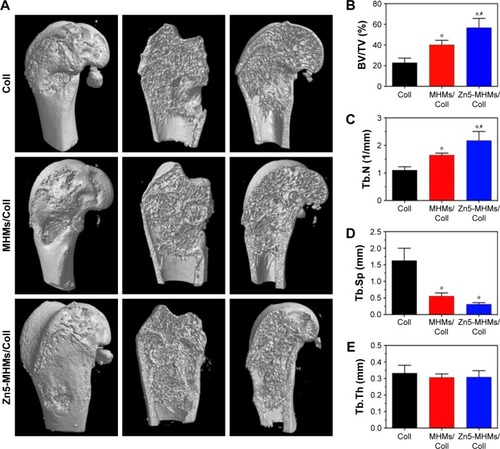
Histological assessment of bone regeneration in the femoral condyle defects
HE staining of representative sections from each group is shown in . In the Coll group, the defect area was mainly occupied by connective tissue, and the newly formed bone structure was observed only near the border of the defects. In the MHMs/Coll group, more bone tissues were observed within the defects compared with the Coll group. Encouragingly, markedly greater amounts of new bone occupied the whole defects implanted with the Zn5-MHMs/Coll scaffolds. Histomorphometric analysis showed trends for new bone formation which were in agreement with the micro-CT data (). The percentage of new bone area in the Zn5-MHMs/Coll group (42.27%±5.74%) was significantly greater (P<0.05) than that in the MHMs/Coll group (32.55%±5.66%) or Coll group (13.16%±3.92%). Scaffold remnants were still present in the defect site at 8 weeks post implantation. All scaffolds from each group were observed to integrate well with the peripheral host tissue.
Figure 8 Histological evaluation of bone regeneration in each group. (A) HE staining for newly formed bone (black arrowhead), connective tissue (blue arrowhead), and scaffold remnants (green arrowhead). (B) The percentage of the new bone area assessed by histomorphometric analysis. *P<0.05 compared to Coll group; #P<0.05 compared to MHMs/Coll group.
Abbreviations: MHMs/Coll, mesoporous hydroxyapatite microspheres/collagen scaffold; HE, hematoxylin and eosin.
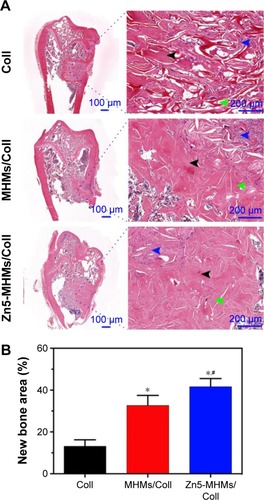
Discussion
In the present study, the Zn-MHMs were successfully synthesized by using creatine phosphate as the organic phosphorus source through a microwave-hydrothermal method. The Zn-MHMs with a mesoporous hollow structure and a high specific surface area were efficient in drug delivery and showed a pH-responsive drug release behavior by using DOX as a model drug. Furthermore, a biomimetic bone tissue engineering scaffold was constructed by incorporating the Zn-MHMs into collagen matrix with a view to mimicking the structure and composition of human trabecular bone and more importantly enhancing the bone formation ability.
The Zn-MHMs were synthesized through a microwave-hydrothermal process, which has been demonstrated to be an efficient, rapid, energy-saving method for the fabrication of CaP nanostructured porous microspheres.Citation28,Citation29 Compared to the inorganic phosphorus sources such as Na2HPO4, the organic phosphorus sources play a key role in the formation of the spherical structure of hydroxyapatite.Citation27,Citation31 In this work, creatine phosphate, a biomolecule that provides energy to cells by synthesizing adenosine 5′-triphosphate, was used as the organic phosphorus source as it has been proved to be a suitable organic phosphorus source for the synthesis of hydroxyapatite microspheres.Citation27 The EDS element mapping showed that Zn was uniformly distributed within the Zn5-MHMs, indicating that Zn was successfully introduced into the MHMs. According to our previous studies,Citation27,Citation32 the mechanism for the formation of the Zn-MHMs might be as follows. Creatine phosphate molecules hydrolyze to produce creatine molecules and PO43− ions, followed by the nuclei formation from a reaction among PO43−, Ca2+, and Zn2+ ions in an aqueous solution. Then, the Zn-doped hydroxyapatite nanosheets are formed through a crystal growth process under microwave-hydrothermal conditions. Finally, the Zn-doped hydroxyapatite nanosheets self-assemble to form hierarchically nanostructured Zn-MHMs driven by the minimization of the free energy of the nanocrystals. The Zn-MHMs consisting of hydroxyapatite nanosheets showed a mesoporous hollow structure and a relatively large specific surface area, which make them favorable for drug delivery. This study investigated the drug loading and release performance of the Zn5-MHMs as the drug nanocarrier. The Zn5-MHMs drug delivery system exhibited a pH-responsive drug release behavior. The amount of DOX released from the Zn5-MHMs at pH 4.5 was much higher than that at pH 6.0 and 7.4, and the drug release rate increased in the following order: pH 7.4 < pH 6.0 < pH 4.5. The lower drug release rate at pH 7.4 may result from the strong electrostatic attraction between the negatively charged Zn5-MHMs and the positively charged DOX, which prevents the escape of DOX molecules from the Zn5-MHMs.Citation33 The pH-responsive drug release behavior may be derived from the pH-dependent dissolution of Zn5-MHMs drug delivery system.Citation34 Moreover, the relationships between the cumulative amount of released DOX and the square root of the release time for the Zn5-MHMs drug delivery system showed good linear relationships with high regression factors of >0.95, indicating that the DOX release from the Zn5-MHMs is governed by Fickian diffusion of the Higuchi model.Citation35 The excellent performance in drug delivery makes Zn-MHMs a promising nanocarrier for the delivery of osteogenic factors such as bone morphogenetic protein 2 (BMP-2). The collagen–hydroxyapatite scaffolds releasing BMP-2 in a controlled fashion has been demonstrated to increase the healing of bone defects.Citation8
In this work, a biomimetic Zn-MHMs/Coll composite scaffold was fabricated by using a lyophilization fabrication process. Our working hypothesis was that scaffolds mimicking the structure and composition of the bone would stimulate osteogenesis in vitro and enhance new bone formation in vivo. The ratio of the Zn-MHMs to collagen was 7:3, which closely resembles the ratio between inorganic and organic components in osseous matrix of young human.Citation36 The Zn-MHMs/Coll composite scaffolds showed an interconnected pore network, and the pore size was in the range of 100–300 μm, which is favorable for the infiltration of cells and blood vessels.Citation37 The Zn-MHMs/Coll scaffolds showed a sustained release of Zn ions, and the release of Zn ions showed a marked dependence on the Zn content in the composite scaffolds, indicating that the release of Zn ions from the Zn-MHMs/Coll scaffolds can be controlled by modifying the content of Zn in the Zn-MHMs. However, high concentration of Zn ions may cause cell toxicity,Citation23 the controlled release of Zn ions from the Zn-MHMs/Coll scaffolds is therefore desirable at concentrations pertinent to stimulate osteogenic response. The concentration of Zn ions released from the Zn-MHMs/Coll scaffolds was within the range of 14.5–76.0 μM, which has been demonstrated to stimulate bone formation in tissue culture and is well below cytotoxic levels.Citation20,Citation38
The rBMSCs are attached and spread well on the composite scaffolds, which are demonstrated to be biocompatible. According to the CCK-8 assay, there was no apparent reduction in cell viability during the culture period. However, on MHMs/Coll and Zn-MHMs/Coll scaffolds, cell viability was found to be lower than that on the Coll scaffolds at days 3 and 7. It was reported that the magnesium-doped hydroxyapatite/collagen scaffold mimicking the hypoxic condition in the osteogenic niche might be responsible for maintaining the cells in a quiescent state.Citation9,Citation39 On the other hand, ion-induced cell death such as that from excessive Ca2+ may account for lower cell viability resulting from the initial ion release from the composite scaffolds.Citation40,Citation41 Collagen-based materials that serve as analogs of organic components of bone matrix have previously been developed for bone regeneration.Citation10,Citation42 It was hypothesized that the incorporation of MHMs or Zn-MHMs would improve the osteoinductive potential of the Coll scaffolds. Previous studies have demonstrated that hydroxyapatite could stimulate the osteogenesis of osteoblasts in vitro and induce in vivo bone formation.Citation43,Citation44 It was reported that the presence of hydrophilic hydroxyl groups (–OH) on a substrate, as found in hydroxyapatite, could modulate osteoblastic differentiation by enhancing the recruitment of signaling and structural biomolecules involved in cell adhesion.Citation45 Habibovic et al reported that the increased specific surface area of CaP ceramics led to more specific surface reactivity and further improved the osteogenic activity of the materials.Citation46 Reports have stated that hydroxyapatite bioceramics with nanostructured surfaces could promote protein adsorption, osteoblast proliferation, osteogenic differentiation, and in vivo bone formation.Citation47,Citation48 Moreover, naive mesenchymal stem cells are demonstrated to be extremely sensitive to the tissue elasticity, and rigid matrices that mimic collagenous bone have been proven to be osteogenic.Citation49 The incorporation of MHMs or Zn-MHMs might improve the mechanical properties of the Coll scaffolds as a result of the reinforcing effect of the microspheres. Cunniffe et al reported that the nanosized hydroxyapatite particles could improve the mechanical properties of collagen-based scaffolds resulting from their high specific surface area and strong bonding.Citation11 With all this in mind, the osteoinductive potential of the MHMs/Coll and Zn-MHMs/Coll scaffolds was explored by examining their capacities to induce osteogenesis of the rBMSCs and new bone formation in a rat femoral condyle defect model. As expected, the MHMs/Coll and Zn-MHMs/Coll scaffolds enhanced the osteogenic differentiation of rBMSCs and in vivo new bone formation compared with the Coll scaffolds. The Zn5-MHMs/Coll scaffolds showed higher osteogenic activity in vitro and achieved a superior repair of bone defects compared with the MHMs/Coll scaffolds, indicating that the introduction of Zn improves the osteoinductivity of the scaffolds. The Zn5-MHMs/Coll scaffolds may potentially provide a better microenvironment resembling the osteoblastic niche for bone augmentation compared with the Coll or MHMs/Coll scaffolds in terms of inducing osteogenesis of mesenchymal stem cells. Luo et al reported that Zn-containing tricalcium phosphate induced a great amount of new bone formation in the paraspinal muscle of canines.Citation26 The mechanism by which Zn influenced the osteogenic differentiation of the rBMSCs was beyond the scope of the present work. However, recent studies reported that Zn transporter–mediated Zn ions behaving as a signaling factor called Zn signal may play a crucial role in cellular events involving bone homeostasis.Citation50 Overall, the introduction of Zn endows the Zn-MHMs and Zn-MHMs/Coll scaffolds with enhanced osteoinductive potential beneficial for bone augmentation.
Conclusion
In this study, novel Zn-MHMs were synthesized through a microwave-hydrothermal method by producing phosphate as an organic phosphorus source. Zn-MHMs consisting of hydroxyapatite nanosheets as the building blocks showed a hierarchically mesoporous hollow structure and a high specific surface area, which are beneficial for drug delivery and tissue engineering. A novel biomimetic Zn-MHMs/Coll composite scaffold was constructed by incorporating Zn-MHMs into collagen matrix with a view to mimicking the structure and composition of human trabecular bone and improving the osteoinductive potential. Compared with the Coll and MHMs/Coll scaffolds, the Zn-MHMs/Coll scaffolds enhanced the osteogenic differentiation of the rBMSCs and encouraged more rapid and superior bone formation and healing, which bodes well for their future applications in bone defect repair and regeneration.
Acknowledgments
Financial support from the National High-Tech Research and Development Program of China (863-Project, No 2015AA020316), National Natural Science Foundation of China (81271961, 81572106, 81271998, 81601886), and the Science and Technology Commission of Shanghai, China (15ZR1431900, 15JC1491001) is gratefully acknowledged. The authors thank Dr Feng Chen for technical assistance.
Disclosure
The authors report no conflicts of interest in this work.
References
- Vallet-RegiMRuiz-HernandezEBioceramics: from bone regeneration to cancer nanomedicineAdv Mater201123445177521822009627
- HabibovicPBarraletJEBioinorganics and biomaterials: bone repairActa Biomater2011783013302621453799
- DimitriouRJonesEMcGonagleDGiannoudisPVBone regeneration: current concepts and future directionsBMC Med20119111021219637
- BucholzRWNonallograft osteoconductive bone graft substitutesClin Orthop Relat Res2002395445211937865
- ArringtonEDSmithWJChambersHGBucknellALDavinoNAComplications of iliac crest bone graft harvestingClin Orthop Relat Res1996329300309
- MarinoAFilippeschiCGenchiGGMattoliVMazzolaiBCiofaniGThe Osteoprint: a bioinspired two-photon polymerized 3-D structure for the enhancement of bone-like cell differentiationActa Biomater2014101043034313
- MarinoABarsottiJDeVGTwo-photon lithography of 3D nanocomposite piezoelectric scaffolds for cell stimulationACS Appl Mater Interfaces2015746255742557926548588
- QuinlanEThompsonEMMatsikoAO’BrienFJLopez-NoriegaALong-term controlled delivery of rhBMP-2 from collagen-hydroxyapatite scaffolds for superior bone tissue regenerationJ Controlled Release2015207112119
- MinardiSCorradettiBTaraballiFEvaluation of the osteoinductive potential of a bio-inspired scaffold mimicking the osteogenic niche for bone augmentationBiomaterials20156212813726048479
- KaneRJWeiss-BilkaHEMeagherMJHydroxyapatite reinforced collagen scaffolds with improved architecture and mechanical propertiesActa Biomater2015176162525644451
- CunniffeGMCurtinCMThompsonEMDicksonGRO’BrienFJContent-dependent osteogenic response of nanohydroxyapatite: an in vitro and in vivo assessment within collagen-based scaffoldsACS Appl Mater Interfaces2016836234772348827537605
- RaiszLGPhysiology and pathophysiology of bone remodelingClin Chem1999458 Pt 21353135810430818
- KatzEPLiSTStructure and function of bone collagen fibrilsJ Mol Biol19738011154758070
- YangMZhouGCastano-IzquierdoHZhuYMaoCBiomineralization of natural collagenous nanofibrous membranes and their potential use in bone tissue engineeringJ Biomed Nanotechnol201511344745625883539
- LandiECelottiGLogroscinoGTampieriACarbonated hydroxyapatite as bone substituteJ Eur Ceram Soc2003231529312937
- BeattieJHAvenellATrace element nutrition and bone metabolismNutr Res Rev19925116718819094319
- ZofkováINemcikovaPMatuchaPTrace elements and bone healthClin Chem Lab Med20135181555156123509220
- ReleaPRevillaMRipollEArribasIVillaLFRicoHZinc, biochemical markers of nutrition, and type I osteoporosisAge Ageing19952443033077484487
- EberleJSchmidmayerSErbenRGStangassingerMRothHPSkeletal effects of zinc deficiency in growing ratsJ Trace Elem Med Biol1999131–2212610445214
- YamaguchiMOishiHSuketaYStimulatory effect of zinc on bone formation in tissue cultureBiochem Pharmacol19873622400740123689432
- SeoHJChoYEKimTShinHIKwunISZinc may increase bone formation through stimulating cell proliferation, alkaline phosphatase activity and collagen synthesis in osteoblastic MC3T3-E1 cellsNutr Res Pract20104535636121103080
- YusaKYamamotoOFukudaMKoyotaSKoizumiYSugiyamaTIn vitro prominent bone regeneration by release zinc ion from Zn-modified implantBiochem Biophys Res Commun201141241227327821820411
- WangHZhaoSXiaoWThree-dimensional zinc incorporated borosilicate bioactive glass scaffolds for rodent critical-sized calvarial defects repair and regenerationColloid Surf B2015130149156
- JinGCaoHQiaoYMengFZhuHLiuXOsteogenic activity and antibacterial effect of zinc ion implanted titaniumColloid Surf B2014117158165
- QiaoYZhangWTianPStimulation of bone growth following zinc incorporation into biomaterialsBiomaterials201435256882689724862443
- LuoXBarbieriDDavisonNYanYde BruijnJDYuanHZinc in calcium phosphate mediates bone induction: in vitro and in vivo modelActa Biomater201410147748524140609
- QiCZhuYJLuBQHydroxyapatite hierarchically nanostructured porous hollow microspheres: rapid, sustainable microwave-hydrothermal synthesis by using creatine phosphate as an organic phosphorus source and application in drug delivery and protein adsorptionChem Eur J201319175332534123460360
- QiCZhuYJChenFFructose 1,6-bisphosphate trisodium salt as a new phosphorus source for the rapid microwave synthesis of porous calcium-phosphate microspheres and their application in drug deliveryChem Asian J201381889423192854
- QiCZhuYJZhaoXYHighly stable amorphous calcium phosphate porous nanospheres: microwave-assisted rapid synthesis using ATP as phosphorus source and stabilizer, and their application in anticancer drug deliveryChem Eur J201319398198723180605
- Olde DaminkLHDijkstraPJvan LuynMJvan WachemPBNieuwenhuisPFeijenJCross-linking of dermal sheep collagen using a water-soluble carbodiimideBiomaterials19961787657738730960
- QiCChenFWuJZhuY-JHaoC-NDuanJ-LMagnesium whitlockite hollow microspheres: a comparison of microwave-hydrothermal and conventional hydrothermal syntheses using fructose 1,6-bisphosphate, and application in protein adsorptionRSC Adv20166403339333402
- QiCZhuYJWuCTSonochemical synthesis of hydroxyapatite nanoflowers using creatine phosphate disodium salt as an organic phosphorus source and their application in protein adsorptionRSC Adv201661296869692
- ZhuYJChenFMicrowave-assisted preparation of inorganic nanostructures in liquid phaseChem Rev2014114126462655524897552
- QiCZhuYJZhangYGJiangYYWuJChenFVesicle-like nanospheres of amorphous calcium phosphate: sonochemical synthesis using the adenosine 5′-triphosphate disodium salt and their application in pH-responsive drug deliveryJ Mater Chem B201533773477354
- AnderssonJRosenholmJSami ArevaALindénMInfluences of material characteristics on ibuprofen drug loading and release profiles from ordered micro- and mesoporous silica matricesChem Mater2004162141604167
- RobinsonRABone tissue: composition and functionJohns Hopkins Med J197914511024376922
- YangSLeongKFDuZChuaCKThe design of scaffolds for use in tissue engineering. Part I. Traditional factorsTissue Eng20017667968911749726
- BrauerDSGentlemanEFarrarDFStevensMMHillRGBenefits and drawbacks of zinc in glass ionomer bone cementsBiomed Mater20116419851992
- CorradettiBTaraballiFPowellSOsteoprogenitor cells from bone marrow and cortical bone: understanding how the environment affects their fateStem Cells Dev20152491112112325517215
- QuinlanEPartapSAzevedoMMJellGStevensMMO’BrienFJHypoxia-mimicking bioactive glass/collagen glycosaminoglycan composite scaffolds to enhance angiogenesis and bone repairBiomaterials201552135836625818442
- AlcaideMPortolésPLópez-NoriegaAArcosDVallet-RegíMPortolésMTInteraction of an ordered mesoporous bioactive glass with osteoblasts, fibroblasts and lymphocytes, demonstrating its bio-compatibility as a potential bone graft materialActa Biomater20106389289919766743
- LyonsFGAl-MunajjedAAKieranSMThe healing of bony defects by cell-free collagen-based scaffolds compared to stem cell-seeded tissue engineered constructsBiomaterials201031359232924320863559
- ChengLFengYYangROsteoinduction of hydroxyapatite/β-tricalcium phosphate bioceramics in mice with a fractured fibulaActa Biomater2010641569157419896564
- LinLChowKLLengYStudy of hydroxyapatite osteoinductivity with an osteogenic differentiation of mesenchymal stem cellsJ Biomed Mater Res A200989232633518431794
- KeselowskyBGCollardDMGarcíAAJSurface chemistry modulates focal adhesion composition and signaling through changes in integrin bindingBiomaterials200425285947595415183609
- HabibovicPSeesTMDoelMAVDBlitterswijkCAVGrootKDOsteoinduction by biomaterials – physicochemical and structural influencesJ Biomed Mater Res A200677474776216557498
- LinKXiaLLiHEnhanced osteoporotic bone regeneration by strontium-substituted calcium silicate bioactive ceramicsBiomaterials20133438100281004224095251
- LinKXiaLGanJTailoring the nanostructured surfaces of hydroxyapatite bioceramics to promote protein adsorption, osteoblast growth, and osteogenic differentiationACS Appl Mater Interfaces20135168008801723862579
- EnglerAJSenSSweeneyHLDischerDEMatrix elasticity directs stem cell lineage specificationCell2006126467768916923388
- FukadaTHojyoSFuruichiTZinc signal: a new player in osteobiologyJ Bone Miner Metab201331212913523468210