Abstract
Introduction
We previously developed anionic ternary bubble lipopolyplexes, an ultrasound-responsive carrier, expecting safe and efficient gene transfection. However, bubble lipopolyplexes have a low capacity for echo gas (C3F8) encapsulation (EGE) in nonionic solution such as 5% glucose. On the other hand, we were able to prepare bubble lipopolyplexes by inserting phosphate-buffered saline before C3F8 encapsulation. Surface charge regulation (SCR) by electrolytes stabilizes liposome/plasmid DNA (pDNA) complexes by accelerated membrane fusion. Considering these facts, we hypothesized that SCR by electrolytes such as NaCl would promote C3F8 encapsulation in bubble lipopolyplexes mediated by accelerated membrane fusion. We defined this hypothesis as SCR-based EGE (SCR-EGE). Bubble lipopolyplexes prepared by the SCR-EGE method (SCR-EGE bubble lipopolyplexes) are expected to facilitate the gene transfection because of the high amount of C3F8. Therefore, we applied these methods for gene delivery to the brain and evaluated the characteristics of transgene expression in the brain.
Methods
First, we measured the encapsulation efficiency of C3F8 in SCR-EGE bubble lipopolyplexes. Next, we applied these bubble lipopolyplexes to the mouse brain; then, we evaluated the transfection efficiency. Furthermore, three-dimensional transgene distribution was observed using multicolor deep imaging.
Results
SCR-EGE bubble lipopolyplexes had a higher C3F8 content than conventional bubble lipopolyplexes. In terms of safety, SCR-EGE bubble lipopolyplexes possessed an anionic potential and showed no aggregation with erythrocytes. After applying SCR-EGE bubble lipopolyplexes to the brain, high transgene expression was observed by combining with ultrasound irradiation. As a result, transgene expression mediated by SCR-EGE bubble lipopolyplexes was observed mainly on blood vessels and partially outside of blood vessels.
Conclusion
The SCR-EGE method may promote C3F8 encapsulation in bubble lipopolyplexes, and SCR-EGE bubble lipopolyplexes may be potent carriers for efficient and safe gene transfection in the brain, especially to the blood vessels.
Introduction
Ultrasound-mediated gene transfection has been extensively studied for efficient gene therapy.Citation1–Citation3 In particular, other groups have reported that ultrasound-responsive bubble formulations and ultrasound irradiation cause blood–brain barrier (BBB) disruption by their cavitation energy and deliver plasmid DNA (pDNA) to the brain.Citation4–Citation6 Recently, Song et alCitation7 have reported that the volume of echo gas in microbubbles may affect the degree of BBB opening. Therefore, the transfection efficiency is expected to be enhanced in the brain depending on the amount of echo gas in bubble formulations. In the field of in vivo gene transfection, cationic macromolecule/pDNA complexes (polyplexes) have been developed.Citation8 However, these cationic polyplexes tend to have hematotoxicity and cytotoxicity owing to their strong interaction with blood components and cell membranes.Citation9–Citation11 Therefore, biocompatible complexes have been prepared by encapsulating cationic polyplexes with liposomesCitation12,Citation13 or coating cationic surfaces of polyplexes with anionic macromolecules via electrostatic interactions.Citation14,Citation15 However, these complexes themselves have no ability to target cells or tissues. To overcome this problem, we developed negatively charged ultrasound-responsive ternary bubble lipopolyplexes consisting pDNA, cationic polymers, and anionic liposomes (ALs).Citation16
Binary pDNA/nanobubble complexes can be prepared in nonionic solutions such as 5% glucose to prevent aggregation.Citation17 On the other hand, ternary bubble lipopolyplexes prepared in 5% glucose have a low capacity for echo gas (C3F8), suggesting that it may be difficult to prepare stable bubble formulations via electrostatic interactions because of their complicated structures. Although the detailed mechanism was unclear, we succeeded in preparing bubble lipopolyplexes with a sufficient capacity for C3F8 encapsulation using the post-inserted phosphate-buffered saline (PBS) method as follows. First, for the pre-C3F8-encapsulated form, ternary complexes were prepared in distilled water, and then a concentrated PBS solution was added before C3F8 encapsulation to be isotonic.Citation16 The existence of electrolytes such as NaCl in nonionic solutions enabled preparation of stable cationic liposome/pDNA complexes (lipoplexes) with a small size via accelerated membrane fusion among liposomes by surface charge regulation (SCR) in lipoplexes.Citation18,Citation19 Therefore, we hypothesized that the existence of electrolytes such as NaCl would affect the preparation of bubble lipopolyplexes via facilitated fusion between liposomal membranes. Consequently, the encapsulation efficiency of C3F8 of bubble lipopolyplexes can be enhanced. We defined this method based on the hypothesis as SCR-based echo gas encapsulation (SCR-EGE) for C3F8 encapsulation. To test the hypothesis, we evaluated the encapsulation efficiency of C3F8 in bubble lipopolyplexes prepared by the SCR-EGE method (SCR-EGE bubble lipopolyplexes). Moreover, we expected that the SCR-EGE bubble lipopolyplexes would facilitate gene transfection in the brain by the promotion of C3F8 encapsulation, and we applied these bubble lipopolyplexes to the brain.
For efficient gene therapy of cerebral diseases, it is important to select an appropriate therapeutic gene depending on the distribution of transgene expression. Therefore, information about transgene distribution is necessary to develop a therapeutic strategy. However, such information in the brain using bubble formulations and ultrasound irradiation is lacking. We have developed an observation system for transgene expression in tissues using a tissue-clearing method and confocal microscopy.Citation20 Tissue clearing enables deep imaging. Therefore, this method is suitable to evaluate the spatial distribution of transgene expression compared with conventional tissue sectioning. In this system, tissue-clearing reagents were selected for different purposes. For example, clear, unobstructed brain imaging cocktails (CUBIC)Citation21 is suitable for deep observation, whereas ClearT2,22 or ScaleSQCitation23 is suitable for labeling biological structures such as blood vessels and the peritoneum using lipophilic dyes. Therefore, multicolor deep imaging of labeled structures and transgene expression was achievable in kidneysCitation24 and peritoneal tissues.Citation25 Considering these aspects, we applied this multicolor deep imaging system to clarify the three-dimensional distribution of transgene expression and blood vessels in the brain.
In this study, we first prepared SCR-EGE bubble lipopolyplexes and measured their physicochemical properties to evaluate whether the SCR-EGE method promotes C3F8 encapsulation. Then, the degree of membrane fusion was evaluated using fluorescent resonance energy transfer (FRET). Next, in vivo transgene expression was examined in mice administered SCR-EGE bubble lipopolyplexes followed by ultrasound irradiation of the brain. Moreover, the three-dimensional distribution of transgene expression in the brain was clarified by multicolor deep imaging using ScaleSQ. We also evaluated whether sustained transgene expression in the brain can be achieved by a CpG-depleted vector, because this is an approach to achieve sustained expression in tissues such as the lungs and liver.Citation26,Citation27
Materials and methods
Materials
1,2-Distearoyl-sn-glycero-3-phosphocholine (DSPC) and 1,2-distearoyl-sn-glycero-3-phospho-(1′-rac-glycerol) (DSPG) were purchased from Avanti Polar Lipids, Inc. (Alabaster, AL, USA). N-(carbonyl-methoxypolyethyleneglycol 2000)-1,2-distearoyl-sn-glycero-3-phosphoethanolamine (mPEG-DSPE) was purchased from NOF Co. (Tokyo, Japan). 4′,6-Diamidino-2-phenylindole (DAPI), 1,1′-dioctadecyl-3,3,3′,3′-tetramethylindocarbocyanine perchlorate (DiI), 3,3′-dioctadecyloxacarbocyanine perchlorate (DiO), and protamine sulfate were purchased from Sigma-Aldrich Co. (St Louis, MO, USA). N,N,N′,N′-tetrakis (2-hydroxypropyl)ethylenediamine was purchased from Tokyo Chemical Industry Co., Ltd. (Tokyo, Japan). Paraformaldehyde (PFA), formamide, urea, 2,2′,2′′-nitrilotriethanol, dimethyl sulfoxide, and polyoxyethylene (10) octylphenyl ether were purchased from Wako Pure Chemical Industries, Ltd. (Osaka, Japan).
Animals
Five-week-old male ddY mice (25–30 g) were purchased from Kiwa Laboratory Animal Co., Ltd. (Wakayama, Japan), housed in cages in an air-conditioned room, and maintained on a standard laboratory diet (MF; Oriental Yeast Co., Ltd., Tokyo, Japan) and water ad libitum. All animal experiments were performed in accordance with the guidelines for animal experimentation of Nagasaki University and approved by the Institutional Animal Care and Use Committee of Nagasaki University (approval number: 1308051086-6).
pDNA
The vector coding firefly luciferase under cytomegalovirus promoter (pCMV-Luc) was constructed as reported previously.Citation28 pZsGreen1-N1 was purchased from Clontech-Takara Bio Inc. (Shiga, Japan). The CpG-depleted-Luc vector, which contains low level of unmethylated CpG dinucleotides, was constructed by subcloning the firefly luciferase cDNA fragment from pCMV-Luc into the pCpG free-MCS vector (Invivogen, Carlsbad, CA, USA). All pDNAs were amplified in Escherichia coli and purified using an EndoFree® Plasmid Giga kit (Qiagen NV, Venlo, the Netherlands).
Construction of bubble lipopolyplexes
ALs were prepared as described previouslyCitation16 and suspended in a 5% glucose solution. To construct bubble lipopolyplexes, pDNA, protamine, and ALs were mixed in a 5% glucose solution at a weight ratio of 1.0:1.5:7.0, respectively, and different volumes of saline (150 mM NaCl) were added to obtain the desired concentration. Then, C3F8 was encapsulated as described previously.Citation16 The particle size (Z-average) and zeta potential were measured by a Zetasizer Nano ZS (Malvern Instruments, Malvern, UK). To evaluate the particle size and zeta potential of bubble lipopolyplexes, pCMV-Luc was used for preparing bubble lipopolyplexes as a model pDNA. Measurements of the particle size and zeta potential were performed in disposable folded capillary cells (DTS1070; Malvern Instruments) at 25°C. For size measurement, the number and duration of measurement were automatically adjusted by instruments. For the measurement of zeta potential, the voltage applied to cells was automatically set at 150 V and the instrument performed 10–100 runs per measurement. Each measurement was performed in triplicate.
FRET analysis
To evaluate the degree of membrane fusion among ALs, we performed FRET analysis.Citation19,Citation29 When DiO is excited at 484 nm, fluorescence from DiO can excite DiI. Therefore, we used DiO as a FRET donor, and DiI as a FRET acceptor. First, we prepared ALs labeled with DiO and DiI. Then, bubble lipopolyplexes were constructed with a mixture of labeled ALs and unlabeled ALs (1:3 [weight/weight]). To evaluate FRET from DiO to DiI, fluorescence intensity spectra were measured at an excitation wavelength of 484 nm using a spectrofluorophotometer (RF-5300PC; Shimadzu Co., Kyoto, Japan). A reduction in FRET between DiO to DiI was considered as an index of membrane fusion.
Quantification of C3F8 by gas chromatography–mass spectroscopy (GC–MS)
The amount of C3F8 in bubble lipopolyplexes was measured as described previously,Citation30 with a slight modification. Briefly, 2 μL of bubble suspension was placed in a gas-tight vial. The sample was heated to disrupt the bubbles and then analyzed by a gas chromatographer (GC-2014; Shimadzu Co.) connected to a flame ionization detector.
Erythrocyte aggregation assay
Erythrocytes from mice were washed three times by suspending in PBS and centrifugation at 2,300 × g. Then, 2% (volume/volume) erythrocyte suspension was prepared. Erythrocyte suspensions were mixed with a 5% glucose solution as the negative control, protamine/pDNA complexes, or SCR-EGE bubble lipopolyplexes containing pCMV-Luc. Subsequently, the suspensions were incubated for 15 min and then observed using an AxioVert A1 microscope (Carl Zeiss Meditec AG, Jena, Germany) equipped with a ×20 objective lens.
In vivo gene transfection in the brain
Mice were anesthetized with three types of mixed anesthetic agents prepared as described previously.Citation31 Scalp fur was removed carefully and 12.5, 25, 37.5, and 50 μg of bubble lipopolyplexes (in terms of pDNA) carrying pCMV-Luc or pCpG free-Luc were injected intravenously. Immediately after injection, ultrasound radiation was applied trans-dermally to the head using a Sonopore-4000 sonicator (Nepa Gene, Chiba, Japan) under the following conditions: frequency, 1.045 MHz; duty, 10%; intensity, 0.25, 0.5, 0.75, and 1 W/cm2; duration, 0, 5, 10, 20, and 120 s using a probe with a diameter of 20 mm.
Luciferase assay
At various time points after transfection, mice were sacrificed and their brain and other organs were harvested. A luciferase assay was performed as reported previously.Citation20 Luciferase activity of 3 × 104 relative light units per gram tissues was regarded as the limit of quantitation.
Observation of transgene distribution in the brain
Twenty-four hours after transfection by bubble lipopolyplexes carrying pZsGreen1-N1, mice were anesthetized and fixed with 4% PFA via perfusion through the left ventricle. Vascular staining with DiI was performed according to a previously described procedure.Citation32 Briefly, 10 mL of DiI solution (120 μM) was perfused through the left ventricle before the fixation. Brains, livers, and lungs were excised, and brains were coronally sectioned at approximately 2 mm thicknesses. Each organ was cleared with three types of tissue-clearing reagents, CUBIC,Citation21 ScaleSQ,Citation23 or ClearT2.Citation22 The procedures for tissue clearing were performed according to the respective references. For nucleic acid staining by DAPI, tissues were immersed in CUBIC containing 5 μg/mL of DAPI for 24 h before the observation. Cleared samples were subsequently observed under a confocal laser scanning microscope (LSM 710; Carl Zeiss) equipped with ×10 or ×20 objective lenses. DAPI, DiI, and ZsGreen1 proteins were excited by lasers at 405, 549, and 493 nm, respectively. The acquisition software was ZEN2012.
Statistical analyses
Statistical comparisons were performed by Tukey’s test for multiple groups. P-values of <0.05 were considered as statistically significant.
Results
Physicochemical properties of bubble lipopolyplexes
To elucidate whether the SCR-EGE method promotes C3F8 encapsulation in bubble lipopolyplexes, we evaluated the effect of the NaCl concentration on the size and zeta potential of bubble lipopolyplexes prepared in a 5% glucose solution. The size of pre-gas-encapsulated bubble lipopolyplexes was not affected by the addition of NaCl (). However, upon addition of more than 3 mM NaCl, the size of post-gas-encapsulated bubble lipopolyplexes was increased significantly; moreover, the zeta potential became less negative and close to neutral.
Figure 1 Physicochemical property of SCR-EGE bubble lipopolyplexes.
Notes: (A) Effect of NaCl concentration on the size and zeta potential of bubble lipopolyplexes. Bubble lipopolyplexes were prepared with pDNA, protamine, and ALs in a 5% glucose solution. Then, varying amounts of NaCl were added, followed by C3F8 encapsulation. The size and zeta potential were measured at both pre- and post-gas encapsulation (n = 3). Data are represented as mean ± SD. *P < 0.05 and **P < 0.01 compared with the size of bubble lipopolyplexes without NaCl (0 mM NaCl; Tukey’s multiple comparison test). (B) Effect of NaCl concentration on C3F8 encapsulation by bubble lipopolyplexes. The amount of C3F8 in bubble suspensions was measured by GC–MS (n = 3). Data are represented as mean ± SD. *P < 0.05, Tukey’s multiple comparison test. (C) Effect of NaCl concentration on the appearance of bubble lipopolyplexes.
Abbreviations: ALs, anionic liposomes; EGE, echo gas encapsulation; GC–MS, gas chromatography–mass spectroscopy; pDNA, plasmid DNA; SCR, surface charge regulation; SCR-EGE, SCR-based EGE; SD, standard deviation.
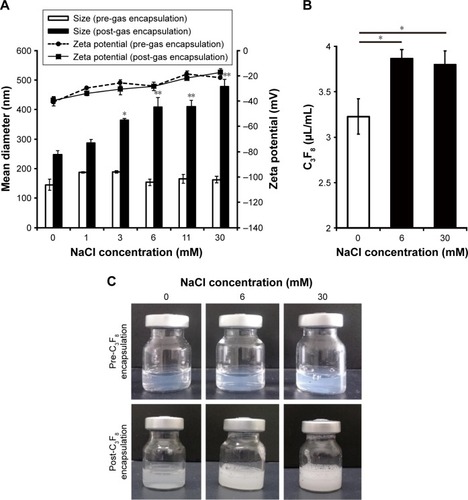
When we measured the amount of C3F8 in bubble lipopolyplexes by GC–MS, the amount of C3F8 was significantly higher in bubble lipopolyplexes with 6 or 30 mM NaCl than without NaCl (). Moreover, the appearance of bubble lipopolyplexes became cloudy by the addition of 6 or 30 mM NaCl ().
FRET analysis to evaluate membrane fusion
Bubble lipopolyplexes were prepared using ALs labeled with DiO and DiI, and then applied to FRET analysis to evaluate the degree of membrane fusion among ALs. We used the DiO/DiI fluorescent intensity ratio (F501/F565) to evaluate FRET between DiO and DiI. As shown in , no obvious change was observed by the addition of NaCl in the pre-C3F8-encapsulated state, and F501/F565 was 0.43 ± 0.04, 0.45 ± 0.04, and 0.43 ± 0.03 for lipopolyplexes with 0, 6, and 30 mM NaCl, respectively. In contrast, the reduction in FRET between DiO and DiI was observed in the post-C3F8-encapsulated state, and F501/F565 was 0.48 ± 0.06, 0.65 ± 0.12, and 0.83 ± 0.09 for bubble lipopolyplexes with 0, 6, and 30 mM NaCl, respectively ().
Figure 2 Evaluation of membrane fusion between liposomes.
Notes: FRET analysis to evaluate membrane fusion among ALs during C3F8 encapsulation. Mean emission spectra of (A) pre- and (B) post-C3F8 encapsulation are shown. The excitation wavelength in both conditions was 484 nm.
Abbreviations: ALs, anionic liposomes; FRET, fluorescent resonance energy transfer.
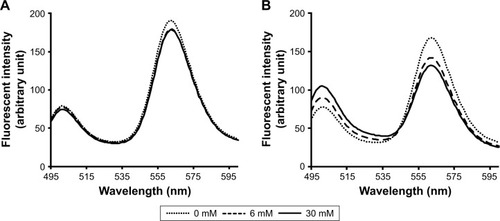
Erythrocyte aggregation assay
To evaluate hemagglutination, we added binary protamine/pDNA complexes or SCR-EGE bubble lipopolyplexes to erythrocyte suspensions. Aggregation was observed for binary protamine/pDNA complexes, whereas aggregations were not observed for SCR-EGE bubble lipopolyplexes ().
Transfection efficiency of bubble lipopolyplexes
Next, we evaluated the transfection efficiency of SCR-EGE bubble lipopolyplexes. Bubble lipopolyplexes carrying pCMV-Luc were administered to mice, and then the mouse brain was irradiated by ultrasound. As shown in , mice transfected by bubble lipopolyplexes with 6 or 30 mM NaCl exhibited approximately 10-fold higher expression of luciferase compared with bubble lipopolyplexes without NaCl.
Figure 3 Transfection efficiency of bubble lipopolyplexes.
Notes: Transfection efficiency of bubble lipopolyplexes in the brain (A) and other organs (B). Mice were intravenously administered with bubble lipopolyplexes with or without NaCl (pDNA, 50 μg), followed by ultrasound irradiation (duration, 120 s; intensity, 1 W/cm2) of the brain. Six hours after transfection, luciferase activities were analyzed in the brain and other organs (n = 3 or 4). Data are represented as mean ± SD. **P < 0.01, Tukey’s multiple comparison test.
Abbreviations: pDNA, plasmid DNA; RLU, relative light units; US, ultrasound.
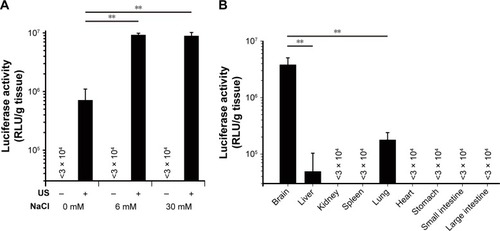
In addition, luciferase activity of the brain, liver, kidneys, spleen, lungs, heart, stomach, and small and large intestines was measured after transfection by bubble lipopolyplexes with 6 mM NaCl and ultrasound irradiation. As a result, luciferase activity in the brain was significantly higher than that in any other organs ().
Optimization of transfection conditions
To optimize transfection conditions, we evaluated the influence of the ultrasound duration, ultrasound intensity, and dose of SCR-EGE bubble lipopolyplexes containing 6 mM NaCl on transfection efficiency in the brain. Luciferase gene expression reached a plateau by ultrasound irradiation at 0.5 W/cm2 for 5 s (). In addition, luciferase gene expression tended to increase as the dose of pDNA was increased (). In subsequent experiments, mice were administered with 50 μg pDNA followed by ultrasound irradiation at 1 W/cm2 for 10 s.
Figure 4 Optimization of transfection condition.
Notes: Luciferase activities under different transfection conditions, ultrasound duration (A), ultrasound intensity (B), and dose of administered pDNA (C) were analyzed 6 h after transfection. Mice were intravenously administered with SCR-EGE bubble lipopolyplexes containing 6 mM NaCl, followed by ultrasound irradiation of the brain. (A) pDNA (50 μg) was administered, and ultrasound irradiation was applied at 1 W/cm2 at different durations. (B) pDNA (50 μg) was administered, and ultrasound irradiation was applied at various intensities for 10 s. (C) Various doses of pDNA were administered, and ultrasound irradiation was applied at 1 W/cm2 for 10 s (n = 3). Data are represented as mean ± SD.
Abbreviations: EGE, echo gas encapsulation; pDNA, plasmid DNA; SCR, surface charge regulation; SCR-EGE, SCR-based EGE; SD, standard deviation.
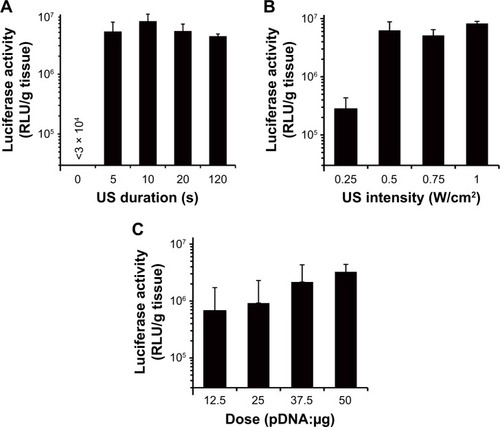
Distribution of transgene expression
The distribution of transgene expression in the brain was analyzed by confocal laser scanning microscopy after tissue clearing. To evaluate the dispersibility of transgene expression, the brain was cleared with CUBIC. Consequently, ZsGreen1 expression was detected in the entire observed area, and the degree of ZsGreen1 expression was apparently higher in mice transfected by bubble lipopolyplexes with 6 mM NaCl (SCR-EGE bubble lipopolyplexes) than those without NaCl (conventional bubble lipopolyplexes; ). Moreover, the distribution of ZsGreen1 in the lungs and liver was evaluated when gene was transfected to the brain by SCR-EGE bubble lipopolyplexes with ultrasound. Consequently, few transgene expressions were detected in the lungs () or liver () when they were cleared with CUBIC (). Next, cerebrovascular staining was performed with DiI, followed by tissue clearing using ScaleSQ or ClearT2. When cleared with ScaleSQ (), ZsGreen1 transfected by both conventional and SCR-EGE bubble lipopolyplexes was observed mainly on blood vessels and partially outside of blood vessels (). Furthermore, the same trend of ZsGreen1 distribution was observed when the brain was cleared with ClearT2 ().
Figure 5 Distribution of transgene expression in the brain.
Notes: Observation of the ZsGreen1 distribution in the brain using tissue clearing. Mice were transfected with conventional or SCR-EGE bubble lipopolyplexes carrying pZsGreen1-N1 under the following conditions: ultrasound duration, 10 s; intensity, 1 W/cm2; pDNA dose; 50 μg. Twenty-four hours after transfection, mice were fixed and cleared using CUBIC (A–G), ScaleSQ (H–J), or ClearT2 (K–M). Transmission color images of the brain after tissue clearing using CUBIC (A), ScaleSQ (H), or ClearT2 (K) are shown. Confocal microscopy images of brains transfected with conventional (B, I, L) or SCR-EGE bubble lipopolyplexes (C, J, M) are shown. The transmission color images and confocal microscopy image of the lungs and liver cleared with CUBIC are shown as control organs (D–G). Green, red, and blue signals indicate ZsGreen1 expression, DiI-labeled blood vessels, and DAPI staining, respectively. Arrows denote ZsGreen1 expression outside of blood vessels.
Abbreviations: CUBIC, clear, unobstructed brain imaging cocktails; DAPI, 4′,6-diamidino-2-phenylindole; DiI, 1,1′-dioctadecyl-3,3,3′,3′-tetramethylindocarbocyanine perchlorate; EGE, echo gas encapsulation; pDNA, plasmid DNA; SCR, surface charge regulation; SCR-EGE, SCR-based EGE.
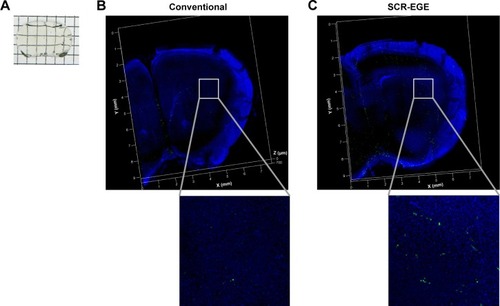
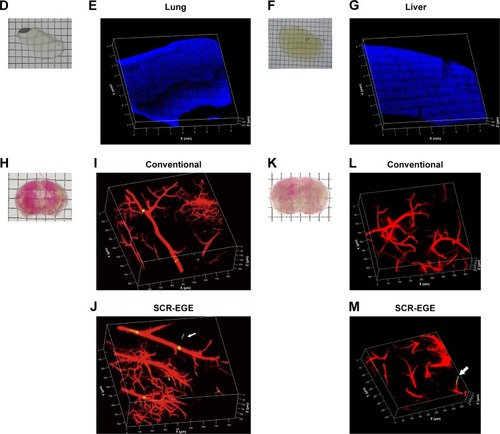
Duration of transgene expression
Bubble lipopolyplexes carrying pCMV-Luc or pCpG- depleted-Luc with or without NaCl were administered to mice, and then the brain was irradiated by ultrasound. Luciferase activity in the brain was determined at 1, 7, 14, and 28 days after transfection. Consequently, sustained expression was observed in brains transfected by bubble lipopolyplexes carrying CpG-depleted-Luc for at least 28 days after transfection (). Conversely, for bubble lipopolyplexes carrying pCMV-Luc, the transgene expression was markedly decreased within 7 days. Moreover, at all time points, the expression levels of luciferase transfected by bubble lipopolyplexes with 6 mM NaCl was higher compared with lipopolyplexes without NaCl.
Figure 6 Sustained transgene expression mediated by CpG-depleted vector.
Notes: Sustained transgene expression in the brain using conventional or SCR-EGE bubble lipopolyplexes carrying pCMV-Luc or CpG-depleted vectors. Each vector was transfected into the brain under the following conditions: ultrasound duration, 10 s; intensity, 1 W/cm2; pDNA dose; 50 μg. At 1, 7, 14, and 28 days after administration, mice were sacrificed and luciferase activity was measured in their brains (n = 4). Data are represented as mean ± SD. *P < 0.05 and **P < 0.01, Tukey’s multiple comparison test.
Abbreviations: EGE, echo gas encapsulation; pCMV-Luc, vector coding firefly luciferase under cytomegalovirus promoter; pDNA, plasmid DNA; RLU, relative light units; SCR, surface charge regulation; SCR-EGE, SCR-based EGE.
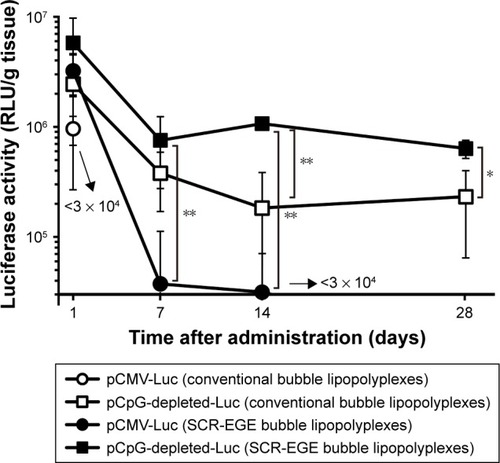
Discussion
The results in this study support the SCR-EGE hypothesis that SCR promotes membrane fusion of lipopolyplexes. Consequently, C3F8 encapsulation of bubble lipopolyplexes can be accelerated. The size and C3F8 content of bubble lipopolyplexes were increased, and the zeta potential became less negative and close to neutral (). Furthermore, their appearance became cloudy after the addition of NaCl (). Our results are supported by a study by Borden et al,Citation33 in which an increase in the size of bubbles was observed after the addition of NaCl to a nonionic solution. Moreover, FRET between each fluorescent dye in liposomes was reduced by the addition of NaCl (). These results suggest that SCR promotes C3F8 encapsulation via accelerated membrane fusion of bubble lipopolyplexes. Recently, Song et alCitation7 reported an increase in the extravasation of Evans blue from blood to brain tissue depending on the amount of injected gas. Therefore, promoting C3F8 encapsulation into bubble lipopolyplexes is also expected to enhance pDNA delivery to the brain, and the promotion of C3F8 encapsulation may result in a high transfection efficiency. The gene transfection efficiency of bubble lipopolyplexes with 6 or 30 mM NaCl was significantly higher than that of bubble lipopolyplexes without NaCl (). Although C3F8 capacity of SCR-EGE bubble lipopolyplexes was increased only 20% from the conventional bubble lipopolyplexes (), the transgene expression of SCR-EGE bubble lipopolyplexes was approximately 10 times higher than that of conventional bubble lipopolyplexes (). Therefore, the other factors may affect the transfection efficiency of bubble lipopolyplexes as well as the increase in C3F8 encapsulation. One possible factor is in vivo stability of the formulation. We previously reported that the SCR lipoplexes retained the primary structure longer than conventional bubble lipopolyplexes in the biological condition.Citation18,Citation19 Therefore, we speculated that SCR-EGE bubble lipopolyplexes could be more stable in the biological condition. When they are distributed to the brain, we think that SCR-EGE bubble lipopolyplexes deliver much higher C3F8 than conventional bubble lipopolyplexes; therefore, high cavitation energy can be generated in the brain in response to ultrasound irradiation. In general, a large formulation can greatly interact with cerebral capillaries. We consider that increasing the size of bubble lipopolyplexes also affects the transfection efficiency in the brain. In fact, the particle size of SCR-EGE bubble lipopolyplexes was increased about 70% from conventional bubble lipopolyplexes (), although the C3F8 capacity of SCR-EGE bubble lipopolyplexes was increased only 20% from conventional bubble lipopolyplexes. Taking these into consideration, we consider that, in addition to the C3F8 capacity, in vivo stability and size are important factors that affect the transfection efficiency to the brain. In addition, transgene expression was detected specifically in the brain (). Furthermore, bubble lipopolyplexes with 6 mM NaCl showed no aggregation with erythrocytes because of their negative surface charge (). This result is compatible with a previous study in which bubble lipopolyplexes were prepared in PBS.Citation16 Our data suggest that the SCR-EGE method promotes C3F8 encapsulation in bubble lipopolyplexes, and SCR-EGE bubble lipopolyplexes may be a potent carrier for efficient and safe gene transfection to the brain.
Thus far, there have been no reports about transfection to the brain using SCR-EGE bubble lipopolyplexes and ultrasound irradiation. Therefore, we optimized the transfection conditions, focusing on the ultrasound duration, ultrasound intensity, and dose of SCR-EGE bubble lipopolyplexes. We found sufficiently high transgene expression under the following conditions: ultrasound duration, 10 s; intensity, 1 W/cm2; pDNA dose; 50 μg (). Therefore, we used these conditions in subsequent experiments to evaluate the transgene distribution and sustained expression.
To develop a therapeutic strategy with bubble lipopolyplexes, we evaluated the distribution of transgene expression achieved by the combination of bubble lipopolyplexes and ultrasound irradiation using tissue clearing. First, we evaluated the spatial dispersibility of transgene expression in the brain using tissue-clearing reagent CUBIC, because the reagent provides high transparency (). Consequently, transgene expression was observed at a depth of 700 μm from the surface and in the entire observed area (). The degree of transgene expression was apparently higher in mice transfected with SCR-EGE bubble lipopolyplexes compared with conventional bubble lipopolyplexes. These data support the quantitative result of luciferase expression in . On the other hand, the number of transgene positive cells appeared to be small (), as compared to the result of luciferase expression (). In the current study, ultrasound was irradiated to whole brain using planner ultrasound; therefore, the density of transgene expression may be low by dispersing the energy of ultrasound. In , in the control organs such as the lungs and liver, few transgene expressions were detected. The results are corresponding to . Taking these into considerations, SCR-EGE bubble lipopolyplexes with ultrasound irradiation to brain may transfect gene efficiently and selectively to brain. Next, to evaluate the spatial positional relationship between cerebral blood vessels and transgene expression, we cleared the brain using ClearT2 () and ScaleSQ (), followed by vascular staining with DiI, because these reagents do not contain a detergent and retain DiI. So far, for gene transfection to the brain using microbubbles and ultrasound irradiation, it has been reported that transgene expression was observed in extravascular cells such as astrocytes and neurons by evaluation through tissue sectioning and immunohistochemical analysis.Citation5,Citation6 Therefore, we expected that bubble lipopolyplexes mediated the extravascular transgene expression. In fact, transgene expression was observed mainly on DiI-labeled blood vessels and partially outside of blood vessels in mice transfected with both conventional and SCR-EGE bubble lipopolyplexes when the brain was cleared with ScaleSQ (). Furthermore, the same trend of transgene expression was observed when the brain was cleared with ClearT2 (). These results are incompatible with other reports using microbubbles. We consider that this difference may be derived from the bubble size. Tung et alCitation34 reported that larger microbubbles have a higher ability for BBB opening, because they tend to contact vascular walls. The size of bubble lipopolyplexes was 400 nm even when C3F8 encapsulation was improved. Therefore, in bubble lipopolyplexes, sonoporation of endothelial cells might mainly occur. Overall, our spatial evaluation using tissue clearing succeeded in clarifying that the SCR-EGE method enhances the transfection efficiency of bubble lipopolyplexes with a tendency to transfect blood vessels.
Cerebral blood vessels are expected to be a potent target of therapeutic gene transfection for the treatment of some cerebral diseases. Transfection of glial cell-derived neurotrophic factor (GDNF) into brain capillary endothelial cells and secretion of GDNF into brain parenchyma are reported to exert protective effects against Parkinson’s disease.Citation35,Citation36 For efficient gene therapy of cerebral diseases, sustained therapeutic transgene expression is desired because these diseases tend to be chronic. A CpG-depleted plasmid vector is known to extend the duration of transgene expression by minimizing the inflammatory response mediated by Toll-like receptor 9.Citation26,Citation27 Therefore, we evaluated the duration of transgene expression in the brain transfected with bubble lipopolyplexes containing a CpG-depleted vector. As shown in , sustained transgene expression mediated by pCpG-depleted-Luc was observed for at least 28 days. In contrast, transgene expression mediated by pCMV-Luc was drastically decreased within 7 days. However, the trans-gene expression level in mice transfected with SCR-EGE bubble lipopolyplexes was higher than that in mice transfected with conventional bubble lipopolyplexes at all time points. These results suggest that sustained high transgene expression is achieved by SCR-EGE bubble lipopolyplexes carrying a CpG-depleted vector. Based on the results of the transgene distribution and sustained expression, we believe that a potent therapeutic strategy can be expected by vascular transfection using SCR-EGE bubble lipopolyplexes carrying CpG-depleted pDNA and subsequent sustained secretion of therapeutic proteins from blood vessels.
Conclusion
In this study, we clarified that the SCR-EGE method can promote C3F8 encapsulated in bubble lipopolyplexes, and that SCR-EGE bubble lipopolyplexes may be potent carriers for efficient and safe transfection to the brain in combination with ultrasound irradiation of the brain. Moreover, spatial evaluation using tissue clearing succeeded in clarifying the three-dimensional distribution of transgene expression in the brain with high transgene expression found on cerebral blood vessels. These findings are valuable to develop therapeutic strategies using bubble lipopolyplexes with ultrasound irradiation.
Acknowledgments
We thank Professor Kazuo Maruyama (Teikyo University) for measuring C3F8 by GC–MS. We also thank Mitchell Arico from Edanz Group (www.edanzediting.com/ac) for editing a draft of this manuscript. This work was supported by JSPS KAKENHI (grant number 16K18862), the Shin-Nihon Foundation of Advanced Medical Research, and Takeda Science Foundation.
Supplementary material
Figure S1 Evaluation of erythrocyte aggregation.
Note: A 5% glucose solution (A), protamine/pDNA complexes (B), and SCR-EGE bubble lipopolyplexes (C) were added to murine erythrocytes. Each erythrocyte suspension was observed at a magnification of 200×.
Abbreviations: EGE, echo gas encapsulation; pDNA, plasmid DNA; SCR, surface charge regulation; SCR-EGE, SCR-based EGE.
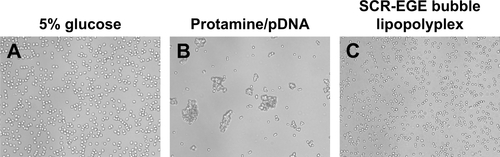
Disclosure
The authors report no conflicts of interest in this work.
References
- KawakamiSHashidaMGlycosylation-mediated targeting of carriersJ Control Release201419054255524915504
- FumotoSKawakamiSCombination of nanoparticles with physical stimuli toward cancer therapyBiol Pharm Bull201437221221624492718
- NewmanCMBettingerTGene therapy progress and prospects: ultrasound for gene transferGene Ther200714646547517339881
- NegishiYYamaneMKuriharaNEnhancement of blood-brain barrier permeability and delivery of antisense oligonucleotides or plasmid DNA to the brain by the combination of bubble liposomes and high-intensity focused ultrasoundPharmaceutics20157334436226402694
- MeadBPMastorakosPSukJSKlibanovALHanesJPriceRJTargeted gene transfer to the brain via the delivery of brain-penetrating DNA nanoparticles with focused ultrasoundJ Control Release20162231010911726732553
- HuangQDengJXieZEffective gene transfer into central nervous system following ultrasound-microbubbles-induced opening of the blood-brain barrierUltrasound Med Biol20123871234124322677255
- SongK-HFanACHinkleJJNewmanJBordenMAHarveyBKMicrobubble gas volume: a unifying dose parameter in blood-brain barrier opening by focused ultrasoundTheranostics20177114415228042323
- KircheisRWightmanLWagnerEDesign and gene delivery activity of modified polyethyleniminesAdv Drug Deliv Rev200153334135811744176
- DekieLTonchevaVDubruelPSchachtEHBarrettLSeymourLWPoly-L-glutamic acid derivatives as vectors for gene therapyJ Control Release200065118720210699280
- BreunigMLungwitzULieblRGoepferichABreaking up the correlation between efficacy and toxicity for nonviral gene deliveryProc Natl Acad Sci U S A200710436144541445917726101
- GebhartCLKabanovAVEvaluation of polyplexes as gene transfer agentsJ Control Release2001732–340141611516515
- SchäferJHobelSBakowskyUAignerALiposome-polyethylenimine complexes for enhanced DNA and siRNA deliveryBiomaterials201031266892690020561681
- PinnapireddySRDuseLStrehlowBSchäferJBakowskyUComposite liposome-PEI/nucleic acid lipopolyplexes for safe and efficient gene delivery and gene knockdownColloids Surf B Biointerfaces20171589310128683347
- KurosakiTKitaharaTFumotoSTernary complexes of pDNA, polyethylenimine, and γ-polyglutamic acid for gene delivery systemsBiomaterials200930142846285319232715
- KoyamaYYamadaEItoTMizutaniYYamaokaTSugar-containing polyanions as a self-assembled coating of plasmid/polycation complexes for receptor-mediated gene deliveryMacromol Biosci200226251256
- KurosakiTKawakamiSHiguchiYDevelopment of anionic bubble lipopolyplexes for efficient and safe gene transfection with ultrasound exposure in miceJ Control Release201417628243424384299
- UnKKawakamiSSuzukiRMaruyamaKYamashitaFHashidaMDevelopment of an ultrasound-responsive and mannose-modified gene carrier for DNA vaccine therapyBiomaterials201031307813782620656348
- KawakamiSItoYFumotoSYamashitaFHashidaMEnhanced gene expression in lung by a stabilized lipoplex using sodium chloride for complex formationJ Gene Med20057121526153316170832
- FumotoSKawakamiSItoYShigetaKYamashitaFHashidaMEnhanced hepatocyte-selective in vivo gene expression by stabilized galactosylated liposome/plasmid DNA complex using sodium chloride for complex formationMol Ther200410471972915451456
- FumotoSNishimuraKNishidaKKawakamiSThree-dimensional imaging of the intracellular fate of plasmid DNA and transgene expression: ZsGreen1 and tissue clearing method CUBIC are an optimal combination for multicolor deep imaging in murine tissuesPLoS One2016111e014823326824850
- SusakiEATainakaKPerrinDWhole-brain imaging with single-cell resolution using chemical cocktails and computational analysisCell2014157372673924746791
- KuwajimaTSitkoAABhansaliPJurgensCGuidoWMasonCClearT: a detergent- and solvent-free clearing method for neuronal and non-neuronal tissueDevelopment201314061364136823444362
- HamaHHiokiHNamikiKScaleS: an optical clearing palette for biological imagingNat Neurosci201518101518152926368944
- OyamaNFuchigamiYFumotoSCharacterization of transgene expression and pDNA distribution of the suctioned kidney in miceDrug Deliv201724190691728585867
- NishimuraKFumotoSFuchigamiYHagimoriMMaruyamaKKawakamiSEffective intraperitoneal gene delivery system using nanobubbles and ultrasound irradiationDrug Deliv201724173774428446052
- HodgesBLTaylorKMJosephMFBourgeoisSAScheuleRKLong-term transgene expression from plasmid DNA gene therapy vectors is negatively affected by CpG dinucleotidesMol Ther200410226927815294174
- YewNSZhaoHPrzybylskaMCpG-depleted plasmid DNA vectors with enhanced safety and long-term gene expression in vivoMol Ther20025673173812027557
- NomuraTYasudaKYamadaTGene expression and antitumor effects following direct interferon (IFN)-γ gene transfer with naked plasmid DNA and DC-chol liposome complexes in miceGene Ther19996112112910341884
- YefimovaSLKurilchenkoIYTkachevaTNMicrospectroscopic study of liposome-to-cell interaction revealed by förster resonance energy transferJ Fluoresc201424240340924101211
- OdaYSuzukiRMoriTDevelopment of fluorous lipid-based nanobubbles for efficiently containing perfluoropropaneInt J Pharm20154871–2647125841568
- KawaiSTakagiYKanekoSKurosawaTEffect of three types of mixed anesthetic agents alternate to ketamine in miceExp Anim201160548148722041285
- LiYSongYZhaoLGaidoshGLatiesAMWenRDirect labeling and visualization of blood vessels with lipophilic carbocyanine dye DiINat Protoc20083111703170818846097
- BordenMACaskeyCFLittleEGilliesRJFerraraKWDNA and polylysine adsorption and multilayer construction onto cationic lipid-coated microbubblesLangmuir200723189401940817665937
- TungYSVlachosFFeshitanJABordenMAKonofagouEEThe mechanism of interaction between focused ultrasound and microbubbles in blood-brain barrier opening in miceJ Acoust Soc Am201113053059306722087933
- HuangRMaHGuoYAngiopep-conjugated nanoparticles for targeted long-term gene therapy of Parkinson’s diseasePharm Res201330102549255923703371
- JiangCKoyabuNYonemitsuYIn vivo delivery of glial cell-derived neurotrophic factor across the blood-brain barrier by gene transfer into brain capillary endothelial cellsHum Gene Ther2004141211811191