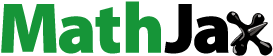
Abstract
Background
Noble metal nanoparticles, due to their good physicochemical properties, have been exploited in biological applications. Among these metals, nanosilver has attracted great attention because of its optical properties and broad-spectrum antimicrobial activities with no drug tolerance.
Purpose
The present study has attempted to conduct chemical synthesis of Fe3O4@PEG-Ag core/shell nanocomposites in aqueous solutions through co-precipitation of Fe3+ and Fe2+ ions, encapsulating the iron oxide core by poly (ethylene-glycol) (PEG) improve its hydrophilicity and biocompatibility, and immobilizing silver ions by application of NaBH4 as a reducing agent.
Patients and methods
The synthesized structures were characterized by Fourier-transform infrared (FT-IR), field emission scanning electron microscopy, energy-dispersive X-ray spectrum, wavelength-dispersive X-ray, vibrating sample magnetometer, inductively coupled plasma-mass spectrometry and transmission electron microscopy methods. Antimicrobial activity of the nanostructures against Staphylococcus aureus, Escherichia coli and Candida albicans was evaluated by broth microdilution based on the methods suggested by Clinical Laboratory Standard Institute. Furthermore, the nanocomposite was tested for possible anti-parasitic effects against Leishmania major promastigotes by MTT assay. Also, its impacts on bacterial cell morphology were defined using atomic force microscopy. Moreover, toxicity of the nanostructure related to animal cell line was determined based on MTT assay.
Results
In general, the synthesized core/shell nanostructure can demonstrate noticeable activity against the evaluated representative microorganisms while its toxicity against animal cell line is not considerable.
Conclusion
This nanostructure can be applied as a smart drug delivery system with the help of an external magnetic field or it can be used as a powerful antibiotic agent along with other antibiotics that can form a shell on its structure.
Introduction
In the past 2 decades, functional nanomaterials have attracted great attention for their unique properties based on their size, shape and compositions.Citation1,Citation2 Emergence of resistance against routine antibiotics has increased dramatically during this period. Some examples include nosocomial infections caused by methicillin-resistant Staphylococcus aureus (MRSA), azole-resistant Candida species and third-generation cephalosporin-resistant (TGCsR) Escherichia coli, which have ended up with high mortality and morbidity in patients.Citation3,Citation4 Another crisis is associated with resistance to antiparasitic drugs, which is more critical in developing countries. Thereby, increased occurrence of resistance to Glucantime (meglumine antimoniate) has been reported by several researches.Citation5,Citation6
Consequently, some new antimicrobial agents with low cost, broad applicability, eco-friendliness, long lifetime and reusability features should be developed. An option to overcome antibiotic resistance is application of novel synthetic nanostructures with antimicrobial capabilities.Citation7–Citation13 The nanostructure can be a metallic nanoparticle (NP). Since noble metal NPs possess good physicochemical properties, they have been used in biological applications. Among noble metals, silver has gained more interest due to its specific optical properties and diverted antimicrobial activities; however, it poses no drug tolerance.Citation14–Citation24 Antimicrobial function of silver is effective in a way that it is safe for animal cells but very toxic for microorganisms (from fungi to viruses), eg, HIV, E. coli and Staphylococcus aureus. Nanosilver particles have high surface-to-volume ratio, small size and high dispersion potency, which facilitate their interactions with microbial surfaces. Thereby, they can be easily adhered to cell walls of different microorganisms to destroy and kill their cells by altering the permeability of cell membrane and disrupting the function of respiration system of pathogen cells. In addition, the genomic substance of cell containing nitrogen, oxygen and sulfur combines with Ag ions that leads to interception of DNA replication.Citation7,Citation25–Citation27
On the other hand, much attention has been devoted to superparamagnetic magnetite (Fe3O4) nano-ferro particles as a result of their high magnetic saturation, low toxicity and facile surface modification using polymers, such as dextran,Citation28 poly(ethylene glycol) (PEG)Citation29 and polyvinylpyrrolidone (PVP).Citation25,Citation26,Citation30 A process requires for the solubility and bioavailability of metals used in NPs.Citation23 Specifically, PEG can demonstrate noticeable biocompatibility, biodegradability, blood circulation times and hydrophilicity.
Among the pathogen agents, E. coli, a Gram-negative bacteria; S. aureus, a Gram-positive bacteria; and also Candida albicans, a dimorphic yeast, are well-known factors reported as agents of nosocomial infections.Citation31 For this reason, the antimicrobial activity of synthesized NPs in various studies against these pathogen organisms has been considered. NPs of cadmium selenide,Citation32 zinc oxide-doped TiO2,Citation33 binary metal oxide nanocomposites using cerium and cadmiumCitation34 are studies that have exhibited antimicrobial effects of mentioned NPs. Furthermore, NPs have been made using plant extracts, which also have inhibitory effects on the growth of pathogens.Citation35,Citation36
Therefore, with respect to the earlier discussions, this study attempts to synthesize and characterize inorganic–organic hybrids (Fe3O4@PEG–Ag) as antimicrobial agents. These agents are expected as promising therapeutics due to their significant chemical stability, negligible toxicity, great hydrophilicity, heat resistance and long life.
Experimental
Materials
Chemicals were purchased from EMD Millipore (Billerica, MA, USA). The reagents and solvents were obtained from Sigma-Aldrich Co. (St Louis, MO, USA) and EMD Millipore and used without further purification.
Preparation of magnetic Fe3O4 nanoparticles (MNPs)
Fe2+ and Fe3+ ions with a molar ratio of 2:1 were chemically coprecipitated to prepare naked Fe3O4 NPs. Typically, FeCl2⋅4H2O (2.147 g, 0.0108 mol) and FeCl3⋅6H2O (5.838 g, 0.0216 mol) were dissolved in 100 mL of deionized water and vigorously stirred (500 rpm) at 85°C under nitrogen atmosphere. Then, 10 mL (one portion) of 25% NH4OH was quickly added to the reaction mixture (Fe2+/Fe3+ salt solution), while a black precipitate of MNPs was immediately formed. After another 25 min, the mixture was cooled to room temperature. Consequently, the ultrafine magnetic particles treated by magnetic separation were washed with deionized water several times.
Preparation of Fe3O4@PEG400
In all, 1 g of Fe3O4 NPs was dispersed in 200 mL of water and sonicated for 20 min. Then, 3 g of PEG400 dissolved in 10 mL of water was added to the solution and sonicated for 10 min again. Using an external magnet, the suspended substance yielded after mechanical agitation at 25°C for 24 h was separated to be washed with water and ethanol. After synthesizing PEG400-functionalized Fe3O4 (Fe3O4@ PEG400), the NPs were dried at 50°C for 12 h.
Preparation of Fe3O4@PEG400/Ag
Fe3O4@PEG400 (200 mg) was dispersed in water (50 mL) and sonicated for 20 min. Subsequently, 20 mL of 10−2 M AgNO3 solution was added to the reaction mixture and stirred for 2 h. Then, freshly prepared aqueous NaBH4 solution (5 × 10−2 M, 25 mL) was added at one portion and stirred for 30 min at room temperature. Finally, the as-prepared nanocomposite was separated by an application of external magnetic field and washed several times by deionized water. The Ag loading of the prepared nanocomposite was measured to be 0.12 mmol/g by ICP.
Determination of biological activities of the microorganisms
The antimicrobial activity of Fe3O4@PEG–Ag was evaluated against four standard microorganism strains, including C. albicans (ATCC 10261), S. aureus (ATCC 2592), E. coli (ATCC 25922) and isolated Leishmania major promastigotes (MRHO/IR/75/ER).
Antibacterial and antifungal activities
Minimum inhibitory concentration (MIC) values were explored through the broth microdilution method proposed by the Clinical Laboratory Standard Institute (CLSI) with some modifications.Citation37 Briefly, the antifungal activity was determined by serial dilution of the NP (0.5–512 µg/mL) in 96-well microtiter plates using the Roswell Park Memorial Institute (RPMI)-1640 medium (Sigma-Aldrich Co.), which was buffered with 3-(N-morpholino)propanesulfonic acid (Sigma-Aldrich Co.). To investigate the antibacterial activity of the NP, it was diluted serially (0.5–256 µL/mL) in a Müller-Hinton medium (EMD Millipore). To target yeasts and bacteria, three colonies of each microorganism were suspended in 5 mL of sterile 0.85% NaCl to give the stock suspensions, and inoculums’ turbidity was adjusted on 0.5 McFarland standard at a wavelength of 630 nm. This preparation method provided stock suspensions of 1–5 × 106 cells/mL and 1–1.5 × 108 cells/mL for yeasts and bacteria, respectively. The working suspension was made by diluting the stock suspension with RPMI (1/1,000) or Müller-Hinton broth (1/100) solutions for yeasts and bacteria, respectively. In all, 0.1 mL of the working inoculum was injected to each well of the microtiter plates, and the plates were incubated at 32°C for 24–48 h for fungi or at 37°C for 24 h for bacteria in a humid atmosphere. A total of 200 µL of uninoculated medium was considered as a sterility blank control. Furthermore, growth controls, ie, media containing inoculums and no NP, were also evaluated. Microorganism growth in each well was examined versus the corresponding well of growth control. MICs were indicated visually to be the lowest concentration of NPs that can provide ≥95% reduction in microorganism growth as compared with the growth control wells.Citation23 Each experiment was repeated three times.
Moreover, the media of the yeast containing plate wells that exhibited no visible yeast growth were further cultured on Sabouraud dextrose agar (EMD Millipore) to determine the minimum fungicidal concentration (MFC). In addition, the media of bacteria consisting of wells with no apparent bacteria growth were cultured on Müller-Hinton agar (EMD Millipore) to recognize the minimum bactericidal concentration (MBC). MFCs and MBCs were identified as the lowest concentrations of microorganism producing no more than four colonies, which refers to a 98% microorganism mortality in the initial inoculums. Concentrations of Ag and Fe ions in the suspension were measured by ICP and equaled to 0.070 and 0.010 mmol/g, respectively.
Disk diffusion assay
To screen the antimicrobial activity of the NP, initially, disk diffusion test was used. Briefly, the tested microorganisms were cultured on proper media. Then, the microorganism strains were suspended in 5 mL of normal sterile saline solution and mixed with vortex thoroughly to obtain a homogeneous suspension. Optical density (OD) of the prepared suspensions was adjusted to reach a turbidity of 0.5 McFarland standards. A swab of sterile cotton, which was moistened by the inoculum suspension, was rubbed on a plate with 90 mm diameter filled with Müller-Hinton agar and supplemented by 2% glucose (for organism nutrition and growth) and 0.5 µg/mL methylene blue (to enhance definition of the zone edge). The plates were dried for 3–10 min. Then, the patterns of antibacterial susceptibility of the NP were determined by soaking the blank disks in NP solutions and drying the Petri dishes in air. After that, the disks were dispensed onto the inoculated plates, the plates were incubated at 37°C for 24 h, and the inhibition zones around the disks were read.
L. major cytotoxicity assay: parasite culture
MRHO/IR/75/ER L. major promastigotes was cultured in the RPMI-1640 medium including L-glutamine, 10%–20% fetal calf serum (FCS) and antibiotics at 24°C for 3 days. Each time, 3 × 106 promastigotes were transferred to 4 mL of the enriched RPMI-1640 medium.
L. major promastigotes were moved to 96-well plates, treated with various NP concentrations and tracked using a light microscope. After 24 h exposure, 25 µL of 5 mg/mL 3-(4,5-dimethylthiazol-2-yl)-2,5-diphenyltetrazolium bromide (MTT) was added to each well, and the plate was incubated for 4 h at 24°C–25°C. The well supernatants were completely removed without disturbing the cell sediments. Then, each well received 100 µL of dimethyl sulfoxide (DMSO). The microplate was shaken for 15 min. After that, the absorbance of the well contents was recorded at 570 nm using a microplate reader. Cell viability was calculated using the following formula: viable cells (%) = (AT − AB)/(AC − AB) × 100, where AC, AT and AB are the absorbances of the untreated (negative control), treated and blank samples, respectively. Viability of the treated and untreated promastigotes was concerned through measuring MTT conversion to its reduced form, which is a measure of cell viability. If the amount of MTT declined, then the toxicity of the NP for promastigotes was declared.
Cell morphology study
The impact of the NP on bacterial cell morphology was evaluated using atomic force microscopy (AFM; Vecco, San Jose, CA, USA). MIC50 dosage of the NP was used to treat fresh bacterial cultures (OD 0.2) for 3 h. Then, the cells were washed with a saline phosphate buffer (pH 7), and 2.5% glutaraldehyde was added to them.Citation11 A drop of the diluted cell suspension was inserted on a cover slip and vacuum dried prior to AFM studies.
MTT assay
Since Fe3O4@PEG–Ag might be toxic to both pathogenic and animal cells, a toxicological assay was conducted on a human cell line to diagnose any health risks during practical applications of the NP. Adipose-derived mesenchymal stem cells (ADMSCs) were purchased from Stem Cell Laboratory of Shiraz University of Medical Sciences and seeded in a 96 well-plate. A total of 5,000 cells/well were used in 100 mL of Dulbecco’s Modified Eagle’s Medium (DMEM) that was supplemented with 10% fetal bovine serum (FBS) and incubated at 37°C for 4 h in a 5% CO2 incubator. After cell cohesion, the supernatant was replaced with an equal volume of fresh medium, and the plate was incubated for 24 h. The well contents were prepared by serial dilution of Fe3O4@ PEG–Ag (2, 4, 8, 16, 32, 64, 128, 256, 512 and 1,024 µg/mL). The control wells consisted of the culture medium with no NP. All NPs and control samples were put in three plate wells to validate the results statistically. After 24 h of cell incubation with various NP concentrations, including the control group, the growth medium was removed and 100 mL of MTT was added to the wells. Incubation was prolonged for 3 h. Then, the medium was removed, and formazan crystals were dissolved in 100 mL of DMSO for 15 min in the incubator. Absorbance of the well contents was read at 595 nm by an enzyme-linked immunosorbent assay (ELISA; Bio-Rad Laboratories Inc., Hercules, CA, USA) reader. Cell percentage viability was defined according to the following formula: cell viability = (Isample/Icontrol) × 100.
Results and discussion
Morphological study
The morphology of the relatively homogeneous NPs synthesized through coprecipitation was studied using field emission scanning electron microscopy (FESEM; ). To gain more insight into the morphology, shape and size of the NPs, transmission electron microscopy (TEM) was carried out. TEM microscopy unraveled relatively good dispersity of the NPs featuring 20–25 nm spherical core/shell morphology (). In addition, the TEM image revealed immobilization of a 5 nm thick layer of PEG coated on the surface of Fe3O4 NPs, which demonstrated the core/shell structure of Fe3O4@PEG–Ag.
Figure 1 FESEM image of Fe3O4@PEG–Ag core/shell nanostructure.
Abbreviations: FESEM, field emission scanning electron microscopy; PEG, poly(ethylene glycol).
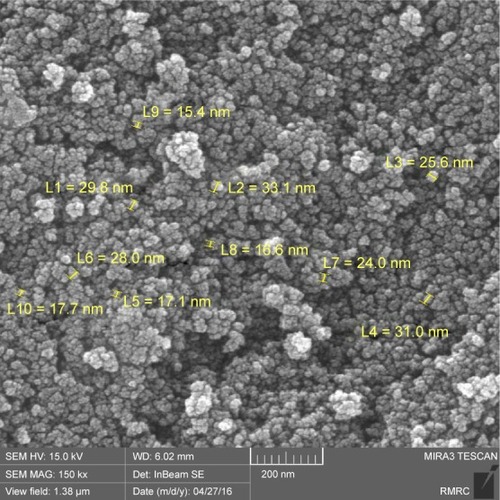
Figure 2 TEM image of Fe3O4@PEG–Ag core/shell nanostructure: the electrostatic forces between Fe3O4, PEG and Ag are the basis of the nanocomposite formation.
Abbreviations: TEM, transmission electron microscopy; PEG, poly(ethylene glycol).
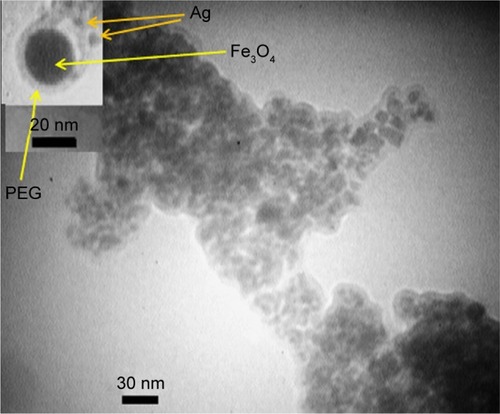
In addition to scanning electron microscopy (SEM) imaging, qualitative information about distribution of different chemical elements that are present in the matrix of the NPs was provided by combining SEM with a wavelength-dispersive X-ray (WDX) detector. The representative SEM image and the associated elemental map of Fe3O4@PEG–Ag are displayed in . As it can be seen in this figure, Ag particles have dispersed well in the composite. Uniformity of the prepared NPs was confirmed by the homogeneous existence of C, O, Fe and Ag throughout the sample based on elemental analysis of the selected area.
Figure 3 (A–E) correspond to elemental mapping (WDX) of composite Fe3O4@PEG–Ag, Fe, O, C and Ag, respectively.
Abbreviations: WDX, wavelength-dispersive X-ray; PEG, poly(ethylene glycol).
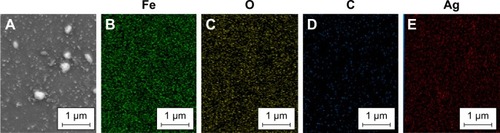
The surface chemical structure of Fe3O4@PEG NPs was characterized by Fourier-transform infrared (FT-IR) spectroscopy. The FT-IR spectrum of PEG-coated Fe3O4 NPs is illustrated in . The presence of magnetite NPs was approved by the appearance of Fe−O bond characteristic absorption at ~563 or 580 cm−1.Citation30 Furthermore, attachment of PEG on the Fe3O4 surface was further verified by observing 2,887 cm−1 (C−H asymmetric stretching) and 1,115 cm−1 (C−O−C stretching) peaks that are attributed to amorphous PEG.
Figure 4 FT-IR spectra of Fe3O4@PEG–Ag.
Abbreviations: FT-IR, Fourier-transform infrared; PEG, poly(ethylene glycol); T, transmittance.
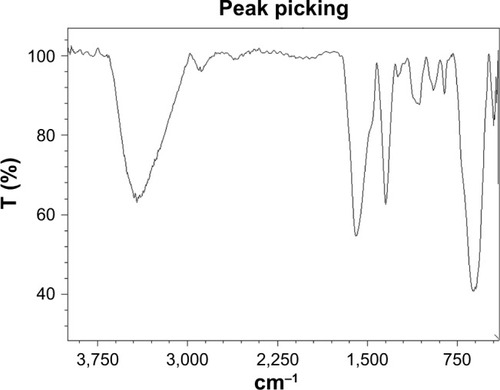
Energy-dispersive X-ray spectrum (EDS) of the NPs is presented in , which includes several signals related to carbon, silver, oxygen and iron. The rise of strong signals in the regions associated with iron and silver declared formation of the nanocomposite of interest. As expected, typical Fe nanocrystals’ optical absorption peaks are located at ~1.0 and 7.0 keV because of surface plasmon resonance. Here, higher amounts of C, N and O are observed due to the presence of PEG as the NP’s shell.
Figure 5 EDS of Fe3O4@PEG–Ag.
Abbreviations: EDS, energy-dispersive X-ray spectrum; PEG, poly(ethylene glycol).
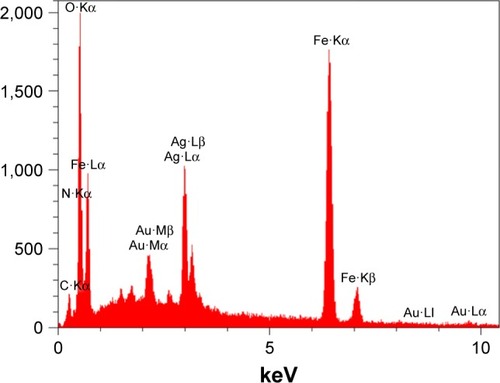
A vibrating sample magnetometer (VSM) was used to analyze the magnetic properties of Fe3O4@PEG–Ag NPs (). As depicted in this figure, Fe3O4@PEG–Ag can show 26.6 emu⋅g−1 saturation magnetization. This value indicates the adequacy of the NPs’ magnetism to facilitate their magnetic separation.
Antimicrobial properties of the nanocomposite
The antibacterial activity of the nanocomposite against E. coli and S. aureus was evaluated in the agar medium and broth dilution, while its antifungal activity was investigated against C. albicans, as one of the pathogenic species, using broth dilution. The results including the inhibition zones of the positive control (tetracycline) are depicted in . The solid test displayed the widest diameter of inhibition zone on the bacteria species for the positive control. Comparison of the findings related to the magnetic NPs, ie, Fe3O4, Fe3O4@ PEG and Fe3O4@PEG–Ag, distinguished the role of silver NPs loaded on the PEG layer in observation of the NP’s antibacterial properties (). In addition, its superior antibacterial activities against S. aureus in comparison with E. coli, MIC, MBC and MFC are summarized in .
Table 1 Antibacterial activities of nanocomposites (diameter of inhibition zone [mm])
Table 2 MIC (µg/mL), MBC (µg/mL) and MFC (µg/mL) of Fe3O4@ PEG–Ag for various microorganisms
MTT assay
Performing MTT assay showed that exposing the cells to the NP for 24 h can provide the concentration-dependent cytotoxicity (). At 2, 4 and 8 µg/mL NP concentration, the viability of the cells after 24 h of incubation was 98.5%, 89% and 100%, respectively. Elevating NP concentration (16, 32, 64, 128, 256, 512 and 1,024 µg/mL) resulted in reduced percentage of cell viability from 100% tô50% after 24 h.
L. major cytotoxicity assays
L. major promastigotes distributed among the plate wells were treated with various NP concentrations and analyzed using a light microscope (). After 24 h of exposure to NPs, 25 µL of 5 mg/mL MTT was added to each well. Then, the plate was incubated at 24°C–25°C for 4 h. In the next step, supernatants of the wells were removed completely without perturbing the precipitated cells, and 100 µL of DMSO was added to each well. The microplate was shaken for 15 min, and the absorbance of the well solutions was recorded at 570 nm by a microplate reader. Cell viability was determined using the following formula:
Figure 9 Cell viability was measured by MTT assay.
Notes: Data are shown as mean ± SD with n = 3. P < 0.0001 compared with the negative control.
Abbreviation: MTT, 3-(4,5-dimethylthiazol-2-yl)-2,5-diphenyltetrazolium bromide.
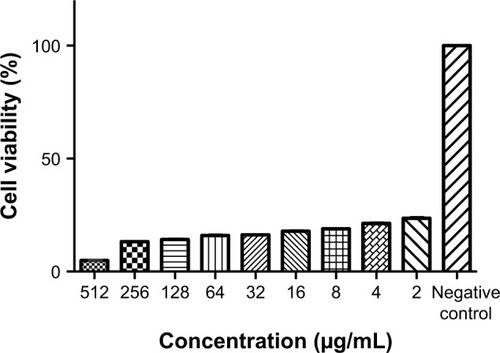
AFM analysis of the bacterial cells showed that NPs affect the microorganisms’ cell walls, and shrinkage morphology of their cells has been shown in .
Conclusion
In this study, Fe3O4@PEG–Ag NPs were synthesized by relying on electrostatic forces and a reducing agent. The structural properties of the NPs were determined through FT-IR, FESEM–EDS, VSM, ICP-MS and TEM techniques. Furthermore, the antimicrobial activity of the NPs was investigated by exposing the particles to several pathogenic microorganisms including S. aureus, E. coli and C. albicans in the framework of broth microdilution method. In addition, the toxicity of the NPs for the animal cell line was evaluated by the MTT assay. The findings revealed that Fe3O4@PEG–Ag nanocomposite can be one of the promising antimicrobial alternatives with an insignificant toxicity. Moreover, this nanostructure can be applied as a smart drug delivery system with the help of an external magnetic field or can be used as a powerful antibiotic agent along with other antibiotics that can form a shell on its structure.
Acknowledgments
We are grateful to Payame Noor University (PNU) and Shiraz University of Medical Sciences for financial support.
Disclosure
The authors report no conflicts of interest in this work.
References
- ZhangZYueXWeiDDMSO-based PbI2 precursor with PbCl2 additive for highly efficient perovskite solar cells fabricated at low temperatureRSC Adv20155127104606104611
- YeERegulacioMDBharathiMSAn experimental and theoretical investigation of the anisotropic branching in gold nanocrossesNanoscale20168154355226645742
- HidronAIEdwardsJRPatelJAntimicrobial-resistant pathogens associated with healthcare-associated infections: annual summary of data reported to the National Healthcare Safety Network at the Centers for Disease Control and Prevention, 2006–2007Infect Control Hosp Epidemiol20082911996101118947320
- MayrhoferSPaulsenPSmuldersFJHilbertFAntimicrobial resistance profile of five major food-borne pathogens isolated from beef, pork and poultryInt J Food Microbiol2004971232915527915
- SundarSDrug resistance in Indian visceral leishmaniasisTrop Med Int Health200161184985411703838
- HadighiRMohebaliMBoucherPHajjaranHKhamesipourAOuelletteMUnresponsiveness to Glucantime treatment in Iranian cutaneous leishmaniasis due to drug-resistant Leishmania tropica parasitesPLoS Med200635e16216605301
- VeisiHHemmatiSShirvaniHVeisiHGreen synthesis and char-acterization of monodispersed silver nanoparticles obtained using oak fruit bark extract and their antibacterial activityAppl Organomet Chem2016306387391
- VeisiHFarajiARHemmatiSGilAGreen synthesis of palladium nanoparticles using Pistacia atlantica kurdica gum and their catalytic performance in Mizoroki–Heck and Suzuki–Miyaura coupling reactions in aqueous solutionsAppl Organomet Chem2015298517523
- VeisiHGhorbani-VagheiRHemmatiSAlianiMHOzturkTGreen and effective route for the synthesis of monodispersed palladium nanoparticles using herbal tea extract (Stachys lavandulifolia) as reductant, stabilizer and capping agent, and their application as homogeneous and reusable catalyst in Suzuki coupling reactions in waterAppl Organomet Chem20152912632
- VeisiHRashtianiABarjastehVBiosynthesis of palladium nanoparticles using Rosa canina fruit extract and their use as a heterogeneous and recyclable catalyst for Suzuki–Miyaura coupling reactions in waterAppl Organomet Chem2016304231235
- KeihanAHVeisiHVeasiHGreen synthesis and characterization of spherical copper nanoparticles as organometallic antibacterial agentAppl Organomet Chem2017317e3642
- KeihanAHVeisiHBiabriPMFacile synthesis of PEG-coated magnetite (Fe3O4) and embedment of gold nanoparticle as a nontoxic antimicrobial agentAppl Organomet Chem20173112e3873
- VeisiHNasrabadiNHMohammadiPBiosynthesis of palladium nanoparticles as a heterogeneous and reusable nanocatalyst for reduction of nitroarenes and Suzuki coupling reactionsAppl Organomet Chem20163011890896
- PirhayatiMVeisiHKakanejadifardAPalladium stabilized by 3, 4-dihydroxypyridine-functionalized magnetic Fe3O4 nanoparticles as a reusable and efficient heterogeneous catalyst for Suzuki reactionsRSC Adv20166322725227259
- VeisiHSedrpoushanAMalekiBHekmatiMHeidariMHemmatiSPalladium immobilized on amidoxime-functionalized magnetic Fe3O4 nanoparticles: a highly stable and efficient magnetically recoverable nanocatalyst for sonogashira coupling reactionAppl Organomet Chem20152912834839
- VeisiHGholamiJUedaHMohammadiPNorooziMMagnetically palladium catalyst stabilized by diaminoglyoxime-functionalized magnetic Fe3O4 nanoparticles as active and reusable catalyst for Suzuki coupling reactionsJ Mol Catal A Chem2015396216223
- VeisiHSedrpoushanAHemmatiSPalladium supported on diaminoglyoxime-functionalized Fe3O4 nanoparticles as a magnetically separable nanocatalyst in Heck coupling reactionAppl Organomet Chem20152912825828
- KhakianiBAPourshamsianKVeisiHA highly stable and efficient magnetically recoverable and reusable Pd nanocatalyst in aqueous media heterogeneously catalysed Suzuki C–C cross-coupling reactionsAppl Organomet Chem2015529259265
- MaJZhangJXiongZYongaYZhaoXSPreparation, characterization and antibacterial properties of silver-modified graphene oxideJ Mater Chem2011211033503352
- LiongMFranceBBradleyKAZinkJIAntimicrobial activity of silver nanocrystals encapsulated in mesoporous silica nanoparticlesAdv Mater Deerfield2009211716841689
- ShiQVitchuliNNowakJOne-step synthesis of silver nanoparticle-filled nylon 6 nanofibers and their antibacterial propertiesJ Mater Chem201121281033010335
- XueWHuangDZengGNanoscale zero-valent iron coated with rhamnolipid as an effective stabilizer for immobilization of Cd and Pb in river sedimentsJ Hazard Mater201834138138928806558
- HuangDXueWZengGImmobilization of Cd in river sediments by sodium alginate modified nanoscale zero-valent iron: impact on enzyme activities and microbial community diversityWater Res2016106152527693995
- GongXHuangDLiuYStabilized nanoscale zerovalent iron mediated cadmium accumulation and oxidative damage of Boehmeria nivea (L.) Gaudich cultivated in cadmium contaminated sedimentsEnviron Sci Technol20175119113081131628850225
- MathewTVKuriakoseSStudies on the antimicrobial properties of colloidal silver nanoparticles stabilized by bovine serum albuminColloids Surf B Biointerfaces2013101141822796767
- ChatterjeeAKSarkarRKChattopadhyayAPAichPChakrabortyRBasuTA simple robust method for synthesis of metallic copper nanoparticles of high antibacterial potency against E. coliNanotechnology201223808510322293320
- JayaprakashNVijayaJJKaviyarasuKGreen synthesis of Ag nanoparticles using Tamarind fruit extract for the antibacterial studiesJ Photochem Photobiol B201716917818528347958
- KaufmanCLWilliamsMRyleLMSmithTLTannerMHoCSuperparamagnetic iron oxide particles transactivator protein-fluorescein isothiocyanate particle labeling for in vivo magnetic resonance imaging detection of cell migration: uptake and durabilityTransplantation20037671043104614557750
- MondiniSCenedeseSMarinoniGOne-step synthesis and functionalization of hydroxyl-decorated magnetite nanoparticlesJ Colloid Interface Sci2008322117317918387621
- D’souzaAJMSchowenRLToppEMPolyvinylpyrrolidone–drug conjugate: synthesis and release mechanismJ Control Release20049419110014684274
- EdmondMBWallaceSEMcClishDKPfallerMAJonesRNWenzelRPNosocomial bloodstream infections in United States hospitals: a three-year analysisClin Infect Dis199929223924410476719
- KaviyarasuKKanimozhiKMatiniseNAntiproliferative effects on human lung cell lines A549 activity of cadmium selenide nanoparticles extracted from cytotoxic effects: investigation of bio-electronic applicationMater Sci Eng C20177610121025
- KaviyarasuKGeethaNKanimozhiKIn vitro cytotoxicity effect and antibacterial performance of human lung epithelial cells A549 activity of zinc oxide doped TiO2 nanocrystals: investigation of bio-medical application by chemical methodMater Sci Eng C201774325333
- MagdalaneCMKaviyarasuKVijayaJJSiddhardhaBJeyarajBPhotocatalytic activity of binary metal oxide nanocomposites of CeO2/CdO nanospheres: investigation of optical and antimicrobial activityJ Photochem Photobiol B2016163778627541568
- EzhilarasiAAVijayaJJKaviyarasuKMaazaMAyeshamariamAKennedyLJGreen synthesis of NiO nanoparticles using Moringa oleifera extract and their biomedical applications: cytotoxicity effect of nanoparticles against HT-29 cancer cellsJ Photochem Photobiol B201616435236027728880
- KaviyarasuKMaria MagdalaneCKanimozhiKElucidation of photocatalysis, photoluminescence and antibacterial studies of ZnO thin films by spin coating methodJ Photochem Photobiol B201717346647528668515
- WaynePClinical and Laboratory Standards Institute (CLSI) Method for Dilution Antimicrobial Susceptibility Tests for Bacteria That Grow AerobicallyApproved standard–8th edWayne, PACLSI2009CLSI document M07-A8