Abstract
Background
Polymeric delivery systems have been elucidated over the last few years as an approach of achieving high therapeutic effect to the local site of malignant disease patients who have cancer. Polypyrrole (Ppy) is a potential organic conducting polymer which has long been recognized as a versatile material due to its excellent stability, conductive properties, and great absorbance in the range of near-infrared (NIR). It is tremendously versatile for use in various biomedical fields such as cancer therapy. NIR irradiation-activated treatment platform technologies are now being considered to be novel and exciting options in potential nanomedicine. However, the realistic photothermal use of Ppy-applied nanomaterials is yet in its early phase, and there are a few disadvantages of Ppy, such as its water insolubility. In the clinic, the common approach for treatment of lung cancer is the delivery of therapeutic active substances through intratumoral administration. Nevertheless, the tumor uptake, regional retention, mechanism of treatment, and tissue organ penetration regarding the developed strategy of this nanomaterial with photothermal hyperthermia are important issues for exerting effective cancer therapy.
Materials and methods
In this study, we developed a cationic Ppy–polyethylenimine nanocomplex (NC) with photothermal hyperthermia to study its physicochemical characteristics, including size distribution, zeta potential, and transmission electron microscopy, scanning electron microscopy, and Fourier transform infrared morphology. We also examined the cellular uptake effect on lung cancer cells, the photothermal properties, intracellularly generated reactive oxygen species (ROS), and cytotoxicity.
Results
The results suggested that this nanocarrier system was able to effectively attach onto lung cancer cells for subsequent endocytosis. The NCs taken up were able to absorb NIR and then converted the NIR light into local hyperthermia with its intracellular photothermal performance to provide local hyperthermic treatment. This regionally generated hyperthermia also induced ROS formation and improved the killing of lung cancer cells as a promising local photothermal therapy.
Conclusion
This development of a nanocarrier would bring a novel therapeutic strategy for lung cancer in the future.
Introduction
Chemotherapy has been mainly used for cancer in these middle and later stage and is able to be administered before or after medical resection, in place of surgery when the tumorous region is unresectable. However, therapeutic approaches would cause a drug sensitized response, low bioavailability, and other harmful side effects.Citation1,Citation2 Since systemic chemotherapy intravenously administrated is not exclusively distributed to the tumorous site, it is actually hard to attain beneficial dosage levels of active medicine inside or around the tumorous region. Moreover, a substantial amount of active medicine regularly accumulates within normal tissues, causing toxic reactions and undesired side effects.Citation3
Thermo-brachytherapy for instance, providing a means of local hyperthermia, involves the grafting of combining radiated seeds of point source intratumorally, near the tumorous region, or at the operating margins of resection, and they have been confirmed as a useful local therapeutic choice to treat the inoperable tumor and to prevent the recurrence of localized tumors. This locally therapeutic approach has been regularly administered to patients who have slow growing tumors, to be a substitute for the surgical option and has revealed efficacy in numerous dangerous cancers such as cervical, breast, and lung tumors.Citation4–Citation6
Polymerically delivered systems providing a therapeutic effect have been considered over several years as an approach for improving on the absence of targeting of lung tumor and serious morbidities related to systemically chemotherapeutic therapies. Some biomaterial depots offer biocompatibility and are formulated to preserve therapeutic effects at sites of tumor for a sustained period of time. Passive targeting also involves the usage of characteristic features of polymeric nanomaterials such as the charged effect on targeting the cancer cell. Cancer cells bear a rather high negative surface charged property compared with normal healthy cells, thus allowing favored attaching by cationic nanoparticle systems.Citation7 Targeting of a positively charged nanomaterials system is achievable by electrostatic cellular binding onto negatively charged phospholipid head-groups favorably expressed on cancer cells with retained properties. Cationically polymeric materials with net positive surface charge feature emerge as a promising option because of their excellent cellular uptake and extremely strong cancer cellular interaction properties. Among the positively charged polymers, polyethylenimine (PEI) has been proved to have the highest delivery efficiency of active substances toward cells owing to its large positively charged feature that facilitates a high degree of drug incorporation and an efficient ability to escape the cell endosome upon an internalized process.Citation8
In hyperthermia treatment, biocompatible conductive polymers have photothermal convertible efficiencies and photostability that would be higher than commercially available inorganic-based photothermal ablation agents.Citation9 Poly-pyrrole (Ppy) functions as one potential organic conductive polymeric material and is extensively applied for organic electronic use due to its excellent stability, conductivity, and great absorbance in the near-infrared (NIR) range.Citation10 Ppy is tremendously useful for various biological applications.Citation11,Citation12 NIR irradiation-activated therapy platform technologies are now being considered as novel and exciting options for potential nanomedicine uses.Citation13 As a valuable photothermal reagent, Ppy has been studied for hyperthermia therapy because of its excellent NIR absorption band gap of photothermal behavior with promising biocompatibility.Citation14,Citation15 However, the practical photothermal use of Ppy-applied nanomaterials is yet in its infant period, and there are a few disadvantages of Ppy, such as its water insolubility.Citation16,Citation17
Ppy-based multifunctional nanomaterials for clinical cancer treatment are an emerging medical trend and an aim for cancer therapy. In the study, synthesis of a PEI nanoencapsulating Ppy (Ppy–PEI NC) and outcomes of its photothermal properties after NIR-triggered alteration are discussed. In the clinic, hyperthermic treatment can be deliberately induced using medicinal or biomedical strategies and has been elucidated and routinely used in hospitals as a therapy for dangerous cancers.Citation18 Local hyperthermic treatment provides a good therapeutic choice for patients who may have issues regarding recurrent or serious cancer.Citation6 In the clinic, the common way for bronchoscopic treating toward lung cancer is delivering of therapeutic active anticancer substances through intratumoral administration.Citation19 However, the tumor uptake, regional retention, and tissue organ penetration regarding the developed strategy of this cationic nanomaterial with photothermal hyperthermia are other important issues for exerting effective cancer therapy.Citation20 For instance, cationic nanoparticles enable increased active substance concentration in the tumor site and improved therapeutic effects.Citation21
In addition, the mechanism of local hyperthermia is related to the localized generating of reactive oxygen species (ROS) for example free radicals and the practical damage to mitochondria in numerous types of cancer cell lines. ROS and mitochondrial dysfunction might be important functions in the cell apoptotic procedure.Citation22 ROS are generally well defined as containing oxygen and active chemical substances.Citation22,Citation23 Usually, ROS are classified as two kinds.Citation23 ROS which are commonly observed in biological systems include hydroxyl radicals, superoxide, and nitric oxide, ozone, hydroxide, peroxynitrite, and hydrogen peroxide.Citation24
In this study, we developed a Ppy–PEI NC and elucidated its physicochemical properties. Its internalization behavior in lung cancer cells, photothermal properties, intracellularly generated ROS, and cytotoxicity mechanism were also elucidated. We hypothesized that this designed carrier system attaches onto the surface of lung cancer cells for subsequent endocytosis. Next, the endocytosed NCs would be able to receive NIR and provide local hyperthermia. This locally generated hyperthermia could induce intracellularly generated ROS and facilitate ablation of lung cancer as a promising photothermal treatment in cancer therapy.
Materials and methods
Preparation of the test samples
Tested chemical products and experimental kits were purchased from Sigma-Aldrich Co. (St Louis, MO, USA) except where announced otherwise and were in analytical grade. Chemicals applied in cell researched works were obtained from Life Technologies (Thermo Fisher Scientific, Waltham, MA, USA).
Ppy–PEI NCs were manufactured with a nano-formulated process that was slightly modified from that previously described.Citation25 In brief, the cationic polymeric PEI (200 mg, 600 Da) was dissolved in a deionized (DI) water (10–20 mL) and subsequently mixed with a prepared amount of monomer of pyrrole (12.5 µL). The above mixed solution was then stirred for 30 min in an acid environment (at pH 0.8). Ferric chloride hexahydrate (12.5 mg/mL, 1 mL) was then added to this aqueous. Next, in a volume polymerized chemical reaction lasting 0.5 h, the acquired black blended fluid was purified with a dialysis bag against DI water to eliminate free PEI and ferric ions. To calculate the NC amount and to obtain NC, the procedure of centrifugation (20 min) and oven dry were performed.
Physicochemical characterization of the test Ppy–PEI NCs
Ppy–PEI NCs were first suspended in different pH concentrations, and then the zeta potential and size distribution data were recorded by dynamic light scattering (DLS) (Malvern Instruments, Malvern, UK), and morphological consequences were visualized by the transmission electron microscopy (TEM) or the scanning electron microscopy (SEM). The Fourier transform infrared (FTIR) spectroscopy was carefully used to resolve molecular structural changes of the test sample. To understand the photothermal behavior, the test sample was immersed in a DI water at different concentrations or the formulations were at different pH environments then exposed to an NIR laser (808 nm, power 2.0 W cm−2, 5 min). The infrared thermographic photographic images were gotten with a thermal camera. The Thermocouple was used to study the quantitative temperature profile. To test the ability of the test Ppy–PEI NCs to attach onto the gelatin surface, a cationic gelatin (A) or anionic gelatin (B) was placed on a confocal dish at room temperature. Before this study, the test gelatin and Ppy–PEI NCs were respectively labeled with cyanine 5 (Cy5) and fluorescein N-hydroxysuccinimide (NHS) esters through covalent conjugation. Next, the fluorescent fluorescein-Ppy–PEI NCs (2.4 mg/mL) were placed on a gelation state of fluorescent Cy5-gelatin (20%, 25°C) on a confocal dish. After 60 min, the unbound fluorescein-Ppy–PEI NCs were flushed with phosphate-buffered saline (PBS) for subsequent confocal laser scanning microscopy (CLSM) examination. A dual image of fluorescent fluorescein-Ppy–PEI NCs and Cy5-gelatin was then spatially imaged by CLSM.
Cellular interactions with the test formulation
Lung cancer cells H460, human lung large cell carcinoma (from ATCC® HTB-177™; American Type Culture Collection, Manassas, VA, USA) were kept in cellgrowth culture medium (5% CO2, 37°C, 10% fetal bovine serum). Test cells were seeded into the confocal dishes, and then these dishes were kept in a cell incubator at 37°C and 5% CO2 overnight. Before study, the attached cells were kept in Hank’s balanced salt solution (HBSS) for 1 h. Afterward, cells on the dish were incubated with different tested formulations for 1 h. The test sample was then flushed 3 times with PBS and stained by 4′,6-diamidino-2-phenylindole (DAPI), a solution of 2,7-dichlorodihydrofluorescein diacetate (DCFH-DA, ROS dye), or Amplex Red (hydrogen peroxide dye) to elucidate biocellular interactions with the developed Cy5-NC system for visualizing the NC and cell biological interactions.Citation26 To create a hyperthermia environment, the test sample with cells were placed in a water bath incubator with an additional heat source, or the cells were treated with NC/NIR. Fluorescent results were visualized through CLSM. The fluorescence signal intensity was studied using ImageJ software.
Cell viability
Test H460 cells were cultured in 96-well plates with cell growth medium at 104 cells/well and 37°C with 5% CO2 overnight. The tested cancer cells were flushed twice with HBSS and then supplemented with 200 µL test Ppy–PEI NCs dissolved in HBSS. After 1 h, the above solution was withdrawn by flushing twice with PBS. Next, with or without remote NIR irradiation (10 min under 2 W/cm2), 200 µL freshly prepared medium together with 20 µL of MTT chemical reagent with a stock mixture (5 mg/mL dissolved in PBS) was used, followed by subsequent cell culture in 4 h at 37°C. Once withdrawing the medium, the formazan formed chemicals were extracted by dimethyl sulfoxide (DMSO) and incubated for 20 min. The recorded absorbance information (formazan) was attained using a microplate reader. For qualitatively imaging cellular viability following incubation with different formulated treatments lasting 1 h, as mentioned (MTT) above, with subsequent NIR irradiation (10 min), flushed cells were then stained by live/dead chemical reagent in a commercial kit, as described in the standard protocol. Briefly, the live/dead viability/cytotoxicity assay kit (Molecular Probes, Eugene, OR, USA) contained dead cell dye (ethidium homodimer) with live cell dye (acetoxymethyl ester of calcein [calcein AM]) stock solutions diluted to their formulated mixture (4 and 2 µM, respectively) in PBS. Next, the cells were placed in this aqueous reagent at room temperature for 30-min reaction. Fluorescent data were measured with microscopy.
Statistical analysis
The experimental data are expressed as average±SD. The Student t-test was conducted to calculate differences between pairs of groups. P<0.05 was deemed to show statistical significance.
Results and discussion
Characteristics of Ppy–PEI NCs
Recently, researchers have observed the extraordinary development of nanotechnologies.Citation27 There has been growing hope that nanoscience will achieve practical noteworthy progress in medication and clinical use in analyses, therapeutic strategies, and avoidance of diseases. Increasing attention in forthcoming biomedical requests for nanoscale treatment is guiding the appearance of the novel arena called nanomedicine.Citation28,Citation29
The versatility of conductive polymers, for instance Ppy, has been widely studied for their exclusive chemical and physical features, and they have various functions in numerous fields such as biology and chemistry sensors, organic electronic devices, and electromagnetic sheltering applications. Conducting polymeric Ppy was also described as having poor dispersion in aqueous phases which restricts its applicability.Citation30
One of the main difficulties in generating dispersed nano-Ppy is the poor homogeneity of Ppy molecules in aqueous systems. Dispersion is worse for coated polymers without polar groups, as the polarity of the coating polymer has an impact on the dispersion of Ppy. In order to overcome this dispersion problem, the Ppy interface is usually coated with a dispersion polymeric agent in an nano-formulation process, such as (eg, heparin,Citation31 polyvinyl acetate,Citation32 and chi-tosanCitation33) previously exposed to polymerized pyrrole under mechanical stirring. These dispersed polymeric molecules are appropriately functionalized organic molecules which allow stabilization of Ppy polymeric molecules in aqueous solutions.
As shown by TEM and photographic data in , Ppy lacks stability in the aqueous phase owing to its hydro-phobicity, so that precipitation and aggregation behaviors occurred after the Ppy material was introduced into water. Once Ppy was stabilized with the polymeric PEI, nanoscale Ppy–PEI NCs were obtained as shown in as a uniformly dark suspended fluid. SEM results suggested that the nano-dimension of Ppy–PEI NCs with a well-dispersed arrangement was possibly due to the smaller size together with stronger repulsive cationic electronic fields of each nanoformulated particle (). Details of the size properties were measured by DLS. The DLS results indicated that particle size tends to increase with increasing pH value, while the zeta potential was reduced (). The PEI-containing nanoparticle should be deprotonated at high pH,Citation34 leading to the lack of interaction from each particle so that PEI would lack a strongly charged surface to generate repulsive force, resulting in particle aggregation.
Figure 1 (A) Results indicating photographic images of polypyrrole (Ppy) and Ppy–PEI NCs and their microscopic TEM. (B) Results of SEM showing prepared Ppy–PEI NCs with nano-size. DLS study shows the size distribution (C), zeta potential (D), and polydispersity index (E) of the different pH environments.
Abbreviations: NC, nanocomplex; PEI, polyethylenimine; SEM, scanning electron microscopy; TEM, transmission electron microscopy; DLS, dynamic light scattering.
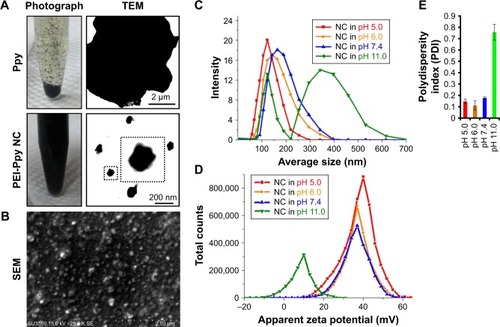
Photothermal properties of Ppy–PEI NCs
The results for the photothermal properties of prepared Ppy–PEI NCs at different concentrations and pH environments after NIR treatment studied by Thermocouple and thermal camera are shown in . The temperature was slightly increased after NIR irradiation in the DI water group. After applying NIR, the recorded temperature of Ppy–PEI NC solutions was increased in a concentration-dependent manner (). Owing to the presence of an aromatic ring of Ppy, the prepared Ppy–PEI NCs have the advantage of noninvasively remotely generating local hyperthermia once exposed to NIR.Citation25 The temperature profile of Ppy–PEI NC solutions irradiated with NIR would be reduced on increasing the pH value (). It was described that the smaller size of photoablation agent particles would relate to higher conductivity as mentioned previously.Citation35,Citation36 Larger nanoparticles or aggregated particle resultant inhomogeneous phase might associate with lower photothermal conversion efficiency.Citation37 Hyperthermic temperature (HT) is a cancer treatment used together with surgical processes, gene immunotherapy, radiotherapy, and chemotherapy.Citation38 In oncology, clinical doctor uses an exterior heat source to locally raise the temperature of tissues and eradicate cancerous cells or obstruct their additional growth. A locally high temperature, as many studies discovered, sensitizes cells to handling modalities and triggers direct damage to cancer cells, thus increasing the irradiation and chemotherapeutic effects with minor or no damage to healthy tissues and organs. The healing abilities, therapeutic outlay, technical issues, and confirmation of efficiency diverge according to the HT method. Management of cancer with hyperthermia has been used previously, but use of this skill was discontinued due to its limitations, including failure to elevate the local temperature toward the object without harming nearby cells, difficulty reaching a uniform heat distribution in the cancer, and intrinsic issues with imperceptible micrometastases.
Figure 2 Photothermal property results. Temperature profile (A) at different concentrations of prepared NC and (B) at different pH environments. (C) Photothermal images (temperature value recorded by Thermocouple). The temperature elevated to 25 degree celsius is considered time 0 second.
Abbreviations: DI, deionized; NC, nanocomplex.
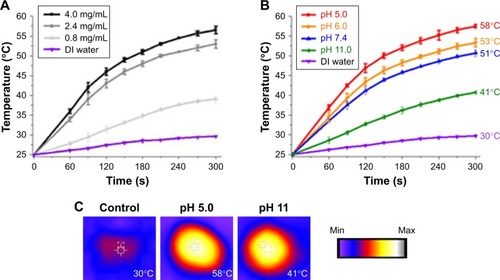
Surface properties of Ppy–PEI NCs
Around solid cancerous biology, neutrophils usually act as a dominant cell species in the tumorous tissue region surrounding infiltration.Citation39 The neutrophils with tumor surrounding tissue are the cells with cationic charged peptides/substances covering the surface.Citation40 Besides, the anionic charges were found to be generated from the huge amount of lactate secretions, a recognized feature of an entirely metabolically active cancerous cell line.Citation40 Thus, this different surface charged feature indicated that targeting negative surface charges of cancer cells by cationic nanoparticles with photothermal properties would provide selective targeting toward negatively charged lung cancer with cytotoxicity. To test this hypothesis of binding affinity, the cationic gelatin represents inflammation cationic tissue and anionic gelatin represents cancer cell surface.Citation41 In pathological conditions, cancer cells also have rather high negatively charged cellular membranes compared to surrounding cells.Citation40,Citation42,Citation43
For elucidation of the binding affinity toward different electronically charged surfaces, the prepared fluorescent Ppy–PEI NC was incubated with a gelatin (A) or gelatin (B) hydrogel, and then the unbound Ppy–PEI NC was flushed with PBS for subsequent CLSM examination. As shown in , the cationic Ppy–PEI NCs that accumulated on the negatively charged gelatin (B) hydrogel were greater than those on the gelatin (A) cationic hydrogel. The repulsive forces generated between the polyelectrolytes of the same charge that prevent interaction; in contrast, different electronic charges will generate an attractive force for molecularly enhanced binding affinity. Additionally, the generated internal heat in the tested hydrogels (anionic hydrogel) with residual cationic PEI-Ppy NP by irradiated NIR around 45°C. The temperature of cationic gel treated group was around 34°C (). Theoretically, these effects from temperature and binding affinity also should be an advantage for local administration of PEI-Ppy NPs with photothermal treatment (PTT) against lung cancer and could cause less damage to surrounding cationic inflammatory tissue.
Figure 3 (A) Fluorescence results show binding affinity of prepared NC in differently charged hydrogels and (B) their thermal properties. (C) Chemical structural change studied by Fourier transform infrared spectroscopy.
Note: *Statistically significant, P < 0.05.
Abbreviations: Cy, cyanine; NC, nanocomplex; NIR, near-infrared; PEI, polyethylenimine.
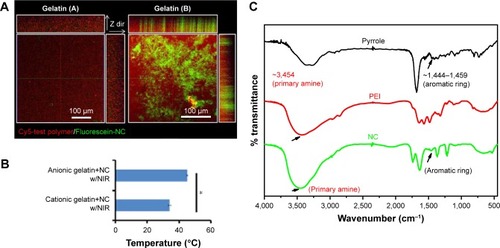
Chemical structure
To further analyse the molecule construction, FTIR was used to study the chemical structure of the synthesized compounds. The pyrrole–PEI NC product was indicated by characteristic peaks contributed by its respective chemicals, such as PEI and Ppy for comparison. The IR spectrum around 1,444~1,459 cm−1 44 possibly originated from stretching vibrations of aromatic ring. According to the chemical characteristic peak of PEI, around 3,454 (probable primary amine) with corresponding pyrrole–PEI NC compound ().Citation45
Recent developments in nanoparticle-assisted hyperthermia treatment support the capability to overcome most of targeted tissues, but some concern regarding the use of nanomaterials remains. It is known that surrounding healthy tissues and organs might display improved thermotolerance toward cancer cells, also the mediating mechanisms are mostly unclear. According to the location of the cancer (eg, deep-seated or superficial), various modalities of treatment should be implemented. Cellular apoptosis is associated with a trigger HT to cause death of cells with producing intracellular oxidative stress. Several studies confirmed that HT should be an applicable cancer treatment. As for terminology, hyperthermia (also called thermotherapy or thermal therapy) is a sort of cancer treatment where body tissues are exposed to high temperatures. An investigation revealed that high temperatures can destroy and eradicate cancer cells, typically with negligible damage to healthy tissues and organs. In PTT, NIR light (at around 650–900 nm) is chosen for its convenient use, its slight absorbance by tissues and skin to permit noninvasive penetration of deeper tissues and organs, and its aptitude to be locally intensive in a particular region.Citation46 The important constituent of this method is a transducer with photothermal properties that can efficiently absorb and convert remote NIR light into heat via a mechanism of nonradiation.
As a useful substitute or enhancement to traditional cancer treatment methods, PTT has garnered substantial attention due to its benefits, including slight invasiveness, few difficulties, and quick recovery. PTT, also called optical hyperthermia or photothermal ablation, requires photo absorbers and a source of NIR light energy, and offers an exact and negligibly invasive substitute for cancer management.Citation46 It is a technique based on local heating due to absorption of light to selectively destroy cancer cells. The photostability of Ppy-based nanoparticles would be contributed from the aromatic structure with dark color in aqueous.Citation47
Over the past decade, numerous distinct categories of photothermal therapeutic agents (PTAs) have been discussed, comprising organic materials and chemicals (eg, indo-cyanine green, polyaniline, and inorganic nanomaterials).Citation48 Once exposed to NIR light, all of these materials are able to create adequate heat to increase the local temperature to achieve hyperthermia and consequently kill cancer cells. It was noted that bioorganic PTT is multifunctional and has good biocompatibility, and thus should be able to be applied for nanobiotechnology.
Cancer cell interactions
A positively charged nanomaterial can interact with negatively charged (anionic) cancer cellular membranes to trigger depolarization of local cell membranes and also trigger subsequent intracellular endocytosis.Citation40,Citation49 Maintaining and preserving nanostructures on external cellular membranes, even with subsequent cellular interaction, and chemical and physical binding approaches to reactive groups characteristically present on their outer cellular interfaces were needed to understand. Cationic nanomaterials are rapidly attracted to cancer cells because of the positive attractive forces.Citation40 It is thought that positively charged nanocarriers may develop optimal bioapplications for cancer treatments in sustainable regional and mucosal therapies. In addition, these positively charged nanocarriers would offer protective effects against enzyme degradation and were revealed to influence the release of medicines or active therapeutic substances in a sustained, controllable manner.Citation50
Fluorescence image showed that Ppy–PEI NCs were endocytosed by H460 cells after 1 h of incubation. The accumulation of fluorescent Ppy–PEI NCs was appeared within cellular organelles near nuclei of H460 cells (). As is known, PEI-complexes cell uptake mechanism might be through clathrin-dependent pathways.Citation51 Furthermore, this developed endocytosed pathway through clathrin mediation can be a potential target for a therapeutic approach for overcoming tumor resistance.Citation52
Figure 4 (A) Fluorescent images show cell interaction with different formulations. (B) Fluorescence intensity was calculated by ImageJ software, and the statistical data analysis compared with NC/NIR (cy5-). NC Fluorescence group, left: NC, right: NC/NIR).
Notes: Black bar is ROS studied group; red bar is H2O2 studied group. *Statistically significant, P < 0.05.
Abbreviations: H2O2, hydrogen peroxide; NC, nanocomplex; NIR, near-infrared; ns, nonsignificant; ROS, reactive oxygen species; DAPI, 4′,6-diamidino-2-phenylindole.
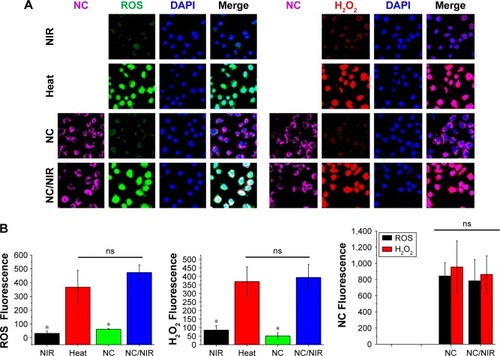
Previous results suggested that positively charged nanoparticles (NPs) might be internalized via clathrin coated vesicles.Citation53 Additionally, the size of nanomaterials may also affect the uptake kinetics and efficiency, the sub-cellular biodistribution, and the internalization mechanism. The dimension-dependent uptake of various biomaterials in diverse cellular lines has been studied with suitable cell internalization at a nanomaterial core dimension in a range of around 60–400 nm, which indicates that there is an ideal size distribution for active cellular uptake.Citation54
ROS detection
As known, hyperthermia stimulates intracellular ROS, and mitochondria functional disorders in numerous cancerous cell lines were found.Citation22 Mitochondria dysfunction and ROS act important roles in the cell apoptotic process. As indicated in , test cells treated with NIR alone or the group received with only Ppy–PEI NCs generated few ROS and few hydrogen peroxide was observed. However, test cells treated with an additional heat source or the group of Ppy–PEI NCs with NIR treatment generated highly ROS including hydrogen peroxide fluorescent products, as analyzed by CLSM and ImageJ software (). The greater amounts of ROS generated may be attributed to local hyperthermia triggered by the intracellular uptake of NIR-photothermal transformable fluorescent Ppy–PEI NCs once NIR treatment. Interestingly, the prepared formulation with NIR transferring to local hyperthermia-caused biological response was suggested to increase ROS, which would be consistent with previous publications.Citation55,Citation56
Cell viability
Hyperthermic treatment noticeably improved the initiation effects of a locally elevated temperature on cellular apoptosis and involvement of intracellular ROS creation. The anticancer effects of this novel treatment might be closely related to the local temperature. Results suggest that hyperthermia therapy can be applied as a potential approach for cancer treatment.Citation57 As shown in , test cells treated with only NIR exhibited lower cytotoxicity as untreated control group (without NIR). After incubating with Ppy–PEI NCs, test cells exhibited relatively low cytotoxicity (above 80% metabolic activity of live cells). In the Ppy group, cells may show a poor adhesion on to hydrophobic and aggregated materials (Ppy), resulting that the low cytotoxic effect after washing with or without NIR treatment. However, the group that received Ppy–PEI NCs applied with NIR treatment displayed a cytotoxic effect compared to the control group. SEM examination was then used to elucidate the detailed morphological changes. Ppy–PEI NCs possibly were seen on the surface of H460 cell membranes, suggesting that receptors-mediated endocytosis would be occurred for subsequent intracellular trafficking (), and NIR provided a local hyperthermic effect. Even heterogenous shape of Ectosomes, the size of Ectosomes (100–1000 nm) would be possibly overlaped with the prepared nanocarrier (100–200 nm) so that the precisely identification way is needed in the future work.
Figure 5 (A) Quantitative results of MTT cell viability with different treatments, comparison of control and PEI-Ppy. (B) The group of Ppy–PEI NCs attached onto cell membranes morphologically imaged by SEM. (C) The cell viability was qualitatively tested with a Live/Dead method.
Note: *Statistically significant, P < 0.05.
Abbreviations: NC, nanocomplex; NIR, near-infrared; PEI, polyethylenimine; Ppy, polypyrrole; SEM, scanning electron microscopy.
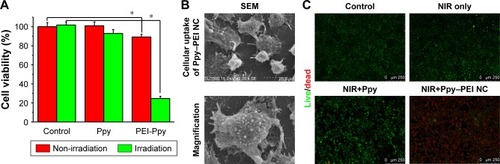
The further elucidation of live/dead cells using ethidium homodimer-1 and calcein AM showed a noteworthy difference in the indicator between dead and living cells. These fluorescent tracer dyes function as a powerful means to evaluate the cell death mechanism in high-content screening tests, as they can record numerous biological actions during the experiment. A high viability was detected with similar tendency observed in the untreated, NIR, and Ppy (with wash) groups. However, the group that received Ppy–PEI NCs and then noninvasive NIR treatment exhibited significant cytotoxicity compared to the other groups (). Thus, our study reveals a role for hyperthermia in vitro provided by the accumulated Ppy–PEI NCs with NIR treatment, which induced lung cancer cell death.
Conclusions
Ppy has been recognized as a versatile conductive biomaterial, which is widely utilized in biomedical applications and appears to have actual promise for progressive biotechnological bioapplications and is a widely used electroresponsive or photothermal material that might have the capability used to treat cancers. In this study, a Ppy–PEI NC was effectively made, and its fundamental features were explored using FTIR, TEM, SEM, and DLS methods. We also examined the lung cancer cell interaction with test Ppy–PEI NCs via fluorescent approaches. The photothermal features and cellular ROS with cytotoxicity were studied. These designed Ppy–PEI NCs were capable of interacting with cultured H460 lung cancer cells. The photothermal behavior, morphological cellular interactions, and cell ROS generation after treating NC/NIR were illustrated in . In future studies, this photothermal nanotechnology might be applied intratumor for treating lung cancer. The in vivo biodistribution, toxicity, and encapsulation of cancer medicine as combination therapy will be further investigated in the future.
Acknowledgments
This paper was supported by the government project from Ministry of Science and Technology (MOST) of Taiwan (106-2314-B-038-022-MY2).
Disclosure
The authors report no conflicts of interest in this work.
References
- WeissRBDonehowerRCWiernikPHHypersensitivity reactions from taxolJ Clin Oncol199087126312681972736
- GelderblomHVerweijJNooterKSparreboomACremophor EL: the drawbacks and advantages of vehicle selection for drug formulationEur J Cancer200137131590159811527683
- SparreboomAvan TellingenONooijenWJBeijnenJHDistributionTTissue distribution, metabolism and excretion of paclitaxel in miceAnticancer Drugs19967178868742102
- PolgárCMajorTCurrent status and perspectives of brachytherapy for breast cancerInt J Clin Oncol200914172419225919
- OdellDDKentMSFernandoHCSublobar resection with brachytherapy mesh for stage I non-small cell lung cancerSemin Thorac Cardiovasc Surg201022132720813314
- WolinskyJBColsonYLGrinstaffMWLocal drug delivery strategies for cancer treatment: gels, nanoparticles, polymeric films, rods, and wafersJ Control Release20121591142622154931
- PrabhuRHPatravaleVBJoshiMDPolymeric nanoparticles for targeted treatment in oncology: current insightsInt J Nanomedicine201510100125678788
- VeisehOKievitFMGunnJWRatnerBDZhangMA ligand-mediated nanovector for targeted gene delivery and transfection in cancer cellsBiomaterials200930464965718990439
- ParkCLeeCKwonOConducting Polymer Based NanobiosensorsPolymers201687249
- LeeJWSernaFNickelsJSchmidtCECarboxylic acid-functionalized conductive polypyrrole as a bioactive platform for cell adhesionBiomacromolecules2006761692169516768385
- LeeJWSernaFSchmidtCECarboxy-endcapped conductive polypyr-role: biomimetic conducting polymer for cell scaffolds and electrodesLangmuir200622249816981917106966
- WangCXuHLiangCIron oxide @ polypyrrole nanoparticles as a multifunctional drug carrier for remotely controlled cancer therapy with synergistic antitumor effectACS Nano2013786782679523822176
- FanJHeNHeQA novel self-assembled sandwich nano-medicine for NIR-responsive release of NONanoscale2015747200552006226568270
- ZhaoRSunXSunJWangLHanJPolypyrrole-modified CuS nanoprisms for efficient near-infrared photothermal therapyRSC Adv20177171014310149
- ManivasaganPQuang BuiNBharathirajaSMultifunctional biocompatible chitosan-polypyrrole nanocomposites as novel agents for photoacoustic imaging-guided photothermal ablation of cancerSci Rep201774359328252638
- ChenFCaiWNanomedicine for targeted photothermal cancer therapy: where are we now?Nanomedicine20151011325597770
- DallasPNiarchosDVrbanicDInterfacial polymerization of pyrrole and in situ synthesis of polypyrrole/silver nanocompositesPolymer200748720072013
- SongCWLokshinaARheeJGPattenMLevittSHImplication of blood flow in hyperthermic treatment of tumorsIEEE Trans Biomed Eng19843119166724614
- HarrisKPuchalskiJStermanDRecent advances in bronchoscopic treatment of peripheral lung cancersChest2017151367468527292045
- HuoSMaHHuangKSuperior penetration and retention behavior of 50 nm gold nanoparticles in tumorsCancer Res201373131933023074284
- WangJJZengZWXiaoRZRecent advances of chitosan nanoparticles as drug carriersInt J Nanomedicine2011676521589644
- HouCHLinFLHouSMLiuJFHyperthermia induces apoptosis through endoplasmic reticulum and reactive oxygen species in human osteosarcoma cellsInt J Mol Sci20141510173801739525268613
- BirbenESahinerUMSackesenCErzurumSKalayciOOxidative stress and antioxidant defenseWorld Allergy Organ J20125191923268465
- Pham-HuyLAHeHPham-HuyCFree radicals, antioxidants in disease and healthInt J Biomed Sci2008428923675073
- MiFLBurnoufTLuSYFlMSyLSelf-targeting, immune transparent plasma protein coated nanocomplex for noninvasive photo-thermal anticancer therapyAdv Healthc Mater20176141700181
- LuKYLinPYChuangEYH2O2-Depleting and O2-Generating Selenium Nanoparticles for Fluorescence Imaging and Photodynamic Treatment of Proinflammatory-Activated MacrophagesACS Appl Mater Interfaces2017965158517228120612
- DowlingAPNanotechnologies DofMaterials Today20047123035
- LiJYoonSJHsiehBYTaiWO’DonnellMGaoXStably doped conducting polymer nanoshells by surface initiated polymerizationNano Lett201515128217822226588215
- LiJArnalBWeiCWMagneto-optical nanoparticles for cyclic magnetomotive photoacoustic imagingACS Nano2015921964197625658655
- RavichandranRSundarrajanSVenugopalJRMukherjeeSRamakrishnaSApplications of conducting polymers and their issues in biomedical engineeringJ R Soc Interface20107Suppl 5S559S57920610422
- XiongGMYapYZChoongCSingle-step synthesis of heparin-doped polypyrrole nanoparticles for delivery of angiogenic factorNanomedicine201611774976526980324
- de OliveiraHPdos SantosMVBdos SantosCGde MeloCPPreparation and electrical and dielectric characterization of PVA/PPY blendsMater Charact2003502–3223226
- HuangJHuXLuLYeZZhangQLuoZElectrical regulation of Schwann cells using conductive polypyrrole/chitosan polymersJ Biomed Mater Res A201093116417419536828
- RacOSuchorska-WoźniakPFiedotMTeteryczHInfluence of stabilising agents and pH on the size of SnO2 nanoparticlesBeilstein J Nanotechnol201452192220125551047
- GhalibHAbdullahIDaikRElectrical conductivity of anionic surfactant-doped polypyrrole nanoparticles prepared via emulsion polymerizationSci Technol2013212459471
- LiX-G LAHuangM-RLiaoYY-GLEfficient and scalable synthesis of pure polypyrrole nanoparticles applicable for advanced nanocomposites and carbon nanoparticlesThe Journal of Physical Chemistry C2010114451924419255
- QinZWangYRandrianalisoaJQuantitative Comparison of Photothermal Heat Generation between Gold Nanospheres and NanorodsSci Rep201662983627445172
- NeedhamDAnyarambhatlaGKongGDewhirstMWA new temperature-sensitive liposome for use with mild hyperthermia: characterization and testing in a human tumor xenograft modelCancer Res20006051197120110728674
- PabingerIPoschFFlamethrowers: blood cells and cancer thrombosis riskHematology Am Soc Hematol Educ Program20142014141041725696887
- ChenBLeWWangYTargeting Negative Surface Charges of Cancer Cells by Multifunctional NanoprobesTheranostics20166111887189827570558
- AdamkoDJWuYAjamianFIlarrazaRMoqbelRGleichGJThe effect of cationic charge on release of eosinophil mediatorsJ Allergy Clin Immunol2008122238339018455220
- OhNParkJHEndocytosis and exocytosis of nanoparticles in mammalian cellsInt J Nanomedicine20149Suppl 15124872703
- ShiDCancer cell surface negative charges: a bio-physical manifestation of the warburg effectNano Life201707177100103n041771001
- AchourBWuQAdvances in Energy and Environment Research: Proceedings of the International Conference on Advances in Energy and Environment ResearchCRC Press
- ZaikovGETrends in molecular and high molecular scienceNovaPublishers2005
- BaoZLiuXLiuYLiuHZhaoKNear-infrared light-responsive inorganic nanomaterials for photothermal therapyAsian J Pharm Sci2016113349364
- EmanuelNNMBuchachenkoALChemical Physics of Polymer Degradation and Stabilization1UtrechtVSP1987
- TianQTangMSunYHydrophilic flower-like CuS superstructures as an efficient 980 nm laser-driven photothermal agent for ablation of cancer cellsAdv Mater201123313542354721735487
- YameenBChoiWIVilosCSwamiAShiJFarokhzadOCInsight into nanoparticle cellular uptake and intracellular targetingJ Control Release201419048549924984011
- TanMLChoongPFDassCRCancerDCRCancer, chitosan nanoparticles and catalytic nucleic acidsJ Pharm Pharmacol200961131219126291
- van der AaMAHuthUSHäfeleSYCellular uptake of cationic polymer-DNA complexes via caveolae plays a pivotal role in gene transfection in COS-7 cellsPharm Res20072481590159817385010
- ZhangYZhangBTRAIL resistance of breast cancer cells is associated with constitutive endocytosis of death receptors 4 and 5Mol Cancer Res20086121861187119074831
- FröhlichEThe role of surface charge in cellular uptake and cytotoxicity of medical nanoparticlesInt J Nanomedicine20127557723144561
- QiuLChenTÖçsoyIÖçsoyIA cell-targeted, size-photocontrollable, nuclear-uptake nanodrug delivery system for drug-resistant cancer therapyNano Lett201515145746325479133
- VolkovRAPanchukIIMullineauxPMSchöfflFHeat stress-induced H(2)O (2) is required for effective expression of heat shock genes in ArabidopsisPlant Mol Biol2006614–573374616897488
- LiFJKondoTZhaoQLEnhancement of hyperthermia-induced apoptosis by a free radical initiator, 2,2′-azobis (2-amidinopropane) dihydrochloride, in human histiocytic lymphoma U937 cellsFree Radic Res200135328129911697127
- LukKHHulseRMPhillipsTLHyperthermia in cancer therapyWest J Med198013231797376656