Abstract
Perfluorooctylbromide nanoparticles (PFOB NPs) are a type of multifunctional nanotechnology that has been studied for various medical applications. Commercial ultrasound contrast agents (UCAs) suffer from the following limitations: short half-lives in vivo, high background signal and restricted distribution in the vascular circulation due to their micrometer dimensions. PFOB NPs are new potential UCAs that persist for long periods in the circulatory system, possess a relatively stable echogenic response without increasing the background signal and exhibit lower acoustic attenuation than commercial UCAs. Furthermore, PFOB NPs may also serve as drug delivery vehicles in which drugs are dissolved in the outer lipid or polymer layer for subsequent delivery to target sites in site-targeted therapy. The use of PFOB NPs as carriers has the potential advantage of selectively delivering payloads to the target site while improving visualization of the site using ultrasound (US) imaging. Unfortunately, the application of PFOB NPs to the field of ultrasonography has been limited because of the low intensity of US reflection. Numerous researchers have realized the potential use of PFOB NPs as UCAs and thus have developed alternative approaches to apply PFOB NPs in ultrasonography. In this article, we review the latest approaches for using PFOB NPs to enhance US imaging in vivo. In addition, this article emphasizes the application of PFOB NPs as promising drug delivery carriers for cancer and atherosclerosis treatments, as PFOB NPs can transport different drug payloads for various applications with good efficacy. We also note the challenges and future study directions for the application of PFOB NPs as both a delivery system for therapeutic agents and a diagnostic agent for ultrasonography.
Introduction
Perfluorooctylbromide (C8F17Br, PFOB; ), which is sometimes called as perflubron, is a linear perfluorocarbon (PFC) well known for its biocompatibility and good tolerance in human beings.Citation1 PFOB is a dense, biochemically inert liquid with a high spreading coefficient. Unlike most PFCs, it is highly hydrophobic and shows small but finite lipophilicity due to the covalently bound bromine atom.Citation2–Citation5 Following in vivo administration, PFOB is not metabolized and instead is eliminated as a vapor from the body through exhalation via the lungs with a 3-day biological half-life.Citation6
Figure 1 The structure of PFOB along with all atoms (red, bromine; yellow, carbon; green, fluorine).
Abbreviation: PFOB, perfluorooctylbromide.
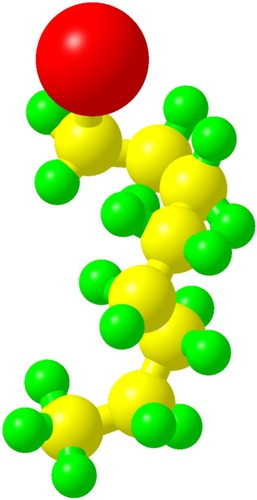
Nanoparticles (NPs), particularly polymer-based NPs, offer several advantages for anticancer drug/gene delivery.Citation7,Citation8 The small volume enables their progressive accumulation in the interstitial space of tumors through the enhanced permeation and retention (EPR) effect and endows them with a long circulation half-life.Citation9 For in vivo ultrasound (US) imaging and drug delivery, PFOB is typically prepared as perfluorooctylbromide nanoparticles (PFOB NPs), which consist of a PFOB core encapsulated within a monolayer of phospholipids or a polymeric shell. PFOB emulsions used in the clinic are marketed as LiquiVent (Alliance Pharmaceutical Corporation, San Diego, CA, USA),Citation10 an oxygen-carrying liquid drug, and Oxygent™ (Alliance Pharmaceutical Corporation, San Diego, CA, USA),Citation11 a blood substitution agent. In addition, the use of PFOB NPs in various medical applications, including 19F or 1H magnetic resonance imaging (MRI) tracers,Citation12–Citation19 cell-tracking agents,Citation20–Citation22 X-ray contrast agentsCitation21,Citation23 and computed tomography (CT) tracers, has been explored.Citation24
US imaging is a clinical diagnostic technique that is frequently used because of its advantages, namely its real-time monitoring capability, low cost, high safety, convenience and portability.Citation25 To improve the visualization in US imaging, US contrast agents (UCAs) have been developed. Mattrey et alCitation26 reported that PFOB NPs considerably increased the echogenicity of the liver relative to that of the kidney 48 hours after intravenous infusion and produced an echogenic rim around VX2 carcinoma, thereby enhancing tumor detection. Therefore, the authors concluded that PFOB represented a promising US contrast material. Lanza et alCitation27 reported that liquid PFC-filled NPs could potentially be used as new UCAs because of their long circulation half-life and acoustic stability. Since then, PFOB, one of the PFCs most frequently used as an NP core,Citation28 has attracted the interest of researchers. PFOB NPs present a low intensity of US reflection and require higher concentrations or more binding events, which are the physical bases of the ability of PFOB NPs to serve as UCAs, to produce a relatively high backscatter signal.Citation29,Citation30 Because of its poor solubility in water and very low speed of sound(approximately 600 m/s), PFOB persists in the circulatory system for long periods and displays a relatively stable echogenic response.Citation31 Consequently, PFOB NPs are designed for therapeutic applications, such as drug delivery or gene transfer, and for potential use as UCAs. The use of PFOB NPs as carriers has the potential advantage of selectively delivering payloads of therapeutic agents to the site of interest while improving the visualization of the site by US imaging. Nevertheless, an important question remains: Does this process really work? This review will focus on the use of PFOB NPs for US imaging and drug delivery in diagnosing and treating various diseases to determine the challenges in and provide future perspectives of the applications of PFOB NPs in targeted therapeutic delivery coupled with targeted US imaging.
Structures of PFOB NPs
For in vivo US imaging and drug delivery, PFOB is typically prepared as PFOB NPs with a surfactant coating for stabilization and functionalization (). Typical PFOB NPs contain a lipid–surfactant mixture encapsulating a liquid core of PFOB using an oil-in-water emulsion solvent evaporation process.Citation32–Citation34 The surfactant mixture consists of phospholipids typically derived from either egg or soybean lecithin, which display good biocompatibility. These phospholipid preparations have been used for many applications such as cosmetic, food and drug applications owing to their safety.Citation35 Thus, the final formulated PFOB NPs mainly comprise a PFOB core encapsulated within a lipid monolayer with a diameter <1,000 nm.Citation36 Other typical PFOB NPs consist of poly(lactide-co-glycolide) acid (PLGA),Citation37,Citation38 poly(lactic acid) (PLA)Citation39 or another polymerCitation26,Citation40 encapsulating a liquid PFOB core prepared using an oil-in-water emulsion solvent evaporation process.Citation41 These polymers are biodegradable, are widely used in drug products and possess good general biocompatibility.Citation42,Citation43 An amphiphilic block copolymer, poly(ethylene glycol)-b-poly(d,l-lactic acid) (PEG-PDLLA), was developed to construct novel self-assembled PFOB NPs with a low content of PFOB in the outer lipophilic layer.Citation44 This self-assembled structure has mainly been studied as a UCA and has been established as a type of PFOB NP. The particle shell formulations and main applications, which are described in the text, are summarized in . Schematics showing the structures of PFOB NPs with different shells functionalized by different ligands are shown in .
Table 1 Broad applications of PFOB NPs
Figure 2 Schematic of the structures of PFOB NPs with different shells functionalized by different ligands.
Notes: (A) Representative images of typical PFOB NPs with a lipid shell for functionalization, representing an extremely versatile platform for targeted US/pool imaging and drug delivery applications. PFOB NPs carry drugs in the lipid layer of their shell. FA and Arg–Gly–Asp are used for ligand-directed, tissue-targeted US imaging. The targeting peptides Arg–Gly–Asp, a cyclic RGD peptide, angiopep-2 and sulfatide are used for ligand-directed tissue-targeted drug delivery. (B) Representative images of other typical PFOB NPs with a polymer shell for functionalization, representing an extremely versatile platform for US pool imaging and drug delivery applications. PFOB NPs carry drugs in the polymer layer of their shell or in the center of the PFOB NPs. FA and angiopep-2 are used for ligand-directed tissue-targeted drug delivery. (C) Representative images of novel self-assembled PFOB NPs with a low PFOB content in the outer lipophilic layer, representing a platform for US pool imaging.
Abbreviations: FA, folic acid; Gd-DTPA, gadolinium diethylene triamine pentacetate acid; GO, graphene oxide; PEG-PDLLA, poly(ethylene glycol)-b-poly(d,l-lactic acid); PFOB, perfluoroo ctylbromide; PFOB NPs, perfluorooctylbromide nanoparticles; US, ultrasound.
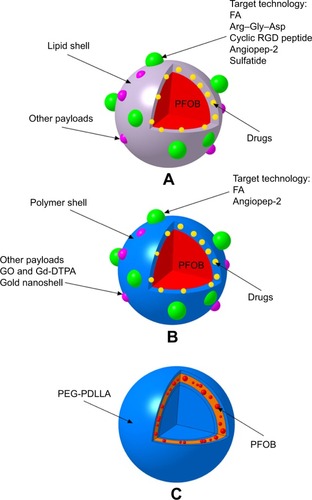
PFOB NPs for US imaging
Commercial UCAs (eg, Optison™ (Mallinckrodt Inc., St. Louis, MO, USA) and Definity® (Lantheus Medical Imaging, N. Billerica, MA, USA)Citation46 comprise encapsulated gas microbubbles with a diameter between approximately 2 and 10 μm. Upon intravenous injection, these UCAs are restricted to the vascular system of perfused organs or tissues. Despite the very sensitive detection offered by the strong nonlinear response of microbubbles, these agents present several limitations due to their short half-lives in vivo and high background signal. Because of their micrometer dimensions, their transportation in the vascular circulation is restricted to only a few minutes, and they cannot traverse outside highly permeable tumor vessels with pores ranging from 400 to 600 nm in diameter to achieve tumor imaging.Citation47 Compared with microbubbles, NPs offer several advantages in targeted US imaging. Their small volume contributes to a long circulation half-life and enables their progressive accumulation in the interstitial space of tumors through the enhanced EPR.Citation9 These NPs have primarily been designed to address key issues, such as longevity and stability in the body.
PFOB was encapsulated within a Pluronic F-68 shell with a diameter of approximately 500 nm by Mattrey et al.Citation26 The resulting PFOB NPs considerably increased the echogenicity of the liver relative to that of the kidney 48 hours after intravenous infusion and produced an echogenic rim around VX2 carcinoma in New Zealand white rabbits, thereby enhancing tumor detection. Therefore, the authors concluded that PFOB NPs represent a promising UCA for selectively increasing detection in the liver and in tumors.Citation26 According to Lanza et al,Citation27 targeted perfluorodichlorooctane (PFDCO)-filled NPs (PFDCO NPs) markedly enhance the acoustic reflectivity of thrombi in vivo in a canine model. Targeted PFDCO NPs persist in the blood for a long period, specifically bind to pre-targeted fibrin and generate a marked acoustical enhancement of acute vascular thrombi without increasing the background signal. In their follow-up study, the authors reported that both targeted PFOB NPs and targeted PFDCO NPs significantly increased the acoustic reflectivity of the nitrocellulose membrane samples and plasma thrombus substrate samples compared with their individual baseline values. Targeted PFOB NPs and targeted PFDCO NPs enhanced reflectivity on both nitrocellulose membrane and plasma thrombus substrates to nearly identical levels.Citation29 Thus, US imaging with site-targeted PFOB NPs is expected to improve the diagnosis and detection of specific pathologies and tissue types, such as fibrin, as described earlier. PFOB NPs, which are resistant to pressure changes and mechanical stress, possess a relatively stable echogenic response without increasing the background signal, exhibit low acoustic attenuation and have received substantial attention with regard to fabricating a new UCA that can overcome the limitations of microbubble UCAs. Unfortunately, PFOB NPs are relatively incompressible and thus generally produce relatively poor echogenicityCitation27,Citation30,Citation48 in circulation. Thus, the low intensity of US reflection has limited the application of PFOB NPs in the field of ultrasonography. Therefore, how to overcome the limitations of PFOB NPs in ultrasonography remains an open question. Accordingly, different researchers have developed alternative approaches to apply PFOB NPs in ultrasonography.
Adaptation of ultrasonography parameters for blood pool imaging
Some researchers have adapted ultrasonography parameters for blood pool imaging. High-frequency US (HFU), also known as US biomicroscopy,Citation49 is an established tool for imaging human beings and small animals in vivo.Citation50 Some researchers have reported the feasibility of detecting thick PLGA-shelled PFOB NPs at a high frequency and explored the ranges of concentrations, acoustic pressures and pulse durations capable of detecting the PLGA-shelled PFOB NPs in the 20–40 MHz frequency range.Citation28 Although the detectability of PFOB NPs would potentially be improved by HFU (>15 MHz), the acoustic responses remain relatively weak.Citation28 PFOB NPs exhibit a low inherent echogenicity and are poor blood pool contrast agents when employed under the conditions used for conventional two-dimensional (2D) echocardiography or harmonic imaging or when imaged with color flow or spectral Doppler.Citation30 Thus, a new ultrasonic imaging modality, power Doppler harmonic imaging (PDHI), has been introduced.Citation51 Wickline et alCitation30 have shown that PFOB NPs coated with a lipid shell with a mean diameter of approximately 400 nm provide excellent blood pool contrast when using PDHI at PFOB NP doses of 0.5 mL/kg. The contrast effect was unaffected by continuous US imaging at high transducer power outputs and persisted for more than 1 hour.Citation30 Li et alCitation52 intravenously injected New Zealand white rabbits with PFOB NPs with a PLA shell for US imaging of their kidneys using both pulse inversion harmonic imaging (PIHI) mode and conventional B-mode. The rabbit kidney was more clearly observed using PIHI mode than using B-mode US imaging. These results were consistent with the abovementioned observations.
Adaptation of the formulations designed for blood pool imaging
The mechanical properties of PFOB NPs are very important for their use as UCAs because USs are mechanical waves.Citation53 In the classical mechanics of shells, the mechanical properties usually depend on the shell thickness-to-radius ratio. Pisani et alCitation53 concluded that the shell thickness-to-radius ratio depends only on the PFOB/PLGA ratio and that this versatile process could be adapted to other biodegradable polymers. In their follow-up study,Citation41 the authors designed a process to obtain PFOB NPs within a PLGA shell of homogeneous thickness to develop more stable UCAs. Flegg et alCitation40 applied a novel modified theory based on Rayleigh scattering of US to a UCA consisting of PFOB encapsulated in polycaprolactone (PCL) and concluded that the shell of the PFOB NPs may play important roles in the scattering from each PFOB NP and the echogenicity of an agglomeration of PFOB NPs. Because the modulation of the thickness-to-radius ratio of PLGA and PFOB allows researchers to tune the compressibility and echogenicity of PFOB NPs in vitro, Jafari et alCitation28 compared the US responses of PFOB NPs with a PLGA shell of different thicknesses. Diou et alCitation54 also utilized the very same strategy with PEGylated PFOB NPs with a PLGA shell to optimize their echogenicity. The results are consistent with a previous report by Pisani et alCitation53 showing that the thickness of the PFOB NP shell is an important parameter for US contrast enhancement. However, the acoustic responses remain relatively weak, and additional experiments are necessary to further modify PFOB NPs and obtain a better acoustic response.Citation28
Consequently, subsequent studies have adapted formulations designed for blood pool imaging but have met with limited success in vivo.Citation55–Citation57 Meanwhile, several researchers have successfully designed PFOB NPs for use in blood pool imaging.
According to Pisani et al,Citation9 the thickness of the PFOB NP shell is an important parameter for US contrast enhancement: thinner shells produce a larger signal-to-noise ratio (SNR) and more compressible PFOB NPs. These authors have designed PFOB NPs with a thick PLGA shell as a blood pool contrast agent. The initial bolus passage enables significant US enhancement of the blood pool in mice during hepatic imaging (14 MHz, tissue harmonic imaging [THI] mode) after intravenous injection.Citation9 Similar multifunctional UCAs featuring gold nanoshells coating the outside of PFOB NPs with a PLA shell have been designed by Ke et alCitation58 and integrated with superparamagnetic iron oxide (SPIO) dissolved in PFOB for combined dual-mode US/CT imaging-guided photothermal tumor ablation. Their platform is different from the PFOB NPs developed by our laboratory or by other researchers, which presented a spherical shape with a smooth surface (), while their PFOB NPs with the gold nanoshell coating presented a rather rough surface morphology (). Several seconds after intravenous injection of their PFOB NPs, the rabbit kidneys showed clear enhancement under the PIHI contrast mode (mechanical index [MI] of 0.70). However, the authors believed that the US contrast enhancement was provided by the PFOB inside and that SPIO was used to obtain more accurate diagnostic information from contrast-enhanced MRI images. We are skeptical about this hypothesis. SPIO has also been utilized as a US imaging material in many studies.Citation25,Citation59,Citation60 Therefore, the US enhancement may be caused by the SPIO, not the PFOB, in their formulation. In the same year, Ke et alCitation39 also designed another multifunctional UCA using a gold nanoshell coating the outside of PFOB NPs with a PLA shell for combined dual-mode US/CT imaging and photothermal cancer therapy. The rabbit kidney showed clear enhancement in PIHI contrast mode (at an MI of 0.59) after intravenous injection of their PFOB NPs.Citation39 A novel multifunctional theranostic UCA using PFOB NPs with a PLA shell surface functionalized with graphene oxide (GO) and a gadolinium diethylene triamine pentacetate acid (Gd-DTPA) was successfully fabricated by Li et al.Citation52 Again, the rabbit kidney showed clear enhancement in PIHI contrast mode (at an MI of 0.42) after intravenous injection of their PFOB NPs.Citation52 Other novel PFOB NPs with an extremely low PFOB content were prepared through the self-assembly of an amphiphilic block copolymer, PEG-PDLLA (). After intravenous injection of these PFOB NPs, all cavities of the heart, liver and kidney, particularly the horizontal axis of the right kidney, displayed a slight and homogeneous enhancement without any highlighted spots, which indicated a good contrast enhancement effect for blood pool imaging. The authors believed that both the compressibility and shell density of their PFOB NPs serving as US scatterers were enhanced, resulting in a much higher echo intensity than that of other PFOB formulations.Citation44
Figure 3 TEM images of normal PFOB NPs (A). TEM images of PFOB NPs coated with a gold nanoshell (B).
Notes: (A) Reprinted from Li X, Qin F, Yang L, Mo L, Li L, Hou L. Sulfatide-containing lipid perfluorooctylbromide nanoparticles as paclitaxel vehicles targeting breast carcinoma. Int J Nanomedicine. 2014;9:3971–3985.Citation33 (B) Reprinted from the study by Ke H, Wang J, Tong S, Jin Y, Wang S, Qu E, Bao G, Dai Z. Gold Nanoshelled Liquid Perfluorocarbon Magnetic Nanocapsules: a Nanotheranostic Platform for Bimodal Ultrasound/Magnetic Resonance Imaging Guided Photothermal Tumor Ablation. Theranostics 2014;4(1):12–23. doi:10.7150/thno.7275. Available from http://www.thno.org/v04p0012.htm.Citation58
Abbreviations: PFOB NPs, perfluorooctylbromide nanoparticles; TEM, transmission electron microscope.
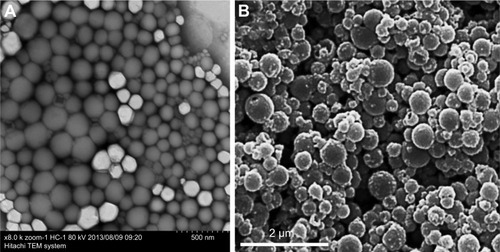
Fabrication of targeted PFOB NPs for tissue-targeted imaging
PFOB NPs may be less liable to nonspecific signal enhancement events since larger concentrations (more binding events) are required to produce a relatively high echogenicity.Citation29,Citation61 Thus, a high contrast between specific and nonspecific agents may be obtained by adding a targeted ligand to PFOB NPs. Although nanosized UCAs achieve passive imaging of tumors via the EPR effect, the specific capability of nanosized UCAs to bind to tumor tissues to achieve US enhancement is still limited.Citation62 Some investigators have recognized the future clinical importance of PFOB NPs in tumor-targeted US imaging and developed targeted PFOB NPs to enhance US imaging; however, this objective was realized only in vitro.Citation61 Subsequently, PFOB NPs targeted to αvβ3 integrins expressed on new vessels in tumors were formulated by adding an “Arg–Gly–Asp” mimetic binding ligand to the lipid layer.Citation63 Tumor-specific enhanced imaging was accomplished by either If (1.99) or Hf receivers (different receivers of ultrasonography) in transgenic K14-HPV16 mice whose ears typically carried squamous metaplasia after intravenous injection of these targeted PFOB NPs.Citation63 Another laboratory modified the surface of PFOB NPs with PEGylated phospholipids to allow them to escape recognition and clearance from the mononuclear phagocyte system and achieve passive tumor targeting. In this study, tumors were observed by ultrasonography only after intratumoral injection. To explain the absence of an echogenic signal in the tumor after intravenous injection of these PEGylated PFOB NPs, a histological analysis was conducted, revealing their limited accumulation within the tumor tissue at a level that was not sufficient to achieve US imaging enhancement.Citation64 The folate receptor (FR) binds to folic acid (FA) with high affinity and mediates its intracellular transport via receptor-mediated endocytosis.Citation65 The FR is overexpressed on the surface of various types of tumors, including pancreatic, prostate, lung, head and neck, breast, ovarian and mesothelioma tumors, whereas it shows limited expression and restricted distribution in normal tissue.Citation66–Citation70 Hu et alCitation71 bound an FA–polyethylene glycol (PEG)–chitosan (CS) conjugate to PFOB NPs through electrostatic interactions, promoting the formation of new FR-mediated PFOB NPs using a layer-by-layer assembly technique. The US signals of the NPs in vitro increased as the concentration of the FR-mediated PFOB NPs increased. Based on the experimental results, the authors predicted that the FR-mediated PFOB NPs are more promising agents for tumor-targeted US imaging.Citation71 In a subsequent study,Citation72 the researchers injected their FR-mediated PFOB NPs along with an immobilized probe into mice via the tail vein and set the ultrasonography parameters to an MI of 0.7 and a gain of 80%. Between 20 and 160 minutes after injection, the images from FR-overexpressing tumors displayed a higher intensity value than those from tumors expressing low levels of FR. Thus, the FR-mediated PFOB NPs achieved specific enhanced imaging of FR-overexpressing tumors and a longer duration of effective enhanced US imaging.Citation72 The abovementioned alternative approaches to applying PFOB NPs in ultrasonography are summarized in .
Table 2 Alternative approaches for applying PFOB NPs to ultrasonography in vivo
PFOB NPs for drug delivery
PFOB NPs have been formulated to adhere to the surface of a target and produce highly concentrated zones.Citation61 These targeted imaging effects may persist in the body from several minutes to possibly several days.Citation73 Therefore, a new generation of PFOB NPs as stable UCAs designed for therapeutic applications such as drug delivery or gene transfer has become available.Citation53 With the attachment of targeting ligands to the PFOB NP surface, PFOB NPs have been specifically directed to bind biomarkers of cancer, angiogenesis, thrombosis and other diseases.Citation74 Furthermore, targeting PFOB NPs to the tissue of interest also localizes drug release to the target site, resulting in a much higher effective drug concentration at that site. In addition, PFOB NPs may function as an efficacious drug delivery vehicle because their safety has been approved by the Food and Drug Administration (FDA).Citation75 PFOB NPs may serve as drug delivery vehicles in which drugs are dissolved in the outer lipid or polymer layer for subsequent delivery to target cells for site-targeted therapy. Therefore, many researchers have designed PFOB NPs with a drug or geneCitation76 payload as effective systems for delivery to a targeted site. In contrast to liposomal drug delivery, which generally requires endocytosis, the mechanism by which targeted PFOB NPs with a lipid-coating transport drugs involves lipid exchange or lipid mixing between the lipid monolayer of the delivery system and the targeted cell membrane, termed “contact-facilitated drug delivery.”Citation77–Citation80 PFOB NPs have been loaded with different drugs for various applications, such as anti-inflammatory arthritis therapy,Citation32,Citation81,Citation82 bone fracture healing,Citation83,Citation84 dust mite-triggered asthma therapy,Citation85 cytotoxicity reduction in the loaded drug,Citation86 analyses of complement activation,Citation87 thrombolytic therapy,Citation88,Citation89 prevention of restenosis,Citation77,Citation90 atherosclerosis therapy and cancer therapy. The capacity to deliver a payload to the targeted site could be of great benefit, leading to the development of targeted anticancer and anti-atherosclerosis therapeutics based on this platform.
Drug delivery for cancer therapy
Although a number of anticancer drugs have been tested clinically, either their low uptake efficacy at the target or systemic toxicity has limited their therapeutic value.Citation91–Citation93 A satisfactory delivery vehicle can facilitate improved pharmacokinetics and biodistribution, decreased toxicities, improved solubility and stability and controlled release.Citation94,Citation95 PFOB NPs represent a potential suitable delivery vehicle, and surface functionalization of these NPs may improve the tolerability, site-specific delivery and controlled release of therapeutic agents.Citation33
Soman et alCitation96 incorporated melittin into the outer lipid monolayer of PFOB NPs. The favorable pharmacokinetics of PFOB NPs allow melittin to accumulate in murine tumors in vivo and produce an obvious reduction in tumor growth without any apparent toxicity compared with melittin alone.Citation96 Vascular cell adhesion molecule 1 (VCAM-1), which is expressed in tumor and atherosclerotic vessels, plays a pivotal role in the course of many inflammatory diseases.Citation97–Citation101 VCAM-1-targeted PFOB NPs were generated by Pan et alCitation99 for in vivo targeting in breast cancer (STAT-1-deficient) models. The authors observed a 4.9-fold increase in the number of targeted PFOB NPs in the tumor vasculature compared with nontargeted PFOB NPs, indicating that VCAM-1-targeted PFOB NPs may function as an ideal breast cancer-targeted delivery system for certain anticancer drugs.Citation99 Sulfatide is a ligand of several extracellular matrix (ECM) glycoproteins, particularly tenascin-C (TN-C), which is highly upregulated in many different cancers, including breast, ovarian and prostate cancer.Citation102,Citation103 Sulfatide, a type of lipid, actively incorporates with other lipids in PFOB NPs and functions as part of the outer lipid monolayer component of the targeted ligand.Citation33 We added sulfatide to PFOB NPs as a targeted delivery system for loading paclitaxel (PTX) to treat breast carcinoma. These sulfatide-targeted PFOB NPs were observed in breast cancer tissues, facilitated the delivery of the drug to the targeted breast cancer tumor () and improved the therapeutic outcomes of PTX in a mouse model ().Citation33 Pan et alCitation104 incorporated a c-Myc inhibitor prodrug into αvβ3-targeted PFOB NPs and reported that the formulation displayed good efficacy in preventing melanoma. Their laboratory subsequently showed that their αvβ3-targeted PFOB NPs loaded with a c-Myc inhibitor prodrug extended the survival time in a mouse model of disseminated multiple myeloma.Citation105 Song et alCitation106 designed PFOB NPs loaded with hollow Bi2Se3 for the timely supply of oxygen under near-infrared irradiation to enhance cancer radiotherapy with good effectiveness. Vu-Quang et alCitation17 designed multifunctional FA-targeted PFOB NPs loaded with doxorubicin in a PLGA shell and showed their good anticancer efficacy in vitro. PEGylated PFOB NPs loaded with PTX, which was dispersed in the PLGA shell of the PFOB NPs, were designed by Boissenot et alCitation107 and produced a promising and statistically significant twofold reduction in CT-26 tumor growth compared with generic Taxol® (Bristol-Myers Squibb Company, Princeton, NY, USA). Angiopep-2, which is known to bind to low-density lipoprotein receptor-related protein-1 (LRP-1), is an ideal dual-targeting moiety for a brain tumor tissue-targeted delivery system that also targets glioblastoma cells.Citation108–Citation111 A novel high-intensity focused US (HIFU)-responsive angiopep-2-targeted PFOB NP drug delivery system with a PLGA shell containing doxorubicin was developed by Luo et alCitation112 to enhance the targeted therapy of glioblastoma. The rate of drug delivery was greatly increased by the application of HIFU irradiation, which enabled the PFOB NP drug delivery system to achieve the strongest efficacy against glioblastoma.Citation112 Interestingly, the locations of the drugs (PTX or doxorubicin) in the PFOB NPs with a polymer shell for cancer therapy developed by Vu-Quang et al,Citation17 Boissenot et alCitation107 and Luo et alCitation112 were ambiguous (). Vu-Quang et alCitation17 and Boissenot et alCitation107 reported that the loaded drug was located in the polymer shell (), whereas Luo et alCitation112 concluded that the doxorubicin used in their study was located in the center of the NPs along with PFOB (). For drug delivery, more studies have focused on PFOB NPs with a lipid coating than on PFOB NPs with a polymer coating. Thus, the location of the drugs in PFOB NPs with a polymer shell should be verified in further studies.
Figure 4 Images of the tissue distribution of PTX in EMT6 tumor-bearing mice obtained at 1 hour (A), 8 hours (B), 12 hours (C) and 24 hours (D) after intravenous administration of different PTX formulations. Values are presented as mean ± SD (n=3). *P<0.05, **P<0.01. (E) Representative images of the fluorescence of different coumarin-6 formulations in frozen slices of breast tumor tissues 1 hour after administration. (F) Representative images of the fluorescence of different coumarin-6 formulations in frozen slices of breast tumor tissue 8 hours after administration. Representative images of tumor sections were obtained at 200× magnification. PTX-NPs, PFOB NPs loaded with PTX; PTX-SNPs, sulfatide-targeted PFOB NPs loaded with PTX; coumarin-6-NPs, PFOB NPs loaded with coumarin-6; coumarin-6-SNPs, sulfatide-targeted PFOB NPs loaded with coumarin-6.
Note: Reprinted from Li X, Qin F, Yang L, Mo L, Li L, Hou L. Sulfatide-containing lipid perfluorooctylbromide nanoparticles as paclitaxel vehicles targeting breast carcinoma. Int J Nanomedicine. 2014;9:3971–3985.Citation33
Abbreviations: PFOB NPs, perfluorooctylbromide nanoparticles; PTX, paclitaxel; SNPs, sulfatide-targeted perfluorooctylbromide nanoparticles.
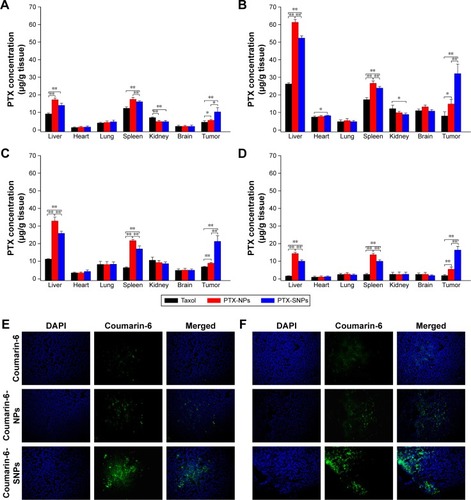
Figure 5 Tumor growth curves of EMT6 tumor-bearing mice after the administration of different PTX formulations.
Notes: Values are presented as mean ± SD (n=6). PTX-NPs, PFOB NPs loaded with PTX; PTX-SNPs, sulfatide-targeted PFOB NPs loaded with PTX; blank SNPs, sulfatide-targeted PFOB NPs. **p<0.01. Reprinted from Li X, Qin F, Yang L, Mo L, Li L, Hou L. Sulfatide-containing lipid perfluorooctylbromide nanoparticles as paclitaxel vehicles targeting breast carcinoma. Int J Nanomedicine. 2014;9:3971–3985.Citation33
Abbreviations: PFOB NPs, perfluorooctylbromide nanoparticles; PTX, paclitaxel; SNPs, sulfatide-targeted perfluorooctylbromide nanoparticles.
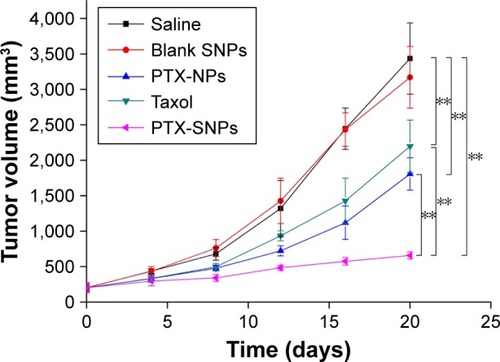
Figure 6 Schematic illustration of the location of doxorubicin or PTX in the PFOB NPs designed by Vu-Quang et alCitation17 and Boissenot et alCitation107 (A). Schematic illustration of the location of doxorubicin in the PFOB NPs designed by Luo et alCitation112 (B).
Abbreviations: FA, folic acid; PFOB, perfluorooctylbromide; PFOB NPs, perfluorooctylbromide nanoparticles; PTX, paclitaxel.
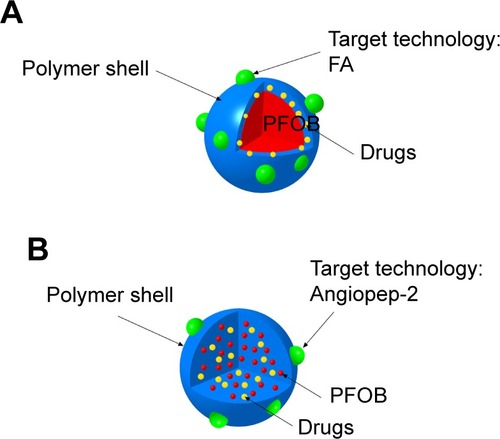
Angiogenesis is a normal physiological process required for the formation of new blood vessels from existing vessels.Citation113 The maintenance, growth and metastasis of solid tumors also require angiogenesis, making it an attractive therapeutic target in the treatment of cancer.Citation114,Citation115 Biomarkers for angiogenesis, such as αvβ3 integrin, vascular endothelial growth factor (VEGF), VEGF receptor-2 (VEGFR2) and α5β1 integrin, can serve as tumor targets.Citation116–Citation119 In the study by Schmieder et al,Citation119 α5β1-targeted PFOB NPs containing fumagillin further decreased the extent of neovessel formation but did not affect the tumor volume in an MDA-MB-435 xenograft mouse model. The MDA-MB-435 tumor model exhibited a very sparse neovasculature with relatively slow growth, which may have caused the negative results.Citation119 One molecular biosignature, αvβ3 integrin, has attracted prominent attention for angiogenesis-targeted applications, because it is involved in endothelial cell recruitment and proliferation, which are important steps in the formation of new blood vessels.Citation74 The utility of αvβ3 integrin as a biomarker of angiogenesis in cancer was studied in the VX-2 xenograft rabbit model. The αvβ3-targeted fumagillin-loaded PFOB NPs designed by Winter et alCitation120 suppress neovasculature formation and inhibit the growth of VX-2 adenocarcinoma xenografts in a targeted manner. The authors subsequently combined the antiangiogenic effect of αvβ3-targeted fumagillin PFOB NPs with zoledronic acid to treat VX-2 adenocarcinoma xenografts. The dual antiangiogenic therapy decreased angiogenesis, stabilized tumor progression and enhanced the anticancer efficacy of chemotherapy.Citation121 Pan et alCitation122 utilized αvβ3-targeted taxane prodrug-loaded PFOB NPs to suppress neovasculature formation and inhibit the growth of VX-2 adenocarcinoma in rabbits in a targeted manner with good efficacy.
Drug delivery for atherosclerosis therapy
Atherosclerotic plaques, which progress from an early atheromatous lesion to a vulnerable plaque through aggressive inflammatory and immune responses, comprise macrophage infiltrates with necrotic core enlargement, neovascular expansion of the vasa vasorum and intraplaque hemorrhage.Citation123,Citation124 Angiogenesis of the vasa vasorum is required for the progression of atherosclerosis;Citation125,Citation126 therefore, αvβ3-targeted PFOB NPs, which were mentioned earlier as an antiangiogenesis tumor therapy, also act as a vehicle for atherosclerosis therapy. Winter et alCitation127 utilized the αvβ3-targeted PFOB NPs for molecular imaging of angiogenesis in subjects with early-stage atherosclerosis and concluded that αvβ3-targeted PFOB NPs can carry several drugs in their lipid monolayers, making them an attractive candidate for drug delivery to early atherosclerotic lesions. The αvβ3-targeted PFOB NPs loaded with fumagillin prepared by Winter et alCitation128 delivered fumagillin and elicited a marked antiangiogenic response with minimal drug dosage. Rabbits with early atherosclerotic lesions administered a single dose of αvβ3-targeted fumagillin PFOB NPs displayed markedly reduced macroscopic adventitial angiogenic expansion of the vasa vasorum compared with the rabbits in the control group.Citation128 In a subsequent study, the authors developed a prolonged antiangiogenesis therapy for atherosclerotic rabbits using atorvastatin and αvβ3-targeted PFOB NPs loaded with fumagillin. The combination of atorvastatin and αvβ3-targeted fumagillin-loaded PFOB NPs synergistically sustained a prolonged antiangiogenic effect in atherosclerotic rabbits.Citation129 VCAM-1-targeted PFOB NPs were generated by Hua et alCitation99 for targeted atherosclerosis therapy in a (ApoE-deficient) mouse model in vivo. Their results prompted subsequent studies showing that VCAM-1-targeted PFOB NPs loaded with a suitable drug are an effective targeted atherosclerosis therapy.Citation99 The shell materials, loaded drugs and their locations and target epitopes of the PFOB NPs as a drug delivery system, which are described in the text, are summarized in .
Table 3 Topical delivery of certain drugs using PFOB NPs as potential carriers for cancer and atherosclerosis therapies
Future perspectives
PFOB NPs are now a multifunctional nanotechnology with potential as both a drug delivery system and a diagnostic platform, and the promising results reported thus far have encouraged us to focus our attention on the following possibilities.
PFOB NPs for delivering drugs while serving as diagnostic agents
PFOB NPs offer great potential for coupling therapeutic functions with diagnostic functions, which indicates that PFOB NPs can serve as theranostic agents.19F, which is highly enriched in PFOB NPs, functions as an excellent probe for quantitative MRI. PFOB NPs have been used to deliver drugs to a targeted site while concurrently providing direct confirmation of drug delivery and therapeutic efficacy via 19F MRI.Citation130 A limitation for 19F MRI is the low signal from targeted/localized PFOB NPs. Thus, PFOB NPs have been modified with payloads of paramagnetic chelates (eg, Gd-DTPA) for high-resolution diagnostic imaging and have been integrated with therapeutic agents for antiangiogenesis therapy.Citation77,Citation120,Citation128,Citation129,Citation131,Citation132
In the clinic, two or more biomedical imaging techniques are usually applied together to achieve more reliable diagnostic results, as each imaging technique has its specific advantages and limitations.Citation58 Hyaluronic acid, a biocompatible and biodegradable material utilized in the development of various drug delivery systems, has also been widely used in the design and fabrication of drug conjugates and NPs for therapy.Citation133 Near-infrared dye-conjugated hyaluronic acid-encapsulating PFOB NPs represent another approach to realize the theranostics: the unique properties of PFOB NPs as contrast agents were enhanced by the coating agents to which they had been conjugated, endowing them with fluorescence in near-infrared imaging and cancer cell-tracking capabilities due to their interaction with hyaluronidases.Citation134,Citation135 Multifunctional UCAs designed by coating the outside of PFOB NPs with hyaluronic acid certainly have potential as theranostic agents for applications in which two or more biomedical imaging techniques are applied together.
PFOB NPs for delivering drugs while serving as diagnostic agents via ultrasonography
As a multifunctional nanotechnology, PFOB NPs have been evaluated for use in various medical applications. In contrast to CT/MRI, US imaging is a more cost-effective and accessible imaging technique and does not use ionizing radiation.Citation136 Nevertheless, the question remains: how do we design PFOB NPs to achieve therapeutic functions coupled with diagnostic functions via ultrasonography?
Importantly, in recent years, two laboratoriesCitation63,Citation72 have designed targeted PFOB NPs that successfully enhanced tumor US imaging in vivo. The most recently reported success in the application of targeted PFOB NPs with a lipid coating to targeted US imaging has inspired a substantial number of subsequent studies on achieving therapeutic functions coupled with diagnostic functions via ultrasonography. The capacity to enhance US imaging will be tremendously improved by developing targeted PFOB NPs to enhance US imaging of the site of interest;Citation63,Citation72 however, there is still no study on the use of PFOB NPs to deliver drugs while serving as diagnostic agents for ultrasonography in vivo. The accumulation of targeted PFOB NPs within the target tissue at a level that is not sufficient to achieve high-quality US imaging enhancement remains a challenge for subsequent studies in this area. Although a single ligand might improve the tumor-targeted US imaging to some extent, the Arg–Gly–Asp-Citation63 or FA-modifiedCitation72 PFOB NPs may not be sufficient to target tumor tissue for higher-quality tumor US imaging. Therefore, additional targeting strategies should be applied to improve the targeting ability of PFOB NPs with a lipid coating. We predict that to address this need, researchers will introduce various biological ligands into PFOB NPs as part of a novel dual-targeting concept, which may provide them with an opportunity to obtain higher-quality tumor US images. This novel dual-targeting concept has already improved the selective delivery of drugs to tumor tissuesCitation137 and is anticipated to facilitate the use of PFOB NPs to further improve tumor US imaging and to achieve the goal of delivering drugs while serving as diagnostic agents for ultrasonography in vivo in the future.
In addition, to extend their applications, PFOB NPs must exhibit minimal toxicity. Recent studies have shown that cell fate can be dictated by the stiffness and topographical characteristics of the ECM.Citation138 Thus, the cellular uptake of NPs can also be regulated, which would influence the efficacy and toxicity of these nanomaterials. Through optimization of the shape and dimensions, particularly the height of nanotopography, the nuclear volume can be modulated through focal adhesion rearrangement to regulate cell function for the end application.Citation139–Citation141 Thus, delineation of the relationships between cell adhesion and nucleus and cell function may provide insight into the rational design of PFOB NPs as novel theranostic agents.
Conclusion
Our laboratory and many other groups have reported that PFOB NPs are a useful system for the delivery of therapeutic agents to target tissues with good efficacy. For drug delivery, more studies have focused on PFOB NPs with a lipid coating than on PFOB NPs with a polymer coating. In summary, we conclude that PFOB NPs, particularly PFOB NPs with a lipid coating, can serve as an effective drug delivery carrier for cancer and atherosclerosis therapies.
Although PFOB NPs are capable of increasing the visualization of US imaging and have been extensively studied, the pace of their development has been relatively slow. Sensitive US imaging will benefit from the optimization of ultrasonography parameters, formulations and targeting abilities, as this nascent application still requires additional efforts. Polymer-coated PFOB NPs have been studied more frequently to optimize ultrasonography parameters and develop formulations for pool imaging in vivo, whereas lipid-coated PFOB NPs can be functionalized with specific ligands and have been studied for targeted tumor ultrasound imaging in vivo.
With a diverse portfolio of therapeutic and potential diagnostic functions via ultrasonography, PFOB NPs may play a promising role in many of the cutting-edge technologies that are currently being developed to fight the most pressing challenges facing the field of medicine today.
Disclosure
The authors report no conflicts of interest in this work.
References
- GiraudeauCGeffroyFMeriauxS19F molecular MR imaging for detection of brain tumor angiogenesis: in vivo validation using targeted PFOB nanoparticlesAngiogenesis201316117117923053783
- WolfsonMRGreenspanJSShafferTHLiquid-assisted ventilation: an alternative respiratory modalityPediatr Pulmonol199826142639710279
- ObraztsovVVGrishanovaAShekhtmanDGSklifasANMakarovKNInteraction of perfluoroctylbromide with liver microsomal monooxygenaseBiokhimiia1993588123412398399771
- LongDMLongDCMattreyRFAn overview of perfluoroctyl-bromide – application as a synthetic oxygen carrier and imaging agent for X-ray, ultrasound and nuclear magnetic resonanceBiomater Artif Cells Artif Organs1988161–34114203052645
- EllenaJFObraztsovVVCumbeaVLWoodsCMCafisoDSPerfluorooctyl bromide has limited membrane solubility and is located at the bilayer center. Locating small molecules in lipid bilayers through paramagnetic enhancements of NMR relaxationJ Med Chem200245255534554212459021
- KrafftMPFluorocarbons and fluorinated amphiphiles in drug delivery and biomedical researchAdv Drug Deliver Rev2001472–3209228
- WangKHuangQQiuFSuiMNon-viral delivery systems for the application in p53 cancer gene therapyCurr Med Chem201522354118413626423086
- ZhangBWangKSiJSuiMShenYCharge-reversal polymers for biodeliveryGuZBioinspired and Biomimetic Polymer Systems for Drug and Gene DeliveryWeinheim, GermanyWiley-VCH Verlag GmbH & Co. KGaA2014223242
- PisaniETsapisNGalazBBridalSLFattalEPerfluorooctyl bromide polymeric capsules as dual contrast agents for ultrasonography and magnetic resonance imagingAdv Funct Mater2008181929632971
- WakabayashiTTamuraMNakamuraTPartial liquid ventilation with low-dose perfluorochemical and high-frequency oscillation improves oxygenation and lung compliance in a rabbit model of surfactant depletionBiol Neonate200689317718216219999
- RiessJGPerfluorocarbon-based oxygen deliveryArtif Cells Blood Substit Immobil Biotechnol200634656758017090429
- JacobyCTemmeSMayenfelsFProbing different perfluorocarbons for in vivo inflammation imaging by 19F MRI: image reconstruction, biological half-lives and sensitivityNMR Biomed201427326127124353148
- GoetteMJKeuppJRahmerJLanzaGMWicklineSACaruthersSDBalanced UTE-SSFP for 19F MR imaging of complex spectraMagn Reson Med201574253754325163853
- SchmiederAHWangKZhangHCharacterization of early neovascular response to acute lung ischemia using simultaneous (19) F/(1)H MR molecular imagingAngiogenesis2014171516023918207
- MorawskiAMWinterPMYuXQuantitative “magnetic resonance immunohistochemistry” with ligand-targeted (19)F nanoparticlesMagn Reson Med20045261255126215562481
- WatersEAChenJYangXDetection of targeted perfluorocarbon nanoparticle binding using 19F diffusion weighted MR spectroscopyMagn Reson Med20086051232123618956417
- Vu-QuangHVindingMSNielsenTUllischMGNielsenNCKjemsJTheranostic tumor targeted nanoparticles combining drug delivery with dual near infrared and 19F magnetic resonance imaging modalitiesNanomedicine20161271873188427133191
- GiraudeauCDjemaiBGhalyMAHigh sensitivity 19F MRI of a perfluorooctyl bromide emulsion: application to a dynamic biodistribution study and oxygen tension mapping in the mouse liver and spleenNMR Biomed201225465466021953998
- DiouOTsapisNGiraudeauCLong-circulating perfluorooctyl bromide nanocapsules for tumor imaging by 19FMRIBiomaterials201233225593560222575831
- PartlowKCChenJBrantJA19F magnetic resonance imaging for stem/progenitor cell tracking with multiple unique perfluorocarbon nanobeaconsFASEB J20072181647165417284484
- BarnettBPRuiz-CabelloJHotaPUse of perfluorocarbon nanoparticles for non-invasive multimodal cell tracking of human pancreatic isletsMol Imaging201164251259
- AhrensETFloresRXuHMorelPAIn vivo imaging platform for tracking immunotherapeutic cellsNat Biotechnol200523898398716041364
- HillMLGorelikovINirouiFTowards a nanoscale mammographic contrast agent: development of a modular pre-clinical dual optical/x-ray agentPhys Med Biol201358155215523523851978
- MattreyRFBrownJJSheltonREOginoMTJohnsonKKMittenRMUse of perfluorooctylbromide (PFOB) to detect liver abscesses with computed tomography. Safety and efficacyInvest Radiol19912697927981938289
- LiuZLammersTEhlingJIron oxide nanoparticle-containing microbubble composites as contrast agents for MR and ultrasound dual-modality imagingBiomaterials201132266155616321632103
- MattreyRFScheibleFWGosinkBBLeopoldGRLongDMHigginsCBPerfluoroctylbromide: a liver/spleen-specific and tumor- imaging ultrasound contrast materialRadiology198214537597627146409
- LanzaGMWallaceKDScottMJA novel site-targeted ultrasonic contrast agent with broad biomedical applicationCirculation19969412333433408989148
- JafariSDiouOMamouJHigh-frequency (20 to 40 MHz) acoustic response of liquid-filled nanocapsulesIEEE Trans Ultrason Ferroelectr Freq Control201461151524402891
- MarshJNHallCSScottMJImprovements in the ultrasonic contrast of targeted perfluorocarbon nanoparticles using an acoustic transmission line modelIEEE Trans Ultrason Ferroelectr Freq Control2002491293811833889
- WicklineSAHughesMNgoFCBlood contrast enhancement with a novel, non-gaseous nanoparticle contrast agentAcad Radiol20029suppl 2S290S29312188251
- Diaz-LopezRTsapisNFattalELiquid perfluorocarbons as contrast agents for ultrasonography and (19)F-MRIPharm Res201027111619902338
- ZhouHFChanHWWicklineSALanzaGMPhamCTAlphavbeta3-targeted nanotherapy suppresses inflammatory arthritis in miceFASEB J20092392978298519376816
- LiXQinFYangLMoLLiLHouLSulfatide-containing lipid perfluorooctylbromide nanoparticles as paclitaxel vehicles targeting breast carcinomaInt J Nanomedicine201493971398525170267
- MorawskiAMWinterPMCrowderKCTargeted nanoparticles for quantitative imaging of sparse molecular epitopes with MRIMagn Reson Med200451348048615004788
- RossiMUse of lecithin and lecithin fractionsIn BioactiVe Egg Compounds200734229239
- KeipertPEOttoSFlaimSFInfluence of perflubron emulsion particle size on blood half-life and febrile response in ratsArtif Cells Blood Substit Immobil Biotechnol1994224116911747849919
- Diaz-LopezRTsapisNLibongDPhospholipid decoration of microcapsules containing perfluorooctyl bromide used as ultrasound contrast agentsBiomaterials20093081462147219097640
- Diaz-LopezRLibongDTsapisNFattalEChaminadePQuantification of pegylated phospholipids decorating polymeric microcapsules of perfluorooctyl bromide by reverse phase HPLC with a charged aerosol detectorJ Pharm Biomed Anal200848370270718818041
- KeHYueXWangJGold nanoshelled liquid perfluorocarbon nanocapsules for combined dual modal ultrasound/CT imaging and photothermal therapy of cancerSmall20141061220122724500926
- FleggMBPooleCMWhittakerAKKeenILangtonCMRayleigh theory of ultrasound scattering applied to liquid-filled contrast nanoparticlesPhys Med Biol201055113061307620463372
- PisaniEFattalEParisJRingardCRosilioVTsapisNSurfactant dependent morphology of polymeric capsules of perfluorooctyl bromide: influence of polymer adsorption at the dichloromethane-water interfaceJ Colloid Interface Sci20083261667118674774
- MakadiaHKSiegelSJPoly lactic-co-glycolic acid (PLGA) as biodegradable controlled drug delivery carrierPolymers (Basel)2011331377139722577513
- ShiveMSAndersonJMBiodegradation and biocompatibility of PLA and PLGA microspheresAdv Drug Deliv Rev199728152410837562
- LiHWangPWangXPerfluorooctyl bromide traces self-assembled with polymeric nanovesicles for blood pool ultrasound imagingBiomater Sci20164697998827121357
- PodellSBurrascanoCGaalMGolecBManiquisJMehlhaffPPhysical and biochemical stability of Optison, an injectable ultrasound contrast agentBiotechnol Appl Biochem199930pt 321322310574690
- KitzmanDWGoldmanMEGillamLDCohenJLAurigemmaGPGottdienerJSEfficacy and safety of the novel ultrasound contrast agent perflutren (definity) in patients with suboptimal baseline left ventricular echocardiographic imagesAm J Cardiol200086666967410980221
- YuanFDellianMFukumuraDVascular permeability in a human tumor xenograft: molecular size dependence and cutoff sizeCancer Res19955517375237567641188
- HughesMSMarshJNHallCSAcoustic characterization in whole blood and plasma of site-targeted nanoparticle ultrasound contrast agent for molecular imagingJ Acoust Soc Am2005117296497215759715
- FosterFSPavlinCJHarasiewiczKAChristopherDATurnbullDHAdvances in ultrasound biomicroscopyUltrasound Med Biol200026112710687788
- FosterFSZhangMYZhouYQA new ultrasound instrument for in vivo microimaging of miceUltrasound Med Biol20022891165117212401387
- Mor-AviVLangRMRecent advances in echocardiographic evaluation of left ventricular anatomy, perfusion, and functionCardiol Rev20019314615911304400
- LiZKeHWangJMiaoZYueXGraphene oxide and gadolinium-chelate functionalized poly(lactic acid) nanocapsules encapsulating perfluorooctylbromide for ultrasound/magnetic resonance bimodal imaging guided photothermal ablation of cancerJ Nanosci Nanotechnol20161632201220927455619
- PisaniETsapisNParisJNicolasVCattelLFattalEPolymeric nano/microcapsules of liquid perfluorocarbons for ultrasonic imaging: physical characterizationLangmuir20062294397440216618193
- DiouOBruletAPehau-ArnaudetGPEGylated nanocapsules of perfluorooctyl bromide: mechanism of formation, influence of polymer concentration on morphology and mechanical propertiesColloids Surf B Biointerfaces201614676276927451363
- HaiatGBertiRGalazBTaulierNAmmanJJUrbachWTwo-dimensional simulation of linear wave propagation in a suspension of polymeric microcapsules used as ultrasound contrast agentsJ Acoust Soc Am201112931642165221428527
- BarnettBPRuiz-CabelloJHotaPFluorocapsules for improved function, immunoprotection, and visualization of cellular therapeutics with MR, US, and CT imagingRadiology2011258118219120971778
- ZhaZWangJZhangSEngineering of perfluorooctylbromide polypyrrole nano-/microcapsules for simultaneous contrast enhanced ultrasound imaging and photothermal treatment of cancerBiomaterials201435128729324120049
- KeHWangJTongSGold nanoshelled liquid perfluorocarbon magnetic nanocapsules: a nanotheranostic platform for bimodal ultrasound/magnetic resonance imaging guided photothermal tumor ablationTheranostics201341122324396512
- LiAZhengYYuJSuperparamagnetic perfluorooctylbromide nanoparticles as a multimodal contrast agent for US, MR, and CT imagingActa Radiol201354327828323319724
- NolteIVinceGHMaurerMIron particles enhance visualization of experimental gliomas with high-resolution sonographyAJNR Am J Neuroradiol20052661469147415956517
- MarshJNPartlowKCAbendscheinDRScottMJLanzaGMWicklineSAMolecular imaging with targeted perfluorocarbon nanoparticles: quantification of the concentration dependence of contrast enhancement for binding to sparse cellular epitopesUltrasound Med Biol200733695095817434667
- KobayashiHTurkbeyBWatanabeRChoykePLCancer drug delivery: considerations in the rational design of nanosized bioconjugatesBioconjug Chem201425122093210025385142
- HughesMSMarshJNArbeitJMApplication of Renyi entropy for ultrasonic molecular imagingJ Acoust Soc Am200912553141314519425656
- Diaz-LopezRTsapisNSantinMThe performance of PEGylated nanocapsules of perfluorooctyl bromide as an ultrasound contrast agentBiomaterials20103171723173119948357
- ZhaoRMatherlyLHGoldmanIDMembrane transporters and folate homeostasis: intestinal absorption and transport into systemic compartments and tissuesExpert Rev Mol Med200911e419173758
- KaneMAThe role of folates in squamous cell carcinoma of the head and neckCancer Detect Prev2005291465315734217
- HartmannLCKeeneyGLLingleWLFolate receptor overexpression is associated with poor outcome in breast cancerInt J Cancer2007121593894217487842
- O’ShannessyDJYuGSmaleRFolate receptor alpha expression in lung cancer: diagnostic and prognostic significanceOncotarget20123441442522547449
- ParkerNTurkMJWestrickELewisJDLowPSLeamonCPFolate receptor expression in carcinomas and normal tissues determined by a quantitative radioligand binding assayAnal Biochem2005338228429315745749
- AssarafYGLeamonCPReddyJAThe folate receptor as a rational therapeutic target for personalized cancer treatmentDrug Resist Updat2014174–6899525457975
- HuYWangYJiangJPreparation and characterization of novel perfluorooctyl bromide nanoparticle as ultrasound contrast agent via layer-by-layer self-assembly for folate-receptor-mediated tumor imagingBiomed Res Int20162016638146427652265
- LiKLiuYZhangSFolate receptor-targeted ultrasonic PFOB nanoparticles: synthesis, characterization and application in tumor-targeted imagingInt J Mol Med20173961505151528487935
- TranTDCaruthersSDHughesMClinical applications of perfluorocarbon nanoparticles for molecular imaging and targeted therapeuticsInt J Nanomedicine20072451552618203420
- WinterPMPerfluorocarbon nanoparticles: evolution of a multimodality and multifunctional imaging agentScientifica (Cairo)2014201474657425024867
- CastroCIBricenoJCPerfluorocarbon-based oxygen carriers: review of products and trialsArtif Organs201034862263420698841
- KanedaMMSasakiYLanzaGMMilbrandtJWicklineSAMechanisms of nucleotide trafficking during siRNA delivery to endothelial cells using perfluorocarbon nanoemulsionsBiomaterials201031113079308620092889
- LanzaGMYuXWinterPMTargeted antiproliferative drug delivery to vascular smooth muscle cells with a magnetic resonance imaging nanoparticle contrast agent: implications for rational therapy of restenosisCirculation2002106222842284712451012
- LeeSJSchlesingerPHWicklineSALanzaGMBakerNAInteraction of melittin peptides with perfluorocarbon nanoemulsion particlesJ Phys Chem B201111551152711527922050303
- PanDPhamCTWeilbaecherKNTomassonMHWicklineSALanzaGMContact-facilitated drug delivery with Sn2 lipase labile prodrugs optimize targeted lipid nanoparticle drug deliveryWiley Interdiscip Rev Nanomed Nanobiotechnol2016818510626296541
- LeeSJSchlesingerPHWicklineSALanzaGMBakerNASimulation of fusion-mediated nanoemulsion interactions with model lipid bilayersSoft Matter20128263024303522712024
- ZhouHFHuGWicklineSALanzaGMPhamCTSynergistic effect of antiangiogenic nanotherapy combined with methotrexate in the treatment of experimental inflammatory arthritisNanomedicine (Lond)2010571065107420874021
- ZhouHFYanHSenpanASuppression of inflammation in a mouse model of rheumatoid arthritis using targeted lipase-labile fumagillin prodrug nanoparticlesBiomaterials201233338632864022922023
- TomlinsonREMcKenzieJASchmiederAHWohlGRLanzaGMSilvaMJAngiogenesis is required for stress fracture healing in ratsBone201352121221923044046
- TomlinsonRESchmiederAHQuirkJDLanzaGMSilvaMJAntagonizing the alphav beta3 integrin inhibits angiogenesis and impairs woven but not lamellar bone formation induced by mechanical loadingJ Bone Mine Res201429919701980
- LanzaGMJenkinsJSchmiederAHAnti-angiogenic nanotherapy inhibits airway remodeling and hyper-responsiveness of dust mite triggered asthma in the Brown Norway ratTheranostics20177237738928042341
- JalloukAPMoleyKHOmurtagKNanoparticle incorporation of melittin reduces sperm and vaginal epithelium cytotoxicityPLoS One201494e9541124748389
- PhamCTThomasDGBeiserJApplication of a hemolysis assay for analysis of complement activation by perfluorocarbon nanoparticlesNanomedicine201410365166024211337
- MarshJNSenpanAHuGFibrin-targeted perfluorocarbon nanoparticles for targeted thrombolysisNanomedicine (Lond)20072453354317716136
- MarshJNHuGScottMJA fibrin-specific thrombolytic nanomedicine approach to acute ischemic strokeNanomedicine (Lond)20116460561521506686
- CyrusTZhangHAllenJSIntramural delivery of rapamycin with alphavbeta3-targeted paramagnetic nanoparticles inhibits stenos is after balloon injuryArterioscler Thromb Vasc Biol200828582082618292395
- WongHLBendayanRRauthAMLiYWuXYChemotherapy with anticancer drugs encapsulated in solid lipid nanoparticlesAdv Drug Deliv Rev200759649150417532091
- DinFUAmanWUllahIEffective use of nanocarriers as drug delivery systems for the treatment of selected tumorsInt J Nanomedicine2017127291730929042776
- WangHLuZWangLNew generation nanomedicines constructed from self-assembling small molecule prodrugs alleviate cancer drug toxicityCancer Res201777246963697429055017
- MishraBPatelBBTiwariSColloidal nanocarriers: a review on formulation technology, types and applications toward targeted drug deliveryNanomedicine20106192419447208
- HowCWRasedeeAManickamSRosliRTamoxifen-loaded nanostructured lipid carrier as a drug delivery system: characterization, stability assessment and cytotoxicityColloids Surf B Biointerfaces201311239339924036474
- SomanNRBaldwinSLHuGMolecularly targeted nanocarriers deliver the cytolytic peptide melittin specifically to tumor cells in mice, reducing tumor growthJ Clin Invest200911992830284219726870
- FrechouMBeray-BerthatVRaynaudJSDetection of vascular cell adhesion molecule-1 expression with USPIO-enhanced molecular MRI in a mouse model of cerebral ischemiaContrast Media Mol Imaging20138215716423281288
- BurteaCBalletSLaurentSDevelopment of a magnetic resonance imaging protocol for the characterization of atherosclerotic plaque by using vascular cell adhesion molecule-1 and apoptosis-targeted ultrasmall superparamagnetic iron oxide derivativesArterioscler Thromb Vasc Biol2012326e36e4822516067
- PanHMyersonJWHuLProgrammable nanoparticle functionalization for in vivo targetingFASEB J201327125526423047896
- GalkinaELeyKVascular adhesion molecules in atherosclerosisArterioscler Thromb Vasc Biol200727112292230117673705
- O’HanlonDMFitzsimonsHLynchJTormeySMaloneCGivenHFSoluble adhesion molecules (E-selectin, ICAM-1 and VCAM-1) in breast carcinomaEur J Cancer200238172252225712441261
- CrossinKLEdelmanGMSpecific binding of cytotactin to sulfated glycolipidsJ Neurosci Res19923346316381282934
- Chiquet-EhrismannRChiquetMTenascins: regulation and putative functions during pathological stressJ Pathol2003200448849912845616
- PanDKimBHuGA strategy for combating melanoma with oncogenic c-Myc inhibitors and targeted nanotherapyNanomedicine (Lond)201510224125125600969
- SoodguptaDPanDCuiGSmall molecule MYC inhibitor conjugated to integrin-targeted nanoparticles extends survival in a mouse model of disseminated multiple myelomaMol Cancer Ther20151461286129425824336
- SongGLiangCYiXPerfluorocarbon-loaded hollow Bi2Se3 nanoparticles for timely supply of oxygen under near-infrared light to enhance the radiotherapy of cancerAdv Mater201628142716272326848553
- BoissenotTFattalEBordatAPaclitaxel-loaded PEGylated nanocapsules of perfluorooctyl bromide as theranostic agentsEur J Pharm Biopharm201610813614427594209
- ShaoKHuangRLiJAngiopep-2 modified PE-PEG based polymeric micelles for amphotericin B delivery targeted to the brainJ Control Release2010147111812620609375
- RuanSYuanMZhangLTumor microenvironment sensitive doxorubicin delivery and release to glioma using angiopep-2 decorated gold nanoparticlesBiomaterials20153742543525453970
- HuangSLiJHanLDual targeting effect of Angiopep-2-modified, DNA-loaded nanoparticles for gliomaBiomaterials201132286832683821700333
- XinHJiangXGuJAngiopep-conjugated poly(ethylene glycol)-co-poly(epsilon-caprolactone) nanoparticles as dual-targeting drug delivery system for brain gliomaBiomaterials201132184293430521427009
- LuoZJinKPangQOn-demand drug release from dual-targeting small nanoparticles triggered by high-intensity focused ultrasound enhanced glioblastoma-targeting therapyACS Appl Mater Interfaces2017937316123162528861994
- AdamsRHAlitaloKMolecular regulation of angiogenesis and lymphangiogenesisNat Rev Mol Cell Biol20078646447817522591
- LiJLHarrisALCrosstalk of VEGF and Notch pathways in tumour angiogenesis: therapeutic implicationsFront Biosci20091430943110
- FolkmanJAngiogenesis and apoptosisSemin Cancer Biol200313215916712654259
- KooimanKFoppen-HarteveldMvan der SteenAFde JongNSonoporation of endothelial cells by vibrating targeted microbubblesJ Control Release20111541354121514333
- KiesslingFFokongSBzylJLederleWPalmowskiMLammersTRecent advances in molecular, multimodal and theranostic ultrasound imagingAdv Drug Deliv Rev201472152724316070
- PochonSTardyIBussatPBR55: a lipopeptide-based VEGFR2-targeted ultrasound contrast agent for molecular imaging of angiogenesisInvest Radiol2010452899520027118
- SchmiederAHCaruthersSDZhangHThree-dimensional MR mapping of angiogenesis with alpha5beta1(alpha nu beta3)-targeted theranostic nanoparticles in the MDA-MB-435 xenograft mouse modelFASEB J200822124179418918697838
- WinterPMSchmiederAHCaruthersSDMinute dosages of alpha(nu)beta3-targeted fumagillin nanoparticles impair Vx-2 tumor angiogenesis and development in rabbitsFASEB J20082282758276718362202
- EsserAKSchmiederAHRossMHDual-therapy with alphavbeta3-targeted Sn2 lipase-labile fumagillin-prodrug nanoparticles and zoledronic acid in the Vx2 rabbit tumor modelNanomedicine201612120121126515754
- PanDSchmiederAHWangKAnti-angiogenesis therapy in the Vx2 rabbit cancer model with a lipase-cleavable Sn 2 taxane phospholipid prodrug using alpha(v)beta(3)-targeted theranostic nanoparticlesTheranostics20144656557824723979
- MorenoPRPurushothamanKRFusterVPlaque neovascularization is increased in ruptured atherosclerotic lesions of human aorta: implications for plaque vulnerabilityCirculation2004110142032203815451780
- MorenoPRPurushothamanKRSirolMLevyAPFusterVNeovascularization in human atherosclerosisCirculation2006113182245225216684874
- McCarthyMJLoftusIMThompsonMMAngiogenesis and the atherosclerotic carotid plaque: an association between symptomatology and plaque morphologyJ Vasc Surg199930226126810436445
- JainRKFinnAVKolodgieFDGoldHKVirmaniRAntiangiogenic therapy for normalization of atherosclerotic plaque vasculature: a potential strategy for plaque stabilizationNat Clin Pract Cardiovasc Med20074949150217712362
- WinterPMMorawskiAMCaruthersSDMolecular imaging of angiogenesis in early-stage atherosclerosis with alpha(v)beta3-integrin-targeted nanoparticlesCirculation2003108182270227414557370
- WinterPMNeubauerAMCaruthersSDEndothelial alpha(v) beta3 integrin-targeted fumagillin nanoparticles inhibit angiogenesis in atherosclerosisArterioscler Thromb Vasc Biol20062692103210916825592
- WinterPMCaruthersSDZhangHWilliamsTAWicklineSALanzaGMAntiangiogenic synergism of integrin-targeted fumagillin nanoparticles and atorvastatin in atherosclerosisJACC Cardiovasc Imaging20081562463419356492
- KanedaMMCaruthersSLanzaGMWicklineSAPerfluorocarbon nanoemulsions for quantitative molecular imaging and targeted therapeuticsAnn Biomed Eng200937101922193319184435
- SchmiederAHWinterPMCaruthersSDMolecular MR imaging of melanoma angiogenesis with alphanubeta3-targeted paramagnetic nanoparticlesMagn Reson Med200553362162715723405
- WinterPMCaruthersSDKassnerAMolecular imaging of angiogenesis in nascent Vx-2 rabbit tumors using a novel alpha(nu) beta3-targeted nanoparticle and 1.5 tesla magnetic resonance imagingCancer Res200363185838584314522907
- GanauMSyrmosNCD’ArcoFEnhancing contrast agents and radiotracers performance through hyaluronic acid-coating in neuroradiology and nuclear medicineHell J Nucl Med2017202166168
- LiangXFangLLiXZhangXWangFActivatable near infrared dye conjugated hyaluronic acid based nanoparticles as a targeted theranostic agent for enhanced fluorescence/CT/photoacoustic imaging guided photothermal therapyBiomaterials2017132728428411450
- GanauMTackling gliomas with nanoformulated antineoplastic drugs: suitability of hyaluronic acid nanoparticlesClin Transl Oncol201416222022324072561
- RapoportNPhase-shift, stimuli-responsive perfluorocarbon nanodroplets for drug delivery to cancerWiley Interdiscip Rev Nanomed Nanobiotechnol20124549251022730185
- KeXLinWLiXWangHXiaoXGuoZSynergistic dual-modified liposome improves targeting and therapeutic efficacy of bone metastasis from breast cancerDrug Deliv20172411680168929092646
- YangYWangKGuXLeongKWBiophysical regulation of cell behavior-cross talk between substrate stiffness and nanotopographyEngineering (Beijing)201731365429071164
- WangKHeXLinthicumWCarbon nanotubes induced fibrogenesis on nanostructured substratesEnviron Sci Nano20174368969928944063
- WangKBruceAMezanRNanotopographical modulation of cell function through nuclear deformationACS Appl Mater Interfaces2016885082509226844365
- SongLWangKLiYYangYNanotopography promoted neuronal differentiation of human induced pluripotent stem cellsColloids Surf B Biointerfaces2016148495827591570