Abstract
Background
Tissue integration and vessel formation are important criteria for the successful implantation of synthetic biomaterials for subcutaneous implantation.
Objective
We report the optimization of plasma surface modification (PSM) using argon (Ar), oxygen (O2) and nitrogen (N2) gases of a polyurethane polymer to enhance tissue integration and angiogenesis.
Methods
The scaffold’s bulk and surface characteristics were compared before and after PSM with either Ar, O2 and N2. The viability and adhesion of human dermal fibroblasts (HDFs) on the modified scaffolds were compared. The formation of extracellular matrix by the HDFs on the modified scaffolds was evaluated. Scaffolds were subcutaneously implanted in a mouse model for 3 months to analyze tissue integration, angiogenesis and capsule formation.
Results
Surface analysis demonstrated that interfacial modification (chemistry, topography and wettability) achieved by PSM is unique and varies according to the gas used. O2 plasma led to extensive changes in interfacial properties, whereas Ar treatment caused moderate changes. N2 plasma caused the least effect on surface chemistry of the polymer. PSM-treated scaffolds significantly (P<0.05) enhanced HDF activity and growth over 21 days. Among all three gases, Ar modification showed the highest protein adsorption. Ar-modified scaffolds also showed a significant upregulation of adhesion-related proteins (vinculin, focal adhesion kinase, talin and paxillin; P<0.05) and extracellular matrix marker genes (collagen type I, fibronectin, laminin and elastin) and deposition of associated proteins by the HDFs. Subcutaneous implantation after 3 months demonstrated the highest tissue integration and angiogenesis and the lowest capsule formation on Ar-modified scaffolds compared with O2- and N2-modified scaffolds.
Conclusion
PSM using Ar is a cost-effective and efficient method to improve the tissue integration and angiogenesis of subcutaneous implants.
Supplementary materials
Figure S1 SEM images of the surface of the scaffolds modified with Ar, N2 and O2 using plasma surface modification for various length of time.
Note: Scale bar: 2 µm.
Abbreviations: Ar, argon; Con, untreated; N2, nitrogen; O2, oxygen; SEM, scanning electron microscopy.
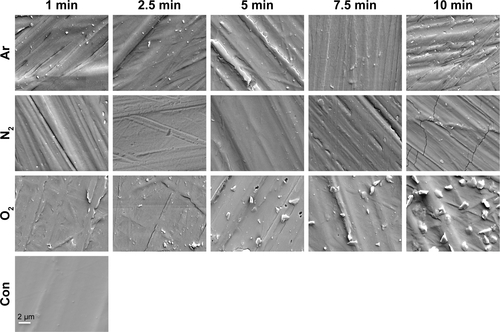
Figure S2 GPC after 5 and 10 minutes of plasma surface modification using Ar, N2 and O2 treatment.
Notes: (A) Effect of plasma modification on Mw. (B) Effect of plasma modification on Mn. (C) Effect of plasma modification on PDI. No significant changes were found after 5 or 10 minutes of Ar or N2 treatment. Ten minutes of O2 treatment caused a significant decrease in the Mw and Mn compared with unmodified scaffolds (P<0.05). **P<0.01.
Abbreviations: Ar, argon; Con, untreated; GPC, gel permeation chromatography; Mn, molecular number; Mw, molecular weight; N2, nitrogen; O2, oxygen; PDI, polydispersity index.
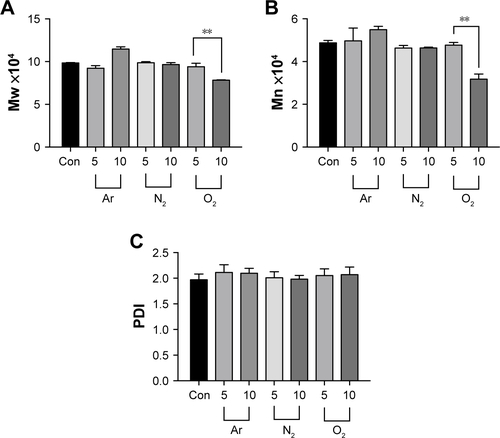
Figure S3 Mechanical properties of the scaffolds after plasma surface modification.
Note: Plasma surface modification showed no change in the tensile Young’s elastic modulus for all exposure times (up to 10 minutes).
Abbreviations: Ar, argon; Con, untreated; N2, nitrogen; O2, oxygen.
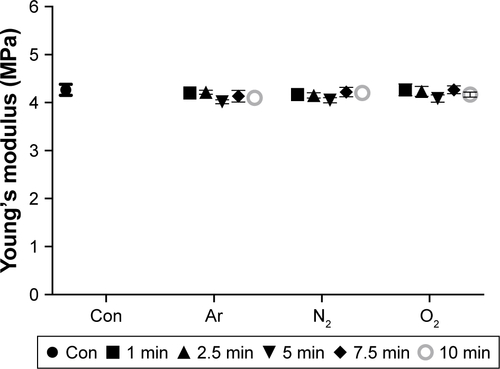
Figure S4 DSC after 5 and 10 minutes of plasma surface modification using Ar, N2 and O2 treatment.
Note: This study showed no changes in the Tg after 5 and 10 minutes of plasma surface modification using Ar, N2 or O2 compared to untreated scaffolds (Con).
Abbreviations: Ar, argon; DSC, differential scanning calorimetry; N2, nitrogen; O2, oxygen; Tg, glass transition temperature.
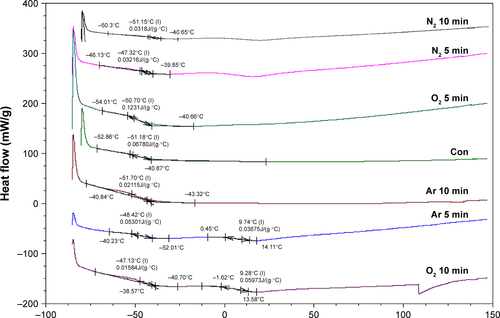
Figure S5 Functional presentation of cell-binding domains of adsorbed fibronectin and vitronectin after plasma surface modification. Total fibronectin (A) and (B) vitronectin protein adsorption onto the scaffolds after 1 hour with 5 minutes of plasma surface modification exposure was analyzed using mAB.
Notes: After 5 minutes of N2 or O2 modification, greater amount of fibronectin was absorbed onto the scaffolds compared with Ar-modified and unmodified scaffolds (Con). Vitronectin adsorption was the greatest after 5 minutes of Ar modification compared with all other scaffolds.
Abbreviations: Ar, argon; Con, untreated; mAB, monoclonal antibodies; N2, nitrogen; O2, oxygen; TCP, tissue culture plate.
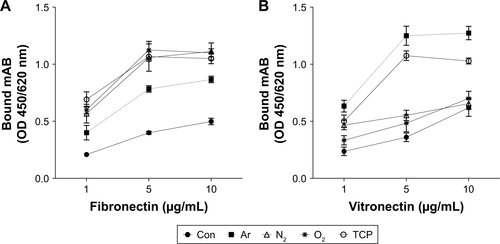
Figure S6 ECM formation by the human dermal fibroblasts after plasma surface modification. Immunocytochemistry confirmed the expression of the ECM markers after 14 days on the plasma-modified and untreated scaffolds (green: collagen type I, elastin, fibronectin; blue: DAPI).
Note: Scale bar: 20 µm.
Abbreviations: Ar, argon; Con, untreated; ECM, extracellular matrix; N2, nitrogen; Neg con, negative control where primary antibody was omitted; O2, oxygen; TCP, tissue culture plate.
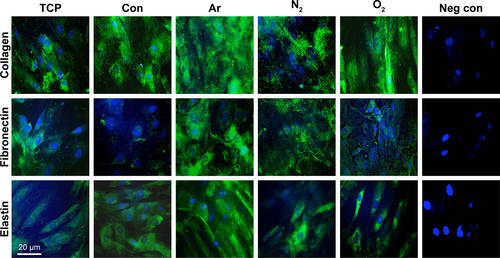
Figure S7 Fibrous capsule formation of the scaffolds treated with plasma surface modification after 12 weeks of subcutaneous implantation.
Notes: (A) Histological sections representing thickness of fibrous capsule at the interface of implant and subcutaneous tissue. Scale bar: 200 µm. (B) Quantification of fibrous capsule thickness shows significantly higher fibrous capsule thickness on the unmodified scaffolds compared with plasma-treated scaffold (*P<0.05).
Abbreviations: Ar, argon; Con, untreated; N2, nitrogen; O2, oxygen.
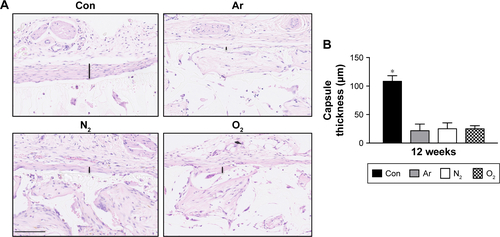
Figure S8 Schematic representation of the mechanism by which cells interact with unmodified and plasma surface-modified scaffolds using Ar, N2 and O2 plasma treatments.
Notes: Control scaffolds show lower protein adsorption and cell attachment, Ar-modified scaffolds show moderate roughness, with higher VN adsorption from serum protein, N2 plasma-modified scaffolds show higher FN adsorption compared with O2 plasma-modified scaffolds, which exhibit the highest surface roughness. Image not to the scale.
Abbreviations: Ar, argon; FN, fibronectin; N2, nitrogen; O2, oxygen; VN, vitronectin.
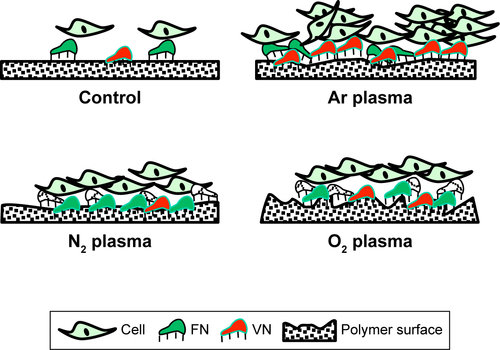
Acknowledgments
This work was funded by the Medical Research Council and Action Medical Research Charity who provided MG a clinical fellowship to conduct this work; GN 2339.
Author contributions
MG performed all experiments and wrote the manuscript. RP performed XPS experiments and analysis of XPS data. VGBM performed analysis of the XPS data. PEB supervised all experiments and wrote the manuscript. DMK supervised all experiments, performed analysis of the data and wrote the manuscript. All authors contributed toward data analysis, drafting and critically revising the paper, gave final approval of the version to be published, and agree to be accountable for all aspects of the work.
Disclosure
The authors report no conflicts of interest in this work.