Abstract
Background
Graphene is considered as a wonder material; it is the strongest material on the planet, super-elastic, and conductive. Its application in biomedicine is huge, with a multibillion-dollar industry, and will revolutionize the diagnostic and treatment of diseases. However, its safety and potential toxicity is the main challenge.
Methods
This study assessed the potential toxicity of graphene oxide nanoplatelets (GONs) in an in vivo animal model using systemic, hematological, biochemical, and histopathological examinations. Normal saline (control group) or GONs (3–6 layers, lateral dimension=5–10 μm, and thickness=0.8–2 nm) at dose rate of 50, 150, or 500 mg/kg were intraperitoneally injected into adult male Wistar rats (n=5) every 48 hours during 1 week to receive each animal a total of four doses. The animals were allowed 2 weeks to recover after the last dosing. Then, animals were killed and the blood was collected for hematological and biochemical analysis. The organs including the liver, kidney, spleen, lung, intestine, brain, and heart were harvested for histopathological evaluations.
Results
The results showed GONs prevented body weight gain in animals after 21 days, treated at 500 mg/kg, but not in the animals treated at 150 or 50 mg/kg GONs. The biochemical analysis showed a significant increase in total bilirubin, with a significant decrease in triglycerides and high-density lipoprotein in animals treated at 500 mg/kg. Nonetheless, other hematological and biochemical parameters remained statistically insignificant in all GONs treated animals. The most common histopathological findings in the visceral organs were granulomatous reaction with giant cell formation and accumulation of GONs in capsular regions. Also, small foci of neuronal degeneration and necrosis were the most outstanding findings in the brain, including the cerebellum.
Conclusion
In conclusion, this study shows that GONs without functionalization are toxic. The future study is a comparison of the functionalized with non-functionalized GONs.
Introduction
Graphene is a carbon-based nanomaterial that is derived from crystalline graphite.Citation1 Graphene is considered a wonder material; it is the strongest material on the planet, super-elastic, and conductive. It has huge applications in medicine, as well as non-medical fields. It is a multibillion dollar industry and will revolutionize our life in the future. Medical applications include drug and gene deliveries, cancer treatment, and development of organs or biomarkers.Citation2 The 2-dimensional (2D) nanostructure of graphene is composed of a single layer of atomic carbons which are organized hexagonally in honeycomb-like networks.Citation3 Generally speaking, there is a large family of graphene nanomaterials (GNMs) that can be fabricated through various methodologies.Citation4–Citation7 Therefore, there are morphologically different types of GNMs, including graphene nano-sheets, nanoplatelets, nano-ribbons, nano-onions, nano-quantum dots, and nano-shells, just to name a few.Citation8–Citation11 Moreover, GNMs can be used either in their intact, pure carbonic form, which is known as pristine graphene, or they can be produced as a functionalized form by subjecting to different chemical or biochemical modifications.Citation12–Citation14 Oxidation is among the common modification techniques that resulted in graphene oxide (GO). GO has attracted a great deal of attention as a promising nanomaterial in various biomedical applications.Citation15,Citation16
The safety and biocompatibility of GO has been a subject of intense research in the scientific community. The reports have been controversial, some reported that GO is nontoxic, even in a high dose of 200 μg/mL after 24 hours of exposure to cells lines,Citation17,Citation18 while others reported that GO interacted with DNA and induced mutagenesis in the MDA-MB-231 human breast cancer cells.Citation19 In fact, GO, even at the low dose of 10 μg/mL, altered gene expression patterns and caused cell apoptosis. Recent studies showed that intravenous injections of GO at 4 mg/kg for five consecutive days caused mutagenesis in mice.Citation19,Citation20
A study investigated the biocompatibility and the distribution of GO (single layer, thickness=1.0 nm, lateral dimension=10–800 nm) in mice following intravenous administration. It showed that GO remained in the blood circulation for a long time, and predominantly accumulated in the lungs. The researchers observed significant pathological changes, including inflammatory cell infiltration, pulmonary edema, and granulomatous reaction at the animals dosed at 10 mg/kg GO after 14 days. However, they did not observe pathological changes in other organs, including the liver, spleen, and kidney in animals treated with 10 mg/kg GO after 14 days. Moreover, GO showed good biocompatibility with red blood cells in their study.Citation21 Accordingly, the potential toxicity and the biocompatibility of GO remain controversial. In fact, parameters including morphology,Citation22,Citation23 size (lateral dimension, thickness, and number of layers),Citation24,Citation25 concentration,Citation17,Citation23 exposure time,Citation26 and surface activity (area, functional group)Citation27 can substantially influence the potential toxicity and biocompatibility of GO and, thus, can convert it from a nontoxic to a highly toxic chemical for living organisms. Considering the aforementioned parameters, there are numerous types of GO with various properties that need to be evaluated for potential toxicities. In toxicological evaluations, it is of paramount importance to provide the sufficient data on the characterization of GO, especially the morphology, size, and surface activity, because, otherwise, this would lead to inconclusive, sometimes contradictory results toward safety profiling of these chemicals. This, in turn, will postpone the promising applications of GO in the biomedical fields. The scarcity of in vivo studies on the toxicity of GO while addressing the complete characteristics of GO was noticed in the most recent literature.Citation28 We have been working on physiochemical properties and functionalization of GOCitation29 and its medical as well as marine applications, the latter as anti-biofouling paint. For medical application, the work is based on the development of organs using a functionalized GO-based nanocomposite scaffold and stem cells technology. Therefore, the toxicology of GO is important and required by the FDA and other regulatory bodies for translation to humans. However, due to its controversial reports on its toxicity, further in vivo studies are crucial to better understand the toxicological effects of the nanoparticles. Our work is based on GO as a nanoplatelet morphology having 3–6 layers, with lateral dimensions of 5–10 μm, and a thickness of 0.8–2 nm. Thus, we embarked on an in vivo toxicological investigation of this special characteristic GO nanoplatelet. The study used Wistar rats as an in vivo animal model. In order to inspect the potential hematotoxicity of GO, major hematological indices were evaluated in the whole blood of animals after exposing to GO. Moreover, we assessed whether the GO show significant changes in the serum levels of biomarkers exploring the potential target organ toxicity of GO through circulating biomarkers. The effects of GO on the serum levels of total protein, lipids, and electrolytes were evaluated to search for any potential interaction of GO with these blood parameters. Finally, the histopathological examinations of major organs of the animals including the liver, kidney, spleen, lung, intestine, brain, and heart were performed to explore the potential dispositional and accumulating fate of GO inside the body, and also the potential specific toxicities of GO toward these organs. To the best of our knowledge, this is the first report on the in vivo toxicological evaluation of this characterized GO in the current literature using mentioned examinations as toxicity endpoints.
Materials and methods
GO and characterization
GO (CAS No of 7732-18-5; carbon purity of 99%) was obtained from the US Research Nanomaterials, Inc. (Houston, TX). Characterization of GO was done using X-ray diffraction (XRD) analysis, Raman spectroscopy, field-emission scanning electron microscopy (FESEM), and transmission electron microscopy (TEM) techniques. XRD measurement was performed on a Philips X’Pert MPD Diffractometer (UK) using Cu Kα radiation (λ=1.5418 A°) at the voltage of 40 kV and the current of 15 mA by scanning in a 2θ range from 0° to 80°. The Raman spectroscopy spectrum was recorded by an Almega Thermo Nicolet Dispersive Raman Spectrometer (Germany) using laser light (λ=532 nm) and in the spectral range of 500–3,000 cm−1. The surface morphology of GO was inspected utilizing FESEM (Sigma, Carl Zeiss, Germany) equipped with an Everhart–Thornley detector (SE2) at the accelerating voltage of 15 kV and working distance of 8.7 mm. TEM (Hitachi S4160, Tokyo, Japan) was used at the accelerating voltage of 30 kV to study the size and morphology of GO. The Brunauer–Emmett–Teller (BET) surface area of GO was acquired through a NOVA 2000e surface analyzer (Quantachrome Instruments, USA).
In vivo animal study
Twenty adult male Wistar rats (8–12 weeks age, body weight of 150–200 g) were obtained from Pasture Institute (Tehran, Iran). The animals were housed in polyethylene cages and maintained under the controlled temperature and 12 hours light/12 hours dark conditions for 1 week before the start of the experiment. They were allowed ad libitum access to the standard pellet and tap water. Animals received humane care, and all the experiments were performed in accordance with the Guidelines of the Institutional Animal Care and Use Committee and were approved by the Animal Ethics Committee of the Faculty of Veterinary Medicine, University of Tehran, Iran (approval No of 7506023.6.17).
Twenty rats were randomly divided into four groups of five animals per group (). Group I was assigned as a vehicle control and received normal saline 0.9% by intraperitoneal injection (5 mL/kg). Groups II–IV were received four doses of GO at 50, 150, or 500 mg/kg every 48 hours during 1 week.
Table 1 animal groups and GONs injections
The selected dose levels of GO were based on our range-finding study. Moreover, our dose selection was in agreement with a recently published study that conducted a comprehensive preclinical safety assessment of a GO pharmaceutical formulation by following regulatory guidelines and performing with good laboratory practice.Citation30
Two weeks after the last dosing, the animals euthanized and blood and organ samples harvested for further toxicological evaluations. The weights of animals were determined before dosing animals with GO and at the final of the experimental period.
Preparation of GO
For each animal, appropriate milligrams of GO were weighed and transferred into the sterile tubes which previously contained normal saline 0.9% solution. Then, the suspensions of nanoparticles were prepared by vortexing the tubes for 10 minutes. GO was homogenously dispersed in normal saline 0.9% solution without producing any agglomerations, and the homogeneity of every dosage was maintained before injecting intraperitoneally into the animals by gentle stirring. All doses of homogenous GO suspensions were prepared freshly and warmed at 37°C each time before the injection.
Hematological and biochemical measurements
Rats were anesthetized with diethyl-ether, and blood samples were collected through the cardiac puncture technique and were divided into two aliquots. The first one was put into BD Vacutainer® blood collection tubes containing K3-EDTA (Beckton & Dickton, Franklin Lakes, NJ, USA) for hematological analysis, and the second one was put into plain tubes in order to prepare the serum samples for biochemical analysis. Serum samples were prepared by centrifugation at 3,000 g for 15 minutes.
Hematological parameters in the whole blood included hemoglobin (Hgb), haematocrit (Hct), white blood cell (WBC) count, red blood cell (RBC) count, mean corpuscular hemoglobin (MCH), mean corpuscular volume (MCV), mean corpuscular hemoglobin concentration (MCHC), and platelet count (PLT). These parameters were analyzed by a hematology autoanalyzer Sysmex Kx-21n (Sysmex Corporation, Japan). The biochemical parameters in the serum samples included blood urea nitrogen (BUN), creatinine (Crea), aspartate aminotransferase (AST), alanine aminotransferase (ALT), alkaline phosphatase (ALP), gamma-glutamyltransferase (GGT), total bilirubin (TB), direct bilirubin (DB), creatine phosphokinase (CPK), total protein (TP), triglyceride (TG), cholesterol (CHO), high density lipoprotein (HDL), and low density lipoprotein (LDL). They analyzed by a biochemical autoanalyzer BT3000 (Bio-Technica, Italy) with standard diagnostic kits (Pars Azmoun Co., Iran). Serum levels of creatine kinase-MB (CK-MB) were measured on the basis of chemiluminescent immunoassay (CLIA) method by a LIAISON® analyzer (DiaSorin, Inc. Germany). Serum electrolytes included sodium (Na+), potassium (K+), and calcium (Ca2+) were determined by an electrolyte analyzer equipped with an ion selective electrode (Caretium Medical Instruments Co., China).
Histopathological examinations
The animals were killed by cervical dislocation after blood collection and immediately underwent an autopsy. The internal organs of animals, including the liver, kidney, spleen, lung, intestine, brain, and heart, were harvested and washed with normal saline and then fixed for 48 hours in a 10% neutral buffered formalin at room temperature. Organs were dehydrated in a graded series of ethanol and xylene and embedded in paraffin and sectioned into slices of 5 μm thickness provided by a rotary microtome. Then, the blocks were deparaffinized and stained with hematoxylin and eosin (H&E). Microscopic evaluations were performed by using a bright-field microscope (Nikon Eclipse 80i, Japan).
Data analysis
The results are presented as means and SD. The one-way ANOVA test with post hoc Bonferroni analysis was used for comparing the groups. A P-value,0.05 was considered as statistically significant. The data analysis was performed by GraphPad Prism Version 5.04 (GraphPad Software, Inc., La Jolla, CA, USA).
Results
The characterization of GO
Results of XRD analysis and Raman spectroscopy are shown in , respectively. XRD analysis of GO samples showed the featured sharp peak at 2θ=~11° indicated structural properties of GO. The Raman spectrum of GO showed a D band at 1,348 cm−1, a G band at 1,585 cm−1, and 2D band at 2,700 cm−1, as GO known peaks. Representative FESEM and TEM images of GO are shown in , respectively. The morphology of GO was generally nanoplatelets with the average number of layers of 3–6. The thickness of nanoplatelets was 0.8–2 nm, and the lateral dimension was 5–10 μm. The specific surface area of GO was 360 m2/g, as determined by BET technique.
Figure 1 Characteristics of GONs. (A) XRD analysis. (B) Raman spectrum. (C) Representative field-emission scanning electron microscopic micrograph of GONs. (D) Representative transmission electron microscopic micrograph of GONs.
Abbreviations: GONs, graphene oxide nanoplatelets; XRD, X-ray diffraction.
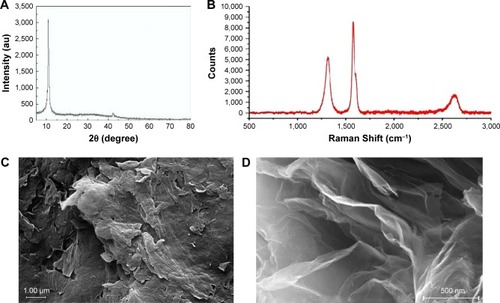
Effects of graphene oxide nanoplatelets (GONs) on body weight changes, hematological, and biochemical parameters
Changes in the body weights of animals are presented in . Rats in the control group showed a statistically significant increase in body weight at the end of the experiment compared with the baseline. Also, we found a significant increase in the body weights of rats receiving 50 and 150 mg/kg GONs at the final of the study period when compared with their pertinent baselines. However, the animals receiving 500 mg/kg GONs did not display a significant body weight increase at the end of the study period when compared with the baseline.
Figure 2 Changes in the body weights of the animals at the baseline and 21 days after receiving repeated doses of GONs via intraperitoneal injections.
Notes: Data are shown as mean±SD (n=5). **P<0.01, ***P<0.001, significantly different when body weights of each group at 21 days were compared with the baseline. Asterisks above the lines indicated significant differences when comparing the body weights of each group at day 21 with the body weight of the 500 mg/kg group at day 21.
Abbreviation: GONs, graphene oxide nanoplatelets.
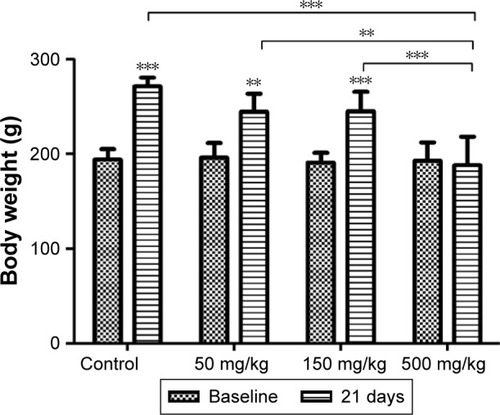
The results of analyzed parameters in the whole blood and the serum are presented in . The levels of TB increased significantly in rats receiving 500 mg/kg GONs when compared with control, but not in the rats receiving 50 or 150 mg/kg GONs. GONs caused a significant decrease in TG and HDL in rats receiving 500 mg/kg GONs when compared with control. However, there were no statistically significant changes in TG and HDL in other GONs treated animals. Also, we did not find any significant changes in other analyzed parameters in the whole blood and the serum.
Table 2 effects of GONs on hematological and biochemical parametersTable Footnotea in rats after 21 days
Histopathological findings
The histology of liver in the control group was normal, with no lesions (). Histopathological examination of the liver of rats receiving 50 mg/kg GONs revealed mild sinusoidal injury and hyperemia (). We observed a granulomatous reaction with some giant cells in the hepatic capsular regions of rats receiving 150 mg/kg GONs. Other histopathological changes in this group included perivascular infiltrations (). Massive foci accumulation of particles revealed in the hepatic capsular region of rats received 500 mg/kg GONs ().
Figure 3 Histopathological effects of GONs on the liver of rats after 21 days. Representative photomicrograph of (A) Control rats (H&E, ×100), (B) Rats receiving 50 mg/kg of GONs (H&E, ×100), (C) rats receiving 150 mg/kg of GONs (H&E, ×100), and (D) Rats receiving 500 mg/kg of GONs (H&E, ×100).
Note: Black arrows indicate the accumulation of GONs.
Abbreviations: GONs, graphene oxide nanoplatelets; H&E, hematoxylin and eosin.
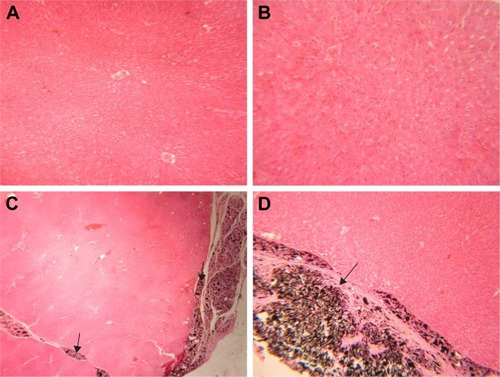
No histopathological lesion in the kidney of control rats was observed (). In the kidney of rats receiving 50 mg/kg GONs, there were small foci of GONs accumulation at the capsular regions. Other histopathological changes in this group were included hyperemia, few foci of interstitial nephritis, and glomerular atrophy (). There was multifocal nephritis in the kidney of rats receiving 150 mg/kg GONs. Also, accumulated particles of GONs at the capsular regions of kidneys were noticed in this group (). Kidney sections in rats receiving 500 mg/kg GONs revealed a granulomatous reaction with giant cell formation in capsular regions, as a consequence of massive accumulation of GONs ().
Figure 4 histopathological effects of GONs on the kidney of rats after 21 days. Representative photomicrograph of (A) control group (H&E, ×200), (B) Rats receiving 50 mg/kg of GONs (H&E, ×100); (C) rats receiving 150 mg/kg of GONs (H&E, ×100), and (D) Rats receiving 500 mg/kg of GONs (H&E, ×40).
Notes: Black arrows indicate glomerular atrophy, a black dashed-arrow indicates interstitial nephritis, Black small arrows indicate capsular accumulation of GONs.
Abbreviations: GONs, graphene oxide nanoplatelets; H&E, hematoxylin and eosin.
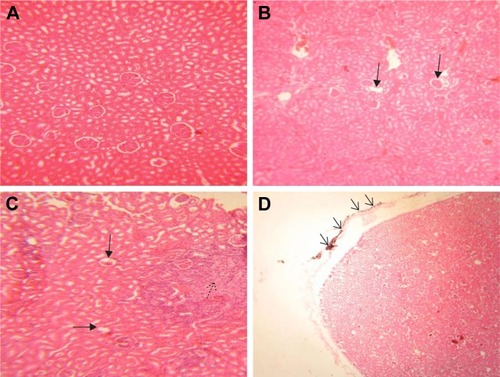
Histology of the spleen was normal in the control group (). In the spleen sections of rats receiving 50 mg/kg GONs, we observed small foci of accumulated GONs particles and hemosiderosis (). There was capsular accumulation of particles in the spleen of rats receiving 150 mg/kg GONs (). Capsular thickening and accumulation of particles observed in rats receiving 500 mg/kg GONs were more noticeable than in the 150 or 50 mg/kg GONs treated groups ().
Figure 5 Histopathological effects of GONs on the spleen of rats after 21 days. Representative photomicrograph of (A) control group (H&E, ×100), (B) Rats receiving 50 mg/kg of GONs (H&E, ×100), (C) rats receiving 150 mg/kg of GONs (H&E, ×100), and (D) Rats receiving 500 mg/kg of GONs (H&E, ×100).
Note: Black arrows indicate the accumulation of GONs.
Abbreviations: GONs, graphene oxide nanoplatelets; H&E, hematoxylin and eosin.
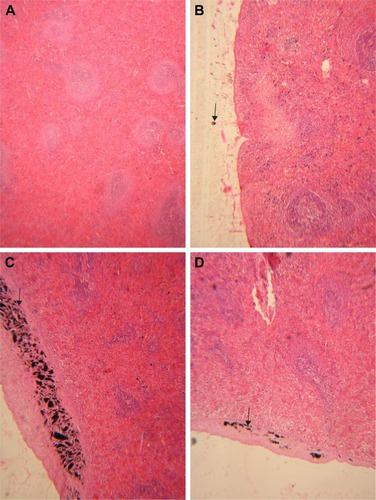
In the lung of rats receiving 50 mg/kg GONs, there was mild alveolar wall thickening. There were small foci of an inflammatory reaction with hemosiderosis in the lung of rats receiving 150 mg/kg GONs. In the lung of rats receiving 500 mg/kg GONs, we observed alveolar wall thickening, hyperemia, and hemosiderosis ().
Figure 6 Histopathological effects of GONs on the lung of rats after 21 days. Representative photomicrograph of (A) control group (H&E, ×100), (B) Rats receiving 50 mg/kg of GONs (H&E, ×40), (C) Rats receiving 150 mg/kg of GONs (H&E, ×40), and (D) Rats receiving 500 mg/kg of GONs (H&E, ×40).
Note: small black arrows indicate alveolar wall thickening.
Abbreviations: GONs, graphene oxide nanoplatelets; H&E, hematoxylin and eosin.
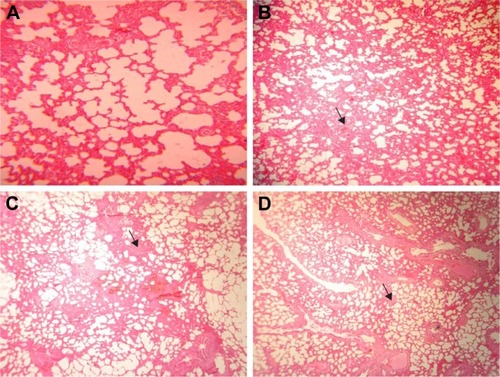
Examinations of intestinal sections revealed mesenteric thickening, along with accumulation of GONs and giant cells. These findings were more noticeable in rats receive 500 mg/kg, in comparison with rats receiving 150 or 50 mg/kg GONs ().
Figure 7 Histopathological effects of GONs on the intestine of rats after 21 days. Representative photomicrograph of (A) Control group (H&E, ×100), (B) Rats receiving 50 mg/kg of GONs (H&E, ×100); (C) rats receiving 150 mg/kg of GONs (H&E, ×100); and (D) Rats receiving 500 mg/kg of GONs show a high accumulation of GONs in the intestinal tissue (H&E, ×40).
Notes: Black arrows indicate GONs accumulation; small black arrows indicate accumulation of GONs in the adventitia layer.
Abbreviations: GONs, graphene oxide nanoplatelets; H&E, hematoxylin and eosin.
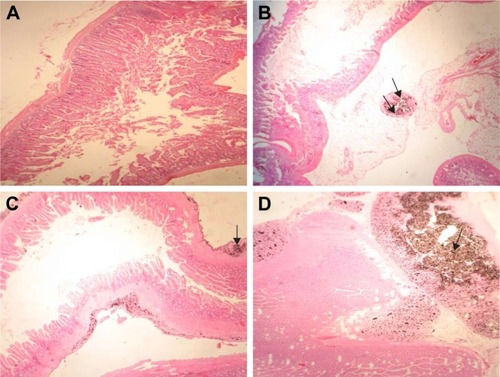
Microscopic examinations of the brain sections displayed abnormal changes in neuronal tissues of all GONs treated groups in comparison with the control group. In fact, some of the neuronal cells of both cerebral and cerebellar cortices showed degeneration and necrosis. These changes were more noticeable in animals receiving 500 mg/kg GONs than in animals receiving 150 or 50 mg/kg GONs. In the cerebellar cortex of all GONs treated animals, we observed the distortion of cerebellar layers that were accompanied by different degrees of degenerated and necrotic neuronal cells (). In particular, degenerated and necrotized Purkinje cells were most conspicuous. Architecture of Purkinje cells perturbed, their cytoplasm shrank, their nuclei disappeared. Also, we observed the loss of Purkinje cells in the Purkinje cell layer. These histopathological alterations were more severe in rats receiving 500 mg/kg GONs when compared with rats receiving either 150 or 50 mg/kg GONs. Also, we observed some degrees of hyperemia and hemorrhage in the cerebellar tissues of treated animals with GONs when compared with control. No morphological changes in meninges and white matter were noticed in any GONs treated groups. Histopathological examinations of hearts disclosed no morphological changes in all GONs treated animals, in comparison with control (data not shown).
Figure 8 Histopathological effects of GONs on the brain of rats after 21 days. Representative photomicrograph of (A) control group (section of cerebellum, H&E, ×200), (B) Rats receiving 50 mg/kg of GONs (section of cerebrum, H&E, ×400), (C) Rats receiving 150 mg/kg of GONs (section of cerebrum, H&E, ×100), and (D) Rats receiving 500 mg/kg of GONs (section of cerebellum, H&E, ×100).
Note: Black arrows indicate degenerated Purkinje cells in cerebellum; small black arrows indicate degenerated neuronal cells in cerebrum.
Abbreviations: ML, molecular layer; PCL, Purkinje cell layer; GL, granular layer; GONs, graphene oxide nanoplatelets; H&E, hematoxylin and eosin.
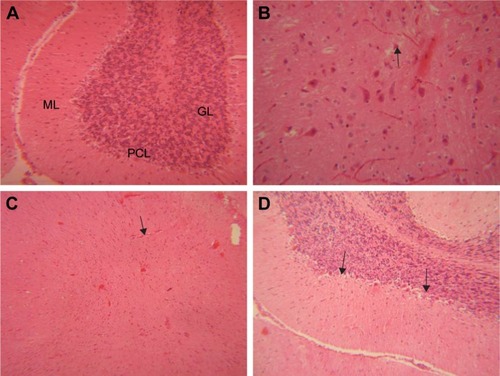
Discussion
Studies on the safety assessment of GO in vivo animal models are still lacking.Citation12,Citation28 In this regard, there is an urgent need for more animal studies to clarify the systemic behaviors of these nanomaterials and their potential toxicities.
In the present study, we evaluated the toxicity of GO, which was characterized as nanoplatelet morphology (GONs) with an average number of 3–6 layers, thickness of 0.8–2 nm, and lateral dimension of 5–10 μm after injecting into adult male Wistar rats. The animals were exposed to four doses of 50, 150, or 500 mg/kg GONs by intraperitoneal injections during 1 week and then allowed 2 weeks to recover. According to the results ( and ), it can be concluded that four cumulative doses of 50 (200 mg/kg) or 150 mg/kg (600 mg/kg) GONs did not affect the body weights of animals or blood and serum parameters after 21 days. On the contrary, GONs at four cumulative doses of 500 mg/kg (2 g/kg) hindered body weight gain and induced a significant increase in serum level of TB and a significant decrease in serum levels of TG and HDL after 21 days ( and ).
Histopathological findings showed that GONs induced dose-dependent injuries in the liver, kidney, spleen, lung, intestine, and brain after 21 days (–).
In the livers, sinusoidal injury, hyperemia, accumulation of GONs in the capsular regions, perivascular infiltration, and granulomatous reaction were observed. In the kidneys, there was GONs accumulation at the capsular regions, hyperemia, interstitial nephritis, glomerular atrophy, and granulomatous reaction. In the spleens, we observed capsular thickening, capsular accumulation of particles, and hemosiderosis. In the lungs, there was alveolar wall thickening along with inflammation, hemosiderosis, and hyperemia. In the intestines, there were serosa thickening, accumulation of GONs, and giant cell formation. In the brains, there were some neuronal cell degenerations and necrosis.
The present results are in agreement with the study of Li et alCitation31 showing that the injection of GO into male Sprague Dawley rats caused no significant changes in the hematological parameters, but induced a significant decrease in serum levels of lipid profiles including HDL. Also, they found that GO induced hyperemia and alveolar wall thickening in the lung, hyperemia and sinusoidal injury in the liver, accumulation of GO and hyperemia in the spleen, and no morphological changes in the heart, which is consistent with our histopathological findings. Another study disclosed the dose-dependent histopathological changes in the kidney of male Sprague Dawley rats upon exposure to different doses of GO.Citation32 This report further confirmed our dose-dependent histopathological findings in the kidney of animals upon exposure to GONs.
In vivo studies on mice as an animal model also showed similar results that are consistent with the present study. For example, Wang et alCitation33 reported the dose-dependent toxicity of GO on mice. GO did not demonstrate any obvious clinical signs of toxicity, including body weight change, in low doses, but showed body weight loss and histopathological changes in high doses. GO caused inflammation and histopathological changes in the lung, liver, spleen, and kidney in a dose-dependent manner. Their report revealed that, by increasing the dose, GO could induce inflammatory responses, including a granulomatous reaction following particle accumulations in internal organs. Previous reports showed that the liver, lung, spleen, and kidney, but not the heart, were among the main target organs for GO toxicity.Citation34,Citation35
In the current study, GONs also accumulated in the serosa of intestinal tissue. The study conducted by Kurantowicz et alCitation36 revealed that GO after intraperitoneal injections in rats formed aggregates in the stomach serous membrane, among mesentery, and in near distance to spleen serosa. However, GO caused no pathological changes in the kidney, lungs, heart, spleen, and liver, and had no effect on the animal weight, biochemical, or hematological parameters during 4 weeks of exposure. They suggested the good biocompatibility of GO, despite the foreign body non-biodegradable behavior of it in rats. The discrepancy between their report and our study is largely due to the differences between GO characteristics, concentration, and experimental design across two studies. Another determinant factor in the biocompatibility and potential toxicity of GO is the existence of functionalization. It has been reported that GONs with dextran functionalization had no effects on hematological indices, serum biochemical parameters, and histopathology of Wistar rats at doses under 125 mg/kg. However, this formulation resulted in minor morphological changes in the liver, lung, spleen, and kidney at doses ≥250 mg/kg. The general patterns of these changes included mild focal congestions, along with particle aggregations.Citation30
Several studies reported that GO with polyethylene glycol (PEG) functionalization mitigate the toxicity. In fact, GO functionalized with PEG had less toxic effects on the hematology, serum biochemistry, and histopathology, despite observing its biodistribution in internal organs including liver, lung, kidney, and spleen.Citation37,Citation38
Our results demonstrated that GONs induced neurotoxic effects in rats. A study by Shang et alCitation39 showed that intraperitoneal injection of GO in male BALB/c mice at a dose of 4 mg/kg for seven consecutive days caused brain damage. The mechanism of this neurotoxicity has been proposed through the lipid peroxidation of the membrane, following excessive generation of reactive oxygen species (ROS) induced by GO, and subsequent overwhelming of antioxidant defenses of cells.
It has been widely accepted that one of the main mechanisms of toxicity of GNMs, including GO, is the generation of intracellular ROS that cause damage to cell macromolecules, including membrane phospholipids, proteins, and DNA, triggering molecular pathways of apoptotic or necrotic cell death.Citation40–Citation42
In the present study, the common finding among the internal organs was inflammatory response, including granulomatous reaction as a consequence of GONs accumulations. Interestingly, it has been shown that GO can activate cell surface toll-like receptors (TLR4 and TLR9) and induce proinflammatory pathways involving NF-κB that ultimately give rise to the production and secretion of cytokines and chemokines.Citation43 Systemic delivery of GO caused significant production of inflammatory cytokines IL-6 and TNF-α in lavage fluids and serum in mice.Citation44
Physical contact of GNMs including GO with cell membranes was reported as another mechanism of toxicity. The experiment by Li et alCitation45 showed that graphene flakes as large as 0.5–10 μm in lateral dimensions entered cells by spontaneous membrane infiltration at corner sites and edge asperities. Otherwise, it has been shown that GO caused ROS generation and subsequent toxicity by accumulating on the cell surface without internalization.Citation46 GO can interact with the cytoskeletal components of the cell, perturbing membrane integrity and inducing cell-cycle alterations, apoptosis, and oxidative stress.Citation47 GO can also cause mitochondrial damage, including the decrease in mitochondrial membrane potential, reduction of ATP production, dysregulation of Ca2+ homeostasis, interference with electron transport chain by disturbing electron transfer, and overproduction of ROS.Citation48,Citation49 The quantitative proteomics approach proposed that GO can interfere with multiple pathways involved in cell energy production and metabolism.Citation50
We acknowledge that the current study has not provided the data regarding the molecular mechanism of toxic injuries of GO including oxidative stress, inflammation, or autophagy. Thus, further investigations are desirable to explore the molecular mechanisms of toxicities of GO.
Another limitation of the present study is the fact that the data was obtained using Wistar rats as an in vivo animal model. Thus, we can not guarantee similar results when other in vivo rodent animal models are used, even by conducting a similar study protocol and using the same characterized GO. In addition, utilization of other in vivo mammalian animal models, especially non-rodents, are required to vet the preclinical safety assessment of GO.
Nonetheless, our results, especially the histopathological findings, can be used for recognizing the characters of toxic injuries of GO on different organs and setting the stage for future investigations on their tissue-specific mechanisms of toxic injuries through considering the abovementioned molecular mechanisms or new ones. Also, the present results are important for safety profiling of the studied GO and, since it showed toxicity, it is suggested not to be considered for clinical applications without taking further actions to reduce its toxicity. It will be interesting to functionalize it with biocompatible coatings and compare its toxicological study results with the present study. Nonetheless, using other GOs with different characteristics, in particular, functionalized GOs are proposed to evaluate for potential toxicity before being considered as promising nanomaterials of clinical applications.
Conclusion
The present study demonstrated the toxicity of GONs with respect to the body weight, biochemical, and histopathological changes following repeated intraperitoneal injections of the nanoparticles in the Wistar rats. GONs at four cumulative doses of 500 mg/kg prevented a significant gain in the body weight of animals over a 21 days period. GONs at this dose level caused a significant increase in serum level of TB, with a significant decrease in the serum levels of TG and HDL over the same period. According to our histopathological findings, it can be concluded that GONs were toxic for the liver, kidney, spleen, lung, intestine, and brain, but not the heart. In general, GONs induced inflammation and a granulomatous reaction in a dose-dependent manner after accumulating inside the body. Our findings can be a useful avenue for further studies to explore the tissue-specific molecular mechanisms of toxicities of GO. The GO is the superior material on the planet and will find many applications in biomedicine. Therefore, uniform strategies for mitigating the toxicity of GOs such as functionalization are encouraged in order to speed up its application to the clinical setting.
Disclosure
This study is a part of Mohammad Amrollahi-Sharifabadi’s PhD thesis. The authors report no conflicts of interest in this work.
References
- NovoselovKSGeimAKMorozovSVElectric field effect in atomically thin carbon filmsScience2004306569666666915499015
- NezakatiTSeifalianATanASeifalianAMConductive polymers: opportunities and challenges in biomedical applicationsChem Rev2018118146766684329969244
- AllenMJTungVCKanerRBHoneycomb carbon: a review of grapheneChem Rev2010110113214519610631
- AvourisPDimitrakopoulosCGraphene: synthesis and applicationsMater Today20121538697
- GeimAKNovoselovKSThe rise of grapheneNat Mater20076318319117330084
- SubrahmanyamKSPanchakarlaLSGovindarajARaoCNRSimple Method of Preparing Graphene Flakes by an Arc-Discharge MethodThe Journal of Physical Chemistry C20091131142574259
- TerronesMBotello-MéndezARCampos-DelgadoJGraphene and graphite nanoribbons: Morphology, properties, synthesis, defects and applicationsNano Today201054351372
- SchinwaldAMurphyFAJonesAMacneeWDonaldsonKGraphene-based nanoplatelets: a new risk to the respiratory system as a consequence of their unusual aerodynamic propertiesACS Nano20126173674622195731
- KosynkinDVHigginbothamALSinitskiiALongitudinal unzipping of carbon nanotubes to form graphene nanoribbonsNature2009458724087287619370030
- ChongYMaYShenHThe in vitro and in vivo toxicity of graphene quantum dotsBiomaterials201435195041504824685264
- EfremovaLVVasilchenkoASRakovEGDeryabinDGToxicity of graphene shells, graphene oxide, and graphene oxide paper evaluated with Escherichia coli biotestsBiomed Res Int2015 Article ID 869361
- NezakatiTCousinsBGSeifalianAMToxicology of chemically modified graphene-based materials for medical applicationArch Toxicol201488111987201225234085
- SawoszEJaworskiSKutwinMToxicity of pristine graphene in experiments in a chicken embryo modelInt J Nanomedicine201493913392225152621
- GurunathanSHanJWParkJHEppakayalaVKimJHGinkgo biloba: a natural reducing agent for the synthesis of cytocompatible grapheneInt J Nanomedicine2014936337724453487
- SuvarnaphaetPPechprasarnSPechprasarnSuejitGraphene-Based Materials for Biosensors: A ReviewSensors201717102161
- LiuJCuiLLosicDGraphene and graphene oxide as new nanocarriers for drug delivery applicationsActa Biomater20139129243925723958782
- ChangYYangSTLiuJHIn vitro toxicity evaluation of graphene oxide on A549 cellsToxicol Lett2011200320121021130147
- BengtsonSKlingKMadsenAMNo cytotoxicity or genotoxicity of graphene and graphene oxide in murine lung epithelial FE1 cells in vitroEnviron Mol Mutagen201657646948227189646
- LiuYLuoYWuJGraphene oxide can induce in vitro and in vivo mutagenesisSci Rep20133346924326739
- PelinMFuscoLLeónVDifferential cytotoxic effects of graphene and graphene oxide on skin keratinocytesSci Rep201774057228079192
- ZhangXYinJPengCDistribution and biocompatibility studies of graphene oxide in mice after intravenous administrationCarbon2011493986995
- ChngELChuaCKPumeraMGraphene oxide nanoribbons exhibit significantly greater toxicity than graphene oxide nanoplateletsNano-scale20146181079210797
- TalukdarYRashkowJLalwaniGKanakiaSSitharamanBThe effects of graphene nanostructures on mesenchymal stem cellsBio-materials2014351848634877
- LiuJHYangSTWangHEffect of size and dose on the biodistribution of graphene oxide in miceNanomedicine20127121801181222830500
- LiuSHuMZengTHLateral dimension-dependent antibacterial activity of graphene oxide sheetsLangmuir20122833123641237222827339
- VallabaniNVMittalSShuklaRKToxicity of graphene in normal human lung cells (BEAS-2B)J Biomed Nanotechnol20117110610721485826
- WangAPuKDongBRole of surface charge and oxidative stress in cytotoxicity and genotoxicity of graphene oxide towards human lung fibroblast cellsJ Appl Toxicol201333101156116423775274
- EmaMGamoMHondaKA review of toxicity studies on graphene-based nanomaterials in laboratory animalsRegul Toxicol Pharmacol20178572428161457
- NezakatiTTanASeifalianAMEnhancing the electrical conductivity of a hybrid POSS-PCL/graphene nanocomposite polymerJ Colloid Interface Sci201443514515525240216
- KanakiaSToussaintJDMullick ChowdhurySDose ranging, expanded acute toxicity and safety pharmacology studies for intravenously administered functionalized graphene nanoparticle formulationsBiomaterials201435257022703124854092
- LiYWangYTuLSub-acute toxicity study of graphene oxide in the Sprague-Dawley ratInt J Environ Res Public Health201613111149
- PatlollaAKRandolphJKumariSATchounwouPBToxicity evaluation of graphene oxide in kidneys of Sprague-Dawley ratsInt J Environ Res Public Health201613438027043588
- WangKRuanJSongHBiocompatibility of graphene oxideNano scale Res Lett2011618
- SyamaSPaulWSabareeswaranAMohananPVRaman spectroscopy for the detection of organ distribution and clearance of PEGylated reduced graphene oxide and biological consequencesBiomaterials201713112113028388498
- JasimDAMénard-MoyonCBéginDBiancoAKostarelosKTissue distribution and urinary excretion of intravenously administered chemically functionalized graphene oxide sheetsChem Sci2015673952396428717461
- KurantowiczNStrojnyBSawoszEBiodistribution of a high dose of diamond, graphite, and graphene oxide nanoparticles after multiple intraperitoneal injections in ratsNanoscale Res Lett20151039826459428
- YangKGongHShiXIn vivo biodistribution and toxicology of functionalized nano-graphene oxide in mice after oral and intraperitoneal administrationBiomaterials201334112787279523340196
- LiBZhangXYYangJZInfluence of polyethylene glycol coating on biodistribution and toxicity of nanoscale graphene oxide in mice after intravenous injectionInt J Nanomedicine201494697470725356071
- ShangSYangS-YLiuZ-MYangXOxidative damage in the kidney and brain of mice induced by different nano-materialsFront Biol20151019196
- MaYShenHTuXZhangZAssessing in vivo toxicity of graphene materials: current methods and future outlookNanomedicine20149101565158025253502
- SasidharanAPanchakarlaLSChandranPDifferential nano-bio interactions and toxicity effects of pristine versus functionalized grapheneNanoscale2011362461246421562671
- ZhangWWangCLiZUnraveling stress-induced toxicity properties of graphene oxide and the underlying mechanismAdv Mater201224395391539722927326
- ChenGYYangHJLuCHChLSimultaneous induction of autophagy and toll-like receptor signaling pathways by graphene oxideBiomaterials201233276559656922704844
- MaJLiuRWangXCrucial role of lateral size for graphene oxide in activating macrophages and stimulating pro-inflammatory responses in cells and animalsACS Nano2015910104981051526389709
- LiYYuanHvon dem BusscheAGraphene microsheets enter cells through spontaneous membrane penetration at edge asperities and corner sitesProc Natl Acad Sci U S A201311030122951230023840061
- ChatterjeeNEomHJChoiJA systems toxicology approach to the surface functionality control of graphene-cell interactionsBiomaterials20143541109112724211078
- MatesanzMCVilaMFeitoMJThe effects of graphene oxide nanosheets localized on F-actin filaments on cell-cycle alterationsBiomaterials20133451562156923177613
- LammelTBoisseauxPFernández-CruzMLNavasJMInternalization and cytotoxicity of graphene oxide and carboxyl graphene nanoplatelets in the human hepatocellular carcinoma cell line Hep G2Part Fibre Toxicol2013102723849434
- DuchMCBudingerGRLiangYTMinimizing oxidation and stable nanoscale dispersion improves the biocompatibility of graphene in the lungNano Lett201111125201520722023654
- ZhouTZhangBWeiPEnergy metabolism analysis reveals the mechanism of inhibition of breast cancer cell metastasis by PEG-modified graphene oxide nanosheetsBiomaterials201435379833984325212524