Abstract
Introduction
Carbon nanotubes (CNTs) have various shapes, including needle-like shapes and curled shapes, and the cytotoxicity and carcinogenicity of CNTs differ depending on their shapes and surface modifications. However, the biological responses induced by CNTs and related mechanisms according to the dispersion state of CNTs have not been extensively studied.
Materials and methods
We prepared multiwalled CNTs (MWCNTs) showing different dispersions and evaluated these MWCNTs in RAW264 cells to determine cytotoxicity, cellular uptake, and immune responses. Furthermore, RAW264 cells were also used to compare the cellular uptake and cytotoxicity of fibrous MWCNTs and spherical carbon nanohorns (CNHs) exhibiting the same degree of dispersion.
Results
Our analysis showed that the cellular uptake, localization, and inflammatory responses of MWCNTs differed depending on the dispersion state. Moreover, there were differences in uptake between MWCNTs and CNHs, even showing the same degree of dispersion. These findings suggested that receptors related to cytotoxicity and immune responses differed depending on the aggregated state of MWCNTs and surface modification with a dispersant. Furthermore, our results suggested that the receptors recognized by the cells differed depending on the particle shape.
Conclusion
Therefore, to apply MWCNTs as a biomaterial, it is important to determine the carcinogenicity and toxicity of the CNTs and to examine different biological responses induced by varying shapes, dispersion states, and surface modifications of particles.
Introduction
Carbon nanotubes (CNTs) are nanomaterials having a structure in which graphene sheets are wound in a tubular shape. CNTs have unique electrical characteristics, thermal properties, and mechanical propertiesCitation1,Citation2 because of their composition (only carbon atoms) and specific shape, and by combining CNTs with other materials, the performance of the base metal is greatly improved. Thus, product development in various fields has been actively carried out,Citation3 such as in radionuclide therapyCitation4 and bioimaging studies,Citation5 and we have developed sockets for artificial joints using tangled multiwalled CNTs (MWCNTs). However, the safety of MWCNTs when used for applications in human beings is still unclear, and further studies are needed to confirm the safety of MWCNTs for use in living organisms.Citation6
Because MWCNTs are foreign substances in the living body, the immune system is activated acutely in their presence, and MWCNTs are then phagocytosed by immune cells, such as macrophages.Citation7 Uptake of foreign substances by these cells represents a toxic stimulus, resulting in an inflammatory reaction and subsequent tissue fibrosis.Citation8,Citation9 MWCNTs incorporated into macrophages induce the expression of immune system proteins, stimulating the secretion of inflammatory cytokines.Citation10,Citation11 In contrast, some studies have shown that exposure of MWCNTs to macrophages does not induce a strong inflammatory response.Citation12–Citation14 Further studies have shown that the immune response to cells following the exposure to MWCNTs varies according to both surface modifications and MWCNT size.Citation15 In addition, MWNT7, a type of MWCNTs, has a structure resembling a needle, similar to asbestos, and shows obvious carcinogenicity.Citation16,Citation17 In contrast, tangled MWCNTs, which are different from the needle-like MWNT7, have been reported to exhibit no carcinogenicity in rats. Therefore, not all CNTs show carcinogenic properties, but CNT size and shape may be related to carcinogenicity.
Untreated CNTs are hydrophobic and have low solubility; therefore, they tend to aggregate in aqueous solution. Furthermore, tangled CNTs, which have not yet been shown to be carcinogenic, can become entangled due to their diameter (several nanometers to several tens of nanometers) and length (up to several micrometers). In previous studies, nondispersive CNTs have been shown to form larger aggregates and show stronger toxicity.Citation15 However, some studies have successfully dispersed CNTs completely and evaluated the CNT particles themselves.Citation18,Citation19 In general, strong ultrasonic treatment is necessary to achieve sufficient dispersion, but can cause shearing of the CNTs.Citation20
In previous studies from our laboratory, highly dispersed CNTs were fabricated using ultrasonic treatment equipment that did not change the length of CNTs by applying medium ultrasonic waves while rotating the sample. Evaluation of highly dispersed CNTs and aggregated tangled CNTs using carcinogenic human bronchus cells demonstrated that only aggregated CNTs were taken up by cells and showed growth suppression.Citation21 However, for macrophages, which are believed to affect the carcinogenicity of CNTs,Citation19 few studies have investigated the effects of fully dispersed tangled CNTs on cell proliferation and immune responses.
In this study, we aimed to clarify the immune responses of highly dispersed CNTs and aggregated CNTs using RAW264 cells as mouse macrophage-like cells. Furthermore, we compared and investigated the influence of the dispersion state, fibrous CNTs, and spherical carbon nanohorns (CNHs) on the immune response.
Materials and methods
Characterization of MWCNTs and CNHs
MWCNT materials were provided by Cnano Technology (FT9110; Santa Clara, CA, USA). FT9110 was manufactured using a catalytic vapor deposition method and had specific properties as described by the manufacturer (diameter: 10–15 nm, length: 10 µm or less, purity: 99.8% or more, iron content: <100 ppm).
CNHs (CNHox; NEC, Tokyo, Japan) had specific properties as described by the manufacturer (diameter of aggregates: approximately 100 nm, purity: 95% or more, graphene content: <5%, iron content: 0%).
Suspension and dispersion of FT9110 and CNHs
FT9110 was sterilized in an autoclave at 121°C for 15 minutes and dried, and 10 mg/mL FT9110 was then vortexed in two dispersants (2% FBS [Biowest, Nuaillé, France] in Dulbecco’s PBS [DPBS] and 0.1% PS [NOF, Tokyo, Japan] in DPBS). FT9110 was dispersed using three different sonicators that were renamed according to the ultrasonic treatment method and output: the PR-1 sonicator (Thinky, Tokyo, Japan) was termed as W-140 (water bath type, 140 W power), the US-1R sonicator (As one, Tokyo, Japan) was termed as W-55 (water bath type, 55 W power), and the W-220 sonicator (Heat Systems-Ultrasonic, Plainview, NY, USA) was termed as H-140 (homogenizer type, 140 W power). CNHs were dispersed at 1 mg/mL by sonication using W-140.
To determine the hydrodynamic size of the agglomerated FT9110 and CNHs, sonicated FT9110 and CNHs were measured using a Zetasizer Nano ZS (Malvern Instruments, Malvern, UK; ), and the zeta potential of the sonicated FT9110 and CNHs was determined with a Zetasizer Nano ZS (). The FT9110 and CNHs were diluted to 0.1 mg/mL, and each measurement was conducted in triplicate.
Table 1 Diameter of FT9110 and CNH particles used in the experiments
Table 2 Zeta potential of FT9110 and CNH particles used in the experiments
Cell culture
RAW264 mouse monocyte macrophages were purchased from Riken (Saitama, Japan). RAW264 cells were cultured in minimum essential medium (MEM; Nacalai Tesque, Kyoto, Japan) with 10% FBS and nonessential amino acid solution (100×; Nacalai Tesque) at 37°C in a 5% CO2 humidified incubator and passaged twice per week. For each experiment, the cells were seeded at a density of 6×104 cells/mL and allowed to adhere for 24 hours.
Cell viability
Cell viability was assessed using an alamar blue assay (alamarBlue® cell viability reagent; Remel, Lenexa, KS, USA). Cells were plated in 96-well plates and incubated for 24 or 48 hours at 37°C in culture medium containing 100 µg/mL FT9110 in a dispersant or in control medium containing only dispersant. After aspiration of the culture medium to exclude the influence of FT9110, 10% alamar blue reagent in culture medium was added to each well, where viable cells metabolized the dye for 60 minutes, resulting in increased fluorescence detected by excitation/emission at 535/590 nm using a plate reader (AF2200; Eppendorf, Hamburg, Germany). Cell viability was calculated as follows: percent cytotoxicity = 100 × experimental value/control value. The media were assayed six times for each treatment condition.
Observation of cells by fluorescence microscopy
Cells cultured on Cellview glass-bottomed advanced TC four compartments (Greiner Bio-one, Frickenhausen, Germany) were exposed to FT9110 and CNHs at 100 µg/mL for 48 hours under the same conditions as described for the cell viability assay. For assessment, cells were stained with nuclear stain (Bisbenzimide H33342 Fluorochrome Trihydrochloride Dimethyl Sulfoxide Solution; Nacalai Tesque) and lysosome stain (Cyto Painter Lysosomal Staining ab 138895; Abcam, Cambridge, UK) 30 minutes before observation. The cells were visualized using an AxioObserverZ1 fluorescence microscope (Carl Zeiss Jena GmbH, Jena, Germany) with a 40× objective lens.
Assessment of FT9110 uptake by flow cytometry
Assessment of FT9110 uptake was determined by flow cytometry. Briefly, the cells were grown in 24-well plates incubated for 24 hours at 37°C in the presence or absence of 10 µg/mL FT9110. The cells were washed with DPBS to remove unbound FT9110, harvested with trypsin, and centrifuged at 400× g for 3 minutes. The precipitated cells were suspended in DPBS containing FBS. Side scatter in a light-scattering analysis was immediately measured up to 10,000 events using a FACSCalibur instrument. Test media were assayed in triplicate for each treatment condition.
Observation by electron microscopy
Cells grown on cover slips in six-well plates were exposed to FT9110 and CNHs (100 µg/mL in RAW264 cells) for 24 hours. Cells were washed twice in DPBS, fixed with 2.5% glutaraldehyde, postfixed with 1% osmic acid, and embedded in Epon embedding resin (Epok 812; Okenshoji, Tokyo, Japan). Sections were cut to 60 nm thickness, stained with uranyl acetate and lead citrate, and visualized under a JEM1400 TEM (JEOL, Tokyo, Japan) at 80 keV.
Cytokine measurement
Cytokines in the culture supernatant were measured with a cytometric bead array flex set system (BD Biosciences, San Jose, CA, USA), according to the manufacturer’s protocol. Briefly, RAW264 cells in 24-well plates were exposed to 10 µg/mL FT9110 for 24 hours, and cytokine capture beads (for TNF, RANTES, MIP-1α, MCP-1, IL-1β, IL-10, and IL-6) were added to the samples or cytokine standards (10–2,500 pg/mL) in flow cytometry tubes. The mixtures were vortexed, and antibodies for fluorescence detection were added to each tube. The samples were then incubated at room temperature for 2 hours. Following incubation, the beads were washed once and resuspended prior to reading with a FACSCalibur apparatus (BD Biosciences). Test media were assayed in triplicate for each treatment condition.
Statistical analysis
Data are presented as mean ± standard error (SE). Statistical significance was determined by analysis of variance followed by the Tukey–Kramer method. Differences with P-values of <0.05 were considered statistically significant.
Results
Cell viability
The viability of cells exposed to Flotube 9110 (FT9110) dispersed in polysorbate 80 (PS) for 24 hours was significantly decreased compared with that of the control, and significant differences were observed between the effects of particles dispersed using the W-140 and the W-55 or H-140 sonicators (W-140: 93.9%, W-55: 64.1%, H-140: 65.4%). After 48 hours, the cell survival rate further decreased with W-55 and H-140 (W-140: 98.9%, W-55: 48.2%, H-140: 42.2%). Cells exposed to FT9110 dispersed with FBS showed 104.4%, 78.6%, and 94.1% viability using W-140, W-55, and H-140, respectively, at 24 hours and 126.5%, 82.3%, and 76.4% viability using W-140, W-55, and H-140, respectively, at 48 hours; thus, there were no decreases in cell viability when compared with the control group ().
Figure 1 Viability of RAW264 cells exposed to FT9110.
Notes: In (A), the cell viability was measured with FT9110 after 24 hours. In (B), the cell viability was measured with FT9110 after 48 hours. (A, B): FT9110 was dispersed in FBS or PS at 100 µg/mL. The control was medium containing each dispersant only. Data are expressed as mean ± SE (n=6). *P<0.05; **P<0.01.
Abbreviations: FT9110, Flotube 9110; PS, polysorbate 80; SE, standard error.
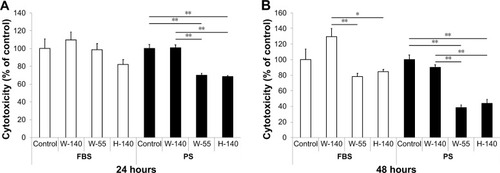
Observation of cells by fluorescence microscopy
Next, we examined the state of cells exposed to FT9110 with a fluorescent microscope. The cells exposed to FT9110 dispersed using the W-140, regardless of the dispersant, were adhered to the glass bottom, similar to control cells, and endocytosed FT9110 (). Moreover, the exposure of RAW264 cells to CNHs dispersed in FBS and PS resulted in intracellular uptake and localization of CNHs to the lysosomal membrane ().
Figure 2 Live cells imaged after incubation with bisbenzimide H33342 fluorochrome trihydrochloride for nuclear staining.
Notes: (A) Control; (B) W-140; (C) W-55; (D) H-140. (B–D) RAW264 cells exposed to FT9110 at 100 µg/mL in 0.1% PS-DPBS. The control was medium containing each dispersant only.
Abbreviations: DPBS, Dulbecco’s PBS; FT9110, Flotube 9110; PS, polysorbate 80.

Figure 3 Live cells imaged after incubation with bisbenzimide H33342 fluorochrome trihydrochloride for nuclear staining and Cyto Painter Lysosomal Staining ab for lysosomes in dispersant (0.1% PS-DPBS and 2% FBS-DPBS).
Notes: (A, D) Control. RAW264 cells exposed to (B, E) FT9110 at 100 µg/ml and (C, F) CNHs at 100 µg/ml. (A–C) RAW264 cells in 0.1% PS-DPBS and (D–F) RAW264 cells in 2% FBS-DPBS. The control was medium containing each dispersant only.
Abbreviations: CNH, carbon nanohorn; DPBS, Dulbecco’s PBS; FT9110, Flotube 9110; PS, polysorbate 80.
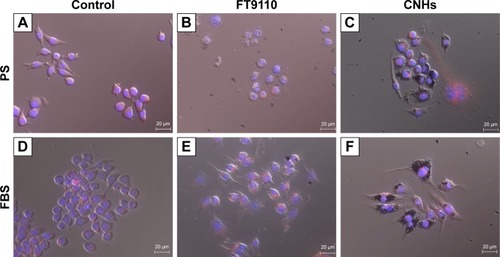
Assessment of FT9110 uptake by flow cytometry
The uptake of FT9110 dispersed using W-55 and H-140 increased significantly compared with the control in both dispersants. The uptake of FT9110 dispersed using W-140 increased significantly compared with that of the control in FBS. In contrast, the uptake of FT9110 dispersed using W-140 with PS was not increased compared with that of the control ().
Figure 4 Uptake of FT9110 by RAW264 cells, as demonstrated by flow cytometry.
Notes: Cultured RAW264 cells were exposed to FT9110 at 10 µg/mL. The control was medium containing each dispersant only. Data are expressed as mean ± SE (n=3). **P<0.01.
Abbreviations: FT9110, Flotube 9110; PS, polysorbate 80; SE, standard error; SSC, side scatter.
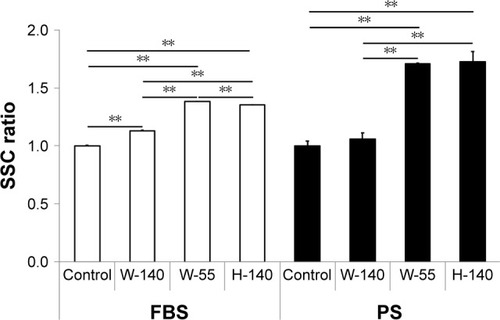
Observation of cells by transmission electron microscopy (TEM)
Cells exposed to FT9110 and CNHs were observed using TEM (). FT9110 dispersed with W-55 was present in a bag-like structure, similar to a phagosome, exhibiting a state of entanglement. In contrast, weak uptake of FT9110 was observed in cells exposed to FT9110 dispersed with W-140, although few aggregates were observed because most particles were well dispersed. CNHs were also incorporated into the lysosomes in large amounts.
Figure 5 Transmission electron micrographs of RAW264 cells exposed to 100 µg/mL FT9110 and CNH uptake in dispersant (0.1% PS-DPBS).
Notes: (A, B) Control, RAW264 cells exposed (C, D) FT9110 sonicated by W-140, (E, F) FT9110 sonicated by W-55. (G, H) CNHs sonicated by W-140. (A, C, E, G) Low magnification images. Scale bars correspond to 1 µm. (B, D, F, H) High magnification images. Scale bars correspond to 500 nm. The control was medium containing dispersant only. Yellow arrows indicate (C–F) FT9110 and (G, H) CNHs. Blue Ns indicate nuclei.
Abbreviations: CNH, carbon nanohorn; DPBS, Dulbecco’s PBS; FT9110, Flotube 9110; PS, polysorbate 80.
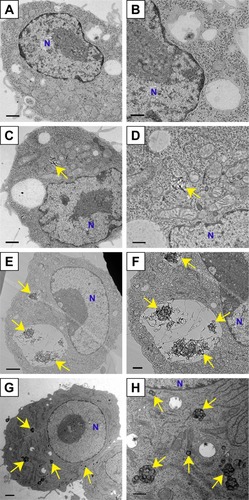
Assessment of inflammatory cytokines secreted from cells after FT9110 exposure
A total of seven cytokines/chemokines were evaluated. Among these, interleukin (IL)-1β, IL-10, and IL-6 were not detected by flow cytometry. In contrast, tumor necrosis factor (TNF), regulated upon activation normal T cell expressed and secreted (RANTES), macrophage inflammatory protein (MIP)-1α, and monocyte chemotactic protein (MCP)-1 showed differential secretion among groups (). Secretion of TNF, RANTES, and MCP-1 was significantly increased when cells were exposed to FT9110 dispersed with W-55 or H-140 using FBS as a dispersant compared with that of the control, whereas MIP-1α secretion decreased. In addition, the secretion of TNF and RANTES was significantly increased from cells exposed to FT9110 subjected to sonication with H-140 and W-55 using PS as a dispersant, the secretion of MCP-1 was increased only from cells dispersed with H-140, and the secretion of MIP-1α was significantly increased with W-140 and H-140. Regardless of the dispersant, FT9110 dispersed using W-140 did not tend to yield different results from the control, although MIP-1α secretion was significantly decreased compared with that of the control in FBS.
Figure 6 Measurement of inflammatory cytokine secretion by RAW264 cells after FT9110 exposure.
Notes: Cultured RAW264 cells were exposed to FT9110 at 10 µg/mL. After 24 hours, levels of cytokine and chemokine secretion were analyzed. (A) TNF. (B) RANTES. (C) MIP-1α. (D) MCP-1. The control was medium containing each dispersant only. Data are expressed as mean ± SE (n=3). *P<0.05; **P<0.01.
Abbreviations: FT9110, Flotube 9110; MCP, monocyte chemotactic protein; MIP, macrophage inflammatory protein; PS, polysorbate 80; RANTES, regulated upon activation normal T cell expressed and secreted; SE, standard error; TNF, tumor necrosis factor.
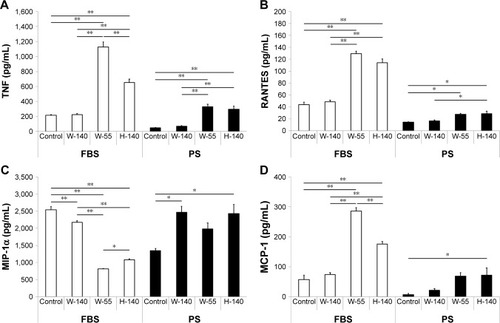
Discussion
In this study, we evaluated the safety and effects of FT9110 in macrophages. Our results provided insights into the potential applications of FT9110 by demonstrating their effects on immune system cells and cytokine/chemokine secretion.
In this study, we found that FT9110 dispersed in PS was cytotoxic after 24 hours, and our findings also suggested that both the aggregation state of the particles and the different dispersants used may have influenced cytotoxicity. There are reports that MWCNT cytotoxicity is affected by impurities.Citation22 The current study employed FT9110 with very few impurities (99.8% purity) and an iron content of <100 ppm. Although an effect of iron content in MWCNTs on cytotoxicity has been reported,Citation23 the iron content of MWCNTs with no effect on cytotoxicity and that of FT9110 was nearly equivalent. Moreover, previous studies have shown that the uptake and cytotoxicity of MWCNTs prepared using various dispersants differed in BEAS-2B human bronchial epithelial cells and MESO-1 human malignant pleural mesothelioma cells and that the exposure of A549 lung cancer cells to CNTs dispersed with surfactant or biologically derived protein resulted in different levels of cytotoxicity and inflammatory reactions.Citation24,Citation25 Consistent with the abovementioned information, our findings showed that cytotoxicity was affected not by impurities contained in FT9110, but rather by the different surface modifications of FT9110 MWCNTs induced by the dispersants.
Notably, aggregated CNT and CNHs showed increased uptake in RAW264 cells. Previous studies have shown that various nanosized materials are taken up into cells by endocytosis.Citation26 In addition, RAW264 cells exposed to MWCNTs produced inflammatory cytokines and showed cytotoxicity,Citation27,Citation28 and when BEAS-2B human bronchial epithelial cells and small airway respiratory epithelial cells (SAEC) were exposed to MWCNTs, damage to the mitotic spindle formation was observed in a concentration-dependent manner, resulting in impaired cell division.Citation29 In this study, aggregated CNT was taken up in RAW264 cells in large amounts, possibly inhibiting cell proliferation by producing inflammatory cytokines and blocking cell division. In contrast, the uptake of FT9110 highly dispersed in FBS was observed in RAW264 cells, although the amount was less than that of aggregated CNT or CNHs. Decreased cell viability was not observed because FT9110 did not accumulate in the cells excessively.
Interestingly, we found that
CNHs dispersed in FBS and PS showed good uptake into the cells. Similarly, in a study of carbon black (CB), which is prepared using the nanocarbon material and is spherical and nanosized, good uptake was observed in the cytoplasm through endocytosis in RAW264 cells.Citation30 In this experiment, spherical CNHs were also potentially taken up into cells by endocytosis, similar to CB, regardless of the dispersant. Moreover, TEM observations showed that highly dispersed FT9110 was scattered in the cytoplasm, whereas aggregated CNT was captured as aggregates in the phagosome inside the cells. We also found that CNHs were taken up into phagosomes. In our previous studies, we reported that different types of CNTs were incorporated into cellsCitation31 and that the same CNTs affected the uptake into cells due to the dispersed state of particles.Citation21 In this study, like our previous study, we found that the aggregated CNT was incorporated into RAW264 cells, but that the amount of material taken up differed dramatically between highly dispersed FT9110 and CNHs showing the same degree of dispersion. Thus, these findings suggested that the mechanisms recognized by the cells differed depending on the state of aggregation and particle shape.
In our experiments, different cytotoxicity and immune responses were obtained depending on the dispersion state of FT9110 and the dispersant. Reports have described that there were differences in protein corona composition and amount among raw single-walled CNTs (SWCNTs), raw MWCNTs, and modified MWCNTs.Citation32 We suspect that the protein corona formed on CNTs depends on the dispersing agent and moreover that the amount and kind of proteins formed by aggregation state based on the size and shape of the CNT differ greatly. The differences in cytotoxicity and immune response obtained in this study may have been due to variations in the protein corona generated by the aggregation state of FT9110 along with its surrounding dispersant and complex conditions.
Recent reports have shown that some receptors, such as the MARCO receptor, which is a type of scavenger receptor, may be involved in cellular uptake of nanomaterials, such as CNTs and CNHs,Citation33–Citation35 and this scavenger receptor is known to be expressed on the membrane surface of RAW264 cells.Citation36 Scavenger receptors on RAW264 cells recognize the negative charges of CNTs,Citation37 and MARCO receptors on J774.1 macrophages recognize proteins adsorbed on the CNT surface and induce uptake and immune responses.Citation38 The aggregated CNT and CNHs taken up in the phagosomes in this study may be taken up via such receptors. In contrast, highly dispersed FT9110 was scattered and incorporated in the cells, but the amount of uptake was clearly less than that of aggregates. This result may be due to the fact that the surface charges and surface modifications of aggregated CNT and CNHs and highly dispersed FT9110 are different. Thus, receptors mediating uptake by macrophages that recognize carbon nanomaterials may differ, even for the same carbon nanomaterial, due to differences in particle shape, aggregation state, and surface modifications.
Cytokine secretion from RAW264 cells exposed to FT9110 varied depending on the dispersion state of FT9110 and the dispersant used. Nanoparticles such as CNTs cause inflammatory reactions, even when they do not affect cell proliferation, and particles of the same size may elicit different immune responses depending on the type of nanoparticles.Citation39,Citation40 Many receptors, such as Toll-like receptors (TLRs) and scavenger receptors, are present on macrophage cell membranes. Exposure of primary human monocyte-derived macrophages to SWCNTs demonstrated that CNTs could be recognized as pathogens, as supported by their effects on chemokine secretion induction through the TLR2/TLR4/MyD88/nuclear factor-κB signaling pathway.Citation41 CNTs and fullerenes may be recognized as pathogens by fitting into the hydrophobic structure inside the TLR of the cell membrane in human lung carcinoma epithelial cells.Citation42 In addition, our findings demonstrated that various cytokines could be secreted in the immune response through different receptors, such as TLRs, based on the combinations of particle size and shape, dispersant, aggregation state, and protein corona.
Cells release extracellular nucleotides and adenosine triphosphate (ATP) when exposed to inflammation or injury.Citation43 The P2Y6 receptor, a member of the purinergic 2 receptor family, which is involved in inflammatory reactions, is expressed on the surface of RAW264 cells. Inflammatory cytokines, such as TNFα, and chemokines, such as MIP-1α and MCP-1, are produced by P2Y6 receptor binding and activation with extracellular nucleotides.Citation44 In addition, P2X4 receptors are expressed on lysosomes in RAW264 cells and may be related to phagocytosis.Citation45 The P2X7 receptor is activated by extracellular ATP and induces cell death by activating the P2X4 receptor.Citation46 In this study, although the highly dispersed FT9110 in PS did not show cytotoxicity, secretion of MIP-1α was significantly increased, and for cells treated with aggregated CNT, secretion of TNF was significantly increased when the FT9110 was dispersed in PS. Both highly dispersed FT9110 and aggregated CNT showed increased secretion of MIP-1α compared with that of the control; however, the amount was lower in the FBS group than in the control. This difference may be related to the dispersion state of the particles and the surface modifications induced by the dispersing agent or dispersion method, which are involved in different mechanisms of the inflammatory reaction. Moreover, the dispersion agent or method was also involved in the inhibition of signal transduction. MIP-1α is a chemokine that activates TNF,Citation47 and TNF has been reported to be related to carcinogenesis.Citation38,Citation48 In this study, highly dispersed FT9110 showed reduced uptake into cells and had little effect on cell proliferation, and secretion of inflammatory cytokines, such as MIP-1α, was observed. In addition, highly dispersed MWCNTs localized in the cytoplasm and inhibited cell division,Citation49 causing genomic instability and inducing DNA damage.Citation50 Although highly dispersed FT9110 does not exhibit cytotoxicity, cellular malignancy may be caused by abnormal cell division, DNA damage, excessive secretion of cytokines associated with carcinogenesis, and other related processes.
In summary, we found that FT9110 size, shape, and dispersion state, as well as the dispersant used, caused very different reactions with regard to cellular uptake, toxicity, and immune response. These differences are presumably due to the fact that the protein corona generated by the aggregation state of CNTs and dispersant may vary, and the receptors on which CNTs act differ depending on the complex conditions to ultimately affect cell responsiveness. More detailed study is required to clarify these mechanisms along with the carcinogenicity of CNTs with the aim of establishing CNTs as therapeutic biomaterials. Moreover, to apply CNTs as biomaterials, it will be necessary to evaluate the shapes and dispersion states of different particles, the dispersant used, and the optimal surface modifications for different purposes.
Conclusion
Highly dispersed and aggregated CNT showed different degrees of uptake into cells and localization in the cells, and the inflammatory cytokines expressed following the exposure to FT9110 varied greatly depending on the aggregation state of the particles and the dispersing agent. Evaluation of the incorporation of FT9110 and CNHs into the cells showed that highly dispersed FT9110 and aggregated CNT or CNHs were taken up intracellularly, although the mechanisms of incorporation varied. Thus, receptors related to intracellular uptake of individual particles and immune responses may differ. This study was performed in vitro; therefore, it is necessary to conduct animal experiments to evaluate CNT uptake and immune responses while considering complicated biological reactions. Because the cellular response varies depending on the state of aggregation and the dispersant used, even for the same type of material, further studies are necessary to clarify the effects of CNT shape, dispersion state, dispersants used, and surface modification to determine the carcinogenicity of CNTs and develop CNTs for clinical applications.
Acknowledgments
We would like to thank the staff at the Department of Instrumental Analysis at the Research Center for Supports to Advanced Science of Shinshu University for their assistance. We would like to thank Editage (www.editage.jp) for English language editing. This study was supported by the Japan Society for the Promotion of Science (JSPS) KAKENHI (grant nos 17H01584, 25350524, 25462365, and 24241045) and Japan Agency for Medical Research and Development (AMED) (grant no JP17km1009852). Chika Kuroda and Katsuya Ueda are co-first authors for this study.
Disclosure
The authors report no conflicts of interest in this work.
References
- de VolderMFTawfickSHBaughmanRHHartAJCarbon nanotubes: present and future commercial applicationsScience2013339611953553923372006
- ShvedovaAAKisinERPorterDMechanisms of pulmonary toxicity and medical applications of carbon nanotubes: Two faces of Janus?Pharmacol Ther2009121219220419103221
- SaitoNHaniuHUsuiYSafe clinical use of carbon nanotubes as innovative biomaterialsChem Rev2014114116040607924720563
- HartmanKBHamlinDKWilburDSWilsonLJ211AtCl@US-tube nanocapsules: a new concept in radiotherapeutic-agent designSmall2007391496149917668431
- RuggieroAVillaCHBanderEParadoxical glomerular filtration of carbon nanotubesProc Natl Acad Sci U S A201010727123691237420566862
- JohnstonHJHutchisonGRChristensenFMA critical review of the biological mechanisms underlying the in vivo and in vitro toxicity of carbon nanotubes: The contribution of physicochemical characteristicsNanotoxicology20104220724620795897
- SatoYYokoyamaANodasakaYLong-term biopersistence of tangled oxidized carbon nanotubes inside and outside macrophages in rat subcutaneous tissueSci Rep20133251623981952
- HussainSSangtianSAndersonSMInflammasome activation in airway epithelial cells after multi-walled carbon nanotube exposure mediates a profibrotic response in lung fibroblastsPart Fibre Toxicol2014112824915862
- ChenTNieHGaoXEpithelial-mesenchymal transition involved in pulmonary fibrosis induced by multi-walled carbon nanotubes via TGF-beta/Smad signaling pathwayToxicol Lett2014226215016224530353
- BrozPDixitVMInflammasomes: mechanism of assembly, regulation and signallingNat Rev Immunol201616740742027291964
- DonaldsonKMurphyFADuffinRPolandCAAsbestos, carbon nanotubes and the pleural mesothelium: a review of the hypothesis regarding the role of long fibre retention in the parietal pleura, inflammation and mesotheliomaPart Fibre Toxicol20107520307263
- BellucciSChiarettiMCucinaACarruGAChiarettiAIMultiwalled carbon nanotube buckypaper: toxicology and biological effects in vitro and in vivoNanomedicine20094553154019572819
- LuoMChenPWangJJThe cytotoxicity of oxidized multi-walled carbon nanotubes on macrophagesScience China Chemistry2016597918926
- SatoYYokoyamaAShibataKInfluence of length on cytotoxicity of multi-walled carbon nanotubes against human acute monocytic leukemia cell line THP-1 in vitro and subcutaneous tissue of rats in vivoMol Biosyst20051217618216880981
- GottardiRDouradinhaBCarbon nanotubes as a novel tool for vaccination against infectious diseases and cancerJ Nanobiotechnology2013113024025216
- PolandCADuffinRKinlochICarbon nanotubes introduced into the abdominal cavity of mice show asbestos-like pathogenicity in a pilot studyNat Nanotechnol20083742342818654567
- TakagiAHiroseANishimuraTInduction of mesothelioma in p53± mouse by intraperitoneal application of multi-wall carbon nanotubeJ Toxicol Sci200833110511618303189
- HaniuHSaitoNMatsudaYElucidation mechanism of different biological responses to multi-walled carbon nanotubes using four cell linesInt J Nanomedicine201163487349722267932
- NagaiHOkazakiYChewSHIntraperitoneal administration of tangled multiwalled carbon nanotubes of 15 nm in diameter does not induce mesothelial carcinogenesis in ratsPathol Int201363945746224200157
- RuanBJacobiAMUltrasonication effects on thermal and rheological properties of carbon nanotube suspensionsNanoscale Res Lett2012712722333487
- KurodaCHaniuHAjimaKThe Dispersion State of Tangled Multi-Walled Carbon Nanotubes Affects Their CytotoxicityNanomaterials2016611E21921928335347
- CuiHFVashistSKAl-RubeaanKLuongJHSheuFSInterfacing carbon nanotubes with living mammalian cells and cytotoxicity issuesChem Res Toxicol20102371131114720402485
- HaniuHMatsudaYTakeuchiKKimYAHayashiTEndoMProteomics-based safety evaluation of multi-walled carbon nanotubesToxicol Appl Pharmacol2010242325626219874835
- HaniuHSaitoNMatsudaYEffect of dispersants of multi-walled carbon nanotubes on cellular uptake and biological responsesInt J Nanomedicine201163295330722228997
- HorieMStoweMTabeiMDispersant affects the cellular influences of single-wall carbon nanotube: the role of CNT as carrier of dispersantsToxicol Mech Methods201323531532223343334
- ConnerSDSchmidSLRegulated portals of entry into the cellNature20034226927374412621426
- van BerloDWilhelmiVBootsAWApoptotic, inflammatory, and fibrogenic effects of two different types of multi-walled carbon nanotubes in mouse lungArch Toxicol20148891725173724664304
- OberdörsterGOberdörsterEOberdörsterJNanotoxicology: an emerging discipline evolving from studies of ultrafine particlesEnviron Health Perspect2005113782383916002369
- SiegristKJReynoldsSHKashonMLGenotoxicity of multi-walled carbon nanotubes at occupationally relevant dosesPart Fibre Toxicol201411624479647
- KongHZhangYLiYSize-dependent cytotoxicity of nanocarbon blacksInt J Mol Sci20131411225292254324240811
- HaniuHSaitoNMatsudaYBiological responses according to the shape and size of carbon nanotubes in BEAS-2B and MESO-1 cellsInt J Nanomedicine201491979199024790438
- ShannahanJHBrownJMChenRComparison of nanotube-protein corona composition in cell culture mediaSmall20139122171218123322550
- KannoSFuruyamaAHiranoSA murine scavenger receptor MARCO recognizes polystyrene nanoparticlesToxicol Sci200797239840617361018
- HiranoSFujitaniYFuruyamaAKannoSMacrophage receptor with collagenous structure (MARCO) is a dynamic adhesive molecule that enhances uptake of carbon nanotubes by CHO-K1 cellsToxicol Appl Pharmacol201225919610322209804
- NakayamaMMacrophage Recognition of Crystals and NanoparticlesFront Immunol2018910329434606
- FitzgeraldMLMooreKJFreemanMWReedGLLipopolysaccharide induces scavenger receptor A expression in mouse macrophages: a divergent response relative to human THP-1 monocyte/macrophagesJ Immunol200016452692270010679110
- WangXGuoJChenTMulti-walled carbon nanotubes induce apoptosis via mitochondrial pathway and scavenger receptorToxicol In Vitro201226679980622664788
- HiranoSKannoSFuruyamaAMulti-walled carbon nanotubes injure the plasma membrane of macrophagesToxicol Appl Pharmacol2008232224425118655803
- DumortierHWhen carbon nanotubes encounter the immune system: desirable and undesirable effectsAdv Drug Deliv Rev201365152120212624056183
- LiuDYangFXiongFGuNThe Smart Drug Delivery System and Its Clinical PotentialTheranostics2016691306132327375781
- MukherjeeSPBondarenkoOKohonenPMacrophage sensing of single-walled carbon nanotubes via Toll-like receptorsSci Rep201881111529348435
- TurabekovaMRasulevBTheodoreMJackmanJLeszczynskaDLeszczynskiJImmunotoxicity of nanoparticles: a computational study suggests that CNTs and C60 fullerenes might be recognized as pathogens by Toll-like receptorsNanoscale2014673488349524548972
- BoeynaemsJMCommuniDGonzalezNSRobayeBOverview of the P2 receptorsSemin Thromb Hemost200531213914915852217
- ZhangZWangZRenHP2Y(6) agonist uridine 5’-diphosphate promotes host defense against bacterial infection via monocyte chemoattractant protein-1-mediated monocytes/macrophages recruitmentJ Immunol201118695376538721444765
- QureshiOSParamasivamAYuJCMurrell-LagnadoRDRegulation of P2×4 receptors by lysosomal targeting, glycan protection and exocytosisJ Cell Sci2007120Pt 213838384917940064
- KawanoATsukimotoMNoguchiTInvolvement of P2×4 receptor in P2×7 receptor-dependent cell death of mouse macrophagesBiochem Biophys Res Commun2012419237438022349510
- DornerBGScheffoldARolphMSMIP-1alpha, MIP-1beta, RANTES, and ATAC/lymphotactin function together with IFN-gamma as type 1 cytokinesProc Natl Acad Sci U S A20029996181618611972057
- WuYZhouBPTNF-alpha/NF-kappaB/Snail pathway in cancer cell migration and invasionBr J Cancer2010102463964420087353
- AsakuraMSasakiTSugiyamaTGenotoxicity and cytotoxicity of multi-wall carbon nanotubes in cultured Chinese hamster lung cells in comparison with chrysotile A fibersJ Occup Health201052315516620379079
- PacurariMYinXJDingMOxidative and molecular interactions of multi-wall carbon nanotubes (MWCNT) in normal and malignant human mesothelial cellsNanotoxicology200823155170