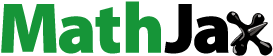
Abstract
Purpose
This study was aimed to develop doxorubicin-loaded quaternary ammonium palmitoyl glycol chitosan (DOX–GCPQ) nanoformulation that could enable DOX delivery and noninvasive monitoring of drug accumulation and biodistribution at tumor site utilizing self-florescent property of doxorubicin.
Materials and methods
DOX–GCPQ amphiphilic polymeric nanoformulations were prepared and optimized using artificial neural network (ANN) and characterized for surface morphology by atomic force microscopy, particle size with polydispersity index (PDI), and zeta potential by dynamic light scattering. Fourier transformed infrared (FTIR) and X-ray diffractometer studies were performed to examine drug polymer interaction. The ANN-optimized nanoformulation was investigated for in vitro release, cellular, tumor, and tissue uptake.
Results
The optimized DOX–GCPQ nanoformulation was anionic spherical micelles with the hydrodynamic particle size of 97.8±1.5 nm, the PDI of <0.3, the zeta potential of 28±2 mV, and the encapsulation efficiency of 80%±1.5%. Nanoformulation demonstrated a sustained release pattern over 48 h, assuming Weibull model. Fluorescence microscopy revealed higher uptake of DOX–GCPQ in human rhabdomyosarcoma (RD) cells as compared to free DOX. In vitro cytotoxicity assay indicated a significant cytotoxicity of DOX–GCPQ against RD cells as compared to DOX and blank GCPQ (P<0.05). DOX–GCPQ exhibited low IC50 (1.7±0.404 µmol) when compared to that of DOX (3.0±0.968 µmol). In skin tumor xenografts, optical imaging revealed significantly lower DOX–GCPQ in heart and liver (P<0.05) and accumulated mainly in tumor (P<0.05) as compared to other tissues.
Conclusion
The features of nanoformulation, ie, small particle size, sustained drug release, and enhanced cellular uptake, potential to target tumor passively coupled with the possibility of monitoring of tumor localization by optical imaging may make DOX–GCPQ an efficient nanotheranostic system.
Introduction
Doxorubicin (DOX), a member of anthracycline family, is an anticancer drug used in the treatment of breast, skin, and hepatocellular cancers, lymphomas, and solid tumors.Citation1,Citation2 Certain issues have been associated with DOX, for instance, after intravenous administration, it undergoes hydrolytic degradation in plasma and rapidly eliminated by enzymes.Citation3 Furthermore, despite having broad anticancer activity, the clinical use of DOX has been offset against its cardiotoxicity.Citation4 Several efforts have been directed to address the above issues of DOX. Recently, the US Food & Drug Administration has approved Doxil® (Janssen Products, LP., Horsham, PA, USA) and Myocet® (Sopherion Therapeutics, LLC, Princeton, NJ, USA), liposomal DOX nanoformulations, but they still pose toxicity problems.Citation5 Therefore, designing DOX delivery system is required to address the limitations associated with DOX and to further increase its antitumor efficacy. Several nanoparticulate-based DOX delivery systems include polymeric nanoparticles, lipid-based nanoparticles, inorganic nanoparticles, nanogels, nanotubes, and nanocrystals, which are in preclinical phase.Citation6–Citation10 Liposomal DOX uptake by reticuloendothelial system (RES) prevents its delivery at tumor site with reduced efficacy.Citation11,Citation12 Nanomedicine, an excipient-free system, is a promising approach to overcome nonselective distribution of chemotherapeutic drugs associated with conventional therapy.Citation13 Among the above delivery systems, the polymeric DOX nanoformulations are more promising in achieving enhanced efficacy with lesser side effects and reduced cytotoxicity by targeting DOX at specific site.Citation14,Citation15 Polymeric formulations also exhibit extended half-life and decreased uptake by liver and demonstrate enhanced permeability and retention (EPR) effect.Citation16
An amphiphilic polymer quaternary ammonium palmitoyl glycol chitosan (GCPQ) was used in the present study, which forms stable micelles as DOX carrier. GCPQ self-assembles at the neutral pH due to its 6-O glycol unit, which enables GCPQ to form micelles and is presented as low-viscosity dispersion (dynamic viscosity <5 mPa s). The GCPQ dispersion sustains its form as long as its concentration remains below 40 mg/mL. The above features make GCPQ favorable for injectable with expanded utility. In addition, GCPQ does not exhibit liver and spleen uptake.Citation17
The functionality of polymeric nanoparticles can be enhanced by the use of fluorescent ligand, thus making optical imaging possible for the distributed nanoparticles in tissues, organs, and tumors.Citation18 Optical imaging can monitor the biodistribution of nanomedicine noninvasively for early cancer detection and the monitoring of cancer therapy.Citation19 Fluorescent polymeric nanoparticles at cellular and organ levels have already been utilized for in vivo imaging of tumor tissues.Citation20 DOX’s auto-fluorescence features (such as florescence absorption and excitation wavelengths of 480 and 590 nm, respectively) enable the monitoring of its bio-distribution to tissues by optical imaging in addition to its anticancer activity, which has made it attractive for cancer research.Citation21,Citation22 Hence, the aim of present study was to prepare DOX–GCPQ as dual property optical drug delivery system (ODDS) without the use of chemical cross-linkers such as glutaraldehydeCitation23 and ionic gelation agents, ie, sodium tripolyphosphate, to minimize the side effects associated with excipients.Citation24 ODDS could deliver DOX at tumor site without nonspecific uptake by heart, liver, and RES and monitored for tissue uptake and tumor accumulation through in vivo optical imaging using a skin tumor model.
Materials and methods
Materials
Glycol chitosan (GC) (G7753), sodium iodide (383112), methyl iodide (67692), sodium bicarbonate (792519), palmitic acid N-hydroxysuccinimide (P1162), N-methyl-2-pyrrolidone (1437202), PBS tablets (P 4417), cholesterol (C8667), pyrene (185515), acetone (65050), DOX (44583), 7,12-dimethylbenz(a)anthracene (DMBA) (D3254), 12-O-tet-radecanoylphorbol 13-acetate (TPA) (P1585), and solvents were supplied by Sigma-Aldrich Co. (St Louis, MO, USA). Sephadex G-25 was purchased from GE Healthcare Bio-Sciences AB (Uppsala, Sweden). Human rhabdomyosarcoma (RD) cells ATCC CCL 136 were received as gift courtesy from National Institute of Health (NIH) (Islamabad, Pakistan). The use of RD cells was approved by Institutional Ethical Committee, University College of Pharmacy, University of Punjab (protocol no HEC/PUCP/1943A-Dated 10-4-16).
Animals
Balb/c mice of age 7–9 weeks and weight 24–28 g purchased from NIH were used for this study. Prior to study, an ethical approval was obtained from the Animal Ethics Committee, University College of Pharmacy, University of Punjab (protocol no AEC/PUCP/1059A-dated 28-5-16). All the experiments in animals in this work were undertaken in accordance with the International Conference on Harmonization ICH guidelines.Citation25
Methods
Synthesis and characterization of nanocarrier
GCPQ was synthesized by the acid degradation of GC followed by covalent coupling of palmitic acid N-hydroxysuccinimide (palmitoylation), and quaternization was achieved by methylation with methyl iodide using a previously reported quaternization method.Citation26,Citation27 Briefly, 500 mg of hydrolyzed GC and 376 mg of sodium bicarbonate added in flask already containing water:ethanol solution (76:24 mL). Palmitic acid N-hydroxysuccinimide solution was added in GC and sodium bicarbonate solution under continuous stirring for 72 h. Product was separated via the evaporation of ethanol and redispersed in distilled water. This mixture was extracted three times with ample diethyl ether to remove any excess of palmitic acid N-hydroxysuccinimide. The mixture was dialyzed (molecular weight cutoff 12–14 kDa) against 5 L of distilled water for 24 h with six changes. The dialysate was freeze dried. Quaternization was carried out by dispersing the palmitoyl GC (300 mg) in 25 mL of N-methyl-2 pyrrolidone for 12 h. Ethanolic solution (5 mL) was prepared by dissolving sodium hydroxide (40 mg) and sodium iodide (45 mg) and added to palmitoyl GC solution. Finally, methyl iodide (1 g) was added and kept under nitrogen gas for 3 h at 36°C in dark. Diethyl ether was used to precipitate quaternary ammonium product and was washed twice with the mixture of diethyl ether and absolute ethanol and redispersed in water. The dialysate (iodide salt) was purified through Amberlite-96 resins. Sodium chloride (0.1 M) and sodium bicarbonate (0.01 M) were utilized for the dialysis of clear eluent up to 4.5 h with three changes. Afterward, 5 L of water was used for extensive dialysis for 24 h with six changes. Finally, GCPQ was obtained as white cotton-like solid after freeze drying. The level of modification of GCPQ after palmitoylation and quaternization was confirmed by NMRCitation27 (400 MHz on Bruker AMX spectrometer; Bruker AXS Inc., Madison, WI, USA). GCPQ polymer was used for the preparation of DOX nanoformulations.
Preparation of DOX–GCPQ nanoformulations
Fixed amount of GCPQ polymer (5 mg/mL) was loaded with varying concentrations of DOX ranging from 2 to 6 mg/mL according to in normal saline by using ultrasonic probe-sonicator (Vibra Cell; Sonics, Newtown, CT, USA) operating at varying amplitudes and times as shown in . For sonication, a probe of diameter 6 mm was put in the middle of the light-protected 2 mL sample placed in ice and sonicated at pulse mode of 50 s on and 10 s off positions.
Table 1 Composition and operating conditions for formulation of DOX–GCPQ
Characterization of DOX nanoformulation
Measurement of size, PDI, and zeta potential
Hydrodynamic diameter, polydispersity index (PDI) value and zeta potential of drug loaded nanoformulations were analyzed by dynamic light scattering (DLS). Analysis was done in triplicate at 25°C (Microtrac Nanotrac Wave II, USA). Sample for atomic force microscopy (AFM) analysis was prepared by drying nanoformulation at 25°C for period of two days in desiccator containing dried silica gel. Surface morphology was studied by acquiring the images on digital AFM instrument at non-contact mode (Alpha Contec, Germany) equipped with silicon cantilever of 7 nm in 5/5 µm scan area size.
Encapsulation efficiency
Nonencapsulated DOX from nanoformulation (DOX– GCPQ) was separated by size exclusion chromatography using Sephadex column G-25. Encapsulation efficiency was calculated according to the following equation:
Calibration curve of DOX was constructed, and its encapsulation efficiency was calculated by measuring the absorbance of both DOX–GCPQ and DOX at 485 nm using UV–Vis spectrophotometer (Model U-2900; Hitachi Ltd., Tokyo, Japan).
Optimization of DOX nanoformulations
The data of properties for five nanoformulations were used for formulation optimization, using artificial neural network (ANN) approach by employing Neural-Power, Ver 3.1 (CPC-X Software, USA). For employing ANN-based optimization, drug concentration, polymer amount, sonication amplitude, and sonication time were taken as the input parameters, while size, PDI, zeta potential, loading efficiency, and their respective SD were measured as outputs using ANN architecture ().
Figure 1 Architect of artificial network employed for the optimization of DOX formulation using GCPQ.
Abbreviations: DOX, doxorubicin; GCPQ, quaternary ammonium palmitoyl glycol chitosan.
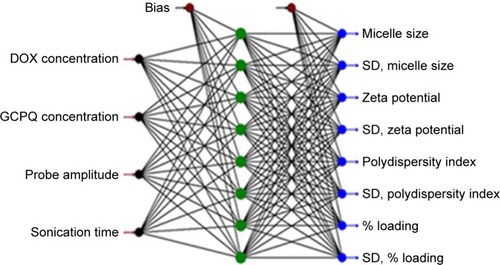
Quick propagation method was used with Tanh as transfer function. The input, output, and hidden layer were set as 4, 8, and 1, respectively, with the number of nodes 8. The aspired criteria for output responses were used as follows: size <100 nm, PDI <1, zeta potential ≥25 mV, and drug loading 80% while SD of all above outputs was set as minimum (ie, 0). Based on the desirability levels of the outputs, ANN approach yields forecasted composition and conditions for the optimized formulation, also with forecasted levels of outputs.Citation18 ANN-generated forecasted inputs (composition and conditions) were used to produce a confirmation formulation which was characterized again.
Characterization of optimized (confirmation) formulation
The optimized formulation was characterized for size, PDI, zeta potential, surface morphology, and percentage of drug loading using the same procedures stated earlier. The optimized nanoformulation was subjected to the additional in vitro and in vivo tests such as Fourier transformed infrared (FTIR), X-ray diffractometer (XRD), in vitro drug release, cellular uptake, in vitro cytotoxicity, and in vivo tumor and tissue uptake studies.
FTIR and XRD studies
Drug–polymer interaction for the optimized DOX nanoformulation was studied through FTIR spectroscopy. FTIR spectra were recorded in mid-IR range (4,000−400/cm using FTIR Spectrophotometer, equipped with software OMNIC™ Version 6.0 a; Thermo Fisher Scientific, Waltham, MA, USA). FTIR spectra of DOX, polymer (GCPQ), and optimized DOX–GCPQ nanoformulation were compared with each other to find the interaction of drug with polymer. Freeze-dried DOX–GCPQ nanoformulation was subjected to XRD studies. Encapsulation of drug-loaded polymeric nanoformulation, its crystallographic properties, and physical state was analyzed by X-ray diffractometer (Bruker AXS Inc.). For XRD, 2ϑ angle was measured from 0 to 1,000°C at the scanning speed of 2°C/min and X-ray radiation source was copper at 40 kV with 40 mA.
In vitro drug release studies
DOX release from optimized DOX–GCPQ was determined by dialysis membrane diffusion technique. Nanoformulation (1 mL) was dialyzed against 200 mL of dissolution medium, PBS, pH 7.4, using the dialysis membrane (molecular weight cutoff 12 kDa). Release pattern was compared with the DOX solution. Studies were conducted at 37°C±0.5°C with continuous stirring at 50 rpm for 48 h. Samples were collected at predetermined time intervals, ie, at 10 min, and then on 0.5, 1.0, 1.5, 2.0, 2.5, 3.0, 4.0, 6.0, 8.0, 12.0, 16.0, 20.0, 24.0, and 48.0 h for the determination of drug concentration by UV–Vis spectrophotometer (Model U-2900) at 485 nm.Citation28 Kinetic modeling of drug release was assessed by the DD-Solver software by entering the percent release data of DOX and DOX–GCPQ. Different release kinetics models () were employed, the best model was selected based on the highest value of coefficient of determination (R2), and in case of a close R2 value for two models, the lowest value of Akaike information criterion (AIC) was used. To understand the release mechanism, n value for DOX–GCPQ was computed using the Korsmeyer–Peppas model.
Table 2 DOX–GCPQ release kinetics models employed in study
The dissimilarity factor (f1) and similarity factor (f2) were also computed between the free and optimized DOX nanoformulation with the equation given.Citation29
Cellular uptake studies
Utilizing DOX self-fluorescent property with excitation wavelength 480 and 560 nm, cellular uptake of the optimized DOX–GCPQ nanoformulation was analyzed by fluorescence microscopy. Human RD cells were seeded at the density of 1.0×105 cells/well in six-well plate (Corning Incorporated, Corning, NY, USA) and allowed to grow for 24 h. Three wells of cells were exposed for each DOX and DOX–GCPQ for 6 h, and experiment was conducted in triplicate. Cells, washed thrice with PBS, were imaged with fluorescence microscopy using GFP filter (Evos® FL Cell Imaging System; Thermo Fisher Scientific). The untreated cells served as control.Citation31
Determination of in vitro cytotoxicity and IC50
Cytotoxicity of the optimized DOX–GCPQ nanoformulation was evaluated by the MTT assay against RD cell line. Cells, 1.0×104/well, were seeded in 96-well plate (Corning Incorporated) and allowed to grow by incubating for 24 h at 37°C in 5% CO2 at 85% humidity incubator (Model NU 5700; Nuaire, Plymouth, MA, USA). After 24 h, the culture medium was removed and, to calculate IC50, three wells of cells were incubated with concentrations ranging from 0.01, 0.08, 0.40, 0.80, 1.30, 1.70, 2.10, 2.50, 3.0, 3.40, 3.80, and 4.30 µmol of GCPQ, DOX, and DOX–GCPQ for 24, 48, and 72 h at 37°C. The drug solution and formulation were removed, MTT was added in each well, and cells were incubated for 4 h at 37°C. The solution was aspirated, and the resulting formazan crystals were dissolved in dimethyl sulfoxide. Microplate reader (Model FL ×800; Biotek, Winooski, VA, USA) was used to measure absorbance at 485 nm.Citation21,Citation32 Cytotoxicity was expressed as percentage of cell viability compared to untreated control cells. IC50 was calculated using the regression analysis implemented in GraphPad Prism Ver 7.Citation33
Tumor and tissue uptake studies using optical imaging technique
Induction of tumor in Balb/c mice
Prior to tumor uptake study, tumor was induced in Balb/c mice using a reported method.Citation34 Briefly, in tumor initiation phase, 0.2 mL of 25 nmol solution of DMBA was applied at the dorsal skin of Balb/c mice. After 2 weeks, 1.7, 3.4, and 6.8 nmol of 12-O-tetradecanoylphorbol 13-acetate (TPA) in 0.2 mL of acetone were applied to mice for tumor promotion up to 5 weeks. Mice were monitored for the development of papillomas.
Administration of DOX and DOX–GCPQ nanoformulation to mice
After 10 days of tumor implantation, when tumor volume of mice reached 50 mm3, mice were divided into two groups (n=6). Group I and Group II were dosed with DOX and DOX–GCPQ nanoformulations at the final concentration of 6 mg/kgCitation35–Citation37 via tail vein. Mice were sacrificed after 24 h of dose administration. Tumor and vital organs including liver, kidney, heart, and spleen were harvested for tumor and tissue uptake studies by fluorescence imaging.
Fluorescence imaging
The fluorescence intensity of the ANN-optimized DOX– GCPQ nanoformulation in tumor and the excised organs (heart, liver, spleen, and kidney) were examined using the iBox Explorer2 (iBox® ExplorerCitation2 Imaging Microscope; UVP Ltd., Cambridge, UK). System was set on 535/45 excitation filter and 605/50 emission filter with the automated Bio-Lite™ MultiSpectral Light Source. Images were taken at 0.17× magnification keeping intensity at 6 using the 3.2 MP OptiChemi 610 camera, Vision Works® LS Acquisition, and analysis software was used to study images.
Statistical analysis
All the experiments were conducted in triplicate, and data were presented as mean ± SD or in the form specified otherwise. In vitro cytotoxicity, as measured by the percentage of cell viability, of free DOX, DOX–GCPQ, and GCPQ at different concentrations was compared using two-way ANOVA. Similarly, the fluorescence intensity between DOX and DOX–GCPQ treatments within different organs was also compared using two-way ANOVA with GraphPad Prism 7.0. A P-value of <0.05 was considered statistically significant difference.
Results
GCPQ characteristics
The GCPQ synthesized in this study demonstrated palmitoylation and quarternization levels of 34.2%±5.188% and 8.23%±2.4%, respectively. Similarly, NMR also showed all peaks as (CD3OD, D2O-9:1) δ=0.89–0.90 (CH3 [palmitoyl]), δ=1.30 (CH2 [palmitoyl]), δ=1.65 (CH2 [palmitoyl β to amino group]), δ=2.05 (CH3 [acetyl-glycol chitosan]), δ=2.2–2.4 (CH2 [palmitoyl α to amino group]), δ=2.7–3.2 (proton at C2 on the sugar ring and CH3-N [dimethyl-amino-glycol chitosan]), δ=3.45 (CH3-N+ [trimethyl-amino-glycol chitosan]), δ=3.5–4.5 (sugar protons), δ=3.30 (methanol protons), δ=4.5–5.0 (water protons), and δ=5.0 (CH [C1 sugar proton, GC]) ().
Characteristics of nanoformulations
Hydrodynamic particle size of DOX–GCPQ nanoformulations with zeta potential, PDI measured by nanosizer, and percentage of loading is given in .
Table 3 Characteristics of the doxorubicin nanoformulations
The data given in were analyzed using Neural-Power, ver 3.1. The combined relative effects of probe amplitude, concentration of drug, and sonication time were shown to be 31.88, 28.14, and 29.79%, respectively, on size, size distribution, zeta potential, and loading efficiency, while the contribution of polymer concentration for nanoformulation properties was only 10.18%, as shown in .
Figure 3 Percentage effect of input factors on DOX–GCPQ formulation.
Abbreviations: DOX, doxorubicin; GCPQ, quaternary ammonium palmitoyl glycol chitosan.
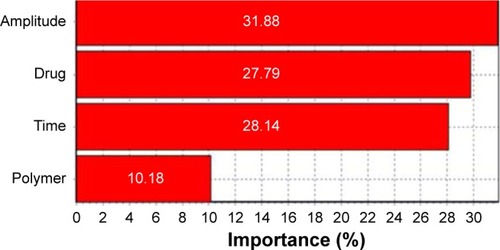
According to desirability criteria, size <100 nm with PDI <1, zeta potential ≥25 mV, and loading 80%, the forecasted optimized levels of the factors along with the predicted properties, generated by Neural Power®, are given in . Optimized composition 1 in (drug-to-polymer ratio of 6:5 mg), sonication amplitude of 60% for 10 min was selected for the preparation and characterization of a validation DOX–GCPQ formulation.
Table 4 Forecasted optimized compositions of the formulations factors and predicted responses based on optimized compositions and conditions
Response surface plots of outputs (properties/responses) for combined effect of inputs were generated, and the ones with paramount effect have been given herein (). The response surface plots supported the effects as revealed in .
Figure 4 Response surface plots of the combined effect of (A) amplitude of sonication and drug concentration on size, zeta potential, PDI, and loading along with SD and (B) amplitude of sonication and time on size, zeta potential, PDI, and loading along with SD.
Abbreviation: PDI, polydispersity index.
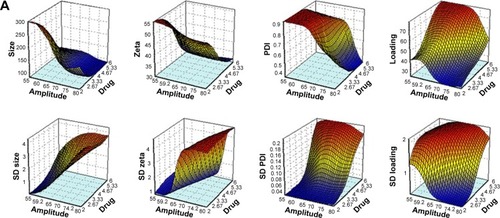
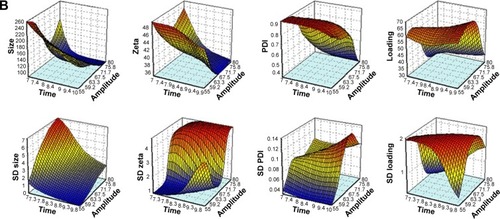
Hydrodynamic particle size of the optimized DOX– GCPQ nanoformulation prepared using the forecasted optimized levels of formulative ingredients and process conditions was 97.8±1.5 nm with the zeta potential of −28±2 mV and the PDI of <0.3 measured by nanosizer. The AFM showed that DOX–GCPQ particles were compact, spherical having smooth surface with an average diameter of 73.3 nm (). The properties of the validation (optimization) formulation were comparable to the aspired as well as the ANN predicted properties of Set 1 ().
FTIR spectra
The spectrum of DOX indicated characteristics peaks/bands at 3,560/cm (stretch, alcohol), 3,311.6/cm (OH and NH, stretch), 2,894.3/cm (CH stretch, aromatic), 1,730/cm (C=O stretch, ketone), 1,630/cm (C=O stretch), 1,282.7/cm (C–O–C, stretch), 1,114/cm (C–O stretch, tertiary alcohol), 1,070/cm (C–O stretch, secondary alcohol), and 988/cm (C–O stretch, primary alcohol). In contrast, the spectrum of DOX–GCPQ indicated that peaks/bands at 3,560/cm (stretch, alcohol), 1,114/cm (tertiary alcohol), 1,070/cm (secondary alcohol), and 988/cm (primary alcohol) were disappeared and the peak corresponding OH and NH was broadened. The band of CH stretch remained intact whereas the peak intensity of carbonyl group was reduced as shown in .
XRD spectra of DOX and DOX–GCPQ nanoformulation
XRD spectra of DOX and DOX–GCPQ nanoformulation () showed that the peaks were obtained at the 2θ of 11.45, 13.12, 18.76, 22.45, 25.42, 31.24, and 38.94 for crystalline DOX, whereas these peaks disappeared in case of DOX–GCPQ nanoformulation, based on which the DOX in nanoformulation was considered to be amorphous. Furthermore, it supported a successful encapsulation of DOX inside polymer.
DOX–GCPQ in vitro release kinetics
As shown in , approximately all of free DOX (100%) diffused out of dialysis membrane into buffer within 10 h. DOX release rate from DOX–GCPQ nanoformulation was considerably slow at pH 7.4. Only 35% of drug came out in the medium within 24 h (). Drug release from nanoformulation followed Weibull model based on R2 and AIC values as shown in . The parameters of Weibull model, ie, a and b, were found to be 12.34 and 0.63, respectively. The n value, based on Korsmeyer–Peppas was 0.59.
Table 5 In vitro drug release data modeling of DOX–GCPQ nanoformulation against DOX
Cellular uptake of DOX–GCPQ
After 6 h incubation of RD cells against DOX and DOX– GCPQ, fluorescence images () showed an enhanced fluorescence intensity of DOX–GCPQ as compared to free DOX.
Figure 9 Cellular uptake and intracellular localization of DOX and DOX–GCPQ nanoformulations in human RD cells observed by fluorescence microscopy of (A) untreated cells, (B) fluorescence of internalized DOX inside RD cells, and (C) fluorescence of internalized DOX–GCPQ inside RD cells.
Note: Magnification 40×.
Abbreviations: DOX, doxorubicin; GCPQ, quaternary ammonium palmitoyl glycol chitosan; RD, rhabdomyosarcoma.
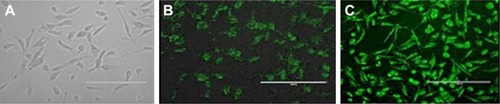
In vitro cytotoxicity
DOX and DOX–GCPQ nanoformulations showed concentration-dependent toxicity at 24 h ( and ). DOX–GCPQ nanoformulation indicated significant cytotoxic effect after 24 h to RD cell as compared to DOX and GCPQ alone (P<0.05). The IC50 value of DOX was found to be 3.0±0.968 µmol as compared to 1.7±0.404 µmol of DOX– GCPQ nanoformulation at 24 h.
Figure 10 In vitro cytotoxicity DOX and DOX–GCPQ nanoformulation in human RD cells observed by inverted microscope after 24 h of incubation.
Notes: (A) Untreated RD cells. (B) Cell viability with DOX. (C) Cell viability with DOX–GCPQ. Magnification 40×.
Abbreviations: DOX, doxorubicin; GCPQ, quaternary ammonium palmitoyl glycol chitosan; RD, rhabdomyosarcoma.
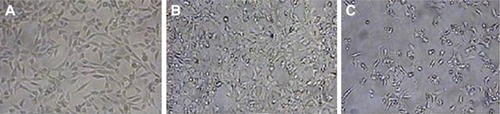
Biodistribution
Free DOX showed nonsignificantly higher (P>0.05) uptake in liver as compared to DOX–GCPQ. In heart and spleen, the biodistribution of DOX–GCPQ was significantly lower (P<0.05) as compared to free DOX. DOX–GCPQ with high fluorescent signal accumulated in tumor tissue in comparison to the other organs such as heart and liver (). When tumor uptake of DOX–GCPQ was compared to that of all organs, significantly higher distribution was noted (P<0.05), except for the kidney (P>0.05).
Figure 12 Biodistribution of intravenously injected DOX and DOX–GCPQ in skin tumor bearing mice.
Notes: (A) Representative fluorescent images of tumor and excised organs 24 h after injection. (B) Quantitative fluorescent intensity of excised organ and tumor (P<0.05). ***P<0.0001.
Abbreviations: DOX, doxorubicin; GCPQ, quaternary ammonium palmitoyl glycol chitosan.
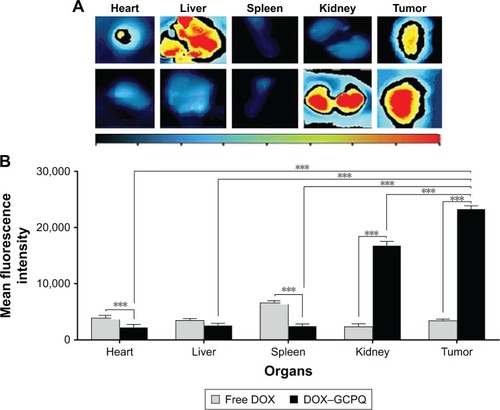
Discussion
Currently, there is a need of developing such anticancer therapy, which can successfully deliver the anticancer drugs to the tumors, as well as simultaneously enable noninvasive assessment of therapy outcome by monitoring its tumor localization/biodistribution.Citation38 The multifunctional nanoparticles attached with fluorescent ligand or containing a self-fluorescent drug can serve this purposes.Citation39 The present study was aimed to develop DOX–GCPQ nanoformulation that could enable drug delivery and noninvasive monitoring of tumor accumulation and biodistribution, utilizing self-florescent property of DOX. For this purpose, hydrophobically modified amphiphilic GC (GCPQ) was synthesized by the acid degradation of GC, followed by covalent coupling of palmitic acid called palmitoylation and quarternization of GC as reported.Citation26,Citation27 The palmitoylation level of 34.2%±5.188% was a reason for negative charge, while the quaternization level of 8.23%±2.4% was a reason for positive charge. The synthesized GCPQ was able to self-assemble at low critical micelle concentration (CMC) into particles of colloidal size that also indicated its colloidal stability.Citation40 DOX was loaded into GCPQ by probe sonication. To achieve the optimized GCPQ nanoparticle with desired features such as size <100 nm, PDI value <1, zeta potential ≥25 mV, and loading 80%, parameters such as drug amount, polymer amount, sonication speed, and the sonication parameters were manipulated since these factors have reported to affect the size of the nanoparticles.Citation41 ANN provides relative importance, as percentage contribution of different inputs for overall properties,Citation42 which along with response surface plots help in finding the optimum levels of the inputs. In this study, the relative effects of the amount of drug, probe amplitude, and sonication time demonstrated their profound influence on the properties of the nanoformulations. The previous findings have reported the probe amplitude and sonication time as the critical factors for the properties of nanoformulation. However, the amount of polymer, in this study, affected blandly contrarily to a previous report where the polymer was reported to be critical for the properties of nanoparticles.Citation41
Applicability of ANN, particularly with the sparse data has already been demonstrated.Citation43 In the present study, the above fact was supported as optimization was achievable even with a smaller number of experimentation (ie, merely with merely with five formulations). Based on the factors and response data, ANN generated five sets of predicted levels of the factors and sonication conditions for optimized nanoparticles. Set 1 given in was selected as the composition and sonication conditions for the development of optimized DOX nanoformulation. According to set 1, the amplitude of 57.50% was round off to 60%. The optimized (validated) formulation was characterized in terms of particle size, PDI, zeta potential, percentage of drug loading, morphology, FTIR, XRD, in vitro release kinetics, cellular uptake, in vitro cytotoxicity, and biodistribution. The properties of the optimized formulation were in close agreement to the predicted properties ().
The average size of optimized DOX–GCPQ nano-formulation was observed to be 73 nm by AFM, may be smaller than that measured by nanosizer probably due to shrinkage and collapse of particle occurred during sample preparation.Citation44 Hydrodynamic size measured by nanosizer is of swollen particles in solution, which gives the diameter of nanoparticle with the liquid layer around the particle while AFM images particles, which are spread and dried on surface.Citation45 Thus, the hydrodynamic diameter is larger than the actual diameter of nanoparticle measured by other techniques where particle size is determined for the dried sample.Citation46 The zeta charge on particle was −28 mV, the PDI was <0.3, and loading was 80%. The particles were compact and spherical with smooth surface, and presented homogeneous population.
In the current study, the comparative FTIR of DOX and DOX–GCPQ indicated involvement of alcoholic OH groups of DOX in hydrogen bonding with the GCPQ polymer. The decrease in peak intensity of carbonyl and ether groups in FTIR also indicated hydrogen bonding due to the polarity of such groups and the H-bonding between GCPQ and DOX resulted in stable nanoformulation. FTIR analysis also showed alteration in chemical structure of DOX in nanoformulation, suggesting altered state of drug from crystalline (when free) to amorphous form (when in nanoformulation). This amorphous state of DOX in nanoformulation was also supported by XRD study. XRD spectra showed that the peaks observed for crystalline DOX were disappeared in case of DOX–GCPQ nanoformulation.Citation47 This observation also supported a successful encapsulation of DOX inside polymer.
All the drug (100%) was available in the dissolution media from free DOX in 10 h, which was consistent with the previous findings.Citation48 DOX release from GCPQ was studied up to 48 h in accordance with the previous studies. The DOX release for GCPQ nanocarrier was found to be 35% until up to 48 h in this study, which was in line with the previous studies where the release was noted around 40%–50%.Citation49 In vitro release study of DOX from DOX–GCPQ nanoformulation at pH 7.4 demonstrated pattern comparable to that of the slow release since polymeric nano-delivery systems, which were designed to deliver the drug at target site via blood circulation with longer circulation time and lesser drug leakage resulted in enhanced targeting of drug with enhanced efficacy.Citation48 Kinetic modeling of release kinetics demonstrated that DOX–GCPQ nanoformulation followed Weibull model. The b value in Weibull model is indicative of drug release and determines the progression of the shape of dissolution curve. The value of b>1 produces sigmoidal release curve with a turning point in the initial phase, b<1 produces steeper increase, and b=1 presents exponential rise in the release curve.Citation30,Citation48 In this study, the b value, 0.636 led to a steeper increase in the drug release curve without a sigmoid portion. Diffusion exponential value (n) of Korsmeyer–Peppas equation was used to determine drug release mechanism. Values of n between 0 and 0.5 indicate that release is Fickan diffusion, and values between 0.5 and 1 indicate anomalous release due to the coupling of diffusion and erosion.Citation50 The n value of 0.59 supported a sustained drug release mechanism, which could be attributed to the hydrogen bonding between DOX and GCPQ polymer in the nanoformulation. When the release profiles of free DOX and DOX–GCPQ nanoformulation were compared, the values of dissimilarity factor (f1), 282.37, and similarity factor (f2), 14.82, were beyond the stipulated f1 and f2 values, ie, 0–15 and 50–100, respectively, to declare the similar release profiles.Citation51
Enhanced in vitro cellular uptake of DOX–GCPQ nanoformulation as compared to the free DOX was observed that could be attributed to several reasons. The mean particle size of the present anionic DOX–GCPQ nanoformulation was below 100 nm, ie, was 73 nm, thus showing enhanced in vitro cellular uptake. Enhanced DOX–GCPQ internalization should present higher cytotoxicity secondary to caveolae-mediated endocytosis followed by the anionic DOX nanoformulation in comparison to the simple diffusion followed by free DOX.Citation52 In caveolae-mediated endocytosis, nanoparticles are not substrate for degradative action of lyso-someCitation53 unlike when they follow simple diffusion, thus resulting into a better accumulation of therapeutic moiety at target site with enhanced efficacy.Citation30 In the caveolae-dependent endocytic pathway, the caveolae 3 isoform, a muscle-specific protein,Citation53 is responsible for more efficient internalization of nanomaterial as compared to simple diffusion.Citation53 Furthermore, the anionic nanoformulation may be endocytosed through interaction with positive sites of proteins in membrane and highly captured by cells due to its repulsive interaction with negatively charged cell surface as reported earlier.Citation54 Besides the above factors, the size of nanoparticle less than 100 nm also shows the efficient nanoformulation-mediated endocytosis by cells.Citation55 Cytotoxicity experiments were conducted at 24, 48, and 72 h of incubation of cells with all concentrations of DOX and DOX–GCPQ.Citation56,Citation57 Since the best cytotoxic results were obtained after 48 h, this time was selected to calculate IC50. The in vitro interaction of DOX–GCPQ nanoformulation with RD cell line showed significant cytotoxic effect as compared to free DOX and GCPQ polymer alone (P<0.05) due to more internal localization of DOX–GCPQ. Thus, DOX–GCPQ showed lower IC50 value demonstrating its cytotoxic effect at lower dose when given as DOX–GCPQ as compared to DOX alone.
The particle size and the anionic nature of the DOX– GCPQ nanoformulation impart the colloidal stability and also refrain it from interacting with mononuclear phagocytic system, up-taken nonspecifically by phagocytic system and opsonization, thus leading to improved circulation time and nonspecific distribution in healthy tissue.Citation58 Higher colloidal stability and kinetically low dissociation allow the DOX– GCPQ nanoformulation to circulate in the blood for longer time and thus, significantly increase its accumulation at the target site due to enhanced permeation by passive targeting and retention effect.Citation40 Furthermore, slow drug release from nanoformulation is advantageous to localize cytotoxic drug to tumor site.Citation48 Henceforth, DOX–GCPQ nanoformulation showed significant improvement in tumor accumulation as compared to the free DOX. The localization of drug in heart, liver, and spleen was significantly lower, in accordance with reported previously where GCPQ’s intravenous distribution kinetics avoided uptake by liver and spleen.Citation17 However, besides tumor, in the present study, the DOX nanoformulation was also significantly accumulated in kidney as compared to free DOX whose primary route of metabolism is liver.Citation59 Observation of higher concentration of DOX–GCPQ in kidney might be ascribed to a changed biodistribution pattern or excretion of DOX–GCPQ via urinary route instead of biliary route reported for free DOX excretion,Citation59 though confirmation is required through detailed biodistribution and elimination studies, respectively. Furthermore, it warrants the assessment of organ-specific toxicity of the developed formulation.
DOX encapsulation in biocompatible nano-drug delivery dosage form while effectively targeting anticancer drug to tumor both actively and passively can minimize its adverse effects,Citation60 particularly the cardiotoxicity by virtue of its lower accumulation in heart. Controlled drug delivery system with smooth drug plasma levels over longer period of time also reduces side effects and enhances efficacy leading to better patient compliance.Citation61
The significantly enhanced accumulation of DOX–GCPQ in tumor site has been associated with higher fluorescence signal than in other organs as compared to free drug. This method of fluorescence measurement is qualitative and semiquantitative, which presents relative uptake by organs as reported earlier.Citation8,Citation39,Citation44,Citation62 The developed DOX–GCPQ nanoformulation has demonstrated simultaneous possibility of an in vivo localization in the tumor site and optical imaging of the tumor; thus, it can be explored for its theranostic potential after detailed kinetics and in vivo imaging studies.
Conclusion
DOX–GCPQ amphiphilic polymeric nanoformulation showed accumulation in skin tumor bearing mice by optical imaging. The study enables noninvasive evaluation and in vivo biodistribution of doxorubicin nanoformulation via fluorescence imaging. The enhanced cellular uptake, desirable small particle size, sustained drug release, and ability to target tumor site passively may make it efficient nanotheranostic system.
Acknowledgments
This work is funded by PAK-NORWAY Institutional Cooperation Program, PK3004, COMSTECH-TWAS project Reference number (12-198 RG/PHA/AS_C-UNESCO FR: 3240270874) and Pakistan Science Foundation, PSF/Res/C-NILOP/Med (330). The authors are thankful to Prof Ijeoma Uchegbu and Prof Andreas G Schatzlein, School of Pharmacy, University College London, for their analytical support in the synthesis and characterization of GCPQ.
Disclosure
The authors report no conflicts of interest in this work.
References
- LeeVHRobinsonJRControlled Drug Delivery: Fundamentals and ApplicationsNew YorkMarcel Dekker1987
- DeepaKSinghaSPandaTDoxorubicin nanoconjugatesJ Nanosci Nanotechnol201414189290424730306
- DanesiRFogliSGennariAContePDel TaccaMPharmacokinetic-pharmacodynamic relationships of the anthracycline anticancer drugsClin Pharmacokinet200241643144412074691
- OutomuroDGranaDRAzzatoFMileiJAdriamycin-induced myocardial toxicity: new solutions for an old problem?Int J Cardiol2007117161516863672
- HilgerRARichlyHGrubertMPharmacokinetics (PK) of a liposomal encapsulated fraction containing doxorubicin and of doxorubicin released from the liposomal capsule after intravenous infusion of Caelyx/DoxilInt J Clin Pharmacol Ther2005431258858916372528
- BharaliDJMousaSAEmerging nanomedicines for early cancer detection and improved treatment: current perspective and future promisePharmacol Ther2010128232433520705093
- LevensonVBiomarkers for early detection of breast cancer: what, when, and where?Biochim Biophys Acta Gen Subj200717706847856
- ChenHZhenZToddTChuPKXieJNanoparticles for improving cancer diagnosisMater Sci Eng R Rep2013743356924068857
- ChidambaramMManavalanRKathiresanKNanotherapeutics to overcome conventional cancer chemotherapy limitationsJ Pharm Pharm Sci2011141677721501554
- WadajkarASMenonJUTsaiYSProstate cancer-specific thermo-responsive polymer-coated iron oxide nanoparticlesBiomaterials201334143618362523419645
- PapahadjopoulosDAllenTMGabizonASterically stabilized liposomes: improvements in pharmacokinetics and antitumor therapeutic efficacyProc Natl Acad Sci U S A1991882411460114641763060
- LasicDDMartinFJGabizonAHuangSKPapahadjopoulosDSterically stabilized liposomes: a hypothesis on the molecular origin of the extended circulation timesBiochim Biophys Acta1991107011871921751525
- LiuYMiyoshiHNakamuraMNanomedicine for drug delivery and imaging: a promising avenue for cancer therapy and diagnosis using targeted functional nanoparticlesInt J Cancer2007120122527253717390371
- SlingerlandMGuchelaarHJGelderblomHLiposomal drug formulations in cancer therapy: 15 years along the roadDrug Discov Today2012173–416016621983329
- MasoodFPolymeric nanoparticles for targeted drug delivery system for cancer therapyMater Sci Eng C2016601569578
- EmamiJRezazadehMHasanzadehFDevelopment and in vitro/in vivo evaluation of a novel targeted polymeric micelle for delivery of paclitaxelInt J Biol Macromol201580294026093319
- LalatsaASchätzleinAGMazzaMLeTBUchegbuIFAmphiphilic poly(L-amino acids) – new materials for drug deliveryJ Control Release2012161252353622613882
- KimJLeeJELeeSHDesigned fabrication of a multifunctional polymer nanomedical platform for simultaneous cancer-targeted imaging and magnetically guided drug deliveryAdv Mater2008203478483
- KunjachanSEhlingJStormGKiesslingFLammersTNoninvasive imaging of nanomedicines and nanotheranostics: principles, progress, and prospectsChem Rev201511519109071093726166537
- VollrathASchubertSSchubertUSFluorescence imaging of cancer tissue based on metal-free polymeric nanoparticles – a reviewJ Mater Chem B201311519942007
- NguyenHNHoangTMNMaiTTTEnhanced cellular uptake and cytotoxicity of folate decorated doxorubicin loaded PLA-TPGS nanoparticlesAdv Nat Sci Nanosci Nanotechnol201562025005
- LiXHirshDJCabral-LillyDDoxorubicin physical state in solution and inside liposomes loaded via a pH gradientBiochim Biophys Acta19981415123409858673
- ThanooBCSunnyMCJayakrishnanACross-linked chitosan micro-spheres: preparation and evaluation as a matrix for the controlled release of pharmaceuticalsJ Pharm Pharmacol19924442832861355537
- ShiraishiSImaiTOtagiriMControlled release of indomethacin by chitosan-polyelectrolyte complex: optimization and in vivo/in vitro evaluationJ Control Release1993253217225
- ICH [homepage on the Internet]2018 Available from: http://www.ich.org/products/guidelines/safety/article/safety-guidelines.htmlAccessed September 13, 2018
- RazaAde la FuenteMUchegbuIFSchätzleinAModified glycol chitosan nanocarriers carry hydrophobic materials into tumoursPaper presented at: Nanotechnology 2010: Bio Sensors, Instruments, Medical, Environment and Energy-Technical Proceedings of the 2010 NSTI Nanotechnology Conference and Expo, NSTI-Nanotech2010
- UchegbuIFSadiqLArastooMQuaternary ammonium palmitoyl glycol chitosan – a new polysoap for drug deliveryInt J Pharm20012241–218519911472828
- JavedIHussainSZUllahISynthesis, characterization and evaluation of lecithin-based nanocarriers for the enhanced pharmacological and oral pharmacokinetic profile of amphotericin BJ Mater Chem B201534283598365
- LokhandwalaHDeshpandeADeshpandeSKinetic modeling and dissolution profiles comparison: an overviewInt J Pharm Bio Sci201341728773
- KouLSunJZhaiYHeZThe endocytosis and intracellular fate of nanomedicines: Implication for rational designAsian J Pharm Sci201381110
- LiFZhengCXinJEnhanced tumor delivery and antitumor response of doxorubicin-loaded albumin nanoparticles formulated based on a Schiff baseInt J Nanomedicine201611387527574421
- WangLZhangJSongMA shell-crosslinked polymeric micelle system for pH/redox dual stimuli-triggered DOX on-demand release and enhanced antitumor activityColloids Surf B Biointerfaces201715211128063272
- MosmannTRapid colorimetric assay for cellular growth and survival: application to proliferation and cytotoxicity assaysJ Immunol Methods1983651–255636606682
- AbelELAngelJMKiguchiKDigiovanniJMulti-stage chemical carcinogenesis in mouse skin: fundamentals and applicationsNat Protoc2009491350136219713956
- JinZHJinMJJiangCGEvaluation of doxorubicin-loaded pH-sensitive polymeric micelle release from tumor blood vessels and anticancer efficacy using a dorsal skin-fold window chamber modelActa Pharmacol Sin201435683984524902790
- AndersCKAdamoBKarginovaOPharmacokinetics and efficacy of PEGylated liposomal doxorubicin in an intracranial model of breast cancerPLoS One201385e6135923650496
- GustafsonDLMerzALLongMEPharmacokinetics of combined doxorubicin and paclitaxel in miceCancer Lett2005220216116915766591
- SajjaHKEastMPMaoHWangYANieSYangLDevelopment of multifunctional nanoparticles for targeted drug delivery and noninvasive imaging of therapeutic effectCurr Drug Discov Technol200961435119275541
- ShalviriACaiPRauthAMHendersonJTWuXYEvaluation of new bi-functional terpolymeric nanoparticles for simultaneous in vivo optical imaging and chemotherapy of breast cancerDrug Deliv Transl Res20122643745325787324
- UchegbuIFCarlosMMcKayCHouXSchätzleinAGChitosan amphiphiles provide new drug delivery opportunitiesPolym Int201463711451153
- Esmaeilzadeh-GharedaghiEFaramarziMAAminiMARouholamini NajafabadiARezayatSMAmaniAEffects of processing parameters on particle size of ultrasound prepared chitosan nanoparticles: an Artificial Neural Networks StudyPharm Dev Technol201217563864722681416
- Agatonovic-KustrinSBeresfordRBasic concepts of artificial neural network (ANN) modeling and its application in pharmaceutical researchJ Pharm Biomed Anal200022571772710815714
- BukhariNIJuliantoTValente PereiraREComputer-aided prediction of cefotaxime sodium stability in aqueous solution at different pH from sparse dataLatin Am J Pharm2018373571578
- ZhangJSunYTianBMultifunctional mesoporous silica nanoparticles modified with tumor-shedable hyaluronic acid as carriers for doxorubicinColloids Surf B Biointerfaces201614429330227107383
- QiJYaoPHeFYuCHuangCNanoparticles with dextran/chitosan shell and BSA/chitosan core-Doxorubicin loading and deliveryInt J Pharm20103931–2177185
- EatonPQuaresmaPSoaresCA direct comparison of experimental methods to measure dimensions of synthetic nanoparticlesUltramicroscopy201718217919028692935
- SharmaRBisenDShuklaUSharmaBX-ray diffraction: a powerful method of characterizing nanomaterialsRecent Res Sci Technol2012487779
- LiuGJinQLiuXLvLChenCJiJBiocompatible vesicles based on PEO-b-PMPC/α-cyclodextrin inclusion complexes for drug deliverySoft Matter201172662669
- YuanAWuJSongCA novel self-assembly albumin nanocarrier for reducing doxorubicin-mediated cardiotoxicityJ Pharm Sci201310251626163523423631
- RamtekeKDighePKharatAPatilSMathematical models of drug dissolution: a reviewSch Acad J Pharm201435388396
- Osei-AsareCKipoSOfori-KwakyeKBoakye-GyasiMComparative in vitro dissolution of commercially available sustained release nifedipine tablet brands in the Kumasi Metropolis, GhanaJ Appl Pharm Sci20155460
- BannunahAMVllasaliuDLordJStolnikSMechanisms of nanoparticle internalization and transport across an intestinal epithelial cell model: effect of size and surface chargeMol Pharm201411124363437325327847
- BenmerahALamazeCClathrin-coated pits: vive la différence?Traffic20078897098217547704
- YeungTGilbertGEShiJSilviusJKapusAGrinsteinSMembrane phosphatidylserine regulates surface charge and protein localizationScience2008319586021021318187657
- SinghRLillardJWNanoparticle-based targeted drug deliveryExp Mol Pathol200986321522319186176
- Shafiu KambaAIsmailMTengku IbrahimTAZakariaZABHassan GusauLIn vitro ultrastructural changes of MCF-7 for metastasise bone cancer and induction of apoptosis via mitochondrial cytochrome C released by CaCO3/Dox nanocrystalsBiomed Res Int201420143114
- CaoNFengSSDoxorubicin conjugated to D-alpha-tocopheryl polyethylene glycol 1000 succinate (TPGS): conjugation chemistry, characterization, in vitro and in vivo evaluationBiomaterials200829283856386518606445
- BlancoEShenHFerrariMPrinciples of nanoparticle design for overcoming biological barriers to drug deliveryNat Biotechnol201533994195126348965
- WangYMeiXYuanJLuWLiBXuDTaurine zinc solid dispersions attenuate doxorubicin-induced hepatotoxicity and cardiotoxicity in ratsToxicol Appl Pharmacol2015289111126335259
- PiktelENiemirowiczKWątekMWollnyTDeptułaPBuckiRRecent insights in nanotechnology-based drugs and formulations designed for effective anti-cancer therapyJ Nanobiotechnology20161413927229857
- JayakumarRNairARejinoldNSMayaSNairSVDoxorubicin-loaded pH-responsive chitin nanogels for drug delivery to cancer cellsCarbohydr Polym201287323522356
- HwangJYParkJKangBJMultimodality imaging in vivo for preclinical assessment of tumor-targeted doxorubicin nanoparticlesPLoS One201274e3446322509306