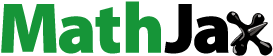
Abstract
The set of nanoelectromechanical systems (NEMS) based on relative motion of carbon nanotubes walls is proposed for use in medical nanorobots. This set includes electromechanical nanothermometer, jet nanoengine, nanosyringe (the last can be used simultaneously as nanoprobe for individual biological molecules and drug nanodeliver). Principal schemes of these NEMS are considered. Operational characteristics of nanothermometer are analyzed. The possible methods of these NEMS actuation are considered. The present-day progress in nanotechnology techniques which are necessary for assembling of NEMS under consideration is discussed. Biocompatibility of carbon nanotubes is analyzed in connection with perspectives of their application in nanomedicine.
Introduction
The considerable progress has been achieved in nanoscience in the last decades. On the one hand the techniques for industrial production of a wide set of nanomaterials have been elaborated. On the other hand the manipulation with nanometer size objects has been demonstrated (see, for example, CitationCumings and Zettl 2000) and principal schemes of nanoelectromechanical systems (NEMS) have been considered (see, for review CitationKottas et al 2005). This progress opens the door to the possibility of application of nanosystems in nanomedicine, particularly in medical nanorobots. Two main problems should be solved to select nanoobjects which are perspective for such applications. Firstly, the design of NEMS which can be used in medical nanorobots should be elaborated. Secondly, the biocompatibility of nanoobjects which are movable elements of this NEMS should be studied.
Essential characteristics of nanoobjects are a very small feature size in the range of 1–100 nanometers (nm). One nanometer spans 3–5 atoms lined up in a row. As one of the most promising nanoobjects, carbon nanotubes (CitationIijima 1991) can be mentioned, which can occur in a variety of modifications (eg, single- or multi-walled, filled or surface modified). Carbon nanotubes are expected to find a broad field of applications in nanoelectronics (logics, data storage or wiring, as well as cold electron sources for flat panel displays and microwave amplifiers), and also as fillers for nanocomposites for materials with special properties.
The arbitrary (CitationYu et al 2000a, Citation2000b) and controlled by a nanomanipulator (CitationCumings and Zettl 2000) relative motion of walls in multi-wall carbon nanotubes allows to consider them as promising candidates for movable components of NEMS. Carbon nanotubes have a set of unique electronic and mechanical properties. For example, th values of both the Young modulus (see for example, CitationYu et al 2000a) and the bending elastic modulus (CitationDai et al 1996) of carbon nanotubes is about 1 TPA, five times greater than the steel. The walls of carbon nanotubes can be metallic or semiconducting depending on wall structure controlled by chiral indexes (CitationSaito et al 1992). Possible nanodevices based on such motion have been proposed elsewhere: constant-force nanosprings and free sliding telescopic arms (CitationCumings and A. Zettl 2000), nanogears (CitationSrivastava 1997), ultra-small switching devices (CitationForro 2000), gigahertz oscillator (CitationZheng and Jiang 2002; CitationZheng et al 2002), and perforating nanodrill (CitationLozovik et al 2003a; CitationLozovik et al 2003b; CitationLozovik and Popov 2004). Moreover the first nanotube-based nanomotor has been realized recently by Fennimore et al (2003).
Here we propose the principal schemes of the following carbon nanotube based NEMS for medical nanorobots:, nanosyringe (which can be used simultaneously as nanoprobe for study of single biological molecules and drug nanodeliver) and jet nanoengine.
Calculations show that conductance of double-wall carbon nanotubes (DWNTs) depends on the length of an overlap of the walls for telescopic DWNTs (CitationKim and Chang 2002) and the relative position of the walls within the unit cell of a DWNT for both telescopic (CitationHanson and Stafström 2003) and shuttle (CitationHanson and Stafström 2003; CitationGrace et al 2004) DWNTs. The measurement of individual carbon nanotube conductance has been experimentally achieved (see, for example, CitationTans et al 1997). Thus these electronic properties of DWNTs opens up the possibility to elaborate NEMS based on the relative motion of walls in DWNTs. For example, variable nanoresistor (CitationLozovik et al 2003a; CitationLozovik et al 2003b; CitationLozovik and Popov 2004) and strain nanosensor (CitationBichoutskaia et al 2006a) based on DWNT have been proposed. Here we use these electronic properties to propose electromechanical nanothermometer that can be used in medical nanorobots.
The chemical inertness of carbon nanotubes allows them to have a quite low toxicity (except possible inhalation toxicity). However it is necessary to take care of purification of nanotubes from metal catalyst and graphite particles that have been shown to be responsible, at least in part, for the toxicity of carbon nanotubes preparations (CitationFiorito et al 2006). Conductivity and mechanical characteristics of nanotubes, as well as chemical inertness and their high aspect ratio, make them a material of choice for medical nanodevices. At present, carbon nanotubes can be produced by different methods and are already available commercially.
The present paper presents the discussion of carbon nanotube biocompatibility studies; gives the description of schemes and operation of electromechanical nanodevices that can be used in nanomedicine and possible ways of these electromechanical nanodevices actuation: by a nanomanipulator, by use of electric field, or by use of magnetic field. The present-day progress in nanotechnology techniques that are necessary for assembling of NEMS based on relative motion of carbon nanotubes walls is also discussed.
Biocompatibility of carbon nanotubes
A potential building material for medical nanodevices is the graphene sheet which can form carbon nanotubes. Carbon fullerenes and nanotubes can be used for sensors or manipulators of medical nanorobots. They can also be used to create electrically conductive polymers and tissue engineering constructs with the capacity to provide controlled electrical stimulation (CitationPolizu et al 2005).
Because of the great interest in using engineered nanomaterials for medical applications, there is some information relevant for assessing health effects reported in this area of literature. Carbon nanostructures are the only class of engineered nanomaterials that have received focused toxicological characterization.
In regard to carbon nanotubes, inhalation toxicity has been the initial concern. CitationHuczko et al (2001) studied the effects of Carbon nanotubes containing soot on guinea pigs intratracheally instilled, by evaluating pulmonary function parameters and bronchoalveolar lavage examination. They showed that after 4 weeks, a single intratracheal instillation of 25 mg Carbon nanotubes containing soot, did not change pulmonary function and did not induce any measurable inflammation in bronchoalver space in the exposed guinea pigs. CitationWarheit and co-workers (2004) evaluated the acute lung toxicity of intratracheally instilled single-wall carbon nanotubes. Exposure to high doses (5 mg/kg) single-walled nanotubes (SWNTs) produced mortality in ∼15% of the instilled rats within 24h postinstillation. This mortality resulted from mechanical blockage of the upper airways by the instillate and was not due to inherent pulmonary toxicity of the instilled SWNTs particulate. In the other animals, the intratracheal instillation exposure to SWNTs produced only a short term, inflammatory and cell injury effects as evidenced by a transient increase in the percentage of bronchoalveolar-recovered neutrophils, enzymes, cell proliferation rates at 24h post exposure, but these values were not sustained through the other post-exposure time periods. The lung histophatology study showed a nondose-dependent series of multifocal granulomas with nonuniform distribution and not progressive beyond 1 month post-exposure. In another study by CitationLam and colleagues (2004) 23 mice were intratracheally instilled with 0, 0.1, 0.5 mg of carbon nanotubes, a carbon black negative control and a quartz positive control and euthanized 7 and 90 days for histopathological studies of the lungs. All nanotubes preparations induced dose-dependent epithelioid granulomas in the lungs and interstitial inflammation in the animals of the 7 days group. These lesions persisted and were more pronounced in the 90 days group. The lungs of some animals also revealed peribronchial inflammation and necrosis that were extended to the alveolar septa. The lungs of mice treated with carbon black were normal, whereas those treated with high-dose quartz revealed mild to moderate inflammation. The authors conclude that, if Carbon nanotubes reach the lungs, they are much more toxic than carbon black and can be more toxic than quartz. A study performed in human volunteers and in animals in order to assess the skin sensitivity to Carbon nanotubes showed that soot containing a high content of Carbon nanotubes did not induce any skin irritation in fourty people subjected to a dermatological testing, nor eyes irritation in rabbits instilled with the soot (CitationHuczko and Lange 2001). CitationShvedova and coworkers (2003) investigated adverse effects of SWNTs using a cell culture of immortalized human epidermal keratinocytes. After 18 hours of exposure they observed formation of free radicals, accumulation of peroxidative products, antioxidant depletion (decrease of intracellular levels of glutathione), oxidation of protein SH groups, and depletion of total antioxidant reserve and vitamin E. Additionally they observed loss of cell viability as well as ultrastructural and morphological changes in cultured skin cells. The researchers concluded that SWNTs exposure can result in accelerated oxidative stress and may produce dermal toxicity in exposed workers. Others (CitationMonteiro-Riviere et al 2005) explored the effects of multi-walled-nanotubes (MWNTs) on human epidermal keratinocytes by examining the cell uptake of these particles by transmission electron microscopy. They observed that chemically unmodified MWNTs were present within cytoplasmic vacuoles and that induced the release of the proinflammatory cytokine interleukine 8 from the keratinocytes in a time dependent manner. Furthermore, CitationSalvador-Morales and co-workers (2006) studied the interaction between MWNTs and double-walled-nanotubes and a part of the human immune system, the complement cascade. The authors conducted haemolytic assays to investigate the activation of the human serum complement system via the classical and alternative pathways. They observed that carbon nanotubes activate human complement via both classical and alternative pathways and concluded that carbon nanotubes may promote damaging effects of excessive complement activation, such as inflammation and granuloma formation.
The effects induced by both C60 fullerenes and SWNTs on human macrophage cells have been also recently explored by CitationFiorito and co-workers (2006). The authors observed that either C60 fullerenes, very highly purified carbon nanostructures, and SWNTs, when very highly purified, induce apoptosis and cell death in a smaller percentage of cells compared to graphite particles, and do not activate macrophage cells to produce inflammatory molecules. These results suggest that SWNTs, when very highly purified and not containing graphite particles, could have a less inflammatory potential towards human macrophages and be less toxic against these cells
Besides the aforementioned work of CitationFiorito et al (2006), the only direct study on carbon nanotube cytocompatibility was by CitationMattson et al (2000) who grew embryonic rat-brain neurons on multiwalled carbon nanotubes. They reported that on unmodified nanotubes, neurons extend only one or two neurites, which exhibit very few branches. In contrast, neurons grown on nanotubes coated with 4-hydroxynonenal (a bioactive molecule) elaborate multiple neuritis which exhibit extensive branching. This result was said to establish the feasibility of using nanotubes as substrates for nerve cell growth and as probes of neuronal function at the nanometer scale (CitationPolizu et al 2005).
Carbon nanotubes have been derivatized with, among other chemical groups, benzyne, thionychloride and octadecylamine. CitationNimmagadda and McFetridge (2004) argued that by integrating SWNT into composite biomaterials, conductivity can be modulated to potentially enhance cardiac tissue remodeling. Three different SWNT preparations were analyzed: as purchased (AP-NT), purified (PUR-NT) and functionalized with glucosamine (GA-NT), over a range of concentrations 0.001–1.0% (wt/vol). This study showed that SWNT functionalized with glucosamine have considerably higher cell viability than “as purchased” or “purified” preparations at equivalent concentrations. Being hydrophilic with improved dispersion, GA-NT holds promise in tissue engineering applications where tissue conductivity may play a significant role in tissue development.
At present, some emerging concepts of carbon-nanotoxicology can be identified from the above exposed data: toxicity of C-nanoparticles depends on several different factors such as their size, their shape, the surface characteristics, the amount of the substances present in the particles preparations. Some issues concerning nanomaterials toxicity are still to be elucidated, nevertheless the possible risks deriving from carbon based nanotools application appear to be a minor problem in comparison with the potential benefits that these new materials can have in the biomedical field.
Nanoelectromechanical systems for medical nanorobots: schemes and operation
Electromechanical nanothermometer
With a considerable progress achieved in recent years in nanotechnology techniques, workable designs of NEMS have been evolved in which elements of electric circuits represent nanoobjects of progressively smaller scale reaching the size of a single molecule. The operation of a number of such NEMS is based on changes in physical characteristics of a system due to the change in the relative position of its components (CitationIsacsson and Nord 2004). In the present paper, a concept of an electromechanical nanothermometer based on the interaction and relative motion of the walls of DWNTs is considered (CitationBichoutskaia et al 2006b). It rests on the measurements of the conductivity of the walls of a DWNT which, under certain conditions, depends on the relative positions of the walls. In these cases, the total conductivity changes significantly with the temperature due to the thermal vibrations of the walls.
Two cases for overlap of the walls are possible: a shuttle DWNT, with equal lengths of the wall overlap and of the short wall and a telescopic DWNT, with the length of the overlap which is less than the length of the short wall. The schemes of the telescopic nanothermometer with the movable inner wall and the shuttle nanothermometer with the movable outer wall are shown on , respectively. The telescopic nanothermometer with the movable outer wall and the shuttle nanothermometer with the movable inner wall can be also possible. The principle of these nanothermometers operation is the following. At zero temperature the relative positions of the walls correspond to the ground state of the system. With increase of temperature other relative positions of the walls become available due to the thermal relative vibration of the walls. In this case the probability p(z) of any wall relative displacement z is proportional to exp (=U(z)/kT) (for the (6,6)@(11,11) DWNT used below as example the dependence of the interwall interaction energy U and conductance G on the angle φ is negligible). Then the total conductance Gtot(T) of the system depends on temperature and can be found by the following expression
Figure 1 The principal schemes of possible electromechanical nanothermometers: the movable wall (1) and the fixed wall (2) with the attached electrode (3). A) telescopic nanothermometer with the movable inner wall, B) shuttle nanothermometer with the movable outer wall.
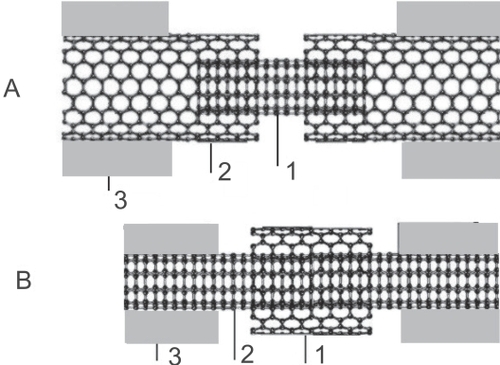
First experimental measurements of temperature dependence of resistance of SWNTs have been recently reported in (CitationGao et al 2005). These measurements show that the resistance of SWNTs does not change for temperatures above 80K. Calculations of the dependence of conductance G of the shuttle (6,6)@(11,11) DWNT with fixed relative positions of the walls on temperature T show a weak dependence (CitationBichoutskaia et al 2006b). Simultaneously the dependences of the conductance G (CitationGrace et al 2004), and the interwall interaction energy U (CitationBichoutskaia et al 2006a, Citation2006b, Citation2006c) on the displacement z of walls along the axis of DWNT have considerable oscillations. The weak dependence G(T) for fixed relative positions of the walls simultaneously with oscillations in dependencies U(z) and G(z) allows us to propose DWNT-based electromechanical nanothermometer.
Since there is nonzero probability p(zmax), where zmax corresponds to maxima of U(z), the diffusion of the shuttle along the long wall is possible. Such diffusion is the process which can suppresses the adequate operation of the nanothermometer. We have calculated the possible minimal size of the nanothermometer for wich this diffusion is negligible on the example of shuttle scheme (see ). Let us place the shuttle in the center of the long wall between electrodes. For adequate operation of the nanothermometer the average displacement d of the shuttle in the result of diffusion during the time t of the nanothermometer operation should be less than the distance L between the electrode and the shuttle.
Thus we obtain
We use EquationEq. (7)(7) to calculate the total length of nanothermometer that operates at average during 100 years at room temperature T = 300 K without failure connecting with relative diffusion of walls and obtain that ltot is about 40 nm or about 150 DWNT unit cells, that is l≈ltot (since ltot is logarithmic function of the parameter a, ltot is approximately the same for the cases of inner and outer movable walls). Note that this value of ltot correspond to the range used at ab initio calculation of the conductance of telescopic and shuttle (6,6)@(11,11) DWNTs (CitationGrace et al 2004). In the case of telescopic scheme of the nanothermometer the length of the gap between the fixed walls should be added to the total length ltot. Thus the proposed nanothermometer can be really of nanometer size and its total size can be determined by the length which is necessary to attach electrodes.
We propose the nanothermometer which can be used for accurate temperature measurement in spatially localized regions with sizes of hundred nanometers. The very small size of proposed electromechanical nanothermometer makes it to be very promising for application in medical nanorobots. The nanothermometer can be calibrated with the use of thermocouple, and since the temperature measurement is based on conductance measurement, the same order of accuracy as in the case of thermocouple using can be achieved.
The fundamentally different scheme of nanotube based nanothermometer was proposed and realized recently (CitationGao and Bando 2002; CitationGao et al 2003). In that scheme the temperature measurement based on measurement thermal expansion of column of liquid gallium inside a nanotube. The change of Ga-column length was observed in situ with the use of a high-vacuum TEM (CitationGao and Bando 2002) or by postmeasurement TEM reading of the length of a Ga oxide layer mark visible inside nanotube (CitationGao et al 2003). Both these techniques need in a high-vacuum TEM and therefore can not been used in medical nanorobots.
Jet nanoengine
The relative motion of core consisting of inner walls of nanotube and shell consisting of outer walls of MWNT has been realized experimentally with the help of nanomanipulator (CitationCumings and Zettl 2000). The simulations show the possibility of fluids flow inside carbon nanotubes (CitationTuzun et al 1996, Citation1997; CitationKang and Hwang 2004a). The experiment shows the internal wetting by water of carbon nanotubes with open ends (CitationBarber et al 2005). Nanopump was recently proposed, in which the core motion is used for inflow and ejection of liquid inside nanotube (that is core plays the role of a piston) (CitationKang and Hwang 2004a). We propose here that the ejection of liquid can be used for propulsion of nanorobot in this liquid ie, nanosize analog of jet engine can be made of MWNT. Several schemes of the jet nanoengine are shown on . On one side piston stroke a water is sucked through the hole into the nanoengine body made of outer wall of MWNT. On another side piston stroke a water is ejected through the nozzle made of inner wall of MWNT. Possible ways of the nanoengine actuation are discussed below. Note that the gap between the plunger and the shell (the interwall distance) is 3.4 Å. Thus the proposed jet nanoengine excludes the possibility that water can penetrate inside medical nanorobot.
Figure 2 The principal schemes of the jet nanoengines. The nanoengine is composed of the nozzle made of the inner nanotube wall with small diameter (1), the medium walls (2) which serve to hold the nozzle, the body (3) made of the outer wall with the hole (4), and the piston made of another nanotube (5). Several ways of piston actuation are possible: (A) by attached nanomanipulator (6), (B) by a magnetic field acting on the magnetic core of the piston, (C) by electric field acting on encapsulated ions, (D) by electric field acting on dipole produced by atoms or molecules adsorbed on the open edge of the walls constituting the piston, by electric field acting on dipole induced by this field in the piston, (6) is the control electrode.
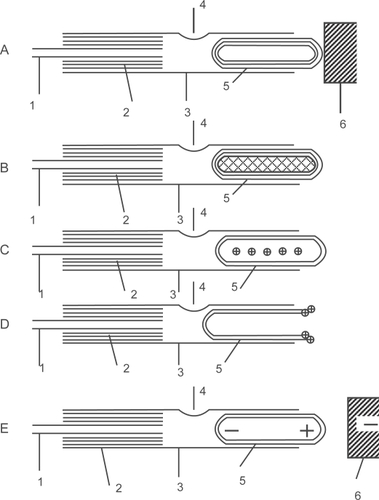
Drug nanodeliver
Not only simple fluids but also large polymeric molecules such as linear polyethylene (CitationWei and Srivastava 2003) and DNA (CitationLau et al 2005) can be adsorbed inside carbon nanotubes. We believe that molecules of drug can be adsorbed inside carbon nanotubes. In this case the long outer wall of MWNT can be used as container with molecules of drug adsorbed inside it and the short core of inner walls can be used as piston for ejection of these molecules from the container. Therefore a nanodeliver made of MWNT can be designed. Several schemes of such nanodelivers corresponding to various ways of piston actuation by electric or magnetic field are shown on . We believe that the source of electric or magnetic field can be not only outside an organism, but also medical nanorobot can be used for local actuation of some of the nanodeliveres preliminarily placed inside organism.
Figure 3 The principal schemes of drug nanodelivers. (A), (B) and (C) The nanodeliveres consisting of a container (1) made of the outer wall of the nanotube, a piston (2) made of the inner walls of the nanotube, and a drug (3). Several ways of piston actuation are possible: by a magnetic field acting on the magnetic core of the piston (A), by electric field acting on encapsulated ions (B) or dipoles produced by atoms or molecules adsorbed on the open edge of the walls constituting the piston (C). (D) The nanodeliver which delivers a drug as result of its diffusion.
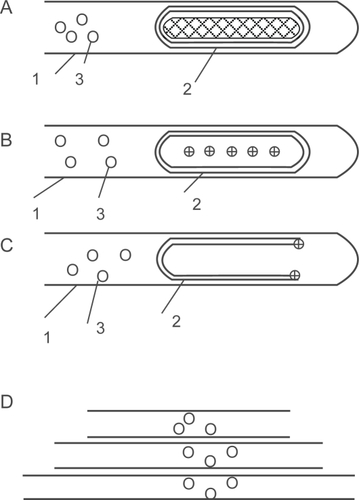
Recent molecular dynamics simulations show the possibility of clusters (CitationHwang et al 2004) and polymeric molecules (CitationWei and Srivastava 2003) diffusion inside carbon nanotube. Therefore the delivery of large biological molecules stored inside a nanotube can occur in result of diffusion of this molecules inside nanotube and subsequent desorption of this molecule from the open end of the nanotube. Evidently that both the diffusion and desorption are random processes. So the delivery of a drug with a desirable time dependence for a one nanotube is not possible. However the charger of hundreds such nanotubes may be used as drug nanodeliver for continuous delivery of set of different molecules with the desirable time dependence (See ). Such a nanodeliver can be transported in vivo at certain place by a medical nanorobot.
Nanosyringe and nanoprobe
In principal the relative motion of all pairs of walls of MWNT is possible. We propose that the many-walled nanotube can be used as also a telescopic nanosyringe, where outer wall of the nanotube is fixed, the motion of the medium wall relative to the outer wall can be used for penetration (that is the medium wall plays the role of the nanosyringe needle) and the motion of the core of inner walls relative to the medium wall can be used to eject molecules adsorbed inside the medium wall (that is the core of inner walls plays the role of the nanosyringe piston). In such a way the delivery of a few number of molecules of drug with nanometer scale accuracy can be possible.
Carbon nanotubes are successfully used as the probe tips of the scanning tunneling microscope (STM) (CitationDai et al 1996) and the atomic force microscope (AFM) (CitationDai et al 1996; CitationWong et al 1998a; CitationWong et al 1998b; CitationNagy et al 1998; CitationHaflier et al 1999; CitationJarvis et al 2000). Note that because of small diameter (1–10 nm) and high aspect ratio the use of carbon nanotube tip allows to study deeper features of nanoobjects (CitationDai et al 1996; CitationWong et al 1998b; CitationNagy et al 1998) and to achieve higher resolution than in the case of the use of conventional silicon tips (CitationWong et al 1998b; CitationNagy et al 1998). Both these advantages of carbon nanotubes tips were demonstrated on example of biological systems (CitationWong et al 1998b). Moreover nanotubes elastically buckle if applied force exceeds critical force 10 nN (CitationDai et al 1996). Therefore the use of carbon nanotubes tips can prevent a damage of delicate organic and biological nanoobjects. We propose that the needle of the described above telescopic nanosyringe can serve simultaneously as a probe of STM or AFM and thus it can be used to determine the place where the molecules of drug adsorbed inside the needle should be delivered. The other usage of this device combining scanning microscope probe and telescopic nanosyringe can be also possible. Firstly telescopic carbon nanotube can be used as probe to reveal the molecule and to study its properties in vivo and after that this nanotube can be used to capture the molecule. Note that molecular dynamics simulation shows the insertion of long polymeric molecules inside carbon nanotubes in the result of Van der Waals interaction (CitationWei and Srivastava 2003). Possible schemes of the nanosyringe and the nanoprobe are shown on .
Figure 4 The principal schemes of electromechanical nanodevices which can operate as a nanosyringe or a nanoprobe. The nanodevice is composed of the fixed outer wall (1) of the nanotube, the movable medium wall (2) which serves as the needle of the nanosyringe or the nanoprobe and contains a drug (3) and the piston (4) made of the inner walls of the nanotube. Two ways of piston and needle actuation are shown: by attached nanomanipulator (A), by magnetic field acting on the magnetic core of the piston (B).
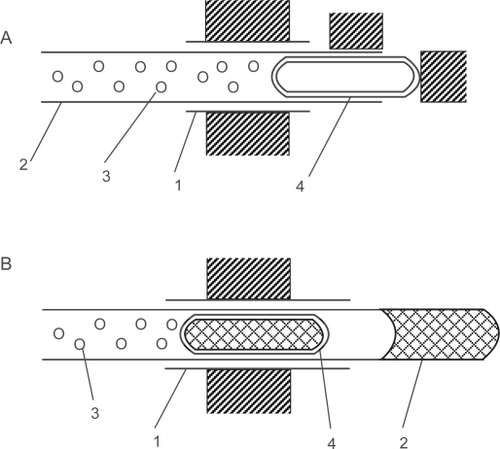
Actuation of nanotube-based NEMS
Let us discuss now possible ways of actuation of the proposed nanotube-based NEMS. In principal three methods are possible: i) the direct actuation with the help of a nanomanipulator, ii) the actuation with the help of electric field, iii) the actuation with the help of magnetic field. Besides the actuation and orientation of nanotube by external electromagnetic field is possible (this problem will be discussed in detail in another place).
The controlled by a nanomanipulator relative motion of walls in MNWNTs has been experimentally achieved (CitationCumings and Zettl 2000). The bottleneck of using of nanomanipulator in medical nanorobots is the size of the nanomanipulator and the way how it gets a power. We believe that “artificial muscles” based on electrochemical actuation of conjugated polymers may be perspective (CitationBaughman 1996). The calculations based on density functional theory shows the elongation of the SWNT if the electric field is directed along its axis (CitationGuo and Guo 2003). Such elongation has the linear dependence on the magnitude of the electric field and can be up to 13% for electric field 0.4 V/Å. This electrostrictive deformation makes carbon nanotubes high-performance actuator material. Therefore “artificial muscles” can be in one’s turn based also on carbon nanotubes: bond length changes at electrochemical double-layer charging of sheets made of single-wall carbon nanotubes have been demonstrated (CitationBaughman et al 1999). The actuation of jet nanoengine with the help of the nanomanipulator is shown on .
The second possibility of the nanotube-based NEMS actuation is the usage of electric field. In this case it is necessary to charge the movable core of wall or to produce a dipole from it.
The calculations based on density functional theory shows the polarization of the SWNT if the electric field is directed along its axis (CitationGuo and Guo 2003). Recently it was experimentally shown that a dipole can be induced in MWNTs with the help of electric field (CitationFan et al 2005). Moreover the rotation of the MWNT in water about the axis which is perpendicular to the MWNT axis was demonstrated in this experiment. For this purpose four electrodes placed crosswise were used. We believe that in the case of telescopically pulled out inner core of walls of a carbon nanotube, a charge can be induced on the end of this core and the relative motion of the core and the shell can be achieved with the use of nonuniform electric field produced by a control electrode. Such a way of jet nanoengine actuation is shown on .
The calculated electrostatic potentials of carbon nanotubes are sufficiently different at open and closed ends (CitationPeralta-Inga et al 2003). Therefore a carbon nanotube wall with one open and another closed ends is a dipole and can be actuated with the help of nonuniform electric field.
It was proposed that movable wall can be charged through doping chemically (CitationZheng and Jiang 2002; CitationZheng et al 2002). For discussed purpose of actuation of relative motion of the inner core of walls of carbon nanotube the charge transfer in result of adsorption on the ends and inside the movable core of inner walls is suitable.
The charge transfer calculations show that the carbon nanotube becomes a dipole in the case of dissociative adsorption of Br2 (CitationPark et al 2005) and H2O (CitationHwang and Lee 2005) molecules on one open edge of the nanotube. Moreover the calculations of electrostatic potentials show distinctive polarized pattern, the direction of which is dependent on the atoms adsorbed on the open edge of the carbon nanotube (CitationMurray et al 2004). Therefore we propose to increase the dipole moment of the movable inner core by an adsorption of electron donors and acceptors on the opposite open edges of wall constituent the movable inner core. In this case the movable inner core of the nanotube can be moved by nonuniform electric field. The jet nanoengine actuated by movable inner core with electron donors adsorbed on the open edges of the walls constituent the core is shown on .
The calculations show that the valence electron of the encapsulated potassium atom of K@C60 is completely transferred to the C60 shell (CitationLi and Tomanek 1994). Therefore it was proposed that the transfer of the valence electron of the encapsulated potassium atom to the outer SWNT takes place in the system K@C60@SWNT (CitationKwon et al 1999). Thus the possibility that metal atoms encapsulated inside MWNTs can become ions with the transfer of valence electrons to the structure which holds the system has been discussed (CitationKang and Hwang 2004b). Evidently that the movable core of inner walls with ions encapsulated inside can be actuated with the help of electric field. The actuation of the inner wall with the encapsulated potassium atom inside relative to outer wall in the 7K+@(5,5)@(10,10) nanotube has been simulated with the help of molecular dynamics technique (CitationKang and Hwang 2004b). The jet nanoengine actuated by the movable inner core with encapsulated ions is shown on .
The set of methods to fill the interior of carbon MWNTs with magnetic materials have been elaborated (CitationTsang et al 1994; CitationZhang et al 1996; CitationDemoncy et al N. 1998). In the case where a movable inner core of walls is filled with magnetic material its motion can be controlled by a magnetic field. Note that the local spin density functional calculations show the increase of the magnetic moments of iron atoms in the iron-filled carbon nanotubes in comparison with a bulk iron (CitationKang et al 2005). The jet nanoengine with the movable inner core filled with a magnetic material is shown on .
The other way of the actuation of the relative sliding of nanotube walls with the help of the magnetic field which is nonuniform along the axis of the nanotube was proposed by CitationLegoas et al (2004). If the metallic wall or the the wall filled with metal moves in a nonuniform magnetic field B variation of magnetic flux Φ induced the current i
Progress in nanotechnology of nanotube-based NEMS production
Let us discuss the present-day progress in nanotechnology techniques that can be used for assembling of NEMS based on relative motion of carbon nanotubes walls.
To achive the possibility for relative motion of carbon nanotubes walls the caps which are closing the ends of walls should be removed. Several methods of opening of carbon nanotubes by oxidation have been elaborated (CitationTsang et al 1993; CitationAjayan et al 1993; CitationTsang et al 1994; CitationLiu et al 1998; CitationGeng et al 2004; CitationRaymundo-pinero et al 2005). These methods include oxidation by an air at temperature 750 °C (CitationAjayan et al 1993), by the carbon oxide at temperature 750 °C (CitationTsang et al 1993), by the concentrated nitric acids (CitationTsang et al 1994), and by the mixture of sulfuric and nitric acids simultaneously with the sonification (CitationLiu et al 1998; CitationGeng et al 2004). MWNTs were opened also by heat treatment in NaOH at temperature between 600 °C and 750 °C (CitationRaymundo-pinero et al 2005). It is shown, that opened ends of walls can be closed by vacuum annealing at temperature 750 °C (CitationLiu et al 1998; CitationGeng et al 2004).
The separated walls (ie, single-wall nanotubes) should be cut into segments with desirable lengths before the assembling of NEMS. The cutting of nanotubes into segments with the length range from 100 to 300 nm has been obtained by oxidation simultaneously with sonification (CitationLiu et al 1998). The method of precise and controlled cutting of MWNTs and SWNTs has been elaborated (CitationEl-Hami and Mitsushige 2003). Walls of nanotubes covered by organo-halogen were cut by conventional transmission electron microscopy. With this reproducible method the desirable length of walls could be obtained with an error ± 3 nm.
The attachment of the nanotubes to the nanomanipulators (which is necessary at the last stage of the NEMS assembling) were achieved in the set of the experiments (CitationCumings and Zettl 2000; CitationYu et al 1999). The voltage was applied to the nanomanipulator arranged near raw nanotube material. The nanotube is polarized by the electric field and jumps to a nanomanipulator due to an electrostatic attraction. Then the nanotube holds by van der Waals forces. A stronger bond can be made using the electron beam to perform localized electron impact-induced dissociation and deposition of gases present in the chamber (‘nanowelding’ technique) (CitationYu et al 1999). The three-dimensional manipulation by carbon nanotube with the use of two nanomanipulators has been also demonstrated (CitationYu et al 1999).
Since the interwall interaction energy potential relief is determined by chirality indexes of the walls (CitationKolmogorov and Crespi 2000; CitationDamnjanović et al 2002; CitationVuković et al 2003; CitationBelikov et al 2004; CitationBichoutskaia et al 2005), some special chirality indexes of the walls are necessary for some of nanotube-based NEMS listed above (for example, for the electromechanical nanothermometer). The direct method to determine chirality indexes of a carbon nanotube from a nanobeam electron diffraction pattern was recently demonstrated (CitationLiu and Qin 2005). The accuracy of this method allows to determine the chirality indexes unambiguously for single-wall nanotubes with diameters up to 4 nm.
The electrical-breakdown technique which allows to remove several walls of MWNT have been elaborated recently (CitationCumings et al 2000; CitationCollings et al 2001a, Citation2001b; CitationBourlon et al 2004a). The direct current passing through MWNT causes the heating of the MWNT in vacuum or heating and oxidation in air. As a result the central part of the MWNT between the electrodes (where is the peak of temperature) is destroyed. The length of the removed part is about 100 nm (CitationCollings et al 2001a). If the distance between the electrodes is less than 100 nm the walls are removed along the whole space between electrodes (CitationCollings et al 2001b). It was shown that the walls of the MWNT are removed stepwise with one removed walls at each step (CitationCollings et al 2001a, Citation2001b; CitationBourlon et al 2004a). Therefore the desirable number of walls can be removed. Such a technique was used to destroy the outer walls near two ends of MWNT and thus fabricate MWNT-based nanomotor with movable shell of outer walls (CitationBourlon et al 2004b).
Let us also propose the possible way to produce a hole in the outer wall of a MWNT (such a hole is necessary for nanotube based jet nanoengine operation). The method of nanoscale modification of carbon nanotubes by focused electron beam irradiation has been recently developed (CitationLi 2004). With the help of this method a set of knobs with diameter about 20 nanometers and controllable separation between knobs has bee fabricated on the nanotube surface. Evidently, that the matter of knobs is amorphous or graphite-like with numerous defects. In this case this matter can be easily removed by the mentioned above techniques which are used for nanotube caps removal (CitationTsang et al 1993, Citation1994; CitationAjayan et al 1993; CitationLiu et al 1998; CitationGeng et al 2004; CitationRaymundo-Pinero et al 2005). As the result the hole in the outer wall can be produced.
As it is discussed above metal atoms encapsulated inside the movable core of inner walls can become ions as a result of the transfer of valence electron to the nanotube and then to the structure which holds the fixed walls. In this case the movable inner core can be moved by electric field. The following technique for production of such nanotubes with encapsulated ions has been proposed (CitationKang and Hwang 2004b). At the first stage the nanotube should be filled by metallofullerenes by exposing in their vapor at high temperature. This method is demonstrated on the example of filling of the SWNTs by Sc2@C84 (CitationSuenaga et al 2003). At the second stage the SWNTs filled with metallofullerenes (pea-pod nanotube) should be annealed into DWNTs encapsulated metal atoms. The annealing of pea-pod nanotubes into DWNTs by electron irradiation (CitationSloan et al 2000) and by heating (CitationBandow et al 2000) has been demonstrated.
The production of carbon MWNTs filled with magnetic material is perspective for control of motion of nanotube-based electromechanical nanodevices with the use of magnetic field. The following methods of such production can be listed. The suspending of nanotubes in nitric acid solution containing hydrated nickel nitrate or cobalt nitrate or iron nitrate leads to filling of nanotubes by oxides of Ni, Co of Fe, respectively (CitationTsang et al 1994). Nanotubes filled with the oxides of Ni, Co or Fe were treated under hydrogen at 400 °C. As a result the oxide has been reduced to the native metal. Nanotubes filled with Fe were produced in arc discharge in the presence of He and Fe(CO)5 gases (CitationZhang et al 1996). Nanotubes filled with Ni were produced in arc discharge by doping a graphite anode with Ni and S (CitationDemoncy et al 1998).
We believe that all the techniques listed above allow to produce the proposed nanotube-based NEMS in nearest future.
Summary
In the present paper we consider possible application of carbon nanotubes-based NEMS in medical nanorobots. Experimental studies of biocompatibility of fullerenes and carbon nanotubes are analyzed. Carbon nanotubes have been shown to have the potential to stimulate inflammatory reactions by human and animal cells and to induce the formation of granuloma in the lungs, when inhaled. Nevertheless their toxicity against human cells, that is the capacity of inducing apoptosis and/or cell death, has been demonstrated to be lower than that of graphite particles. Thus we believe that nanotube-based nanorobots can be used in nanomedicine on condition that nanotube-contained components of nanorobots should be removed from the organism after the using.
The set of carbon nanotube-based NEMS for medical nanorobots is proposed. This set includes electromechanical nanothermometer, jet nanoengine, nanosyringe that can be used simultaneously as nanoprobe for study of single biological molecules and drug nanodeliver for apiece delivery of drug molecules. It is shown that such a NEMS can be really of nanometer size. The possible methods of actuation of these nanotube-based NEMS are discussed. The different schemes of the proposed NEMS corresponding to the different methods of their actuation (with the nanomanipulator, by use of electric or magnetic field) are considered. The discussion of the progress in nanotechnology techniques that are necessary for the production of the proposed NEMS shows that they can be realized in nearest future.
Acknowledgements
This work has been partially supported by the Russian Foundation of Basic Research (grants 05-02-17864 and 06-02-81036-Bel). Authors are grateful to the participants of the IV International symposium “Fullerenes and Fullerene-like Structures in Condensed Matter” for valuable discussions. The authors would like to thank the Natural Sciences and Engineering Research Council of Canada (NSERC) and Fonds de Recherche en Santé du Québec (FRSQ).
References
- AjayanPMEbbesenTWIchihashiT1993Opening of carbon nanotubes with oxygen and implication for fillingNature36252224
- BandowSTakizawaMHiraharaK2000Raman scattering study of double-wall carbon nanotubes from the chains of fullerenesin single-wall nanotubesChem Phys Lett3374854
- BarberAHCohenSRWagnerHD2005External and internal wetting of carbon nanotubes with organic liquidsPhys Rev B71 115443–1–5
- BaughmanRH1996Conducting polymer artificial musclesSynth Met7833953
- BaughmanRHCuiCZakhidovAA1999Carbon nanotube actuatorsScience28413404410334985
- BelikovAVLozovikYENikolaevAG2004Double-wallnanotubes: classification and barriers to walls relative rotation, sliding and screwlike motionChem Phys Lett385728
- BichoutskaiaEPopovAMEl-BarbaryA2005Ab initio study of relative motion of walls in carbon nanotubesPhys Rev B71 113403–1–4
- BichoutskaiaEPopovAMHeggieMI2006aMulti-walled nanotubes: commensurate-incommensurate phase transition and NEMS applicationsFullerenes, Nanotubes and Carbon Nanostructures14 (in print)
- BichoutskaiaELozovikYuEPopovAM2006bElectromechanical nanothermometerPhys Lett A (submitted)
- BichoutskaiaEHeggieMIPopovAM2006aInterwall interaction and elastic properties of double-wall carbon nanotubesPhys Rev B73 045435–1–9 (2006)
- BourlonBGlattiDCPlacaisB2004aGeometrical dependence of high-bias current in multiwalled carbon nanotubesPhys Rev Lett92 026804–1–4.
- BourlonBGlattiDCForroL2004bCarbon nanotube based bearing for rotational motionNano Lett470912
- CollingsPGHersamMArnoldM2001aCurrent saturation and electrical breakdown in multiwalled carbon nanotubesPhys Rev Lett86312831
- CollingsPGArnoldMAvourisPh2001bEngenering carbon nanotubes and nanotube circuits using electrical breakdownScience29270610
- CumingsJZettlA2000Low-Friction nanoscale linear bearings realized from multiwall carbon NanotubesScience289602410915618
- CumingsJCollinsPGZettlA2000Peeling and sharpering of multiwall nanotubesNature40658610949291
- DaiHHafnerJHRinzlerAG1996Nanotubes as nanoprobes in scanning probe microscopyNature38414750
- DamnjanovićMVukovićTMiloševićI2002Super-slippery carbon nanotubes: symmetry breaking breaks frictionEur Phys J B251314
- DemoncyNStephanOBrunN1998Filling carbon nanotubes with metals by the arc-discharge method: the key role of sulfurEur Phys J B414757
- El-HamiKMitsushigeK2003Organo-halogen uses for controlled cutting of carbon nanotubesInt J of Nanoscience21258
- FanDLZhuFQCammarataRC2005Controllable high-speed rotations of nanowiresPhys Rev Lett94247208
- FioritoSSerafinoAAndreolaF2006Effects of fullerenes and single-wall carbon nanotubes on murine and human macrophagesCarbon4411016
- ForroL2000Beyond gedanken experimentScience2895479
- GaoYBandoY2002Carbon nanothermometer containing galliumNature41559960011832929
- GaoYBandoYLiuZ2003Temperature measurements using a gallium-filled nanotube nanothermometerAppl Phys Lett83291316
- GaoBChenYFFuhrerMS2005Four-point resistance of individual single-wall carbon nanotubesPhys Rev Lett95 196802–1–4
- GengHZZhangXBMaoSH2004Opening and closing of single-wall carbon nanotubesChem Phys Lett39910913
- GraceIMBaileySWLambertCJ2004Electron transport in carbon nanotube shuttles and telescopesPhys Rev B70 153405–1–4
- GuoWGuoY2003Giant axial electrostrictive deformation in carbon nanotubesPhys Rev Lett91 115501–1–4.
- HaflierJHCheungCLieberCM1999Growth of nanotubes for probe microscopy tipsNature3987612
- HanssonAStafströmS2003Intershell conductance in multiwall carbon nanotubesPhys Rev B67 075406–1–6
- HuczkoALangeHCalkoE2001Physiological testing of carbon nanotubes: are they asbestos-like?Fullerene Science and Technology92514
- HuczkoALangeH2001Carbon nanotubes: Experimental Evidence for a Null Risk of Skin Irritation and AllergyFullerene Science and Technology924750
- HwangHJKwonOKKangJW2004Copper nanocluster diffusion in carbon nanotubeSol St Commun12968790
- HwangYGLeeYH2005Adsorption of H20 molecules on open ends of single-walled carbonnanotubesJ of Korean Physical Society42S267S271
- IijimaS1991Helical microtubules of graphitic carbonNature345568
- IsacssonANordT2004Low-frequency cuurent noise of the single-electron shuttleEurophys Lett6670814
- JarvisSPUchihashiTIshidaT2000Local solvation shell measurements in water using a carbon nanotube probeJ Phys Chem B, 1042660914
- KangJWHwangHJ2004aNanoscale carbon nanotube motor schematics and simulations for micro-electro-mechanical machinesNanotechnology1516338
- KangJWHwangHJ2004bGigahertz actuator of multiwall carbon nanotubes encapsulating metallic ions: molecular dynamics simulationJ Appl Phys9639005
- KangY-JChoiYMoonC-Y2005Electron and magnetic properties of single-wall carbon nanotubes filled with iron atomsPhys Rev B71 115441–1–7
- KimDHChangKJ2002Electron transport in telescoping carbon nanotubesPhys Rev B66 155402–1–5
- KolmogorovANCrespiVH2000Smoothest Bearings: Interlayer Sliding in Multiwalled Carbon NanotubesPhys Rev Lett8547273011082637
- KottasGSClarkeLIHorinekD2005Artifitial molecular rotorsChem Rev10512817615826014
- KwonYKTomanekDIijimaS1999‘Bucky shuttle’ memory device: synthetic approach and molecular dynamics simulationsPhys Rev Lett8214703
- LamCJamesJTMcCluskeyR2004Pulmonary toxicity of single-wall nanotubes in mice 7 and 90 days after intratracheal instillationToxicological Sciences771263414514958
- LauEYLightstoneFCColvinME2005Dynamics of DNA encapsulated in a hydrophobic nanotubeChem Phys Lett412827
- LegoasSBColuciVRBragaSF2004Gigaherts nanomechanical oscillators based on carbon nanotubesNanotechnology1518490
- LiJ-M2004Fabrication of carbon nanotube drive shaft componentNanotechnology155515
- LiYSTomanekD1994How free are encapsulated atoms in C60Chem Phys Lett2214538
- LiuJRinzlerAGDaiH1998Fullerene PipesScience280125369596576
- LiuZQinLC2005A direct method to determine the chiral indices of carbon nanotubesChem Phys Lett408759
- LozovikYuEMinoginAVPopovAM2003aNanomachines based on carbon nanotubesPhys Lett A31311221
- LozovikYuEMinoginAVPopovAM2003bPossible nanomachines: nanotube walls as movable elementsJETP Letters776315
- LozovikYuEPopovAM2004Nanomachines based on Carbon nanotubes walls motion: operation modes and control forcesFullerenes, Nanotubes and Carbon Nanostructures1248592
- MattsonMPHaddonRCRaoAM2000Molecular functionalization of carbon nanotubes and use as substrates for neuronal growthJournal of Molecular Neuroscience141758210984193
- Monteiro-RiviereNANemanichRJInmanAO2005Multi-walled carbon nanotube interactions with human epidermal keratinocytesToxicological Letters15537784
- MurrayJSLanePConchaMC2004Electrostatic potentials and local ionization energies on the surface of functionalized carbon and boron-nitrogen nanotube modelsNano and Giga Challenges in MicroelectronicsCracow, Poland913–17 Book of Abstracts175
- NagyGLevyMScarmozzinoR1998Carbon nanotube tipped atomic force microscopy for measurements of <100 nm etch morphology on semiconductorsAppl Phys Lett7352931
- NimmagaddaAMcFetridgeP2004Single walled carbon nanotubes: an assessment of biocompatibility for tissue engineering applicationsCardiovascular Pathology13S81S82
- ParkNMiyamotoYLeeK2005Band gap sensetivity of bromine adsorption at carbon nanotubesChem Phys Lett4031359
- Peralta-IngaZLanePMurrayJS2003Characterization of surface electrostatic potentials of some (5,5) and (n,1) carbon and (n,1) boron-nitrogen nanotubesNano Lett32128
- PolizuSPoulinSPoulinPNanoscale Surface of Carbon Nanotubes Fibers for Medical Applications: Structure and Chemistry Revealed by TOF-SIMS AnalysisApplied Surface Science Accepted, December 2005
- Raymundo-pineroECacciagueraTSimonP2005A single step process for the simultaneous purification and opening of multiwalled carbon nanotubesChem Phys Lett41218490
- SaitoRFujitaMDresselhausG1992Electronic structure of chiral graphene tubulesAppl Phys Lett60220406
- Salvador-MoralesCFlahautESimE2006Complement activation and protein adsorption by carbon nanotubesMolecular Immunology4319320116199256
- ShvedovaAACastranovaVKisinER2003Exposure to nanotube material: assessment of nanotube cytotoxicity using human keratinocyte cellsJ Toxicol Environ Health66190118
- SloanJDunin-BorkowskiREHutchisonJL2000The size distribution, imaging and obstructing properties of C60 and higher fullerenes formed within arc-grown single-walled carbon nanotubesChem Phys Lett3161918
- SrivastavaDW1997A phenomenological model of the rotation dynamics of carbon nanotube gears with laser electric fieldsNanotechnology818692
- SuenagaKOkazakiTWangC-R2003Direct imaging of Sc2@C84 molecules encapsulated inside single-wall carbon nanotubes by high-resolution electron microscopy with atomic sensitivityPhys Rev Lett90 055506–1–4
- TansSJDevoretMHDaiH1997Individual single-wall carbon nanotubes as quantum wiresNature3864747
- TsangSCHarrisPJFCreenMLH1993Thinning and opening of carbon nanotubes by oxidation using carbon oxideNature3625202
- TsangSCChenYKHarrisPJF1994A simple chemical method of opening and filling of carbon nanotubesNature37215961
- TuzunRENoidDWSumpterBG1996Dynamics of fluid flow inside carbon nanotubesNanotechnology72416
- TuzunRENoidDWSumpterBG1997Dynamics of He/C60 flow inside carbon nanotubesNanotechnology81128
- VukovićTDamnjanovićMMiloševićI2003Interaction between layers of multi-wall carbon nanotubesPhysica E1625968
- WarheitDBLaurenceBRReedKL2004Comparative pulmonary toxicity assessment of single-wall carbon nanotubes in ratsToxicological Sciences771172514514968
- WeiCSrivastavaD2003Theory of transport of long polymer molecules through carbon nanotube channelsPhys Rev Lett91 235901–1–4
- WongSSJoselevichEWolleyAT1998aCovalently functionalized nanotubes as nanometre-sized probes in chemistry and biologyNature3945269665127
- WongSSHarperJDLansburyPT1998bCarbon nanotube tips: high-resolution probes for imaging biological systemsJ Am Chem Soc1206034
- YuMFDyerMJSkidmoreGD1999Three-dimensional manipulation of carbon nanotubes under a scanning electron microscopeNanotechnology1024452
- YuMFLourieODyerMJ2000aStrength and breaking mechanism of multiwalled carbon nanotubes under tensile loadScience2876374010649994
- YuMFYakobsonBIRuoffRS2000bControlled sliding and pullout of nested shells in individual multiwalled carbon nanotubesJ Phys Chem B104876467
- ZhangGLAmbeFdu Marche Van VoorthuysenEH1996Growth mechanism for iron-filled carbon nanotubulesJ Appl Phys8057982
- ZhengQJiangQ2002Multiwalled carbon nanotubes as gigahertz oscillatorsPhys Rev Lett88 045503–1–3
- ZhengQLiuJZJiangQ2002Excess van der Waals interaction energy of a multiwalled carbon nanotube with an extruded core and the induced core oscillationsPhys Rev B65 245409–1–6