Abstract
Purpose
Mesoporous silica (MS) have been considered as a biocompatible compound and found to have various pharmaceutical applications. Recently, novel approaches in applications of MS as antidote agents were introduced. In this study, the capacity of ethylenediaminetetraacetic acid modified mesoporous silica (MS-EDTA) was evaluated in in vitro and in vivo adsorption of copper (Cu).
Methods
The MS-EDTA was characterized by fourier transform infrared (FT-IR) and X-ray diffraction, while surface area was determined by N2 adsorption–desorption technique. Morphological studies were observed by high resolution-transmission electron microscopy and field emission-scanning electron microscopy and the sizes were determined by dynamic light scattering. The capacity of these particles for copper adsorption was investigated in vitro in both 1.2 and 7.2 pH. In in vivo animal study, the Cu adsorption efficiency of MS-EDTA in Cu-overdosed mice was evaluated. In this case, an animal model of acute copper poisoning was prepared.
Results
The MS-EDTA with surface area of 352.35 was synthesized. Scanning electron microscope showed spherical particle formation with less than 500 nm in size. Transmission electron microscope images showed porous and honeycomb structure. FT-IR spectroscopy showed an appropriate formation of functional groups. Particle efficiency was investigated for Cu adsorption. MS-EDTA in both media showed a high adsorption capability for Cu (II) adsorption in pH=1.2 and pH=7.2. In addition, the study of Langmuir, Freundlich, and Redlich–Peterson adsorption models showed that copper adsorption by MS-EDTA followed the Freundlich model with multi-layer adsorption. In vivo evaluation showed that MS-EDTA could alleviate the symptoms of acute copper poisoning by lowering Cu plasma levels.
Conclusion
Structural evaluation showed successful formation of MS-EDTA. In vitro analysis demonstrated that supreme Cu adsorption occurs in both pH conditions (7.2 and 1.2), and was especially more favorable in simulated intestinal pH (7.2). The in vivo studies in animal models with acute Cu poisoning showed that MS-EDTA could be a potent antidote agent.
Introduction
Physicochemical features of nanoscale materials and structures, including surface-to-volume ratio, surface chemistry, optical, electrical, thermal, and magnetic properties, make nanotechnology beneficial for medical and pharmaceutical purposes.Citation1 Having a technology with the ability of controlled absorption, carry, release, sensing, and/or separation of certain substances (such as drugs or therapeutic agents) in vivo or ex vivo is worthwhile to gain diagnostic or therapeutic goals.Citation2,Citation3 To achieve this, requires biocompatible particles with identical sizes, adjustable pore sizes and volumes, large interacting surface area, and acceptable capacity of functionalization, desirable biodistribution, lifetime, and toxicity.Citation4,Citation5 Liposomes,Citation6 micelles,Citation7 dendrimers,Citation8 magnetic nanoparticles,Citation9 gold nanoparticles,Citation10 quantum dots,Citation11 and carbon nanotubesCitation12 are those which may present some, but not all, of these features. However, porous materials are capable of displaying these necessary features.Citation13
Mesoporous solid particles’ surface area is absolutely enormous; in some cases, reaching 1000 m2g−1.Citation14 Mesoporous silica nanoparticles (MSNs) are size-tunable nanoparticles that possess well-ordered pores with sizes of 2–50 nm and a large surface area that can be functionalized or interact with drugs, ions or other molecules.Citation15 Silica is used as a food additive and in cosmetics, and also has been categorized as “Generally Recognized as Safe” by FDA.Citation16 Moreover, several studies have been shown that MSNs have high biocompatibility and that some specific doses (up to 100 µg.mL−1) do not have any toxicity.Citation15 For instance, Qianjun et al, evaluated the effects of MSN size on in vivo biodistribution and urinary excretion and concluded that MSNs with different particle sizes (80–360 nm) accumulate mainly in the liver and spleen with a minority of them in the lungs and a few in the kidney and heart and also that MSNs cause no tissue toxicity after 1 month.Citation17
MSNs have tunable pores with high surface area and good functionality, making them a desirable encasement for a wide range of molecules.Citation18 MSNs can be synthesized by different chemical methods using either hard or soft and organic or non-organic templates, but mostly is completed by soft templates, such as block copolymers and surfactants that are amphiphilic molecules.Citation15 Structure, composition, pore size and volume, and functional groups can be designed and tailored during synthesis by controlling moieties stoichiometry, surfactant types, and reaction conditions.Citation19 Therefore, different types of MSNs have been synthesized and studied, including MCM-41 (Mobil Composition of Matter/No. 41), MCM-48 (Mobil Composition of Matter/No. 48), MCM-50 (Mobil Composition of Matter/No. 50) MCM-48, HMS (hexagonal mesoporous silica), SBA-15 (Santa Barbara Amorphous), and so on.Citation20
MCM-41 is an ordered and uniform two-dimensional mesoporous silica (MS) that is widely studied. Cetyl-trimethylammonium bromide (CTAB) is the surfactant commonly used to synthesize MCM-41.Citation21 Generally, nano-sized particles (e.g. MSNs) internalize into cells by endocytosis, and the internalization pathway is highly dependent on structural and chemical properties of nanomaterials such as shape, size, and surface functionalization. There is some evidence that suggests clathrin-mediated endocytosis and caveolae-mediated endocytosis as the main internalization route of MSN.Citation15 Altogether, MSNs are one of the best potential candidates for applications such as catalysis,Citation22 sensors,Citation23 drug delivery systems,Citation24 imaging agents,Citation25 separation,Citation26 micro-extraction,Citation27 and as potent adsorbent.Citation28
A therapeutic substance that counteracts the toxicity mechanisms of a specified xenobiotic is an antidote. Antidotes are playing an important role in the treatment of poisoning.Citation29 Recently, MSNs have also been used as an antidote agent that can adsorb toxic agents in or out of cells. In the study that was conducted by Farjadian et al, MCM-41 was used as potential adsorbent of acetaminophen and phenobarbital while showed a better performance compared to activated charcoal that was the traditional method.Citation30 Subsequently, in 2017, ethylenediaminetetraacetic acid (EDTA)-modified MSN was used to remove iron toxicity in an animal model.Citation31 MS materials have shown high potential in heavy metals adsorption. In a study MS, iron oxide nanocomposites were used to remove heavy metals, with successful results in adsorbing Ni, Cd, Cr, Zn, and Pb ions.Citation32 Also, EDTA-modified MSNs were used to adsorb Pb in different pH, and a favorable adsorption performance was gained as a result.Citation33
Wilson disease is an autosomal recessive disorder caused by a mutation in the ATP7B geneCitation34 with a relative prevalence of one in every 30,000 births.Citation35 Copper is accumulated in various organs and tissues, such as the liver and brain,Citation36 and can induce a wide range of symptoms, such as brain disorders.Citation35 The usual drug for this disease is oral D-penicillamine, a chelating agent for copper.
Although this drug can adsorb extra copper, many side effects have been observed with its use, including immunologic reactions, skin lesions (such as epidermolysis bullosa), systemic lupus erythematosus, nephrotic syndrome, Goodpasture syndrome, Ehlers-danlos syndrome, myasthenia gravis, polymyositis, and thrombocytopenia. Also, in some patients, clinical symptoms such as dystonia and Parkinson have been seen with penicillamine consumption.Citation37
Recent studies on antidotes have shown that functionalized MSNs can be applied as an appropriate antidote for overdose of iron. Farjadian et al, sought to adsorb iron by using MSN-EDTA and has provided great results.Citation31 It is predicted that MSNs could adsorb other metals, such as copper, and therefore, one could contemplate their use as a suitable antidote for copper in copper toxicity. This study aims to synthesize and evaluate the kinetics and isotherms of EDTA-functionalized mesoporous silica particles (MS-EDTA) as an adsorbent of Cu(II) in gastrointestinal pH simulated in vitro conditions and Cu-overdosed mice.
Methods and materials
Materials
CTAB, tetraethoxysilane (TEOS), 3-aminopropyl triethoxysilane (APTES), thionyl chloride (SOCl2), copper sulfate pentahydrate (CuSO4.5H2O), dimethyl sulfoxide (DMSO), and ethylenediaminetetraacetic acid were purchased from Merck chemical company (Sigma-Aldrich). Hydrochloric acid (HCl), ethanol, and acetone were bought from Kimia Mavad (Tehran, Iran).
Preparation of amino-functionalized MCM-41 (MS-NH2)
MS-NH2 was prepared according to a reported procedure.Citation38 Briefly, 6.6 mmol of CTAB was dissolved in 100 mL of 1:1 deionized (DI) water/ethanol solution, then 13 mL of ammonia was added. After obtaining a clear solution, 3.085 mL of TEOS and 0.37 mL of APTES was added dropwise to the solution over 10 mins while stirring. The solution was stirred for 2 hrs at 80°C to produce a white mixture. The product was washed three times with DI water and ethanol, then was dried overnight (at 60°C) in an oven. Acidic ethanol (6 mL HCl/200 mL ethanol) was used to extract the surfactant templates (48 hrs, 90°C).
Preparation of EDTA-functionalized MCM-41 (MS-EDTA)
The preparation of MS-EDTA was performed according to the reported procedure.Citation31 Briefly, 1.7 g EDTA was dissolved in 20 mL of DMSO, then 0.42 mL of thionyl chloride (SOCl2) was added dropwise. After 10 mins, 1 g of MS-NH2 was added to the solution and stirred for 20 hrs at room temperature. Samples were centrifuged (3×10 mins, 5000 rpm) and washed three times with ethanol and acetone, then dried by freeze-dryer.
Characterization
Morphology of particles was characterized by high resolution-transmission electron microscopy (HR-TEM) by JEOL JEM 2010 instrument. Field emission-scanning electron microscopy (FE-SEM) was performed with Zeiss Merlin PV instrument to determine the size of particles and energy-dispersive X-ray spectroscopy (EDX) to determine the type of elements in the sample. Dynamic light scattering (DLS), by Microtrac, was used to determine hydrodynamic diameter. Fourier transform infrared spectroscopy (FT-IR) with KBr by BRUKER (Germany) was performed to obtain an infrared spectrum of absorption or emission of particles, X-ray diffraction (XRD) was performed by 40 kV MPD 3000 instrument to obtain detailed information about structure properties. Copper adsorption by MS-EDTA was investigated by Varian atomic absorption spectroscopy (AAS). Physical and chemical composition of particles and also the Brunauer Emmett Teller (BET) technique have been performed by Micromertics TriStar II plus (America) to measure particles surface area.
Adsorption tests
To determine the copper adsorption by MS-EDTA, samples containing CuSO4/MS-EDTA with concentration ratios of CuSO4 (mmol)/MS-EDTA (NH2+EDTA) mmol: 0.2, 0.5, 1, 2.5, 5, 7.5, 10, 50, 150, 500, 1000, and 25,000 were prepared in pH=1.2 and pH=7.2 mediums. Samples were mixed at 40°C for 2 hrs in a shaker. After adsorption, the samples were investigated by AAS.
To determine the copper adsorption isotherm adsorption capacity, EquationEquation 1(1) was utilized:
In this equation, m (g) is the sorbent’s mass (MS-EDTA). C0 (mmol L−1) is the initial concentration of copper, Ce (mmol L−1) is the concentration of copper at equilibrium, V (L) is solution volume, and q is the amount of copper adsorption per MS.
Adsorption isotherm models
Three adsorption models Langmuir, Freundlich, and Redlich–Peterson were applied to fit with derived amounts of adsorbed copper mass (mmol per gram) of MS-EDTA.Citation39,Citation40 The Langmuir isotherm model is based on EquationEquation 2(2) , which is describing a continuous monolayer adsorption process surrounding homogeneous solid adsorbent.
In the equation mentioned above, q (mmol/g) is the adsorbed copper mass (mmol) of MS-EDTA (g−1) at equilibrium. Kl and qm are the empirical constant of adsorption (L mmol−1) and qm maximum uptake amount (mmol g−1), respectively.
The Freundlich isotherm model based on EquationEquation 3(3) . This model is applied to characterize heterogeneous systems in multiple adsorption layers.
In this equation, Kf is Freundlich adsorption constant [mmol g−1(L mmol−1)1/n]. 1/n represents adsorption intensity; when its value is between 0 and 1, there is equal adsorption chances and energies for all active sites.
Redlich–Peterson is an isotherm model; a combination of both Langmuir and Freundlich model equations. This isotherm is based on EquationEquation 4(4) .
In EquationEquation 4(4) , A and B are the capacity and the equation constant, respectively. Also, “g” lies between 0 and 1. If “g” was 1, this model would be the same as the Langmuir adsorption model, and if “g” value was between 0 and 1, it would be similar to the Freundlich model.
Kinetic models
To investigate the effect of time on copper adsorption by MS (mmol g−1), a sample with Cu/MS-EDTA; 150 (Cu mmol/MS-EDTA mmol) was prepared. Then, adsorption kinetic models (i.e. pseudo-first-order, pseudo-second-order, and intraparticle diffusion model) were studied to predict the rate of adsorption. The pseudo-first-order equation is described as follows:
In this equation, qt is the amount of Cu adsorbed (mmol g−1) at any time, q is the mass of adsorbed copper (mmol g−1) in equilibrium, t (min) and K1 (min−1) is time and constant of adsorption rate at equilibrium, respectively.
Pseudo-second-order equation based on EquationEquation 6(6) .
In the equation above, k2 (mmol g−1 min−1) is the adsorption rate constant at equilibrium, while t, q, and qt are defined in EquationEquation 5(5) .
Intraparticle diffusion model is stated in EquationEquation 7(7) .
In EquationEquation 7(7) , C is the border layer thickness; t and qt are defined before.
In vivo animal model
The effect of MS-EDTA nanoparticles was evaluated against acute copper toxicity in mice.Citation41 For this purpose, animals were allotted in the following groups (n=6/group): (A) Copper-treated animals which received 5000 mg/kg of copper sulfate by gavage;Citation41 (B) Copper + MS-EDTA nanoparticles (1 mg/kg, gavage); (C) Copper + MS-EDTA nanoparticles (10 mg/kg, gavage); and (E) Copper + EDTA (10 mg/kg, gavage). The investigated antidotes were administered 2 hrs after copper intoxication. Serum and liver tissue Cu levels, in addition to serum biomarkers of organ injury, were monitored 24 hrs after copper administration. All the experiments using laboratory animals were performed in conformity with the guidelines for care and use of experimental animals approved by an ethics committee in Shiraz University of Medical Sciences, Shiraz, Iran (#1396-01-74-14350). A MindrayBS-200® chemistry analyzer (Guangzhou, China) and standard kits (Pars Azmun®, Tehran, Iran) were used to measure plasma biomarkers of organ injury.Citation42 Plasma Cu levels were measured using atomic adsorption spectroscopy.
Results
The main aim of this study was the potential application of MS-EDTA in the treatment of copper poisoning in paradigms like Wilson disease (). For this purpose, MS-EDTA was synthesized. Firstly, MS-NH2 was prepared by the co-condensation method in the presence of a surfactant template (CTAB) in ammonia solution while TEOS and APTES were applied as silane coupling agents. To introduce EDTA into MS pore walls, the carboxyl functional group of EDTA was activated by thionyl chloride (thionyl chloride/EDTA; 1:1), and MS-NH2 was admixed with activated EDTA solution to obtain MS-EDTA.
Figure 1 Schematic illustration of MS-EDTA administration in the treatment of copper toxicity.
Abberviation: MS-EDTA, ethylenediaminetetraacetic acid modified mesoporous silica.
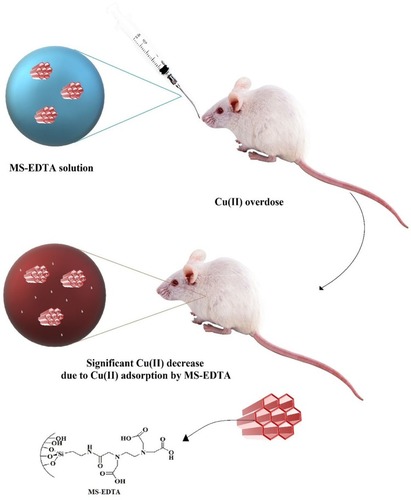
Morphology and characterization of MS-EDTA
The FT-IR spectrums show symmetric and asymmetric stretching bonds of Si–O groups that appear at 790 and 1065 cm−1, respectively. Furthermore, the N-C=O band at 1480–1520 cm−1 and 1630 cm−1 is respectively related to amide and carboxylic acid (C=O) functional groups (). BET calculations have shown MS-EDTA with a surface area of 352.35 m2g−1 and pore size of 2.4 nm, while a drastic change is observed when compared with the MS-NH2 surface area that was calculated to be 921.51 m2g−1. Herein, the surface area decrease could be attributed to the successful introduction of EDTA in MS-NH2 pores. The MS-EDTA crystallographic information which was received by low angle XRD has shown the expected crystallinity of mesoporous material by revealing a sharp peak at 0.8° for the (100) xyz directions and a broader peak at 2.5° resulting from additional Brag reflections ().
The MS-EDTA morphological features, size, and shape, are investigated using FE-SEM () and HR-TEM () images. The FE-SEM image of MS-EDTA shows that most of the particles have a spherical shape and are monodispersed. These observations reveal that particles have sizes less than 500 nm with rough surfaces. In addition, TEM images confirm the formation of spherical porous structures with well-ordered pores. Also, EDX analysis was carried out to determine the amount of organic layer formation (i.e. aminopropyl and EDTA) in the MS-EDTA walls ().
Figure 2 FT-IR spectroscopy of MS-NH2 and MS-EDTA.
Abbreviations: FT-IR, fourier transform infrared; MS-EDTA, ethylenediaminetetraacetic acid modified mesoporous silica.
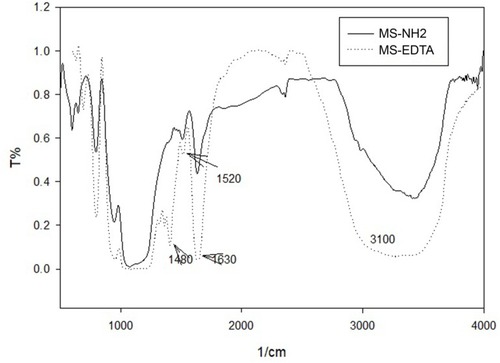
Figure 3 XRD pattern of MS-EDTA.
Abbreviations: XRD, X-ray diffraction; MS-EDTA, ethylenediaminetetraacetic acid modified mesoporous silica.
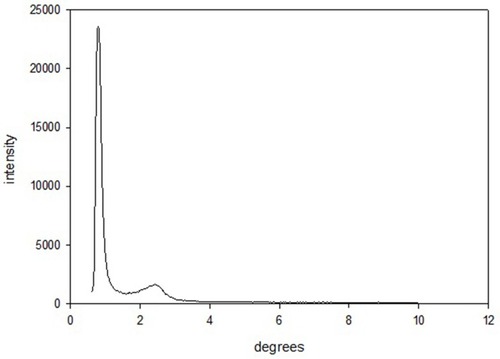
Figure 4 FE-SEM image of MS-EDTA.
Abbreviations: FE-SEM, field emission-scanning electron microscopy; MS-EDTA, ethylenediaminetetraacetic acid modified mesoporous silica.

Figure 5 HR-TEM images of MS-EDTA.
Abbreviations: HR-TEM, high resolution-transmission electron microscopy; MS-EDTA, ethylenediaminetetraacetic acid modified mesoporous silica.
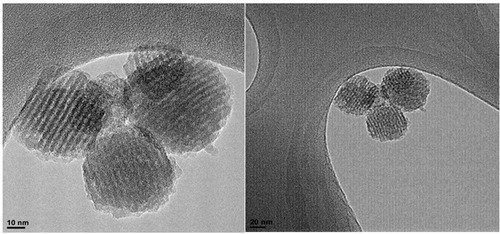
Figure 6 EDX analysis of MS-EDTA.
Abbreviations: EDX, energy-dispersive X-ray spectroscopy; MS-EDTA, ethylenediaminetetraacetic acid modified mesoporous silica.

DLS provides the average size of nano-spheres based on the intensity of particles in the fluid environment. The average particle size in the sample is about 471 nm ().
Cu adsorption
Based on the results, Cu adsorption percentage by the MS in pH 1.2 and 7.2 is found to be higher than 80% and 95%, respectively. Results are shown in ). In vitro adsorption evaluations were carried out in pH 1.2 (the same as pH of stomach environment) and pH 7.2 (the same as intestinal pH). The adsorption isotherm graphs are plotted based on the amount of q (mmol/g) against the concentration of copper in equilibrium (mmol L−1). Adsorption is described in a wide range of concentrations. ) indicates adsorption data that is fitted with Langmuir and Freundlich adsorption models, so the parameters extracted from the three models and their comparison are given in .
Figure 8 Adsorption percentage of copper by MS-EDTA in pH 7.2 (A) and in pH 1.2 (B).
Abbreviation: MS-EDTA, ethylenediaminetetraacetic acid modified mesoporous silica.
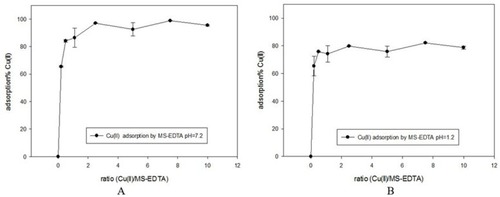
Figure 9 Adsorption isotherm of copper on MS-EDTA in aqueous media with pH 7.2 (A) and in pH 1.2 (B).
Abbreviation: MS-EDTA, ethylenediaminetetraacetic acid modified mesoporous silica.

Table 1 The parameters extracted from the three adsorption models and their comparison with Cu(II) adsorption on MS-EDTA
Using the Redlich–Peterson model (n<1), it is determined that copper adsorption by MS follows the Freundlich model with multi-layer adsorption. In this way, with the formation of the primary EDTA complexes with Cu (II) and the entry of the sulfide ions, it is created to absorb other copper ions, and copper ions can be embedded in multi-layers of the MS-EDTA.
Kinetic models
Three kinetic models of the pseudo-first-order, pseudo-second-order, and intraparticle diffusion were used to investigate copper adsorption by MS-EDTA that are shown in –. The results show that Cu (II) adsorption by the MS-EDTA in the first 2 hrs corresponds to the intraparticle diffusion model. At this time, Cu(II) enter the MS-EDTA pores without interacting with functional groups and then conforms to the pseudo-second-order model, the Cu(II) could bond to the functional groups in the porosity and the adsorption increases. The parameters obtained from the pseudo-second-order kinetic model are shown in .
Figure 10 Pseudo-first-order model in pH 1.2 and 7.2.
Abbreviation: MS-EDTA, ethylenediaminetetraacetic acid modified mesoporous silica.
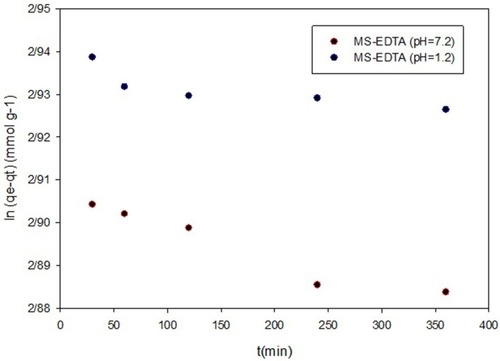
Figure 11 Pseudo-second-order model in pH 1.2 (A) and pH 7.2 (B).
Abbreviation: MS-EDTA, ethylenediaminetetraacetic acid modified mesoporous silica.
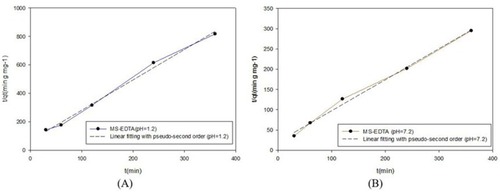
Figure 12 Intraparticle diffusion model; (A) pH 7.2 and (B) pH 1.2.
Abbreviation: MS-EDTA, ethylenediaminetetraacetic acid modified mesoporous silica.
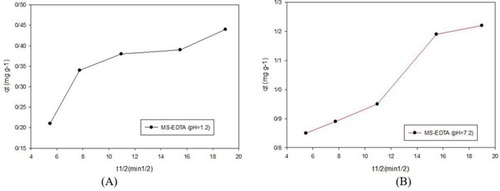
Table 2 Parameters derived from kinetic adsorption for MS-EDTA
In vivo animal model
Herein, an animal in vivo study attempts to evaluate the Cu adsorption efficiency of MS-EDTA in Cu-overdosed mice. In this case, an animal model of acute copper poisoning was prepared by IV injection of copper sulfate (5000 mg/kg) to the mice. As a result, the Cu level of serum and plasma markers of liver injury, including LDH, ALT, and AST were elevated in the Cu-treated group. As it was discovered, serum Cu and the biomarkers of injury in Cu-treated mice are significantly reduced by administration of either EDTA or synthesized MS-EDTA. The effects of MS-EDTA were not dose-dependent in the current study (). The MS-EDTA treated group was considerably more effective in comparison with EDTA. Although the serum Cu level in the antidote-treated mice is a bit higher than the control level, periodic antidote administration could bring normal serum Cu level back (). Altogether, MS-EDTA Cu adsorption capability is a promising feature that could handle Cu-overdose in cases such as Wilson disease treatment.
Figure 13 Plasma biomarkers of organ injury in copper-treated mice including (A) alanine aminotransferase (ALT), (B) aspartate aminotransferase (AST), and (C) lactate dehydrogenase (LDH) along with Cu level were assessed 24 hrs after Cu administration. Data are presented as mean ± SD (n=6). ***Indicates significantly different as compared with the Cu group (P<0.001) “a” indicates significantly different as compared with the control group (P<0.05).
Abbreviations: MS-EDTA, ethylenediaminetetraacetic acid modified mesoporous silica; ns, not significant.
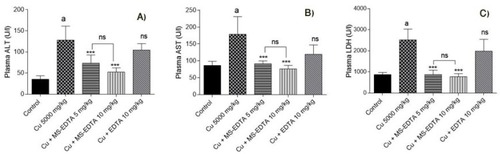
Figure 14 Serum Cu(II) level in overdosed mice. ***Indicates significantly different as compared with the Cu group (P<0.001). “a” indicates significantly different as compared with MS-EDTA-treated groups (P<0.01).
Abbreviations: MS-EDTA, ethylenediaminetetraacetic acid modified mesoporous silica; ns, not significant.
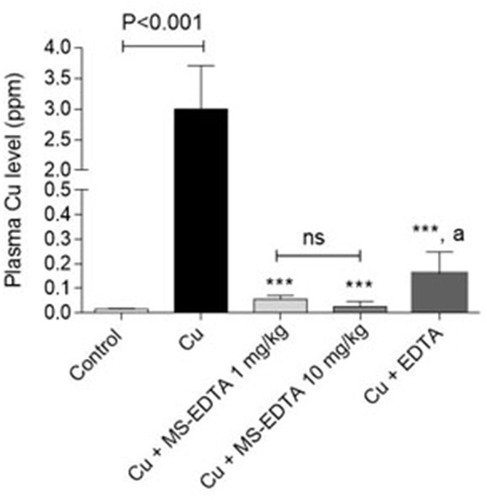
Discussion
MS-EDTA was synthesized by the co-condensation method and characterized. The nitrogen adsorption–desorption method illustrated type IV isotherm for MS-EDTA the characteristic of the mesoporous structure. Also, using this method, the surface-to-volume ratio was about 352.35 m2/g, the pore size was 2.4 nm,Citation17 and the pore volume capacity was 0.11 cm3/g. It should be noted that the surface area of the MS-NH2 was 921.51 m2/g, which resulted in a significant decrease in EDTA loading. SEM shows spherical particle formation with less than 500 nm in size, and the DLS confirms this information (471 nm). Based on the TEM, it became clear that the particles had a porous morphology and a honeycomb structure. In a synthesized MS-EDTA, a broad exponential peak in the reflection is observed in the region of 0.7 to about 1 in 2-theta, which is characterized by the MS structure and confirms the data obtained from the TEM. To investigate the presence of the desired functional groups at the internal surface of the porosity, FT-IR was performed, and it confirmed that the EDTA is bonded to the porosity surface and functional groups are available. On the other hand, based on the data obtained from thermogravimetric analysis, the amount of EDTA loaded in the MS cavities was 0.4 mmol/g, which fully matches the results of EDX. Particle efficiency was investigated for copper adsorption in pH=1.2 and pH=7.2. MS-EDTA in both media showed a high adsorption capability for Cu (II) adsorption, however, because of the protonation of the amine group at pH=1.2, in a medium with pH similar to the stomach area, it showed less adsorption and, conversely, in the environment with pH the same as the intestinal environment with pH=7.2 shows a higher adsorption. The high adsorption of copper with MS-EDTA can be explained by the multi-layer adsorption of copper salt. The EDTA structure at pH=7.2 has a negative charge and four non-protonated carboxyl groups that can be actively combined with copper. However, even with the protonation of the amine group in the acidic environment, MS-EDTA also shows good adsorption in pH=1.2. In addition, the study of Langmuir, Freundlich, and Redlich–Peterson adsorption models showed that copper adsorption by MS-EDTA follows the Freundlich model with multi-layer adsorption, in which copper is adsorbed with EDTA and formed a complex between them. Following the formation of this complex, copper ions adsorb sulfate ions and allow the re-adsorption of another layer of copper ions onto sulfate ions, which greatly increases the adsorption capacity. The study of three kinetic models, pseudo-first-order, pseudo-second-order, and intraparticle diffusion, showed that copper adsorption by MS-EDTA in the first 2 hrs complies with the intraparticle diffusion model. During this time, copper ions enter the MS pores without interacting with functional groups. After that corresponds to the pseudo-second-order model, at which time the copper ions with the functional groups in the porosity bonded and the adsorption increased. In vivo evaluation showed that MS-EDTA could be recognized as a potent antidote agent in acute copper poisoning. Although EDTA could reduce the Cu level of plasma but significant results obtained by treatment with MS-EDTA. On the other hand, as MS-EDTA particle might show an affinity for other metal ions rather than Cu,Citation31 disturbances in vital metal ions (e.g. Fe, Mg, and Zn) might occur in their chronic administration (e.g. in experimental models of Wilsons disease). Hence, these metal ions could be administered as supplementary agents to prevent electrolytes and ion disturbances.
Conclusion
We conclude that EDTA-functionalized MS is spherical particles which were synthesized in co-condensation method and with well-ordered honeycomb pores, have an eligible surface-to-volume ratio, pore size, and the pore volume capacity for effective adsorption of copper ions. Particle efficiency was investigated for copper adsorption in pH=1.2 (same as stomach environment pH), and pH=7.2 (same as intestinal environment pH) and a high adsorption capability was observed in both media for copper adsorption, especially in pH 7.2 medium which was much more satisfying attributed to the negatively charged functional groups. The in vivo evaluation showed the potential effect of MS-EDTA in the reduction of Cu plasma level in mice with Cu acute poisoning. Thus, MS-EDTA can be recognized as an antidote of copper poisoning cases. On the other hand, these particles might be useful for decreasing complications associated with Cu accumulation in the body (e.g. in Wilson’s disease). Therefore, evaluating the biological activity of MS particles in experimental models of Wilson’s disease might help to develop safe therapeutic options against this complication in humans.
Disclosure
The authors report no conflicts of interest in this work.
Acknowledgment
The authors would like to thank the Research Council of Shiraz University of Medical Science for supporting this work under grant No.1396-01-74-14350.
References
- Whitesides GM. Nanoscience, nanotechnology, and chemistry. Small. 2005;1:172–179. doi:10.1002/smll.20040013017193427
- Brigger I, Dubernet C, Couvreur P. Nanoparticles in cancer therapy and diagnosis. Adv Drug Deliv Rev. 2012;64:24–36. doi:10.1016/j.addr.2012.09.006
- Farjadian F, Moghoofei M, Mirkiani S, et al. Bacterial components as naturally inspired nano-carriers for drug/gene delivery and immunization: set the bugs to work? Biotechnol Adv. 2018;36(4):968–985. doi:10.1016/j.biotechadv.2018.02.01629499341
- Park K. Controlled drug delivery systems: past forward and future back. J Controlled Release. 2014;190:3–8. doi:10.1016/j.jconrel.2014.03.054
- Edgar JYC, Wang H. Introduction for design of nanoparticle based drug delivery systems. Curr Pharm Des. 2017;23(14):2108–2112. doi:10.2174/138161282266616102515400327784242
- Lamichhane N, Udayakumar T, D’Souza W, et al. Liposomes: clinical applications and potential for image-guided drug delivery. Molecules. 2018;23(2):288. doi:10.3390/molecules23020288
- Hosseini M, Farjadian F, Makhlouf ASH. Smart stimuli-responsive nano-sized hosts for drug delivery. Industrial Applications Intelligent Polym Coat. 2016;1–26. doi.org/10.1007/978-3-319-26893-4_1
- Noriega-Luna B, Godínez LA, Rodríguez FJ, et al. Applications of dendrimers in drug delivery agents, diagnosis, therapy, and detection. J Nanomater. 2014;2014:39. doi:10.1155/2014/507273
- Dadfar SM, Roemhild K, Drude NI, et al. Iron oxide nanoparticles: diagnostic, therapeutic and theranostic applications. Adv Drug Deliv Rev. 2019;138:302–325. doi:10.1016/j.addr.2019.01.00530639256
- Hornos Carneiro MF, Barbosa JF. Gold nanoparticles: a critical review of therapeutic applications and toxicological aspects. J Toxicol Environ Health Part B. 2016;19(3–4):129–148. doi:10.1080/10937404.2016.1168762
- Medintz IL, Mattoussi H, Clapp AR. Potential clinical applications of quantum dots. Int J Nanomedicine. 2008;3(2):151.18686776
- Ahmed W, Elhissi A, Dhanak V, Subramani K. Chapter 18—carbon nanotubes: applications in cancer therapy and drug delivery research. Emerging Nanotechnol Dent. 2nd ed.; Subramani, K., Ahmed, W., Eds 371–389. doi:10.1016/B978-0-12-812291-4.00018-2
- Baeza A, Ruiz-Molina D, Vallet-Regí M. Recent advances in porous nanoparticles for drug delivery in antitumoral applications: inorganic nanoparticles and nanoscale metal-organic frameworks. Expert Opin Drug Deliv. 2017;14(6):783–796. doi:10.1080/17425247.2016.122929827575454
- Eurov DA, Kurdyukov DA, Stovpiaga EY, et al. Hypersonic properties of monodisperse spherical mesoporous silica particles. J Phys D Applied Phys. 2014;47(33):5. doi:10.1088/0022-3727/47/33/335303
- Farjadian F, Roointan A, Mohammadi-Samani S, Hosseini M. Mesoporous silica nanoparticles: synthesis, pharmaceutical applications, biodistribution, and biosafety assessment. Chem Eng J. 2019;359:684–705. doi:10.1016/j.cej.2018.11.156
- BernArdos A, KouřimsKá L. Applications of mesoporous silica materials in food-a review. Czech J Food Sci. 2013;31(2):99–107. doi:10.17221/240/2012-CJFS
- He Q, Zhang Z, Gao F, Li Y, Shi J. In vivo biodistribution and urinary excretion of mesoporous silica nanoparticles: effects of particle size and PEGylation. Small. 2011;7(2):271–280. doi:10.1002/smll.20100145921213393
- Yang P, Gaib S, Lin J. Functionalized mesoporous silica materials for controlled drug delivery. Chem Soc Rev. 2012;41(9):3679–3698. doi:10.1039/c2cs15308d22441299
- ALOthman ZA. A review: fundamental aspects of silicate mesoporous materials. Materials (Basel). 2012;5(12):2874–2902. doi:10.3390/ma5122874
- Mehmood A, Ghafar H, Yaqoob S, Gohar U, Ahmad B. Mesoporous silica nanoparticles: a review. J Dev Drugs. 2017;6(2):1000174. doi:10.4172/2329-6631.1000174.
- Narayan R, Nayak U, Raichur A, Garg S. Mesoporous silica nanoparticles: a comprehensive review on synthesis and recent advances. Pharmaceutics. 2018;10(3):118. doi:10.3390/pharmaceutics10030118
- Farjadian F, Hosseini M, Ghasemi S, Tamami B. Phosphinite-functionalized silica and hexagonal mesoporous silica containing palladium nanoparticles in Heck coupling reaction: synthesis, characterization, and catalytic activity. RSC Adv. 2015;5(97):79976–79987. doi:10.1039/C5RA16131B
- Duhan S, Tomer VK. Mesoporous silica: making “sense” of sensors. Adv Sensor Detect Mater. 2014;6:149–192. doi:10.1002/9781118774038.ch6
- Karimi M, Mirshekari H, Aliakbari M, Sahandi-Zangabad P, Hamblin MR. Smart mesoporous silica nanoparticles for controlled-release drug delivery. Nanotechnol Rev. 2016;5(2):195–207. doi:10.1515/ntrev-2015-0057
- Cha BG, Kim J. Functional mesoporous silica nanoparticles for bio‐imaging applications. Wiley Interdiscip Rev. 2019;11(1):e1515.
- Abdelsamad AM, Khalil AS, Ulbricht M. Influence of controlled functionalization of mesoporous silica nanoparticles as tailored fillers for thin-film nanocomposite membranes on desalination performance. J Memb Sci. 2018;563:149–161. doi:10.1016/j.memsci.2018.05.043
- Farjadian F, Azadi S, Mohammadi-Samani S, Ashrafi H, Azadi A. A novel approach to the application of hexagonal mesoporous silica in solid-phase extraction of drugs. Heliyon. 2018;4(11):e00930. doi:10.1016/j.heliyon.2018.e0093030456326
- Cashin VB, Eldridge DS, Yu A, Zhao D. Surface functionalization and manipulation of mesoporous silica adsorbents for improved removal of pollutants: a review. Environ Sci. 2018;4(2):110–128.
- Jacobsen D, Haines JA. The relative efficacy of antidotes: the IPCS evaluation series. International Programme on Chemical Safety. Arch Toxicol Suppl. 1997;19:305–310.9079217
- Farjadian F, Ahmadpour P, Samani S, Hosseini M. Controlled size synthesis and application of nanosphere MCM-41 as potent adsorber of drugs: a novel approach to new antidote agent for intoxication. Microporous Mesoporous Mater. 2015;213:30–39. doi:10.1016/j.micromeso.2015.04.002
- Farjadian F, Ghasemi S, Heidari R, Mohammadi-Samani S. In vitro and in vivo assessment of EDTA-modified silica nano-spheres with supreme capacity of iron capture as a novel antidote agent. Nanomed. 2017;13(2):745–753. doi:10.1016/j.nano.2016.10.012
- Kalantari S, Yousefpour M, Taherian Z. Synthesis of mesoporous silica/iron oxide nanocomposites and application of optimum sample as adsorbent in removal of heavy metals. Rare Metals. 2017;36(12):942–950. doi:10.1007/s12598-016-0709-4
- Huang J, Ye M, Qu Y, et al. Pb (II) removal from aqueous media by EDTA-modified mesoporous silica SBA-15. J Colloid Interface Sci. 2012;385(1):137–146. doi:10.1016/j.jcis.2012.06.05422874637
- Ranucci G, Di Dato F, Leone F, Vajro P, Spagnuolo M, Iorio R. Penicillamine-induced elastosis perforans serpiginosa in wilson disease: is useful switching to zinc? J Pediatr Gastroenterol Nutr. 2017;64(3):e72–e73. doi:10.1097/MPG.000000000000061325341025
- Yu H, Xie -J-J, Chen Y-C, et al. Clinical features and outcome in patients with osseomuscular type of Wilson’s disease. BMC Neurol. 2017;17(1):34. doi:10.1186/s12883-017-0818-128212618
- Favre E, Lion-François L, Canton M, et al. Cognitive abilities of children with neurological and liver forms of Wilson disease. J Pediatr Gastroenterol Nutr. 2017;64(3):436–439.27482767
- Walshe J. Penicillamine neurotoxicity: an hypothesis. ISRN Neurol. 2011;2011 10.5402/2011/464572
- Etienne M, Lebeau B, Walcarius A. Organically-modified mesoporous silica spheres with MCM-41 architecture. N J Chem. 2002;26(4):384–386. doi:10.1039/b110741k
- Ho Y-S. Isotherms for the sorption of lead onto peat: comparison of linear and non-linear methods. Pol J Environ Stud. 2006;15:1.
- Farjadian F, Schwark S, Ulbricht M. Novel functionalization of porous polypropylene microfiltration membranes: via grafted poly (Aminoethyl methacrylate) anchored schiff base toward membrane adsorbers for metal ions. Polym Chem. 2015;6(9):1584–1593. doi:10.1039/C4PY01521E.
- Chen Z, Meng H, Xing G, et al. Acute toxicological effects of copper nanoparticles in vivo. Toxicol Lett. 2006;163(2):109–120. doi:10.1016/j.toxlet.2005.10.00316289865
- Heidari R, Mohammadi H, Ghanbarinejad V, et al. Proline supplementation mitigates the early stage of liver injury in bile duct ligated rats. J Basic Clin Physiol Pharmacol. 2019;30(1):91–101. doi:10.1515/jbcpp-2017-0221