Abstract
Polylactide (PLA) electrospun fibers have been reported as a scaffold for bone tissue engineering application, however, the great hydrophobicity limits its broad application. In this study, the hybrid amphiphilic poly(ethylene glycol) (PEG)/hydrophobic PLA fibrous scaffolds exhibited improved morphology with regular and continuous fibers compared to corresponding blank PLA fiber mats. The prepared PEG/PLA fibrous scaffolds favored mesenchymal stem cell (MSC) attachment and proliferation by providing an interconnected porous extracellular environment. Meanwhile, MSCs can penetrate into the fibrous scaffold through the interstitial pores and integrate well with the surrounding fibers, which is very important for favorable application in tissue engineering. More importantly, the electrospun hybrid PEG/PLA fibrous scaffolds can enhance MSCs to differentiate into bone-associated cells by comprehensively evaluating the representative markers of the osteogenic procedure with messenger ribonucleic acid quantitation and protein analysis. MSCs on the PEG/PLA fibrous scaffolds presented better differentiation potential with higher messenger ribonucleic acid expression of the earliest osteogenic marker Cbfa-1 and mid-stage osteogenic marker Col I. The significantly higher alkaline phosphatase activity of the PEG/PLA fibrous scaffolds indicated that these can enhance the differentiation of MSCs into osteoblast-like cells. Furthermore, the higher messenger ribonucleic acid level of the late osteogenic differentiation markers OCN (osteocalcin) and OPN (osteopontin), accompanied by the positive Alizarin red S staining, showed better maturation of osteogenic induction on the PEG/PLA fibrous scaffolds at the mineralization stage of differentiation. After transplantation into the thigh muscle pouches of rats, and evaluating the inflammatory cells surrounding the scaffolds and the physiological characteristics of the surrounding tissues, the PEG/PLA scaffolds presented good biocompatibility. Based on the good cellular response and excellent osteogenic potential in vitro, as well as the biocompatibility with the surrounding tissues in vivo, the electrospun PEG/PLA fibrous scaffolds could be one of the most promising candidates in bone tissue engineering.
Introduction
Bone defects resulted from trauma, abnormalities, infection, or tumor resection have presented a severe challenge for the regeneration of cartilage, bone, and teeth in the tissue regeneration field. As the traditional treatments for bone lesions, autologous bone grafts are restricted because of donor site morbidity and potential secondary wounds, while allogeneic bone grafts are limited because of the risk of potential transmission of pathogens.Citation1 As a consequence, various bone substitutes with similar structure and function to autologous bones have been proposed. As temporary templates for cell seeding, migration, proliferation, and differentiation prior to the regeneration of natural extracellular matrix (ECM) or biological functional tissue,Citation2,Citation3 biomaterial scaffolds for bone tissue engineering applications require consideration of proper toughness for cell adhesion, porous three-dimensional structure for cell migration and new bone tissue ingrowth, as well as good osteoconductivity to promote bone repair.Citation4,Citation5 In recent years, electrospun fibers as bone scaffolds have received great attention for the following reasons: (1) their morphologies are similar to the structures of natural ECM, (2) their intrinsic large surface area-to-volume ratios offer sufficient space for cell adhesion and proliferation, and (3) their interconnected porous structures can provide windows for nutrient and metabolic waste exchange,Citation6,Citation7 as well as promote vascularization to apply nutrient and drain the consumed metabolites in vivo.Citation8
The electrospinning technique was originally developed by Zeleny in 1914,Citation9 and was first patented in the 1930s.Citation10 Electrospinning is a remarkably simple and versatile technique capable of producing microscale or nanoscale polymer fibers directly from a variety of polymers and composite materials. However, there was little interest in electrospinning or electrospun fibers outside of the filter industry until the mid-1990s.Citation11 As a remarkably simple and versatile technique, electrospinning has received great attention in a broad range of biomedical applications, such as sensors,Citation12 photovoltaic cells,Citation13 chemically- and biologically-resistant clothing, carriers for drug delivery system, barriers for the prevention of adhesion, substrates for wound dressing, supports for biocatalysis, active components for biosensing,Citation14 and especially as scaffolds for tissue engineering during the past two decades. To be successfully applied in bone tissue engineering, electrospun fibers essentially need to have good cell responses and osteoconductivity. In fact, electrospun nonwoven polymer fabrics have been extensively applied as scaffolds for bone-like cellsCitation15–Citation17 and mesenchymal stem cells (MSCs)Citation18,Citation19 based on the advantages of operation facility, easy accommodation, and interconnected porous structures. Furthermore, some studies have certified that electrospun fibers alone can be tailored to fabricate functional scaffolds in bone tissue engineering and enhance bone regeneration to a certain extent without any inflammatory reaction.Citation11,Citation20
During recent decades, many electrospun polymers have been used for bone tissue engineering, which include both nonbiodegradable and biodegradable polymers either with a synthetic or natural origin. Among them, nonbiodegradable electrospun polymers such as polyurethane and polyester urethane could interfere with tissue turnover and remodeling due to their slow degradation, even though they possessed substantial mechanical stability.Citation21,Citation22 Many biodegradable electrospun polymers have been fabricated such as collagen,Citation23 chitosan,Citation24 poly(glycolic acid),Citation25 polylactide (PLA),Citation26 poly(ɛ-caprolactone),Citation27 poly(lactide-co-glycolide),Citation28 and polyanhydride.Citation29 Among the biodegradable electrospun polymers, PLA has been used clinically in bone repair due to its tunable mechanical properties, and it can degrade gradually to natural metabolites along with the construction of new tissue and finally be replaced by the new tissue. However, based on their inherent hydrophobic nature, the resorbable biocompatible PLA scaffolds may reduce expression of phenotypic markers by adherent osteoblasts,Citation30 and further influence cell attachment and proliferation due to the denaturation of adhesive ligands of cells,Citation24,Citation31 which are far from desirable. During recent years, some efforts have been undertaken to graft hydrophilic moieties in order to make PLA scaffolds more conducive for cell attachment, proliferation, and differentiation. As reported, the blending of amphiphilic polyether poly(ethylene glycol) (PEG) to PLA can modulate the inherent hydrophilicity property, crystallinity, tensile strength, and degradation behaviors.Citation32 Accordingly, electrospun hybrid scaffolds were prepared in this study by blending PLA with amphiphilic PEG as filler to tailor the scaffolds’ hydrophilic properties, and to improve cell responses such as cell attachment, proliferation, and osteoblastic differentiation.Citation33,Citation34
MSCs isolated from bone marrow are multipotent cells that can be induced to differentiate into a variety of mesenchymal tissues including bone, cartilage, tendon, fat, and muscle both in vitro and in vivo.Citation1 MSCs have been widely applied in tissue engineering because they are easy to get and manipulate, can be easily differentiated, are biocompatible almost without immune response or tumorigenesis, and especially because they pose no ethical or legal problems.Citation35 Therefore, MSCs are well suited to act as seed cells for successful tissue renewal and replacement of damaged and diseased musculoskeletal tissues in bone tissue engineering.Citation36
In this study, the uniform and continuous PEG/PLA fibrous scaffolds presented good cell responses and excellent osteogenic ability in the MSCs differentiation procedure. For further application in bone repair in vivo, the biocompatibility of the fibrous scaffolds were specially examined with hematoxylin and eosin staining after being transplanted into the thigh muscle pouches of rats. All the results demonstrated that the PEG/PLA hybrid fibrous scaffolds showed ideal potential for broad application in bone tissue engineering.
Materials and methods
Materials
Semicrystalline grade PLA 4032D with approximately 2% of D-lactic acid monomer used in this study was supplied by NatureWorks LLC (Minneapolis, MN). PEG (number average molecular weight = 4000) was purchased from Sigma-Aldrich (St Louis, MO). PLA and PEG were dried in a vacuum oven at 110°C and 50°C for 24 hours before use, respectively. Dichloromethane was obtained from Kelong Chemical (Chengdu, China) and used as solvent. All other used chemical agents were of analytical reagent grade.
Electrospinning process
A known amount of dried PEG and PLA (PEG/PLA = 5/95) were dissolved in dichloromethane with vigorous stirring for 6 hours to form a 60 mg/mL transparent and homogeneous solution. PEG/PLA solution was electrospun at a high voltage of 18 kV driven by a high voltage power supply (High Voltage Technology Institute, Beijing, China) under 60% humidity at room temperature. The obtained solution was directly transferred into a 20 mL plastic syringe (Becton, Dickinson and Company, East Rutherford, NJ) at the feeding rate of 6 mL/hour, which was set by a syringe pump (Smiths Medical Instrument Company, Zhejiang, China). The spinneret was capped with a blunt-end needle with an inner diameter of 0.5 mm, and the distance between the spinneret and the grounded aluminum foil collector was 12 cm. Pure PLA fibers were also prepared in the same processing conditions for comparison. All obtained electrospun fibrous scaffolds were dried under vacuum for 48 hours to eliminate any potential residual solvents and kept in a desiccator for further characterization and application.
Morphological characterization
In order to investigate the surface morphologies and the micro-structure of the electrospun PEG/PLA hybrid fibrous scaffold, scanning electron microscopy (SEM) analysis was conducted (JSM-5900 Low Vacuum SEM; JEOL, Tokyo, Japan). After being seeded with cells for predetermined time, the fibrous scaffolds were fixed using 2.5% glutaraldehyde overnight at 4°C. Subsequently, they were dehydrated in ethanol with a series of concentrations (30%, 50%, 70%, 80%, 90%, 95%, and 100%) and dried in vacuum. All the samples were sputter-coated with a thin layer of gold using an SBC-12 Ion Sputtering Coater (KYKY Technology Development Ltd, Beijing, China) and observed at 20 kV accelerating voltage. The average diameters of the electrospun fibers were obtained by analyzing at least 40 measurements randomly per specimen.
Isolation and culture of rat bone marrow MSCs
The neonate Sprague Dawley rats (3 days old) were purchased from the Laboratory Animal Center of Sichuan University (Chengdu, China). The experiments were performed based on the Principles of Laboratory Animal Care formulated by the National Society for Medical Research and the Guide for the Care and Use of Laboratory Animals,Citation37 and all protocols were operated according to the Animal Care and Use Committee of West China Hospital, Sichuan University. The fresh femur were harvested aseptically from the neonate rats which were anesthetized and sterilized with alcohol and povidine. Citation38 The bone marrow was flushed out with Dulbecco’s modified Eagle’s medium (Life Technologies, Carlsbad, CA) supplemented with 10% fetal bovine serum (Thermo Fisher Scientific, Waltham, MA), 100 unit/mL penicillin (Life Technologies) and 100 μg/mL streptomycin (Life Technologies) using a 1 mL syringe. The obtained solution was cultured in a 75 cm2 tissue culture flask at 37°C, 5% carbon dioxide. Culture medium was changed every 3–4 days, and MSCs approached confluency after about 1 week. Rat bone MSCs were trypsinized briefly with 0.25% trypsin and subcultured at 1:3 ratio. In this study, passage-3 MSCs were used for research on cell adhesion, proliferation, and differentiation. For osteogenic differentiation, MSCs were cultured in osteogenic culture medium, which was Dulbecco’s modified Eagle’s medium supplemented with 10% fetal bovine serum, 100 unit/mL penicillin, 100 μg/mL streptomycin, 50 g/mL ascorbic acid, 10 mM glycerophosphate, and 10−8 M dexamethasone (Sigma-Aldrich).
Cell viability evaluation and fluorescence microscopy observation
The viability of MSCs cultured on the PEG/PLA hybrid fibrous scaffold was evaluated by 3-(4,5-dimethylthiazol- 2-yl)-2,5- diphenyltetrazolium bromide assay. The mechanism of this assay is that metabolically active cells react with a tetrazolium salt to produce a soluble formazan dye. The rat bone MSCs were cultured on the electrospun fibrous scaffolds at 37°C under 5% carbon dioxide for the predetermined time. Subsequently, 20 μL of 3-(4,5-dimethylthiazol-2-yl)-2,5- diphenyltetrazolium bromide stock solution (5 mg/mL) in phosphate buffered saline (PBS) was added to each well and the cells were further incubated for another 4 hours. The precipitated formazan was dissolved in 100 μL dimethyl sulfoxide, and the absorbance at 570 nm was measured with spectrophotometer (Spectronic™ 20D+; Thermo Fisher Scientific). Experiments were repeated in triplicate.
In order to be observed by fluorescence microscopy (DM IL; Leica Microsystems, Wetzlar, Germany), the rat bone MSCs were stained by fluorescein diacetate and 2-(4-amidinophenyl)-6-indolecarbamidine dihydrochloride (Sigma-Aldrich), and cell morphology was also observed by optical microscopy.
In vitro MSCs differentiation study
Messenger ribonucleic acid (mRNA) extraction and real-time polymerase chain reaction (PCR) analysis
The total RNA of cultured MSCs was isolated using TRIzol® Reagent (Life Technologies) according to the manufacturer’s protocol. Briefly, 1 μg total RNA was first reversely transcribed to complementary DNA by Moloney Murine Leukemia Virus Reverse Transcriptase (Life Technologies). Then the real-time PCR reaction was carried out in a 96-well format with Taq polymerase (Thermo Fisher Scientific) and the SYBR® Premix Ex Taq™ II (Perfect Real-Time) PCR kit (Takara Biotechnology, Dalian, China) on a DNA Engine® PTC-200 Thermal Cycler (Bio-Rad Laboratories, Hercules, CA). The primers of differentiation markers are listed in . The levels of β-actin mRNA in each sample were used as an internal control, relative quantities were analyzed by 2−ΔΔCt method. All reactions were carried out in triplicate and the results were analyzed by Gene Expression Analysis for iCycler iQ® Real-Time PCR Detection System (Bio-Rad). The products were visually analyzed on 2% agarose gel.
Table 1 Nucleotide primers used for reverse transcription polymerase chain reaction
Alkaline phosphatase (ALP) activity assay
MSCs were cultured in osteogenic culture medium, and their ALP activity was assessed on day 4, 7, 14, and 21. The cultured cell layer was rinsed twice with PBS 1× and lysed by ALP buffer (GenMed LLC, San Diego, CA). Protein content was estimated using the Bradford assay method (GenMed). The hydrolysis of a colorless organic phosphate ester p-nitrophenyl phosphate to the yellow p-nitrophenol was catalyzed by ALP. The absorbance of the yellow product was measured at 405 nm using a spectrophotometer (Spectronic™ 20D+; Thermo Fisher Scientific). ALP activity was also visually stained using reagent B as AP-Red concentrated solution and reagent A as AP-Red accentuator according to the manufacturer’s protocol (ZSGB-BIO, Inc, Beijing, China). The cultured cells were rinsed twice with PBS 1× and immersed in the alkaline-dye mixture for 10 minutes at room temperature. Subsequently, the dyed cells were rinsed in deionized water and investigated microscopically.
Alizarin red S (ARS) staining and quantitation
ARS staining was used to quantify the mineralization of MSCs grown on the PEG/PLA hybrid fibrous scaffold with a glass coverslip as a fiber-supporting substrate, and cells on the glass coverslip alone were included as a control. The cell-scaffold construct was washed twice with PBS 1× and fixed with 10% formaldehyde for 10 minutes. After being washed with PBS 1×, the constructs were incubated with the staining solution (1% ARS in 2% ethanol) for 5 minutes. Subsequently, the staining solution was removed and the constructs were washed three times with distilled water to get rid of excessive color. To quantify the mineralization, the constructs were desorbed using 10% cetylpyridinium chloride (Sigma-Aldrich) for 1 hour. The solution was collected and the absorbance at 570 nm was measured with the spectrophotometer, which was performed in triplicate wells.
Histological analysis in vivo
To further evaluate the tissue responses to the implanted scaffold in vivo, the PEG/PLA fibrous scaffold was transplanted into the thigh muscle pouches of rats. All animal care and experimental procedures were conducted according to institutional Animal Care and Use guidelines. Briefly, the Sprague Dawley rats (4 weeks old, 200 ± 20 g) were first anesthetized with intraperitoneal injection of chloral hydrate (0.3 mL/100 g) until effectively anesthetized. Subsequently, the PEG/PLA fibrous scaffold was transplanted into the thigh muscle pouches. Three rats were randomly sacrificed at predetermined time of 2 weeks and 4 weeks, and the excised implant and surrounding muscle were fixed in 10% formalin for histological analysis. The fixed specimens were serially dehydrated in a graded series of ethanol washes and embedded in paraffin, then eight micron-thick sections were cut and stained according to standard protocols for hematoxylin and eosin staining. All hematoxylin and eosin results were analyzed with a digital image analysis system (Eclipse E600 microscope with DXM 1200 digital camera; Nikon Corporation, Tokyo, Japan).
Statistics
Statistical analysis was performed using the GraphPad Prism (v 5.0; GraphPad Software, Inc, La Jolla, CA) and the statistical significance of the difference between groups was found by one-way analysis of variance. Quantitative data were represented as mean ± standard deviation and statistical significance was set at P < 0.05.
Results
Morphology analysis of the electrospun PEG/PLA fibrous scaffolds by SEM
The morphology of the electrospun fibrous scaffolds was observed by SEM (). Under optimized electrospinning conditions (eg, solvent, solution concentration), PEG/ PLA (PEG/PLA = 5/95, optimized by the authors, data not shown) hybrid solution was electrospun to form smooth uniform fibers (). The average diameter of the PEG/PLA electrospun fibers obtained was 2.01 ± 0.32 μm, which was one to two orders of magnitude smaller than mammalian cells, and included the range of feature sizes known to facilitate contact guidance.Citation39 For pristine PLA fiber mats as presented in , the diameter of the electrospun fibers had a broad distribution ranging from 200 nm to 5 μm, some with spindle-shaped structures. The irregular and discontinuous fibers may be due to the intrinsic properties of PLA solution such as hydrophobicity, viscosity, and surface tension.Citation28,Citation29 The spun PLA/PEG fibers were long and continuous, which may be attributed to the reduction of solution viscosity caused by the use of the low molecular weight PEG as lubricant.
Cell behavior of MSCs on the PEG/PLA fibrous scaffolds
Cell morphology and spreading, as well as cell interaction with the electrospun fibrous scaffolds were studied by SEM (). On the day following cell seeding (), MSCs had already expanded on the surface of the electrospun fibrous scaffolds, and the anchoring ligands of the cells had stretched out to elongate along individual fibers thinner than themselves. Meanwhile, the seeded cells were thin and narrow in shape, and adhered to a relatively small area of the scaffold.Citation40 Subsequently, MSCs started to migrate through the pores and integrated well with the surrounding fibers on day 3 (). Moreover, it became extremely difficult to determine the exact boundary of a single cell since the cells grew massively and formed a continuous layer. MSCs on the fibrous scaffolds expanded even more and almost reached confluence after 5 days in culture (). Interestingly, MSCs began to penetrate into the fibrous scaffold through the interstitial pores between the fibers and grow underneath the fiber network from day 3. The penetration of cells into the fibrous scaffolds was significantly meaningful for the cytocompatibility evaluation and very important for favorable application in tissue engineering. This is because the functional tissue can only be regenerated when cells migrate through the scaffolds, but not when they just stay on the scaffold surface.Citation41
Figure 2 Scanning electron microscope observations of rat bone mesenchymal stem cell adhesion and growth on the electrospun poly(ethylene glycol)/polylactide hybrid membranes for 1 day (A, B), 3 days (C, D), and 5 days (E, F) at different magnification (A, C, E: 500×; B, D, F: 1000×).

Cell viability and metabolic activity of MSCs cultured on the PEG/PLA electrospun fibrous scaffolds showed a statistically significant increase compared with those on the tissue culture plate 1 day and 3 days after seeding (). However, the cells on the scaffolds proliferated slightly up to day 5 when the MSCs began to differentiate, which is in accordance with osteoblast proliferation and differentiation having conflicting properties.Citation42 The higher cell viability for the PEG/PLA electrospun fibrous scaffolds demonstrates that the electrospinning scaffolds can accelerate initial attachment of the cells and promote cells to penetration into fiber substrates, which is important for the application of biomaterial scaffolds.Citation4,Citation33 In order to further confirm cytocompatibility of the PEG/PLA electrospun fibrous scaffolds, fluorescent microscopic images of MSCs grown on the scaffolds were taken. As shown in , the cells were observed to adhere and spread actively with a physiological adherence pattern on both the tissue culture plate and the fibrous scaffolds.
Figure 3 Mesenchymal stem cells viability and metabolic activity evaluation on the poly(ethylene glycol)/polylactide hybrid membranes, with the tissue culture plate as the control.
Note: Error bars represent standard deviation; n = 3.
Abbreviations: PEG/PLA, poly(ethylene glycol)/polylactide; OD, optical density.
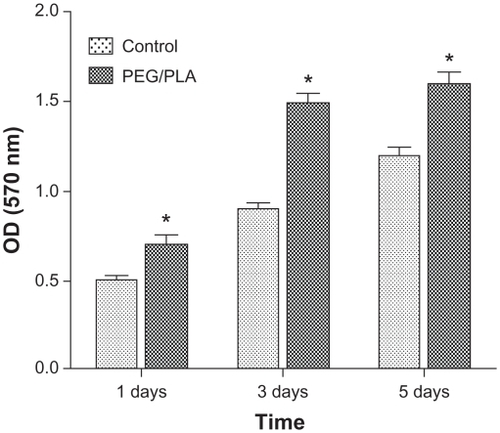
Figure 4 Fluorescence micrographs show the cytocompatibility of the poly(ethylene glycol)/polylactide electrospun fibrous scaffolds with mesenchymal stem cells (C, D). Mesenchymal stem cells cultured on the tissue culture plate were the control (A, B). The cells were stained with fluorescein diacetate in green color (A, C) and 2-(4-amidinophenyl)-6-indolecarbamidine dihydrochloride for cell nuclei in blue (B, D). Mesenchymal stem cells on the poly(ethylene glycol)/polylactide electrospun fibrous scaffolds under white light were also included at different magnification (E, F).
Note: Scale bar = 100 μm.
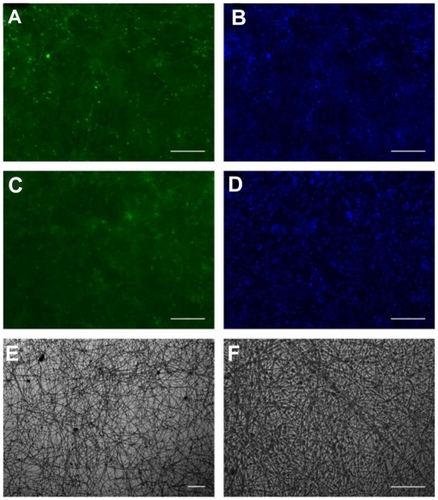
MSCs osteogenic development on the PEG/PLA fibrous scaffolds
In addition to attachment and proliferation, the ability to support cultured cell differentiation is another actual applicability of the scaffold. By analyzing the genes of different clusters and their expression profiles, there were three main phases of osteogenic development procedure as reported: proliferation, matrix maturation, and mineralization,Citation43 as shown in .
Figure 5 Graphical summary of the three phases and time schedule of osteogenic induction: proliferation, matrix maturation, and mineralization.
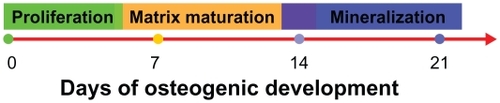
Gene expression profile during osteogenic procedure
To confirm the osteogenic differentiation of MSCs on the PEG/PLA fibrous scaffolds, induction of osteoblast-specific genes was examined by real-time polymerase chain reaction (PCR) (). As the earliest osteogenic marker, the transcriptional factor Cbfa-1 drove the pluripotent MSCs to preosteoblasts by initiating the expression of many bone specific genes.Citation44 Col I comprised 90%–95% of the organic material of mineralized ECM and served as a template upon which mineral deposited, which was well known as one of the mid-stage osteogenic markers.Citation45 The expression of Cbfa-1 and Col I was upregulated during the whole period of differentiation; maximum expression was reached on day 21. Furthermore, the expression of Cbfa-1 and Col I of MSCs on the scaffolds was significantly higher than those on the glass coverslip throughout differentiation. The maturation of differentiation was accomplished with the secretion of the late osteogenic differentiation markers OCN (osteocalcin) and OPN (osteopontin). After 14 days of osteogenic induction, the secreted OCN and OPN on the scaffolds showed much higher expression compared with the control. By evaluating the expression of the osteoblast phenotypic markers, MSCs cultured on the PEG/PLA fibrous scaffolds presented better osteogenic differentiation ability.
Figure 6 Gene expression profile of rat bone mesenchymal stem cells in osteogenic cultures. (A) Agarose gel electrophoresis result of the real-time polymerase chain reaction product shows the effects of the culture scaffold on gene expression on day 7, 14, and 21. (B) Quantitative analysis for Col I, Cbfa-1, OCN, and OPN expression was performed by Gene Expression Analysis for iCycler iQ® Real-Time PCR Detection System (Bio-Rad Laboratories, Hercules, CA); relative gene expression levels were normalized by the housekeeping gene, β-actin.
Note: *Represents statistically significant difference (P < 0.05), n = 3.
Abbreviation: PEG/PLA, poly(ethylene glycol)/polylactide.

ALP activity analysis
As a well-known marker of the osteogenic phenotype, ALP is a glycoprotein on the cell membrane that catalyzes the hydrolysis of phosphate esters at an alkaline pH and plays an important role in bone matrix mineralization process.Citation46 In this study, ALP activity was visualized by ALP staining () on day 14 and evaluated quantitatively in terms of μmol of p-nitrophenyl phosphate production per mg of protein per hour () on day 4, 7, 14, and 21. ALP expression is known to peak after the end of the proliferative stage and before matrix maturation during osteoblastic differentiation.Citation47 As presented in , ALP activity of MSCs cultured both on glass coverslip and fibrous scaffolds presented an ongoing increase with culture time up to 14 days and then a decrease on day 21, which suggested that osteodifferentiation was occurring. Additionally, ALP activity was significantly higher for the PEG/PLA fibrous scaffolds than the control from day 7, which indicated that the presence of the bone-like matrix enhanced differentiation from MSCs to osteoblast-like cells.
Figure 7 Alkaline phosphatase activity expressed by the cells after culturing for 4, 7, 14, and 21 days. A glass coverslip was used as a fiber-supporting substrate and data on the glass coverslip was included as a control. (A) Alkaline phosphatase activity quantitative evaluation on day 4, 7, 14, and 21. Alkaline phosphatase staining was also performed on control (B) and poly(ethylene glycol)/polylactide hybrid scaffolds (C) on day 14.
Notes: Scale bar = 50 μm.*Represents statistically significant difference (P < 0.05), n = 3.
Abbreviations: PEG/PLA, poly(ethylene glycol)/polylactide; pNP, p-nitrophenyl phosphate.
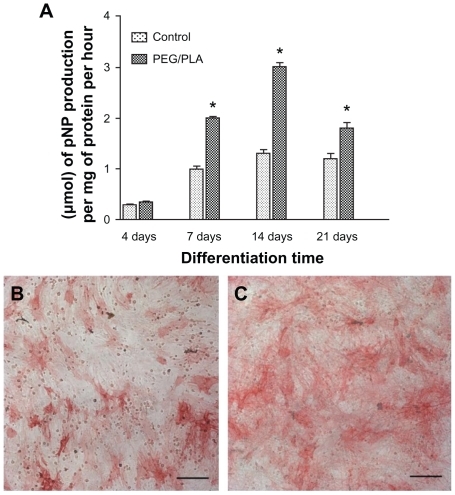
ARS staining and mineralization quantification
The ARS dye binds to calcium salts selectively and is widely used for calcium mineral histochemistry. present the images of ARS staining of MSCs cultured on glass coverslip and PEG/PLA fibrous scaffolds on day 21 in osteogenic culture medium. The red stains (bone nodules) were ARS–calcium chelating products.Citation48 As shown in , the cells cultured on PEG/PLA fibrous scaffolds clearly showed matrix mineralization with more intense ARS staining than the control. To quantify mineralization, constructs were desorbed using 10% cetylpyridinium chloride for 1 hour, and the absorbance of the solution was measured at 570 nm (n = 3). The results of the absorbance analysis quantitatively confirmed the higher calcium deposition of the PEG/PLA fibrous scaffolds (, P < 0.05).
Figure 8 Analysis of the mineral deposition in osteogenic mesenchymal stem cells on day 21 by Alizarin Red S staining. Photographs of the Alizarin Red S staining show higher calcium deposition of the poly(ethylene glycol)/polylactide fibrous scaffolds with a glass coverslip as the fiber-supporting substrate (A) than on the glass coverslip as control (B). The quantification result confirmed the higher Alizarin Red S staining of the poly(ethylene glycol)/polylactide fibrous scaffolds (C).
Note: *Represents statistically significant difference (P < 0.05), n = 3.
Abbreviations: PEG/PLA, poly(ethylene glycol)/polylactide; OD, optical density.
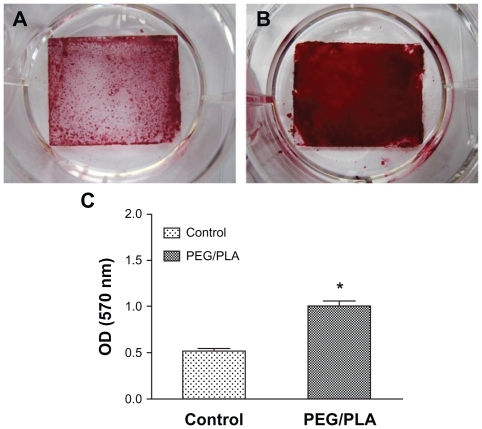
On the whole, the PEG/PLA fibrous scaffolds presented good cellular compatibility and excellent osteogenic potential in vitro. Therefore, they could be one of the most promising candidates for potential application in tissue engineering.
Histologic analysis of tissue response to the PEG/PLA fibrous scaffolds
The PEG/PLA fibrous scaffold was transplanted into the thigh muscle pouches of rats, and the histologic results were analyzed to evaluate the tissue responses in vivo. The host response was determined by measuring the inflammatory cells, ie, macrophage, polymorphonuclear leukocyte, lymphocyte, plasma cell, and giant cell. As depicted in , an acute inflammation reaction occurred at week 2, and the inflammatory cells infiltrated into the PEG/PLA fibrous scaffold (3815 ± 317/mm2). However, the inflammation reaction attenuated dramatically and the number reduced to 201 ± 84/mm2 at 4 weeks ().Citation5 Meanwhile, the surrounding muscle maintained its physiological characteristics the whole time, as confirmed by both macroscopic observation (data not shown) and histologic analysis (). In general, the PEG/PLA fibrous scaffold presented good biocompatibility in vivo.
Figure 9 Histologic analysis of tissue response to poly(ethylene glycol)/polylactide fibrous scaffolds. There was a dense accumulation of inflammatory cells presented around the poly(ethylene glycol)/polylactide fibrous scaffolds at 2 weeks (A) but attenuated at week 4 (B). Meanwhile, the surrounding muscle presented good physiological characteristics both at 2 weeks (C) and 4 weeks (D).
Note: Scale bar = 50 μm.
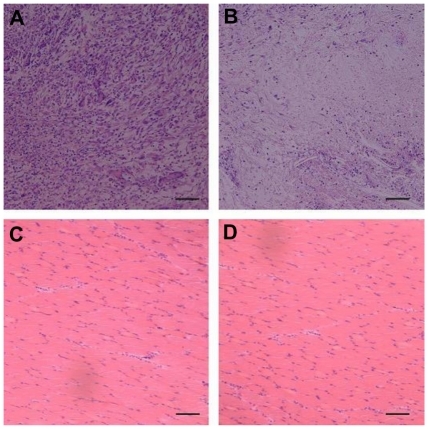
Discussion
When used as bone tissue engineering scaffolds, electrospun fibers offer a range of attractive features such as large surface area-to-volume ratios, interconnected porous structures, ease of construction into different shapes, and similar structures to natural ECM. The advantages mentioned above made the electrospun fibers ideal for cell adhesion and proliferation as well as for nutrient and metabolic waste exchange. Meanwhile, bone marrow MSCs are multipotent cells that have been considered as ideal seed cells in the bone repair.Citation49 Therefore, the aim in the present study was to evaluate the cell response of obtained PEG/PLA electrospun hybrid scaffolds to MSCs, and the osteogenic development behavior of MSCs cultured on the PEG/PLA electrospun fibrous scaffold in vitro. Surrounding tissue response to the fibrous scaffolds in vivo was also evaluated by transplanting the PEG/PLA electrospun hybrid scaffold into the thigh muscle pouches of rats.
As shown by SEM, the average diameter of the uniform and continuous hybrid PEG/PLA fibrous scaffolds was one to two orders of magnitude smaller than mammalian cells, which included the range of feature sizes to facilitate contact guidance.Citation33 The diameter of the pure PLA electrospun fibers had a broad distribution ranging from 200 nm to 5 μm, some with spindle-shaped structures. It was presumed that hybrid PEG/PLA fibrous scaffolds would present good cell response and facilitate osteoblastic differentiation.
To validate the cell response of PEG/PLA fibrous scaffolds, the behavior of MSCs cultured on the scaffolds, including the cell morphology and cell interaction with the electrospun fibrous scaffolds by SEM, cell viability, and metabolic activity by 3-(4,5-dimethylthiazol-2-yl)- 2,5-diphenyltetrazolium bromide accompanied with the fluorescent microscopic images, were evaluated. MSC behavior confirmed the presumption that MSCs interacted and integrated well with surrounding fibers. Moreover, MSCs attached and proliferated better on the scaffolds than on the glass coverslip. As reported, the cells gradually penetrated into the fibrous structures composed of large diameter fibers due to the large pore area of the fibrous structures, but failed to penetrate into the fibrous structures composed of small diameter fibers.Citation27 As the interstitial pore sizes of obtained PEG/PLA fibrous scaffolds were larger than 10 μm, it was possible for MSCs to fully penetrate into the fibrous scaffolds through these pores. MSCs on the scaffolds formed a continuous layer and even migrated through the pores to grow underneath the fiber network, which is significant for favorable application in tissue engineering as the functional tissue would be regenerated through the scaffolds and not just on the scaffold surface.
In addition to attachment and proliferation, the osteoinductive capacity of a scaffold to support differentiation of cultured cells is another important aspect suggesting actual applicability of the scaffold. Of the several kinds of osteogenic cells, bone marrow MSCs seem to be the optimal seeding cells for bone tissue engineering owing to the advantages mentioned above. As briefly depicted in , there were three main phases of osteogenic development: proliferation, matrix maturation, and mineralization. As reported, the blending of PEG to PLA can modulate the hydrophilicity property and significantly enhance osteoblastic differentiation.Citation33,Citation34 Representative markers of the osteogenic procedure were comprehensively analyzed by mRNA quantitation and protein analysis (–). As the earliest osteogenic marker, mRNA level of Cbfa-1 was upregulated during the whole differentiation period and reached maximum expression on day 21. The transcriptional factor Cbfa-1 can drive the pluripotent MSCs to preosteoblasts by initiating the expression of many bone specific genes, so the higher expression of Cbfa-1 for MSCs on the PEG/PLA fibrous scaffolds presented better differentiation potential. The well-known mid-stage osteogenic markers were Col I and ALP. The higher Col I mRNA level provided a favorable template for the mineral deposition for the reason that the Col I comprised 90%–95% of the organic material of mineralized ECM. The bone-forming potential of MSCs was further analyzed by evaluating the expression of ALP activity, since ALP is regarded to be an important phenotype of bone-forming cells. Of particular note, ALP activity of MSCs at all evaluation times was significantly higher on the PEG/PLA fibrous scaffolds than on the pure glass coverslip (). At the mineralization stage of differentiation, the maturation of the osteogenic induction was confirmed by the high expression of the late osteogenic differentiation markers OCN and OPN accompanied with positive ARS staining (). In accordance with previous results, the expression of the late osteogenic differentiation markers confirmed the presumption that the PEG/PLA fibrous scaffolds is better at encouraging cells to differentiate into bone-associated cells in vitro, which will ultimately be useful as bone constructs in bone tissue engineering.
Based on the good cellular compatibility and excellent osteogenic potential, an experiment on surrounding tissue response to the PEG/PLA fibrous scaffolds in vivo was briefly performed. Acute inflammation reaction presented at 2 weeks, but attenuated at week 4 without unfavorable influence on the ambient muscle, which indicated that the PEG/ PLA fibrous scaffolds can get along well with surrounding tissues in vivo.
On the whole, the obtained PEG/PLA fibrous scaffolds could be one of the best potential bone implant candidates in bone tissue engineering. However, to confirm the practical usefulness of this novel PEG/PLA hybrid fibrous scaffold, more in-depth studies on the hybridized fibers are under way, in particular animal tests to find clinical applications such as guided bone regeneration and tissue engineering matrices.
Conclusion
In this study, uniform PEG/PLA fibrous scaffolds were successfully prepared by electrospinning and they exhibited improved morphology with regular and continuous fibers compared to corresponding pristine PLA fiber mats. The obtained PEG/PLA fibrous scaffolds favored MSC attachment and proliferation, furthermore, MSCs penetrated into the fibrous scaffold through the interstitial pores which was meaningful for cytocompatibility evaluation. After comprehensively analyzing the representative markers of the osteogenic procedure at mRNA level and protein level in vitro, as well as the histologic result of the tissue responses in vivo, it can be concluded that the prepared PEG/PLA fibrous scaffolds present excellent osteogenic potential and prospective use for bone tissue engineering.
Acknowledgments
This work was financially supported by the National 863 project (2007 AA021902) and New Century Excellent Talents in University (NCET-08-0371). We would like to give warm thanks to Wang Hui (Analytical and Testing Center, Sichuan University) for her great help in SEM analysis and Ge Yan (Regenerative Medicine Research Center, West China Hospital, Sichuan University) for her kind guidance in the preparation of MSCs.
Disclosure
The authors report no conflict of interest in this work. The authors are solely responsible for the content and writing of the paper.
References
- DongSWYingDJDuanXJBone regeneration using an acellular extracellular matrix and bone marrow mesenchymal stem cells expressing Cbfa1Biosci Biotechnol Biochem200973102226223319809195
- LutolfMPHubbellJASynthetic biomaterials as instructive extracellular microenvironments for morphogenesis in tissue engineeringNat Biotechnol2005231475515637621
- MartinsAChungSPedroAJHierarchical starch-based fibrous scaffold for bone tissue engineering applicationsJ Tissue Eng Regen Med200931374219021239
- FangRZhangEXuLWeiSElectrospun PCL/PLA/HA based nanofibers as scaffold for osteoblast-like cellsJ Nanosci Nanotechnol201010117747775121138024
- MunchELauneyMEAlsemDHSaizETomsiaAPRitchieROTough, bio-inspired hybrid materialsScience200832259071516152019056979
- PhamQPSharmaUMikosAGElectrospinning of polymeric nanofibers for tissue engineering applications: a reviewTissue Eng20061251197121116771634
- KangYMKimKHSeolYJRheeSHEvaluations of osteogenic and osteoconductive properties of a non-woven silica gel fabric made by the electrospinning methodActa Biomater20095146246918676190
- RouwkemaJRivronNCvan BlitterswijkCAVascularization in tissue engineeringTrends Biotechnol200826843444118585808
- ZelenyJThe electrical discharge from liquid points, and a hydrostatic method of measuring the electric intensity at their surfacesPhys Rev1914326991
- FormhalsAProcess and apparatus for preparing artificial threadsUnited States patent 1 975 5041934
- XieJLiXXiaYPutting electrospun nanofibers to work for biomedical researchMacromol Rapid Commun200829221775179220011452
- HeWYongTTeoWEMaZRamakrishnaSFabrication and endothelialization of collagen-blended biodegradable polymer nanofibers: potential vascular graft for blood vessel tissue engineeringTissue Eng2005119–101574158816259611
- Heydarkhan-HagvallSSchenke-LaylandKDhanasoponAPThree-dimensional electrospun ECM-based hybrid scaffolds for cardiovascular tissue engineeringBiomaterials200829192907291418403012
- DzenisYMaterial science. Spinning continuous fibers for nanotechnologyScience200430456791917191915218134
- ChiuJBLiuCHsiaoBSChuBHadjiargyrouMFunctionalization of poly(L-lactide) nanofibrous scaffolds with bioactive collagen moleculesJ Biomed Mater Res A20078341117112717593546
- WutticharoenmongkolPPavasantPSupapholPOsteoblastic phenotype expression of MC3T3-E1 cultured on electrospun polycaprolactone fiber mats filled with hydroxyapatite nanoparticlesBiomacromolecules2007882602261017655356
- MeechaisueCWutticharoenmongkolPWaraputRPreparation of electrospun silk fibroin fiber mats as bone scaffolds: a preliminary studyBiomed Mater20072318118818458470
- XinXHussainMMaoJJContinuing differentiation of human mesenchymal stem cells and induced chondrogenic and osteogenic lineages in electrospun PLGA nanofiber scaffoldBiomaterials200728231632517010425
- ShihYRChenCNTsaiSWWangYJLeeOKGrowth of mesenchymal stem cells on electrospun type I collagen nanofibersStem Cells200624112391239717071856
- KimKHJeongLParkHNBiological efficacy of silk fibroin nanofiber membranes for guided bone regenerationJ Biotechnol2005120332733916150508
- LeeCHShinHJChoIHNanofiber alignment and direction of mechanical strain affect the ECM production of human ACL fibroblastBiomaterials200526111261127015475056
- RiboldiSASampaolesiMNeuenschwanderPCossuGManteroSElectrospun degradable polyesterurethane membranes: potential scaffolds for skeletal muscle tissue engineeringBiomaterials200526224606461515722130
- NieHSohBWFuYCWangCHThree-dimensional fibrous PLGA/ HAp composite scaffold for BMP-2 deliveryBiotechnol Bioeng200899122323417570710
- JiangHFangDHsiaoBSChuBChenWOptimization and characterization of dextran membranes prepared by electrospinningBiomacromolecules20045232633315002991
- OuchiTIchimuraSOhyaYSynthesis of branched poly(lactide) using polyglycidol and thermal, mechanical properties of its solution-cast filmPolymer2006471429434
- CuiYLQiADLiuWGBiomimetic surface modification of poly(L-lactic acid) with chitosan and its effects on articular chondrocytes in vitroBiomaterials200324213859386812818559
- JuYMChoiJSAtalaAYooJJLeeSJBilayered scaffold for engineering cellularized blood vesselsBiomaterials201031154313432120188414
- ZongXHKimKFangDFRanSFHsiaoBSChuBStructure and process relationship of electrospun bioabsorbable nanofiber membranesPolymer2002431644034412
- DeitzelJMKleinmeyerJHarrisDBeck TanNCThe effect of processing variables on the morphology of electrospun nanofibers and textilesPolymer2001421261272
- StephanssonSNByersBAGarciaAJEnhanced expression of the osteoblastic phenotype on substrates that modulate fibronectin conformation and integrin receptor bindingBiomaterials200223122527253412033600
- LinYWangLZhangPSurface modification of poly(L-lactic acid) to improve its cytocompatibility via assembly of polyelectrolytes and gelatinActa Biomater20062215516416701873
- ShethMAnanda KumarRDaveVGrossRAMcCarthySPBiodegradable polymer blends of poly(lactic acid) and poly(ethylene glycol)J Appl Polym Sci199766814951505
- BadamiASKrekeMRThompsonMSRiffleJSGoldsteinASEffect of fiber diameter on spreading, proliferation, and differentiation of osteoblastic cells on electrospun poly(lactic acid) substratesBiomaterials200627459660616023716
- LiebETessmarJHackerMPoly(D,L-lactic acid)-poly(ethylene glycol)-monomethyl ether diblock copolymers control adhesion and osteoblastic differentiation of marrow stromal cellsTissue Eng200391718412625956
- KimSJKimMROhJSHanIShinSWEffects of polycaprolactone- tricalcium phosphate, recombinant human bone morphogenetic protein-2 and dog mesenchymal stem cells on bone formation: pilot study in dogsYonsei Med J200950682583120046425
- MurdochADGradyLMAblettMPKatopodiTMeadowsRSHardinghamTEChondrogenic differentiation of human bone marrow stem cells in transwell cultures: generation of scaffold-free cartilageStem Cells200725112786279617656642
- Institute of Laboratory Animal ResourcesGuide for the Care and Use of Laboratory AnimalsWashington, DCNational Academies Press1996
- KimHSLeeHJYeuISYiJSYangJHLeeIWThe neovascularization effect of bone marrow stromal cells in temporal muscle after encephalomyosynangiosis in chronic cerebral ischemic ratsJ Korean Neurosurg Soc200844424925519096686
- SinghviRStephanopoulosGWangDIEffects of substratum morphology on cell physiologyBiotechnol Bioeng199443876477118615800
- ShinTJParkSYKimHJLeeHJYoukJHDevelopment of 3-D poly(trimethylenecarbonate-co-epsilon-caprolactone)-block-poly (p-dioxanone) scaffold for bone regeneration with high porosity using a wet electrospinning methodBiotechnol Lett201032687788220213523
- TongHWWangMLiZYLuWWElectrospinning, characterization and in vitro biological evaluation of nanocomposite fibers containing carbonated hydroxyapatite nanoparticlesBiomed Mater20105505411120876957
- FujiharaKKotakiMRamakrishnaSGuided bone regeneration membrane made of polycaprolactone/calcium carbonate composite nano-fibersBiomaterials200526194139414715664641
- KultererBFriedlGJandrositzAGene expression profiling of human mesenchymal stem cells derived from bone marrow during expansion and osteoblast differentiationBMC Genomics200787017352823
- BruderSPJaiswalNHaynesworthSEGrowth kinetics, self-renewal, and the osteogenic potential of purified human mesenchymal stem cells during extensive subcultivation and following cryopreservationJ Cell Biochem19976422782949027588
- ChunCLimHJHongKYParkKHSongSCThe use of injectable, thermosensitive poly(organophosphazene)-RGD conjugates for the enhancement of mesenchymal stem cell osteogenic differentiationBiomaterials200930316295630819712969
- StigbrandTPresent status and future trends of human alkaline phosphatasesProg Clin Biol Res19841663146504935
- DattaNPhamQPSharmaUSikavitsasVIJansenJAMikosAGIn vitro generated extracellular matrix and fluid shear stress synergistically enhance 3D osteoblastic differentiationProc Natl Acad Sci U S A200610382488249316477044
- PrabhakaranMPVenugopalJRamakrishnaSElectrospun nanostructured scaffolds for bone tissue engineeringActa Biomater2009582884289319447211
- SeongJMKimBCParkJHKwonIKMantalarisAHwangYSStem cells in bone tissue engineeringBiomed Mater20105606200120924139