Abstract
Objective
To review the feasibility of coupling the techniques of random amplified polymorphic DNA (RAPD) with carbon nanotube-based modified electrode for guanine/deoxyguanine triphosphate (dGTP) electrochemical sensing for mapping of the pancreatic cancer genetic fingerprint and screening of genetic alterations.
Methods
We developed a new method to study the electrochemical behavior of dGTP utilizing carbon multiwalled nanotube (MWNT)-modified glassy carbon electrodes (GCEs). RAPD was applied for amplification of DNA samples from healthy controls and patients with pancreatic cancer under the same conditions to determine the different surplus quantity of dGTP in the polymerase chain reaction (PCR), thereby determining the difference/quantity of PCR products or template strands. Using this method we generated a genetic fingerprint map of pancreatic cancer through the combination of electrochemical sensors and gel electrophoresis to screen for genetic alterations. Cloning and sequencing were then performed to verify these gene alterations.
Results
dGTP showed favorable electrochemical behavior on the MWNTs/GCE. The results indicated that the electrical signal and dGTP had a satisfactory linear relationship with the dGTP concentration within the conventional PCR concentration range. The MWNTs/GCE could distinguish between different products of RAPD. This experiment successfully identified a new pancreatic cancer-associated mutant gene fragment, consisting of a cyclin-dependent kinase 4 gene 3′ terminal mutation.
Conclusion
The coupling of RAPD and nanoelectrochemical sensors was successfully applied to the screening of genetic alterations in pancreatic cancer and for mapping of DNA fingerprints.
Introduction
The high lethality rate of pancreatic cancer necessitates further improvement of therapeutic modalities, and it is clinically urgent to find new means to accurately detect early development of pancreatic cancer. Besides environmental exposure, genetic susceptibility to pancreatic cancer is also recognized as an important factor influencing the development of the disease in different individuals. As such, the identification of genetic differences related to the development of pancreatic cancer could lead to significant breakthroughs in early diagnosis and treatment.Citation1–Citation3
Electrochemical biosensing technology, which is characterized by high efficiency and sensitivity, easy and simple operation, and zero contamination, has been employed in molecular recognition and separation as well as in gene purification, and it is currently a cutting-edge research field in the life sciences.Citation4–Citation7 Carbon nanotubes demonstrate good electrical properties, nanosize effects, large specific surface areas, and favorable biocompatibility, and they are widely used in electrochemical biosensing technology.Citation8–Citation11 Carbon nanotube-modified glassy carbon electrodes (GCEs) can be used for simultaneous determination of deoxyguanine triphosphate (dGTP) and guanine, providing the possibility for direct detection of dGTP without separation of polymerase chain reaction (PCR) products. This study screened for gene mutations associated with pancreatic cancer by employing the techniques of arbitrarily primed PCR (random amplified polymorphic DNA [RAPD]) and electrochemistry to detect dGTP. It has been shown that RAPD, which employs random short-sequence primers, can be used to generate gene maps and is not limited to the detection of specific gene mutations or polymorphisms.Citation12 This study applied these new methods to achieve genetic fingerprinting of pancreatic cancer, overcoming the limitations of traditional RAPD and improving the screening efficiency to create a technology platform for screening of genetic susceptibility to complex diseases. These results have far-reaching scientific significance.
Material and methods
Instrument and reagents
The Model CHI 660C electrochemical analyzer (Shanghai Chenhua Instruments, Shanghai, People’s Republic of China) adopts a three-electrode system. The working electrode is a carbon multiwalled nanotube (MWNT)-modified GCE, the counter electrode is a platinum wire electrode, and the reference electrode is an Ag/AgCl electrode (saturated KCl). The following reagents were used in this study: MWNTs with a purity of >95%, an inside diameter of <10 nm, 5–15 μm length, ash content <0.2 wt%, and a unit surface area of 40–300 m2/g (Nano-Tech Port, Shenzhen, People’s Republic of China); dGTP (SBS Genetech, Beijing, People’s Republic of China); guanine (Oxoid, Hampshire, UK); and N,N-dimethyl formamide (DMF; Ruijinte Chemicals, Tianjin, People’s Republic of China). All reagents used were analytically pure, and all solutions were prepared with secondary quartz distilled water, with 10 × PCR buffer and MgCl2 (Promega, Madison, WI) used for PCR. Detection of dGTP in the proof sample and in the arbitrarily primed PCR reaction utilized the electrochemical work station described previously.
Carbon nanotube activating treatment
A total of 1 g of carbon nanotubes was placed in a concentrated sulfuric acid-nitric acid (volume ratio 3:1) mixture (100 mL) and heated and stirred at a constant temperature of 70°C for 12 hours. The solution was then filtered and washed until the pH value of the cleaning solution reached 7.0 and then subjected to vacuum drying at 60°C for 24 hours to obtain carboxylated carbon nanotubes.
Preparation of carbon nanotube-modified GCE
The GCE was successively polished in 0.30 μm and 0.05 μm Al2O3 and then subjected to ultrasonic cleaning for 3 minutes in 1:1 nitric acid, absolute ethyl alcohol, and redistilled water. It was then dried with nitrogen gas for later use. A quantity of carboxylated carbon nanotubes were placed into a spherical flask, sterilized deionized water was added, and ultrasonic dispersion was performed for 45 minutes to prepare a 1 mg/mL carbon nanotube dispersion. A total of 8 mL of dispersion was applied on to the surface of the GCE, and the electrode was fixed in a vacuum dryer. A GCE with a uniform layer of MWNTs was thus obtained.
Electrochemical behavior of dGTP on the MWNTs/GCE
Different concentrations of the dGTP proof sample were added into the PCR buffer. Enrichment was then performed for 2 minutes with constant stirring. The oxidation currents of the different concentrations of dGTP were measured using cyclic voltammetry and pulse differential indication methods. Before testing, nitrogen gas was supplied to the electrolytic cell for 5 minutes, so as to remove the dissolved oxygen. The standard curve was generated based on the electrical signals and dGTP concentrations.
RAPD amplification of DNA samples from patients and healthy controls
Primer design and RAPD amplification conditions are indicated in .
Table 1 List of random primersTable Footnotea
Arbitrarily primed PCR amplification
DNA templates of patient and healthy control groups were diluted to the same concentration, and amplification was perform based on the following conditions by using the random primers detailed in . The 50 μL reaction mixture contained 200 ng of genomic DNA, 10 mmol/L Tris HCl (pH 9.0), 50 mmol/L KCl, 0.1% Triton, 2 mmol/L MgCl2, 0.25 mmol/L dNTPs, 100 ng of sense primer, 100 ng of antisense primer, and 3.0 U Taq-DNA polymerase. Cycling conditions included an initial step at 94°C for 5 minutes; 45 cycles of 94°C for 1 minute, 36°C for 1 minute, and 72°C for 2 minutes; and a final elongation step at 72°C for 7 minutes. PCR products (10 μL) were separated using 1.5% agarose gel electrophoresis.
Cloning and sequencing
On the basis of the results obtained using the nanobiosensors, significant differences in surplus dGTP were determined. We then analyzed the remaining PCR products (pancreatic cancer and healthy control groups) by agarose gel electrophoresis. After separating and purifying the differential PCR fragments, the fragments were ligated into the pMD18-T plasmid (TaKaRa Bio, Shiga, Japan). The ligation reactions were transformed into Escherichia coli DH5 competent cells and incubated for 24 hours. The isolated plasmids were sequenced using an ABI PRISM7700 sequencer (Sangong Biotech, Shanghai, People’s Republic of China). The sequences were then compared with the human genomic sequence (NCBI, Bethesda, MD).
Results
Detection of guanine and dGTP
There was a significant difference between the oxidation peak potentials of dGTP and guanine, as determined using the MWNTs/GCE (see ). The oxidation peak of dGTP was about 1.0 V, and that of guanine was between 0.65 V and 0.70 V, which was consistent with previously published observations.Citation5 This indicated that the MWNTs/GCE could effectively distinguish between the oxidation peak potentials of dGTP and guanine, making it possible to selectively detect the composition within the PCR system without separation of the reaction products.
Different concentrations of dGTP demonstrated a favorable linear relationship
As is shown in , the oxidation peak current of dGTP increased with the increasing concentration. Within the range of 5–50 μmol/L, the oxidation peak current of dGTP showed a perfect linear relation with concentration, with a regression of Ipa = 0.0204C–0.086, r = 0.9966 (unit of Ipa is μA and that of C is μmol/L). This experiment showed that the MWNTs/ GCE could effectively detect changes in dGTP concentration in the PCR system and could be used to screen for genetic alterations amplified using random primers.
Figure 2 DPV diagram (A) of deoxyguanine triphosphate of different concentrations on multiwalled nanotube-modified glassy carbon electrode and the working curve (B) reflecting the relationship between oxidation peak current and concentration change.
Abbreviations: dGTP, deoxyguanine triphosphate; DPV, differential pulse voltammetric.
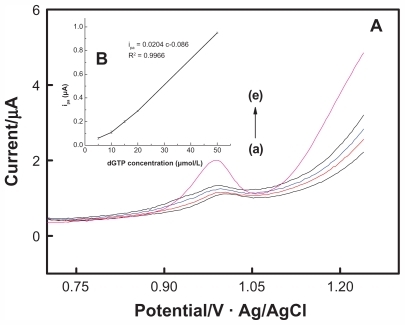
Analysis of gene mutations
As is shown in , compared with the healthy control, the concentration of dGTP in the peripheral blood DNA of patients with pancreatic cancer changed remarkably after 40 cycles of amplification, and the peak current difference was 0.33 μA. It could be estimated based on the linear equation obtained from that the dGTP concentration consumed in the reaction for the patients with pancreatic cancer was 23.8 μmol/L higher than the healthy control (theoretically, when consuming 20 μmol/L of dGTP, a 50 μL reaction would be able to synthesize 250 ng or 1.25 pmol of an 800 bp sequence).
Figure 3 Diagrams of differential oxidation current between pancreatic cancer patient and normal control (A) and the gel electrophoresis of polymerase chain reaction product (B) on multiwalled nanotube-modified glassy carbon electrode.
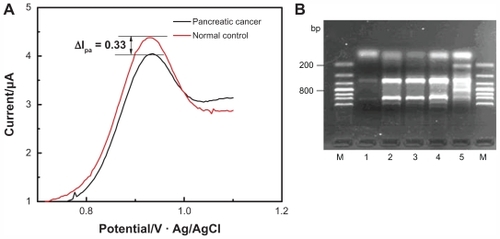
It can be observed from the gel electrophoresis analysis that two more fragments with a size of about 200 bp and 800 bp were amplified from the pancreatic cancer sample than the healthy control, as shown in . The results showed that the electrochemical sensor could be used to detect changes in dGTP quantity and thus could be used to screen for gene alterations associated with pancreatic cancer.
Purification of sequencing results
The differentially amplified bands (between the pancreatic cancer and healthy control reactions; 200 bp and 800 bp) were purified, cloned, and sequenced (). The underlined sequence in is consistent with amplification with random primer No. 2 (AACGGTCACT), and the associated sequence is a fragment of plasmid. This sequence was identical to that of the 3’ end of a cyclin-dependent kinase gene of Homo sapiens located on chromosome 1.
Genetic fingerprinting
Using random primer combinations (the primer added to the PCR system is from #1 to #5), amplification was performed using peripheral blood DNA samples from healthy controls (gel electrophoresis lane A) or patients with pancreatic cancer (gel electrophoresis lanes B → G) as DNA templates under the same PCR conditions. The results showed that the resulting PCR products were significantly different, based on either agarose gel electrophoresis or electrochemical sensing technology for detection of the PCR-based substrate (dGTP) ().
Figure 5 DPV curve generated from different pancreatic cancer samples amplified via arbitrarily primed polymerase chain reaction and detected using a multiwalled nanotube-modified glassy carbon electrode.
Abbreviation: DPV, differential pulse voltammetric
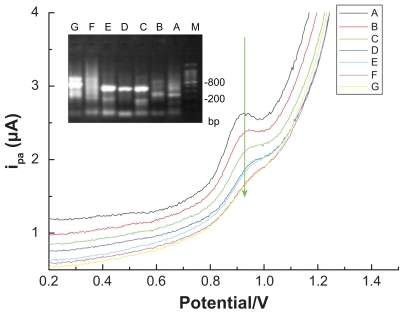
As can be observed in , the different pancreatic cancer DNA templates that were amplified via arbitrarily primed PCR presented different banding patterns following gel electrophoresis, indicating a difference between individuals with pancreatic cancer. Furthermore, these differences could also be identified using the electrochemical sensor, which presented significantly different concentration curves.
Discussion
As the generation III human genetic mark, single nucleotide polymorphisms (SNPs) reflect the difference between individual phenotypes, disease susceptibility, and the response to drugs and the environment, for example. As such, SNPs are an ideal means for the study of malignant tumors and other complex diseases.Citation13,Citation14 Thanks to the optimization of gene sequencing technology and the close cooperation between international research groups in recent years, case-control studies detailing the relationship between SNPs and cancer risk have been increasing rapidly.Citation15 However, these types of studies are still limited by the current methodology. Compared with the human gene database and the complex varieties of human diseases, the number of SNPs/mutations clearly associated with human disease is limited.Citation16
The development of interdisciplinary approaches promotes progress in gene testing technologies. In this study we developed a technical platform that combined arbitrarily primed PCR and nanoelectrochemical sensing for screening of pancreatic cancer susceptibility genes. There are many points to consider in respect of the use of RAPD in combination with electrochemical sensor analysis that have significantly improved the efficiency of screening for genetic alterations. In terms of PCR product identification, the traditional practice has been to interpret differential banding patterns following agarose gel electrophoresis. However, this method itself has many flaws, such as poor sensitivity and specificity. Furthermore, the more significant limitation lies in the fact that arbitrarily primed PCR utilizes short-sequence primers, and thus the products under different conditions would be significantly different. As such, it would be impossible to handle such a huge project using traditional technology.Citation17 By using nanoelectrochemical sensing technology to detect arbitrarily primed PCR products or substrates, we screened for differentially amplified genes between patients with pancreatic cancer and controls and identified a cyclin-dependent kinase gene. This gene is involved in cell-cycle regulation and progression through the cell cycle. Mutations in this gene could lead to arrest in the G1 phase of the cell cycle and thus possibly influence the development of pancreatic cancer.Citation18,Citation19
With regard to this approach, another point to consider is that arbitrarily primed PCR technology is a new molecular marking technology based on traditional PCR, and the amplified fragment polymorphism reflects polymorphisms in the DNA.Citation20–Citation24 Any specific primer has its unique binding site in the DNA template sequence. Any insertion, deletion, or base mutation in the primer binding site within the DNA template may result in a change in the distribution of these specific binding sites and thus bring about a change in the quantity/ size of the amplification product. Specific primer sets can only test for DNA polymorphisms in specific areas of the genome; however, a series of primers can be used to expand the detection area to encompass the whole genome. Hence, RAPD could be used for polymorphism detection of the entire genome and for construction of genomic fingerprints.Citation25,Citation26 The experimental design depicted in shows the backward estimation of template-strand conditions based on the detection of reaction substrate consumption using nanoelectrochemical sensing technology.
In case of a base change at site 2, the sequence no longer matches with the random primers, and the reaction does not produce product B. Another point to consider is that this detection technology is based on determining the concentration of dGTP without introducing any foreign substance to the PCR system.Citation20,Citation21 In this study, GCE surface-modified carbon nanomaterial was used for the study of the electrochemical behavior of guanine and dGTP at normal PCR concentrations, and it provided a theoretical basis for the development of new DNA detecting methods and design of guanine oxidation-based biosensors. This method could have profound significance and application value to studies involving clinical medicine and genetic engineering.Citation22,Citation23
The development of pancreatic cancer is the result of environmental and genetic factors, and the poor understanding of the relationship between gene abnormalities and pancreatic cancer greatly restricts the application of molecular diagnoses for the early screening of high risk groups. Nanoelectrochemical sensors have unique oxidation electrical behaviors in terms of detection of PCR reaction substrates (dGTP), and the use of random primers generates different amplification products under different PCR conditions. In this study we estimated the difference in amplification product from the template by detecting substrate consumption after the amplification of DNA templates from the patients with pancreatic cancer and healthy controls under the same conditions. The results showed that the new design was effective at distinguishing genetic alterations. Although some defects are observed in this principle, this paper proposes a new method of studying the differential gene of complex diseases, and provides a valid example of applying carbon nanotubes to clinical gene study.
Acknowledgments
Financially supported by the Project Foundation of Fujian Provincial Education (JA10143), National High Technology Investigation Project Foundation of China (2008AA02Z433), National Natural Science Foundation of China (20975021, 20805006), and Major Program Foundation of Fujian Medical University (09ZD013).
Disclosure
The authors report no conflicts of interest in this work.
References
- OkazakiTJiaoLChangPSingle-nucleotide polymorphisms of DNA damage response genes are associated with overall survival in patients with pancreatic CancerClin Cancer Res2008142042204818381943
- PierceBLAhsanHGenome-wide “pleiotropy scan” identifies HNF1A region as a novel pancreatic cancer susceptibility locusCancer Res2011714352435821498636
- PetersenGMAmundadottirLFuchsCSA genome-wide association study identifies pancreatic cancer susceptibility loci on chromosomes 13q22.1, 1q32.1 and 5p15.33Nat Genet20104222422820101243
- EkramiASamarbaf-ZadehARKhosraviAValidity of bio-conjugated silica nanoparticles in comparison with direct smear, culture, and polymerase chain reaction for detection of Mycobacterium tuberculosis in sputum specimensInt J Nanomedicine201162729273522114503
- YangTZhangWDuMJiaoKA PDDA/poly(2,6-pyridinedicarboxylic acid)-CNTs composite film DNA electrochemical sensor and its application for the detection of specific sequences related to PAT gene and NOS geneTalanta20087598799418585173
- RasoolyAJacobsonJDevelopment of biosensors for cancer clinical testingBiosens Bioelectron2006211851185816458498
- LiuRHYangJLenigkRSelf-contained, fully integrated biochip for sample preparation, polymerase chain reaction amplification, and DNA microarray detectionAnal Chem2004761824183115053639
- ChengJGuYJWangYJNanotherapeutics in angiogenesis: synthesis and in vivo assessment of drug efficacy and biocompatibility in zebrafish embryosInt J Nanomedicine201162007202121976976
- LiJQiuJDXuJJSynergistic effect of Prussian blue grafted carbon nanotubes/poly(4-vinyl-pyridine) composites for sensitive amperometric sensingAdv Funct Mater20071715741580
- LinLQLiuQCWangLMEnzyme-amplified electrochemical biosensor for detection of PML–RARα fusion gene based on hairpin LNA probeBiosens Bioelectron20112827728321840703
- NairKLJagadeeshanSNairSAKumarGSVBiological evaluation of 5-fluorouracil nanoparticles for cancer chemotherapy and its dependence on the carrier, PLGAInt J Nanomedicine201161685169721980233
- LaoKXuNLStrausNAWhole genome amplification using single-primer PCRBiotechnol J2008337838218293327
- LoebLAMonnatRJJrDNA polymerases and human diseaseNat Rev Genet2008959460418626473
- LoebLAHuman cancers express mutator phenotypes: origin, consequences and targetingNat Rev Cancer20111145045721593786
- WrightFAStrugLJDoshiVKGenome-wide association and linkage identify modifier loci of lung disease severity in cystic fibrosis at 11p13 and 20q13.2Nat Genet20114353954621602797
- LangeSSTakataKWoodRDDNA polymerases and cancerNature Rev Cancer2011119611021258395
- FengKZhaoJWuZSHigh-sensitive electrochemical detection of point mutation based on polymerization-induced enzymatic amplificationBiosens Bioelectron2011263187319121239161
- VenterJCAdamsMDMyersEWThe sequence of the human genomeScience20012911304135111181995
- GaoFLiuQCWangMNovel mutation of the cyclin-dependent kinase 4 gene in a Chinese patient with intimal sarcoma of the pulmonary arteryChin Med J20091221107110919493449
- Oliveira-BrettAMVivanMFerandesIRElectrochemical detection of in situ adriamycin oxidative damage to DNATalanta20025695997018968575
- PedanoMLRivasGAAdsorption and electrooxidation of nucleic acidsm at carbon nanotubes paste electrodeElectrochem Commun200461016
- DeféverTDruetMRochelet-DequaireMReal-time electrochemical monitoring of the polymerase chain reaction by mediated redox catalysisJ Am Chem Soc2009131114331144119722651
- LiQBatchelor-McAuleyCComptonRGElectrochemical oxidation of guanine: electrode reaction mechanism and tailoring carbon electrode surfaces to switch between adsorptive and diffusional responsesJ Phys Chem B20101147423742820446746
- DaveyJWHohenlohePAEtterPDGenome-wide genetic marker discovery and genotyping using next-generation sequencingNat Rev Genet20111249951021681211
- AtienzarFAVenierPJhaANDepledgeMHEvaluation of the random amplified polymorphic DNA (RAPD) assay for the detection of DNA damage and mutationsMutat Res200252115116312438012
- SinghKPRoyDIdentification of novel breast tumor-specific mutation(s) in the q11.2 region of chromosome 17 by RAPD/AP-PCR fingerprintingGene2001269334311376935