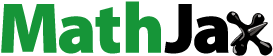
Abstract
Background
The purpose of this study was to devise a novel electrochemical immunosensor for ultrasensitive detection of alfa-fetoprotein based on Fe3O4/Au nanoparticles as a carrier using a multienzyme amplification strategy.
Methods and results
Greatly enhanced sensitivity was achieved using bioconjugates containing horseradish peroxidase (HRP) and a secondary antibody (Ab2) linked to Fe3O4/Au nanoparticles (Fe3O4/Au-HRP-Ab2) at a high HRP/Ab2 ratio. After a sandwich immunoreaction, the Fe3O4/Au-HRP-Ab2 captured on the electrode surface produced an amplified electrocatalytic response by reduction of enzymatically oxidized hydroquinone in the presence of hydrogen peroxide. The high content of HRP in the Fe3O4/Au-HRP-Ab2 could greatly amplify the electrochemical signal. Under optimal conditions, the reduction current increased with increasing alfa-fetoprotein concentration in the sample, and exhibited a dynamic range of 0.005–10 ng/mL with a detection limit of 3 pg/mL.
Conclusion
The amplified immunoassay developed in this work shows good precision, acceptable stability, and reproducibility, and can be used for detection of alfa-fetoprotein in real samples, so provides a potential alternative tool for detection of protein in the laboratory. Furthermore, this immunosensor could be regenerated by simply using an external magnetic field.
Introduction
It is well known that alfa-fetoprotein (AFP), an oncofetal glycoprotein with a molecular weight of approximately 70,000 Da, is a tumor marker that occurs mainly in hepatocellular carcinoma, yolk sac tumors, and the serum of patients with other malignant tumors.Citation1–Citation3 The average concentration of AFP in healthy human serum is <20 ng/mL, and serum AFP levels often increase in a number of disease states.Citation4 Therefore, detection of trace amounts of AFP is of great importance. Thus far, various immunosensors and immunoassays based on different measurement principles have been reported for determination of AFP, including enzyme-linked immunosorbent assay (ELISA),Citation5 electrochemiluninescence,Citation6,Citation7 chemiluminescence,Citation8,Citation9 surface plasmon resonanceCitation10,Citation11 and quartz crystal microbalance.Citation12,Citation13 ELISA is the most widely used immunoassay method in the laboratory. However, concentrations of tumor-related proteins are very low in the early stages of cancer, and are beyond the detection limit of ELISA. Moreover, their lengthy analysis requires highly skilled personnel, specially equipped laboratories, and expensive reagents.Citation14 Thus, new methods that can rapidly and conveniently monitor tumor-related proteins are highly desirable. Electrochemical immunosensors, based on specificity of antigen–antibody interactions with electrochemical transducers, have attracted considerable interest because of their intrinsic advantages, such as low cost, high sensitivity, simple instrumentation, and excellent compatibility with miniaturization technologies.Citation15 Therefore, different electrochemical immunosensors, particularly amperometric immunosensors, have been developed and applied extensively for the determination of AFP.Citation16–Citation18
In order to meet the increasing demand for early and ultrasensitive detection of tumor markers, three primary signal amplification strategies using nanomaterials have been developed.Citation19 The first method involves the use of metal and semiconductor nanoparticles directly as electroactive labels to amplify the electrochemical detection of proteins.Citation20,Citation21 The second method uses nanoparticles as carriers for loading a large amount of electroactive species to amplify the detection signal.Citation22,Citation23 The third method is the most extensively employed, and uses enzyme-functionalized nanoparticles as labels. Enhanced sensitivity was achieved by loading a large amount of enzyme towards an individual sandwich immunological reaction event. Recently, various types of nanomaterials have been used as carriers for loading enzymes and antibodies to enhance sensitivity, including gold nanoparticles,Citation24 irregularly shaped gold nanoparticles,Citation25 nanosilica particles,Citation26 carbon nanoparticles,Citation27,Citation28 carbon nanotubes,Citation29 and graphene oxide.Citation30 For example, Chen et al have proposed a highly sensitive electrochemical immunosensor using irregular gold nanoparticles as carriers of horseradish peroxidase (HRP)-anti-AFP for signal amplification.Citation25 Similarly, Lin et al have reported use of graphene oxide, a novel tracer, to label HRP and the antibody, and developed an ultrasensitive immunoassay method for detection of phosphorylated p53 (S392).Citation30
Recently, hybrid nanomaterials consisting of two or more different nanoscale functionalities have attracted much attention due to their novel combined properties and multiple potential applications.Citation31 Immunomagnetic beads in particular are widely used in enrichment and separation of particular proteins in biology samples.Citation32–Citation34 Among them, magnetic composite Fe3O4/Au nanoparticles have attracted particular attention, owing to the combined functions of Fe3O4 and gold. Fe3O4 nanoparticles have a typical super-paramagnetic nature, and provide a convenient means for separation, isolation, and purification of biological samples via an external magnetic field when the functional reagents are attached onto the surface of the particles.Citation35,Citation36 Their unique structural, electronic, and optical properties make gold nanoparticles very attractive for several applications in biotechnology.Citation37–Citation39 Further, gold nanoparticles can provide a natural environment for bimolecular immobilization and facilitate electron transfer of biosensors because of their large surface area, interesting electrochemical properties, and good biocompatibility.Citation40–Citation42 Therefore, magnetic composite Fe3O4/Au nanoparticles have had more and more applications in areas such as immunoassays, protein immobilization, cell purification, and magnetically controlled transport of anticancer drugs.Citation43–Citation46
Our present work focuses on enzyme-enriched, magnetic functionalized nanoparticles in signal amplification and separation for ultrasensitive detection of AFP. Magnetic composite Fe3O4/Au nanoparticles were synthesized by assembling gold nanoparticles on SH-modified Fe3O4 and using them as nanocarriers for immobilization of HRP and a secondary antibody (Ab2). Greatly amplified sensitivity is achieved using bioconjugates featuring HRP and Ab2 linked to Fe3O4/Au at a high HRP/Ab2 ratio for replacement of singly labeled secondary antibodies. A modified glass carbon electrode with porous nanostructured gold film was used to attach the primary antibody (Ab1). The analytical procedure consists of immunoreaction of the antigen (AFP) with Ab1, followed by binding of Fe3O4/Au-HRP-Ab2. Electrochemical detection was performed in the presence of H2O2 and hydroquinone (). The results demonstrated that an immunosensor based on this amplification strategy has a good dynamic range from 0.005 to 10 ng/mL and a low detection limit of 3 pg/mL for AFP. This simple, cost-effective, and sensitive immunosensor could find wide potential application in clinical analysis.
Materials and methods
Reagents and materials
Monoclonal mouse antihuman AFP and AFP test kits were obtained from Zhengzhou Biocell Biochem Company (Zhengzhou, China). Fe3O4 (20 nm in diameter), 3-mercaptopropyltriethoxysilane (3-MPTES) and HRP (EC 1.11.1.7, RZ > 3.0, A > 250 U/mg) were purchased from Sigma-Aldrich, St Louis, MO. Hydrogen tetrachloroaurate (III) tetrahydrate (HAuCl4·4H2O), trisodium citrate, hydroquinone, H2O2, and bovine serum albumin were obtained from Sinopharm Group Chemical Reagent Company Ltd (Shanghai, China). All reagents used were of analytical reagent grade, and all solutions were prepared with double deionized water. Phosphate-buffered solutions 0.1 M of various pH values were prepared by mixing stock solutions of NaH2PO4 and Na2HPO4, and then the pH was adjusted with 0.1 M NaOH and H3PO4.
Apparatus
Cyclic voltammetry measurements were performed using an electrochemical analyzer CHI 660 (Shanghai Chenhua Instrumental Corp, China) connected to a personal computer. A three-compartment electrochemical cell contained a platinum wire auxiliary electrode, a saturated calomel reference electrode, and a modified glass carbon electrode (GCE, 2 mm in diameter) as working electrode. The sizes of the nanoparticles were estimated from transmission electron microscopy (H-7650, Hitachi Instruments, Tokyo, Japan). The surface topographic features and composition of variously modified electrodes were characterized using scanning electron microscopy coupled with energy dispersive spectroscopy (SEM-EDS, S3400N Hitachi). The x-ray powder diffraction images were obtained using a Bruker D8 Advance diffractometer (Bruker, Karlsruhe, Germany). Ultraviolet-visible spectra were recorded using a TU-1901 spectrophotometer (Purkinje General Instrument Co, Beijing, China). The x-ray photoelectron spectra were taken using an AXIS UltraDLD spectrometer (Shimadzu Co, Tokyo, Japan). Vibrating sample magnetometry (Lake Shore 7410) was used to perform magnetic measurements on the dried samples to evaluate the magnetic properties of the nanoparticles.
Preparation of Fe3O4/Au nanoparticles
shows the procedure used to prepare the Fe3O4/Au. First, gold nanoparticles was prepared using the Frens method. Citation47 In brief, 0.01 wt% HAuCl4 (100 mL) was boiled with vigorous stirring in a flask. Trisodium citrate 1 wt% solution (2.5 mL) was added quickly to the boiling solution, resulting in a color change from pale yellow to dark red, indicating formation of gold nanoparticles. The solution was maintained for 10 minutes at boiling temperature.
Fe3O4/Au nanoparticles were obtained by self-assembling gold nanoparticles onto the surface of SH-modified Fe3O4 according to the literature with modification.Citation48 Fe3O4 nanoparticles are commercially available. Experimentally, Fe3O4 0.2 g was dispersed in 50% ethanol 90 mL by ultrasound, and then 3-MPTES 300 μL and ammonium hydroxide 500 μL were added dropwise. The mixture was stirred at 60°C for 6 hours under a nitrogen atmosphere. Uncoated Fe3O4 was deleted by means of washing with 0.1 M HCl (six times, for four hours each). The deposit was separated by a magnetic stirrer, which was rinsed three times with double deionized water and then ethanol to give SH-modified Fe3O4. Following that, the gold nanoparticles were added to the SH-modified Fe3O4, and shaken slightly for 4 hours at room temperature to make the gold assemble on the surface of the formed nanoparticles. After magnetic separation, the Fe3O4/Au obtained were rinsed with double deionized water and suspended in 10 mL water.
Preparation of Fe3O4/Au-HRP-Ab2 conjugates
shows the procedure used to prepare the Fe3O4/Au-HRP-Ab2 bioconjugate. Initially, 1.0 mL of a 1.0 mg/mL Fe3O4/Au nanoparticle suspension was adjusted to pH 8.2 using Na2CO3. Thereafter, 200 μL of HRP at 1 mg/mL and 200 μL of Ab2 at 10 μg/mL were added to the dispersion, and the mixture was stirred overnight at 4°C. After magnetic separation and washing three times, the resulting mixture was redispersed in 1.0 mL of pH 7.4 phosphate-buffered solution containing 1% bovine serum albumin and stored at 4°C.
Fabrication of the immunosensor
The procedure followed to prepare the proposed immunosensor is schematized in . The GCE was polished with 0.3 μm and 0.05 μm alumina, followed by successive sonication in distilled water and ethanol for 5 minutes and dried in air. A porous nanostructure gold film was then deposited at a voltage of −0.5 V for 50 seconds on the clean GCE that was immersed in 5 mL 0.08 M HAuCl4 solution containing 0.004 M lead acetate. After thorough washing with water, the electrode was immediately followed by incubation with 20 μL 0.5 mg/mL Ab1 for one hour. After washing with 0.05% Tween-20 and phosphate-buffered solution, Ab1/Au/GCE was incubated in 3% bovine serum albumin and phosphate- buffered solution at 37°C for one hour to block excess active groups and nonspecific binding sites on the surface. The electrode was then washed with 0.05% Tween-20 and phosphate-buffered solution before use.
Immunoassay procedure for detection of AFP
A sandwich immunoassay was used for determination of AFP. The immunosensor, Ab1/Au/GCE, was incubated with 20 μL of a different concentration of standard AFP antigen for 30 minutes at 25°C, followed by washing with 0.05% Tween-20 and phosphate-buffered solution. Next, the electrode was incubated with 20 μL of Fe3O4/Au-HRP-Ab2 for 30 minutes at 25°C, followed by washing with 0.05% Tween-20 and phosphate-buffered solution to remove the nonspecific adsorption of conjugate. Electrochemical detection was performed in 5 mL of pH 6.5 phosphate-buffered solution containing 2 mM hydroquinone and 5 mM H2O2, and from −0.6 to 0.6 V (versus saturated calomel reference electrode) with a scan rate of 100 mV/second.
Results and discussion
Characterization of Fe3O4/Au-HRP-Ab2 conjugation
In this work, Fe3O4/Au was as an excellent carrier for HRP and Ab2 because of its good biocompatibility, large surface area, and superparamagnetic properties. Transmission electron microscope images provide more detailed morphological information on the prepared nanoparticles (). They demonstrated that both Fe3O4 and Fe3O4/Au nanoparticles were spherical in structure, monodispersed, and of appropriate size. The average diameter of the Fe3O4/Au nanoparticles was about 40 nm (). Upon deposition of gold onto the Fe3O4 nanoparticles, the diameter of the particles increased by about 20 nm, demonstrating that the thickness of the gold was about 10 nm.
Figure 2 Transmission electron microscopic images of (A) Fe3O4 and (B) Fe3O4/Au. Diameter distribution of (C) Fe3O4/Au nanoparticles.

compares the x-ray powder diffraction pattern for the Fe3O4 nanoparticles and Fe3O4/Au nanoparticles. In (curve a), the diffraction peak positions at 2θ = 30.0°, 35.3°, 42.6°, 53.4°, and 62.5° can be attributed to the (220), (311), (400), (422), (511), and (440) planes of Fe3O4 in a cubic phase (space group Fd3 m), respectively. In (curve b), the diffraction peak positions at 2θ = 38.1°, 44.2°, 64.5°, 77.5°, and 81.6° can be attributed to the (111), (200), (220), (311), and (222) planes, respectively. Other peaks can be attributed to Fe3O4, and the simultaneous observation of both Fe3O4 and gold peaks verified successful assembly of Fe3O4/Au nanoparticles.
Figure 3 (A) X-ray powder diffraction patterns for (a) Fe3O4 nanoparticles and (b) Fe3O4/Au nanoparticles. (B) X-ray photoelectron spectra binding-energy spectra for a sample of Fe3O4/Au.
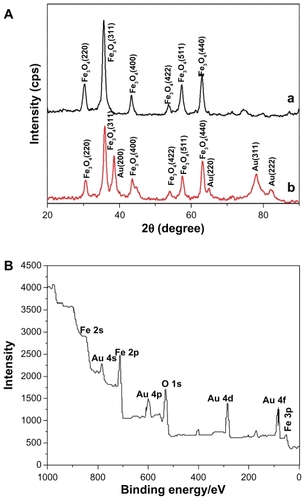
The surface composition of elements such as iron and gold in the Fe3O4/Au nanoparticles were determined by x-ray photoelectron spectra as shown in . shows gold peaks including Au 4s, Au 4p, Au 4d, and Au 4f appearing on the surface of the Fe3O4 nanoparticles. The Au 4f appearing at 80–90 eV can be assigned to the Au0. The Fe3O4 showed Fe 2p at 700–720 eV. The x-ray photoelectron spectra result was in agreement with a report by Cui et al indicating that gold had been successfully assembled on the surface of the magnetic Fe3O4 nanoparticles.Citation48
The magnetic properties of the nanoparticles are illustrated in . It can be seen that a small coercivity or remanence existed around room temperature, indicating the Fe3O4 and Fe3O4/Au have superparamagnetic properties. As a result, the Fe3O4/Au could be isolated from solution by an adscititious magnet. It can also be seen that the saturation magnetization for Fe3O4/Au is much smaller than that for Fe3O4. The saturation magnetization values were 76.8 emu/g and 54.9 emu/g for Fe3O4 and Fe3O4/Au nanoparticles, respectively. The saturation magnetization value of the magnetic nanoparticles declined after coating with a layer of gold. This lower saturation magnetization value is due to the presence of nonmagnetic gold.
Figure 4 Magnetization hysteresis for (a) Fe3O4 and (b) Fe3O4/Au nanoparticles recorded at room temperature.
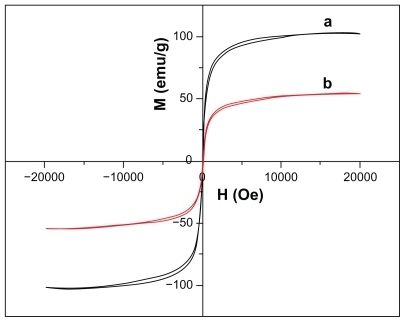
The Fe3O4/Au-HRP-Ab2 obtained was characterized by ultraviolet-visible spectroscopy and, as shown in , the Fe3O4 nanoparticles did not show any obvious absorbance peaks in ultraviolet-visible spectroscopy (curve a). However, the Fe3O4/Au nanoparticles showed a clear absorption band containing a maximum centered at 540 nm (curve c). This band showed a red shift in comparison with pure gold nanoparticles (curve b, 520 nm). This finding suggests that a combination of Fe3O4 nanoparticles and gold nanocomposites leads to a red shift of resonant wavelength. After Ab2 and HRP were conjugated onto the Fe3O4/Au, two absorption peaks around 280 nm and 404 nm were observed (curve f), which can be ascribed to the typical absorption of Ab2 (curve d) and HRP (curve e).
SEM-EDS characterization of gold nanoparticles modified electrode with direct electroposition
SEM-EDS were used to investigate the successful fabrication of the immunosensor. shows the energy dispersive spectroscopy analysis of Au/GCE showing Au-M (2.0 keV), Au-Lα (9.7 keV), and Au-Lβ (11.4 keV), which indicates successful electrodeposition of gold on the GCE. Furthermore, scanning electron microscopy was used to investigate the Au/GCE before and after modification with Ab1. As shown in , many small porous gold nanoparticles were obtained on the Au/GCE. Obviously, the porous gold film-modified electrode had a larger surface compared with the ordinary glassy carbon electrode, which could provide a larger surface area for the immobilization of Ab1. Upon immobilization of Ab1, obvious aggregation of the trapped biomolecules could be observed on the surface (), indicating successful immobilization of Ab1.
Electrochemical characterization of the immunosensor
As shown in , the cyclic voltammograms of Ab1/Au/GCE did not show any detectable signal in pH 6.5 phosphate-buffered solution (curve a). Upon adding 2 mM hydroquinone and 5 mM H2O2 to the phosphate-buffered solution, Ab1/Au/GCE exhibited a pair of stable and well defined redox peaks at −145 mV and 345 mV (curve b), corresponding to the electrochemical redox response of hydroquinone on the electrode surface. When incubating the immunosensor with 5 ng/mL AFP antigen, no obvious change in signal was observed. However, after incubation with Fe3O4/Au-HRP-Ab2, the resulting sandwich immunocomplex displayed an obvious increase in electrocatalytic reduction current (curve c) because of the introduction of HRP onto the electrode surface by the immunoreaction.
Figure 7 Cyclic voltammograms obtained at (a) Ab1/Au/GCE in pH 6.5 phosphate-buffered solution, (b) Ab1/Au/GCE, (c) Fe3O4/Au-HRP-Ab2/AFP/Ab1/Au/GCE, in pH 6.5 phosphate-buffered solution containing 2 mM hydroquinone and 5 mM H2O2.
Abbreviations: HRP, horseradish peroxidase; GCE, glass carbon electrode.

Comparison of detection antibodies with various labels
To investigate further the effect of the synthesized Fe3O4/Au on the sensitivity of the electrochemical immunosensors, three types of detection antibodies were used, ie, HRP-anti-Ab2, Au-HRP-Ab2, and Fe3O4/Au-HRP-Ab2 (). As shown in , upon addition of H2O2 to the substrate solution, an obvious catalytic characteristic appeared, with a distinct increase in the reduction current and a decrease in the oxidation current. The catalytic current is mainly due to the labeled HRP toward the reduction of H2O2 with the help of hydroquinone as an electron mediator. As shown in , we found that the electrochemical immunosensor exhibited a larger current shift using Au-HRP-Ab2 () as the detection antibody than when using HRP-anti-Ab2 (). The reason for this might be that the gold nanoparticles with a high surface-to-volume ratio and good biocompatibility can load more HRP and Ab2. Moreover, gold nanoparticles have good conductivity, which can enhance enzyme activity.Citation49 More significantly, the electrochemical responses could be improved by using Fe3O4/Au-HRP-Ab2 () rather than Au-HRP-Ab2 (). A possible reason is that Fe3O4 can allow more room for attachment of gold nanoparticles, which indirectly increases the amount of bound Ab2 and HRP. When one antibody molecule on the surface of Fe3O4/Au-HRP-Ab2 is reacted with the corresponding antigen, other HRP molecules can enter and participate in the electrochemical reaction. At the same time, Fe3O4/Au can enhance the electron transfer rate between the enzyme and the electrode.Citation50,Citation51
Figure 8 Cyclic voltammograms of the electrochemical sandwich immunosensors toward 5 ng/mL alfa-fetoprotein in the absence (A) and presence (B) of 5 mM H2O2 in an electrolytic cell in phosphate-buffered solution containing 2 mM hydroquinone by using various detection antibodies (a) HRP-anti-Ab2, (b) Au-HRP-Ab2, and (C) Fe3O4/Au-HRP-Ab2.
Abbreviations: HRP, horseradish peroxidase; Ab2, secondary antibody.
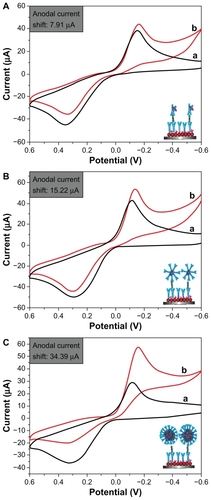
Optimization of immunoassay conditions
Because of the coimmobilization of enzymes and antibodies on the Fe3O4/Au nanocarrier, the ratio of HRP and Ab2 is the most important factor in the response signal. As shown in , one can see that the electrocatalytic current increases with an increasing ratio of HRP/Ab2, and the maximum response is achieved at a ratio of 100:1. An increase in the HRP/Ab2 ratio could increase the total amount of HRP loaded per Fe3O4/Au nanocarrier, which would be expected to increase the response amplification for this sandwich immunoassay. However, reducing the amount of Ab2 in the immunoassay may decrease the efficiency of the immune reaction to the AFP antigen captured at the electrode surface, which may result in a decreased response. Therefore, an HRP/Ab2 ratio of 100:1 was selected as the optimal condition in which to prepare the Fe3O4/Au-HRP-Ab2 conjugate. To determine the amount of active HRP in the Fe3O4/Au-HRPAb 2 conjugate dispersion, the mixture was reacted with the TMB substrate for HRP. The reaction product was read at 650 nm. These results were compared with a standard curve constructed using pure HRP as part of an enzyme activity experiment. The concentration of active HRP in the Fe3O4/Au-HRP-Ab2 conjugate dispersion was determined to be 7.14 μg/mL.
Figure 9 Effects of (A) HRP/Ab2 ratio, (B) pH of phosphate-buffered solution, and (C) incubation time on electrochemical responses. A 1 ng/mL AFP concentration was used during the incubation process.
Abbreviations: Ab2, secondary antibody; AFP, alfa-fetoprotein; HRP, horseradish peroxidase.
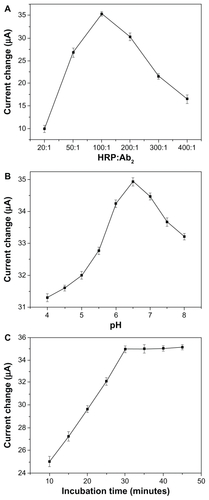
The pH of the detection solution is an important parameter because the acidity of the solution is greatly affected by the activity of immobilized proteins. The effect of pH of the solution on the amperometric response for AFP is shown in . The current response was increased by increments in pH from 4.0 to 6.5 and then decreased when pH exceeded 6.5. Therefore, a 0.1 M solution of pH 6.5 was selected as the optimum solution for AFP detection.
The temperature and time taken for the antigen-antibody interaction to occur greatly influenced the sensitivity of the sandwich-type immunoassay developed. Considering the practical applications of the proposed system in clinical immunoassays, all experiments were carried out at room temperature (25°C ± 1.0°C). Under this condition, the effect of various incubation times (10–45 minutes) on the immunosensor current was investigated at 1 mg/mL of AFP. As shown in , the reduction current increased with increasing incubation time and tended to level off after 30 minutes, indicating optimal formation of the sandwich-type immunocomplexes. A longer incubation time could not improve the response. Therefore, 30 minutes of incubation time was used for the detection of AFP in this study.
Analytical performance
The cyclic voltammograms of the immunosensor was investigated by assaying various AFP standards using Fe3O4/Au-HRP-Ab2 as trace labels and H2O2 as the enzyme substrate. The reduction current increased with incremental AFP concentration in the incubation solution. As shown by curve a in , the increase in reduction current was proportional to AFP concentration in the range of 0.005–10 ng/mL; the linear regression equation is Δip(μA) = 13.3 × logCAFP (ng/mL) + 42.2 with a detection limit of 3 pg/mL (R2 = 0.988). For comparison, we also investigated the analytical performance of the immunosensor using Au-HRP-Ab2 and HRP-anti-AFP as detection antibodies, respectively. The regression equations, linear ranges, and detection limits are as follows:
Figure 10 Calibration curves for the electrochemical immunosensor towards the AFP standard using various detection antibodies: (a) Fe3O4/Au-HRP-Ab2, (b) Au-HRP-Ab2, and (c) HRP-anti-AFP (inset: cyclic voltammograms of the immunosensor using Fe3O4/Au-HRP-Ab2 towards AFP standard with various concentrations from bottom to top: 0, 0.005, 0.01, 0.05, 0.5, 1, 5 and 10 ng/mL in pH 6.5 phosphate-buffered solution containing 5 mM H2O2 and 2 mM hydroquinone).
Abbreviations: Ab2, secondary antibody; AFP, alfa-fetoprotein; HRP, horseradish peroxidase.
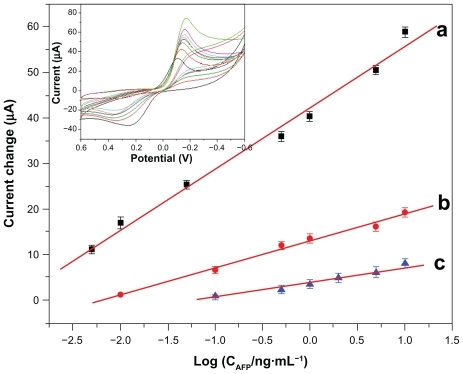
These results suggest that Fe3O4/Au could greatly improve the sensitivity and work range of the immunosensor. Although the threshold of human serum AFP is 10 ng/mL, actual serum samples should be diluted during the clinical diagnostic process, so the concentrations detected are usually <10 ng/mL. Small volume samples are preferable. Thus, the sensitivity of this immunoassay was adequate for practical application.
Regeneration of immunosensors is of great interest to immunoanalysts. Traditional regeneration of the immunosensor usually requires a different regeneration solution, such as an acid, alkali, or electrolyte solution at a high concentration to break the linkage between the antigen and antibody. In the present work, the immunosensor could be regenerated by simply using an external magnetic field to remove the Fe3O4/Au-HRP-Ab2-bonded antigen. Experimental results indicated that the immunosensor could retain 90.7% of the initial current response for 18 applications once regenerated.
Immunosensor specificity, reproducibility, and stability
Specificity is an important feature of the immunosensor. To investigate this, the immunosensor was incubated with 1 ng/mL AFP containing a different interference substance, such as carcinoma antigen 125, carcinoembryonic antigen, human IgG, prostate-specific antigen, and bovine serum albumin. No remarkable change of currents was observed in comparison with the results obtained in the presence of AFP only, indicating good selectivity of the proposed AFP immunosensor.
The reproducibility of the proposed immunosensor was evaluated by intra-assay and interassay coefficients of variation. The intra-assay precision of the analytical method was evaluated by analyzing one immunosensor for six replicate determinations. Coefficients of variation for the intra-assay were 3.6% and 4.7% at 0.1 ng/mL and 1.0 ng/mL AFP, respectively. Similarly, the interassay coefficients of variation for six immunosensor determinations were 3.9% and 5.5% at 0.1 ng/mL and 1.0 ng/mL AFP, respectively. These results demonstrate the acceptable reproducibility and precision of the proposed immunosensor.
We also examined the stability of the developed immunosensor. When not in use, the immunosensor could be stored in pH 7.0 phosphate-buffered solution at 4°C for at least 3 weeks without any obvious signal change. Moreover, the immunosensor retained 89.4% of its initial response after a storage period of 4 weeks. The slow decrease in current response may be attributed to gradual deactivation of biomolecules immobilized on the nanoparticle surface.
Application of the immunosensor in human serum
The electrochemical immunosensor was further validated by assaying six serum specimens gifted from the Nanfang Medical Hospital, China. The serum was prepared by collecting whole blood, leaving it undisturbed for 15–30 minutes at room temperature to clot, and then removing the clot by centrifugation at 1000–2000 rpm for 10 minutes in a refrigerated centrifuge, and collecting the supernatant serum. describes the correlation between the partial results obtained by the proposed immunosensor and the ELISA method, indicating no significant difference between the two methods, ie, the proposed immunosensor could be used for satisfactory clinical determination of AFP levels in human serum.
Table 1 Comparison of serum AFP levels determined using two methods
Conclusion
We have successfully designed a multienzyme labeling Fe3O4/Au strategy as part of a signal amplification procedure and demonstrated its use in the ultrasensitive, selective, and accurate quantification of AFP by electrochemical immunoassay. Highlights of the developed immunoassay are: Fe3O4/Au as the enzyme-loading carrier can load many enzyme molecules on each Fe3O4/Au nanoparticle, thus enhancing the sensitivity of the immunosensor; using Fe3O4/Au as signal enhancers favors rapid separation and purification of the signal antibody on an external magnetic field; after each detection, the immunosensor can be easily regenerated by applying an external magnetic field. The proposed immunosensor has high sensitivity, good reproducibility, stability, and accuracy. We anticipate that this method can be extended for determination of other proteins and have promising potential in clinical applications.
Acknowledgments
This work was supported by the National Natural Science Foundation of China (20805024, 81072336), the Natural Science Foundation of Ningbo (2011A610018, 2011A610006), the Social Development Project of Ningbo (2011C50038), and the KC Wong Magna Fund in Ningbo University, Science and Technology Planning Project of Guangdong Province (2010A030300006, 2008A050200006).
Disclosure
The authors report no conflicts of interest in this work.
References
- GitlinDPerricelliAGitlinGMSynthesis of α-fetoprotein by liver, yolk sac, and gastrointestinal tract of the human conceptusCancer Res1972329799824111729
- WonYSLeeSWTargeted retardation of hepatocarcinoma cells by specific replacement of alpha-fetoprotein RNAJ Biotechnol200712961461917360066
- BaderaDRiskinaAVafsiaOAlpha-fetoprotein in the early neonatal period – a large study and review of the literatureClin Chim Acta2004349152315469851
- TatsutaMYamamuraHLishiHKasugaiHOkudaSValue of serum alpha-fetoprotein and ferritin in the diagnosis of hepatocellular carcinomaOncology1986433063102429242
- JiaCPZhongXQHuaBNano-ELISA for highly sensitive protein detectionBiosens Bioelectron2009242836284119339168
- WangHYSunDYTanZAGongWWangLElectrochemiluminescence immunosensor for α-fetoprotein using Ru(bpy)32+-encapsulated liposome as labelsColloids Surf B Interfaces201184515519
- YuanSRYuanRChaiYQSandwich-type electrochemiluminescence immunosensor based on Ru-silica@Au composite nanoparticles labeled anti-AFPTalanta2010821468147120801357
- FuZFHaoCFeiXQJuHXFlow-injection chemiluminescent immunoassay for α-fetoprotein based on epoxysilane modified glass microbeadsJ Immunol Methods2006312616716647079
- BiSYanYMYangXYZhangSSGold nanolabels for new enhanced chemiluminescence immunoassay of alpha-fetoprotein based on magnetic beadsChem Eur J2009154704470919291715
- HomolaJSurface plasmon resonance sensors for detection of chemical and biological speciesChem Rev200810846249318229953
- TeramuraYJIwataHLabel-free immunosensing for a-fetoprotein in human plasma using surface plasmon resonanceAnal Biochem200736520120717459320
- LuoYChenMWenQJRapid and simultaneous quantification of 4 urinary proteins by piezoelectric quartz crystal microbalance immunosensor arrayClin Chem2006522273228017053152
- ChouSFHsuWLHwangJMChenCYDetermination of α-fetoprotein in human serum by a quartz crystal microbalance-based immunosensorClin Chem20024891391812029008
- ZhangYXZhangZJYangFA sensitive immunoassay for determination of hepatitis B surface antigen and antibody in human serum using capillary electrophoresis with chemiluminescence detectionJ Chromatogr B2007857100107
- WangJIbanezAChatrathiMPEscarpaAElectrochemical enzyme immunoassays on microchip platformsAnal Chem2001735323532711721936
- LinJHHeCYZhangLJZhangSSSensitive amperometric immunosensor for a-fetoprotein based on carbon nanotube/gold nanoparticle doped chitosan filmAnal Biochem200938413013518848914
- WeiQMaoKXWuDA novel label-free electrochemical immunosensor based on graphene and thionine nanocompositeSensor Actutators B2010149314318
- SuBLTangDPLiQFTangJChenGNGold-silver-graphene hybrid nanosheets-based sensors for sensitive amperometric immunoassay of alpha-fetoprotein using nanogold-enclosed titania nanoparticles as labelsAnal Chim Acta201169211612421501720
- WuYFChenCLLiuSQEnzyme-functionalized silica nanoparticles as sensitive labels in biosensingAnal Chem2009811600160719140671
- CuiRJPanHCZhuJJChenHYVersatile immunosensor using CdTe quantum dots as electrochemical and fluorescent labelsAnal Chem2007798494850117927140
- HoJALinYCWangLSHwangKCChouPTCarbon nanoparticle-enhanced immunoelectrochemical detection for protein tumor marker with cadmium sulfide biotracersAnal Chem2009811340134619159204
- CuiRJLiuCShenJMGaoDZhuJJChenHYGold nanoparticle-colloidal carbon nanosphere hybrid material: preparation, characterization, and application for an amplified electrochemical immunoassayAdv Funct Mater20081821972204
- JieGFHuangHPSunXLZhuJJElectrochemiluminescence of CdSe quantum dots for immunosensing of human prealbuminBiosens Bioelectron2008231896189918406128
- CuiRJHuangHPYinZZGaoDZhuJJHorseradish peroxidase-functionalized gold nanoparticle label for amplified immunoanalysis based on gold nanoparticles/carbon nanotubes hybrids modified biosensorBiosens Bioelectron2008231666167318359217
- TangJSuBLTangDPChenGNConductive carbon nanoparticles-based electrochemical immunosensor with enhanced sensitivity for α-fetoprotein using irregular-shaped gold nanoparticles-labeled enzyme-linked antibodies as signal improvementBiosens Bioelectron2010252657266220483583
- TangDPSuBLTangJRenJJChenGNNanoparticle-based sandwich electrochemical immunoassay for carbohydrate antigen 125 with signal enhancement using enzyme-coated nanometer-sized enzyme-doped silica beadsAnal Chem2010821527153420095621
- TangJHuangJXSuBLChenHFTangDPSandwich-type conductometric immunoassay of alpha-fetoprotein in human serum using carbon nanoparticles as labelsBiochem Eng J201153223228
- DuDZouZXShinYSFunctionalized graphene oxide as a nanocarrier in a multienzyme labeling amplification strategy for ultrasensitive electrochemical immunoassay of phosphorylated p53 (S392)Anal Chem2010822989299520201502
- MalhotraRPatelVVaqueJPGutkindJSRuslingJFUltrasensitive electrochemical immunosensor for oral cancer biomarker IL-6 using carbon nanotube forest electrodes and multilabel amplificationAnal Chem2010823118312320192182
- DuDWangLMShaoYYWangJEngelhardMHLinYHSensitive immunosensor for cancer biomarker based on dual signal amplification strategy of graphene sheets and multienzyme functionalized carbon nanospheresAnal Chem20118374675221210663
- SanchezCJulianBBellevillePPopallMApplications of hybrid organic- inorganic nanocompositesJ Mater Chem20051535593592
- TangDPYuanRChaiYQUltrasensitive electrochemical immunosensor for clinical immunoassay using thionine-doped magnetic gold nanospheres as labels and horseradish peroxidase as enhancerAnal Chem2008801582158818220412
- XuLXKimMJKimKDChoaYHKimHTSurface modified Fe3O4 nanoparticles as a protein delivery vehicleColloids Surf., A2009350812
- ParkMEChangJHHigh throughput human DNA purification with aminosilanes tailored silica-coated magnetic nanoparticlesMater Sci Eng., C20072712321235
- KimDKZhangYVoitWSuperparamagnetic iron oxide nanoparticles for bio-medical applicationsScripta Mater20014417131717
- PankhurstQAConnollyJJonesSKDobsonJApplications of magnetic nanoparticles in biomedicineJ Phys D: Appl Phys200336167181
- NasrMBGoodeDPNguyenNQuantum optical coherence tomography of a biological sampleOpt Commun200928211541159
- SkrabalakSEChenJYSunYGGold nanocages: synthesis, properties, and applicationsAcc Chem Res2008411587159518570442
- SkrabalakSEChenJYAuLLuXMLiXDXiaYNGold nanocages for biomedical applicationsAdv Mater2007193177318418648528
- AmbrosiACastanedaMTKillardAJSmythMRAlegretSMerkociADouble-codified gold nanolabels for enhanced immunoanalysisAnal Chem2007795232524017579481
- AlfredoMChristianSEFreitasBDFernandezAGMarisaMCArbenMRapid identification and quantification of tumor cells using an electrocatalytic method based on gold nanoparticlesAnal Chem200981102681027419911778
- AmbrosiAAiroFMerkocAEnhanced gold nanoparticle based ELISA for a breast cancer biomarkerAnal Chem2010821151115620043655
- QiuJDXiongMLiangRPPengHPLiuFFacile preparation of magnetic core/shell Fe3O4@Au nanoparticle/myoglobin biofilm for direct electrochemistryBiosens Bioelectron2009242649265319230651
- ParkHYSchadtMJWangLYFabrication of magnetic core@ shell Fe oxide@Au nanoparticles for interfacial bioactivity and bio- separationLangmuir2007239050905617629315
- LiuHLSonnCHWuJHLeeKMKimYKSynthesis of streptavidin-FITC-conjugated core–shell Fe3O4-Au nanocrystals and their application for the purification of CD4+ lymphocytesBiomaterials2008294003401018649937
- SonSJReichelJHeBSchuchmanMLeeSBMagnetic nanotubes for magnetic-field-assisted bioseparation, biointeraction, and drug deliveryJ Am Chem Soc20051277316731715898772
- FrensGControlled nucleation for the regulation of the particle size in monodisperse gold suspensionsNature Phys Sci19732412022
- CuiYLZhangLYSuJPreparation and mechanism of Fe3O4/Au core/shell super-paramagnetic microspheresSci Ser B200144404410
- XuJShangFJLuongJHTRazeebKMGlennonJDDirect electrochemistry of horseradish peroxidase immobilized on a monolayer modified nanowire array electrodeBiosens Bioelectron2010251313131819914056
- KouassiGKIrudayarajJMcCartyGExamination of cholesterol oxidase attachment to magnetic nanoparticlesJ Nanobiotechnology200531915661076
- ThandavanKGandhiSSethuramanSA novel nanostructured iron oxide-gold bioelectrode for hydrogen peroxide sensingNanotechnology20112255055515