Abstract
Background
p-Phenylenediamine (PDA) or its related chemicals are used more extensively than oxidative hair dyes. However, permanent hair dyes such as PDA are known to have potent contact allergy reactions in humans, and severe allergic reactions are problematic.
Methods
PDA-incorporated nanoparticles were prepared based on ion-complex formation between the cationic groups of PDA and the anionic groups of poly(γ-glutamic acid) (PGA). To reinforce PDA/PGA ion complexes, glycol chitosan (GC) was added. PDA-incorporated nanoparticles were characterized using field-emission scanning electron microscopy, Fourier- transform infrared (FT-IR) spectroscopy, dynamic light scattering, and powder X-ray diffractometry (XRD).
Results
Nanoparticles were formed by ion-complex formation between the amine groups of PDA and the carboxyl groups of PGA. PDA-incorporated nanoparticles are small in size (<100 nm), and morphological observations showed spherical shapes. FT-IR spectra results showed that the carboxylic acid peak of PGA decreased with increasing PDA content, indicating that the ion complexes were formed between the carboxyl groups of PGA and the amine groups of PDA. Furthermore, the intrinsic peak of the carboxyl groups of PGA was also decreased by the addition of GC. Intrinsic crystalline peaks of PDA were observed by XRD. This crystalline peak of PDA was completely nonexistent when nanoparticles were formed by ion complex between PDA, PGA, and GC, indicating that PDA was complexed with PGA and no free drug existed in the formulation. During the drug-release experiment, an initial burst release of PDA was observed, and then PDA was continuously released over 1 week. Cytotoxicity testing against HaCaT human skin keratinocyte cells showed PDA-incorporated nanoparticles had lower toxicity than PDA itself. Furthermore, PDA-incorporated nanoparticles showed reduced apoptosis and necrosis reaction at HaCaT cells.
Conclusion
The authors suggest that these microparticles are ideal candidates for a vehicle for decreasing side effects of hair dye.
Keywords:
Introduction
In recent decades, permanent hair dyes have received considerable attention due to their potent contact allergen p-phenylenediamine (PDA). PDA or its related chemicals are used more extensively than oxidative hair dyes. However, hypersensitivity, immune response, or severe allergic reactions following hair-dyeing have been reported.Citation1 In particular, consumer-available PDA-containing hair dyes are known to be potent immune activators that lead to increased inflammatory cytokines.Citation2 Furthermore, PDA may cause deoxyribonucleic acid (DNA) cleavage or gene toxicity against human skin keratinocyte cells under light irradiation.Citation3 Derivatives of PDA chemicals are known to induce DNA damage and carcinogenic reactions in human skin keratinocyte cells.Citation4,Citation5
Nanoparticles have been extensively investigated to attain efficient drug delivery to a desired site of action and to control drug release for a desired length of time.Citation6,Citation7 Due to their small sizes, nanoparticles can be administered via various routes such as parenteral, oral, ocular, dermal or transdermal, and intranasal inhalation.Citation8–Citation14 Nanoparticles are regarded as ideal vehicles for targeted delivery to a specific body site and for reduction of unwanted side effects of bioactive agents.Citation7–Citation9 Particularly, nanoparticles are known to be efficient carriers for topical delivery of bioactive agents to the skin and hair.Citation13,Citation14 Lademann et al reported that dye-containing nanoparticles penetrate much deeper into the hair follicles and are stored in the hair follicles longer than the nonparticle form.Citation14 Hair ingredient-encapsulated nanoparticles are known to enhance hair growth.Citation15 Furthermore, the intrinsic small size of nanoparticles is regarded as playing an important role in skin penetration and dermal drug delivery.Citation16
In this study, the formation of nanoparticles incorporating PDA was investigated. PDA-incorporated nanoparticles were prepared through ion-complex formation between hair dye and poly(γ-glutamic acid) (PGA)/chitosan. The physicochemical properties of PDA-incorporated nanoparticles were studied using dynamic light scattering (DLS), Fourier-transform infrared (FT-IR), powder X-ray diffractometry (XRD), and field-emission scanning electron microscopy (FE-SEM). Furthermore, the cytotoxicity of PDA-incorporated nanoparticles was tested using human skin keratinocyte cells.
Materials and methods
Materials
PGA (molecular weight 200,000–500,000 g/mol) was purchased from WakoPure Chem Co (Osaka, Japan). Glycol chitosan (GC) was a product of MP Biomedicals, LLC (Santa Ana, CA). Propidium iodide (PI) and PDA were purchased from Sigma-Aldrich Co (St Louis, MO). Fluorescein isothiocyanate (FITC)-annexin V was obtained from Santa Cruz Biotechnology, Inc (Santa Cruz, CA).
Preparation of PDA-incorporated nanoparticles
PGA was distributed in 5 mL of deionized water and magnetically stirred for 30 minutes. PDA (5–40 mg) dissolved in 2 mL of deionized water was then added to this solution and stirred for 30 minutes. GC dissolved in 5 mL deionized water was added to this solution, and stirred for 30 minutes at room temperature. The resulting solutions were then lyophilized or analyzed.
Characterization of PDA-incorporated nanoparticles
Particle size of nanoparticles was measured with DLS (DLS-7000; Otsuka Electronics Co, Osaka, Japan).
FT-IR spectroscopy (FT-IR 8700; Shimadzu, Osaka, Japan) was employed for ion-complex formation between PDA and polymers. PDA or lyophilized nanoparticles were used for analysis.
The morphology of the nanoparticles was observed with FE-SEM (S-4700; Hitachi Co Ltd, Tokyo, Japan). Microspheres were placed on double-sided tape attached to a graphite surface. Each sample was coated with gold/palladium using an ion sputter (JFC-1100; Jeol Ltd, Tokyo, Japan). Coating was done at 20 mA for 4 minutes. Observation was done at 15 kV.
XRD diffractograms were employed to analyze crystallinity of drug and drug-incorporated nanoparticles using a Rigaku D/Max-1200 (Rigaku, Tokyo, Japan) equipped with Ni-filtered Cu Kα radiation (40 kV, 20 mA). The conditions of powder XRD measurement were as follows:
Data type = binary; goniometer = 1; attachment = 1; scan mode = continuous
Mode 2 (R/T) = reflection; scan axis = 2θ/θ
Start angle = 10,000; stop angle = 80,000; scan speed = 5000; sampling interval = 0.050; θ angle = 5000; 2θ angle = 10,000; fixed time = 0.01; full scale = 1000; counting unit = CPS; target = Cu
Wavelength, Kα1 = 1.540510; wavelength, Kα2 = 1.544330; wavelength, Kα = 1.541780; wavelength, Kβ = 1.392170
40.0 kV; 20.0 mA
PDA, PGA, GC, and PDA-incorporated nanoparticles were used to measure powder XRD. For physical mixture of empty nanoparticles and PDA, 90 mg of empty nanoparticles was mixed with 10 mg of PDA.
Drug-release study
The drug-release test was as follows: 5 mg of PDA-incorporated nanoparticles were redistributed in 5 mL of phosphate-buffered saline (PBS) (0.1 M, pH 7.4), and then this solution was placed in a dialysis tube. This dialysis tube was introduced into a 100 mL bottle with 95 mL of PBS (0.01 M, pH 7.4). At predetermined time intervals, whole media were taken and exchanged with fresh PBS. The concentration of released PDA was measured with a high performance liquid chromatography (HPLC) system as reported by Hooff et al.Citation17 The Flexar™ HPLC system (PerkinElmer, Waltham, MA) consisted of a Solvent Manager 5-CH Degasser, an autosampler, a quaternary LC pump, a column oven, and an ultraviolet-visible detector. Gradient elution was performed as follows: elution solvent, 90% methanol with 10% water, and 0.01 M ammonium hydroxide (pH 9). The gradient was initiated at 5% elution solvent for 4.5 minutes, and subsequently a linear gradient led to 95% elution solvent in 5 minutes, kept for 2 minutes, and brought back to 5% elution solvent in 0.5 minutes. A Luna phenyl-hexyl column from Phenomenex (Aschaffenburg, Germany) was kept at 20°C for the separation. PDA was monitored at wavelengths of 240 nm and 310 nm.
Cell culture
For cytotoxicity testing of PDA and PDA-incorporated nanoparticles, HaCaT human keratinocyte cell line obtained from the Korean Cell Line Bank (Seoul, Korea) was maintained in Dulbecco’s modified Eagle’s medium (DMEM) medium at 37°C and 5% CO2 atmosphere.
MTT (3-[4,5-dimethylthiazol-2-yl]-2, 5-diphenyltetrazolium bromide) assay
The medium for cell culture and proliferation inhibition assay was DMEM supplemented with 10% fetal bovine serum; cells were maintained in this medium in a 5% CO2 incubator (37°C). The cytotoxicity of PDA and PDA-incorporated nanoparticles was determined using an MTT cell proliferation assay. HaCaT cells were seeded in 96-well plates at a density of 1 × 104 cells/well and incubated for 1 day in a 5% CO2 incubator at 37°C. PDA and PDA-incorporated nanoparticles in deionized water were then diluted with serum-free DMEM media and then added to the HaCaT cells. The control was treated with serum-free DMEM media. Three days later, cell viability was assayed using the MTT colorimetric assay. After the incubation period, 30 μL of MTT (5 mg/mL in PBS, pH 7.4) was added to each well and incubated for 4 hours. The formazan crystals were then solubilized with DMSO, and the absorbance (560 nm test/630 nm reference) was determined using an automated computer-linked microplate reader (Molecular Device Co, Sunnyvale, CA). Each experimental value was the mean of eight wells.
Flow cytometry analysis
Two reagents, PI and FITC-annexin V, were used to identify apoptosis and necrosis of HaCaT cells, respectively. Cells were treated with various concentrations of PDA and PDA-incorporated nanoparticles for 24 hours. After that, cells were harvested and washed with PBS. The pellets were resuspended with binding buffer (HEPES [10 mM 4-(2- hydroxyethyl)-1-piperazineethanesulfonic acid] pH 7.4, 150 mM NaCl, 5 mM KCl, 1 mM MgCl2, 1.8 mM CaCl2) containing FITC-annexin V (1 μg/mL) and further incubated for 30 minutes. Ten minutes prior to termination of incubation, PI (10 μg/mL) was added to stain necrotic cells under dark conditions. Following that, the cells were immediately analyzed using a FACScan (Becton Dickinson Biosciences, San Jose, CA) flow cytometer.
Results and discussion
Characterization of PDA-incorporated nanoparticles
PDA-incorporated nanoparticles were prepared via ioncomplex formation between cationic groups of PDA and anionic groups of PGA (see ). As shown in , when PGA was added to deionized water, it was not soluble in water. However, clear solution was obtained when PDA was added. This result might indicate that ion complexes were formed between the amine groups of PDA and the carboxyl groups of PGA.
Figure 1 Schematic illustrations of PDA-incorporated nanoparticles based on ion-complex formation between PDA and PGA. Weight ratio of PDA/PGA was 20/50 (mg/mg). The opaque solution of PGA was readily changed to a transparent solution by addition of PDA.
Abbreviations: PDA, p-phenylenediamine; PGA, poly(γ-glutamic acid).
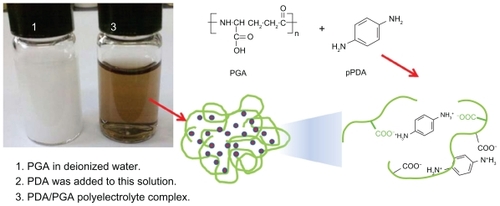
Prior to studying its chemical properties, the potential of nanoparticle formation was investigated. To investigate its colloidal properties, DLS was used to test particle size and FE-SEM was used to observe morphology. As shown in , the particle size was about 76.9 nm. Furthermore, spherical nanoparticles were observed during FE-SEM observation, as shown in . Chemical properties of PDA-incorporated nanoparticles were analyzed using FT-IR (see ). As shown in , the primary amine peak and the carboxylic acid peak appeared for PDA and PGA, respectively. Interestingly, the intrinsic carboxylic acid peak of PGA was significantly decreased at peak analysis of nanoparticles. Furthermore, the primary amine peak of PDA was also decreased in its nanoparticles, indicating that ion complexes were formed between amine groups of PDA and carboxyl groups of PGA.
Figure 2 Typical particle size (A) and SEM photo (B) of PDA-incorporated PGA nanoparticles.
Note: Composition of PDA/PGA is PDA5 in .
Abbreviations: PDA, p-phenylenediamine; PGA, poly(γ-glutamic acid); SEM, scanning electron microscopy.
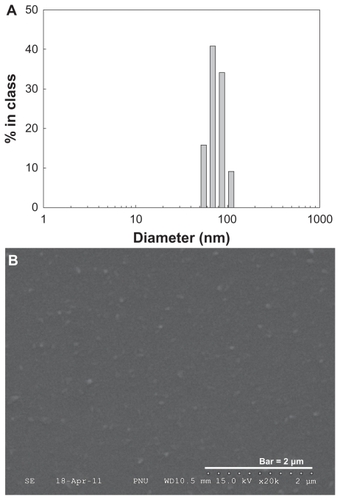
Figure 3 FT-IR analysis of PDA-incorporated nanoparticles.
Notes: PDA and PGA were used as a solid powder, and lyophilized PDA-incorporated nanoparticles (PDA5 in ) were used for FT-IR measurement. Carboxylic groups of PGA were decreased by complexation with PDA at PDA5 measurement.
Abbreviations: FT-IR, Fourier-transform infrared; PDA, p-phenylenediamine; PGA, poly(γ-glutamic acid).
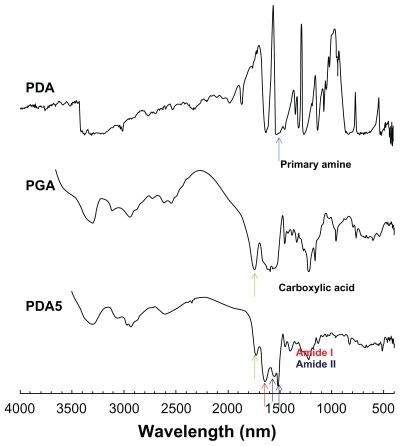
To reinforce PDA-incorporated nanoparticles, GC was added, as shown in (top pictures). Furthermore, changes of physicochemical properties were tested relative to the changes in PDA content and GC content, as shown in and . As shown in , the opaque PGA solution became a brown, transparent solution after addition of PDA (, top-left), indicating that the transparent solution was obtained by the formation of nanosized ion complexes. When GC was added, the particle size was increased (), and the nanoparticle solution became slightly turbid, as shown in (top-right).
Table 1 Characterization of PDA-incorporated submicroparticles of PGA/GC complexes
Figure 4 Photos of the PDA-incorporated nanoparticles. Photo in top-left box is of ion complexes of PDA-incorporated PGA nanoparticles: PDA/PGA weight ratio (w/w) (a) 50/5; (b) 50/10; (c) 50/20; and (d) 50/40. The top-right box is of nanoparticles after addition of GC, and the bottom photo is of PDA-incorporated nanoparticles.
Note: The composition of PDA/PGA/GC is illustrated in .
Abbreviations: GC, glycol chitosan; PDA, p-phenylenediamine; PGA, poly(γ-glutamic acid).
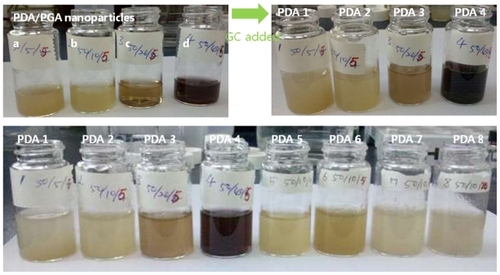
As shown in , the free carboxylic acid peak of PGA decreased with increase in PDA content, indicating that ion complexes between carboxyl groups and amine groups were increased and free carboxylic acid was decreased, as shown in . At a fixed amount of PDA, particle size was not significantly changed by variations in the amount of GC, as shown in . The peak of free carboxylic acid of PGA also decreased with increasing GC content, indicating that ion complexes between amine groups of GC and carboxyl groups of PGA had increased.
Figure 5 FT-IR analysis of PDA-incorporated nanoparticles, showing the effect of PDA content on the chemical properties of nanoparticles.
Notes: The composition of PDA/PGA/GC is illustrated in . PDA, PGA, and GC were used as a solid powder, and lyophilized PDA-incorporated nanoparticles (PDA1–5 in ) were used for FT-IR measurement. As PDA content increased, the carboxylic acid peak of PGA gradually decreased.
Abbreviations: GC, glycol chitosan; FT-IR, Fourier-transform infrared; PDA, p-phenylenediamine; PGA, poly(γ-glutamic acid).
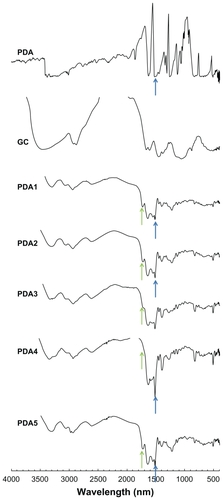
Figure 6 FT-IR analysis of PDA-incorporated nanoparticles showing the effect of GC addition on the chemical properties of nanoparticles.
Notes: The composition of PDA/PGA/GC is illustrated in . GC was used as a solid powder, and lyophilized PDA-incorporated nanoparticles (PDA5–8 in ) were used for FT-IR measurement. The carboxylic acid peak of PGA decreased with increasing GC content.
Abbreviations: GC, glycol chitosan; FT-IR, Fourier-transform infrared; PDA, p-phenylenediamine; PGA, poly(γ-glutamic acid).
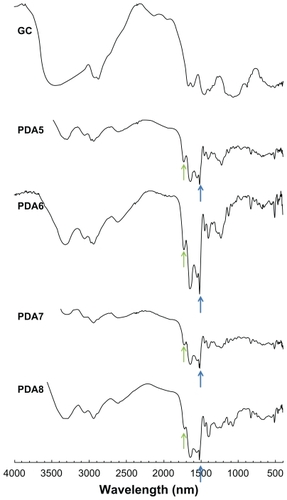
To investigate the physical state of the nanoparticles, powder XRD was employed (see ). As shown in , PDA had sharp crystalline peaks, while peaks of PGA and GC were relatively broad. Interestingly, crystalline peaks of PDA disappeared due to ion-complexes with PGA and nanoparticle formation, while physical mixtures of PDA and PGA still showed both sharp crystalline peaks of PDA and broad peaks of PGA. These results indicate that ion complexes between PDA, PGA, and GC were formed, and no large amount of free drug existed in the complexes with PGA. However, as shown in , a small amount of free drug may have remained when PDA content was 40 mg (PDA4 in and [arrow]).
Figure 7 XRD analysis of PDA-incorporated nanoparticles.
Notes: The composition of PDA/PGA/GC is illustrated in . PDA, PGA, and GC were used as a solid powder, and lyophilized PDA-incorporated nanoparticles (PDA1–8 in ) were used for XRD analysis. The physical mixture was PGA/PDA (weight ratio = 5:1). PDA itself has sharp crystalline peaks, while nanoparticles showed broad peaks for all compositions.
Abbreviations: GC, glycol chitosan; PDA, p-phenylenediamine; PGA, poly(γ-glutamic acid); XRD, X-ray diffractometry.

Drug release from PDA-incorporated nanoparticles
PDA release properties were investigated in vitro (see ). As shown in , PDA was continuously released over 1 week. PDA release rate increased with escalating PDA content. These results may be due to PDA being liberated at higher drug contents and being decomplexed easily from the nanocomplexes due to competition with each other. As shown in , the PDA release rate was also increased by the addition of GC. These results indicated that the increase in the amount of amine groups may enhance competition in the ion complexes with carboxylic acid, and this fact may increase release rate. For all samples, the initial burst release was observed until 6 hours, after which time, the PDA release rate was relatively sustained.
Figure 8 PDA release from nanoparticles: the effects of (A) PDA content and (B) the addition of GC.
Note: The composition of PDA/PGA/GC is illustrated in .
Abbreviations: GC, glycol chitosan; PDA, p-phenylenediamine; PGA, poly(γ-glutamic acid).
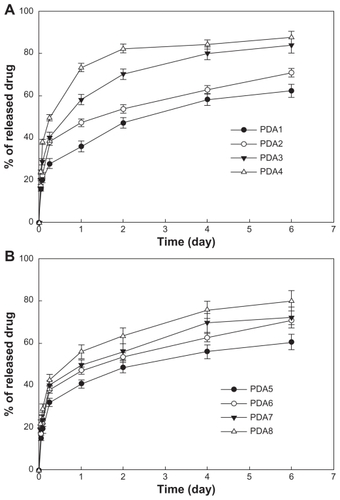
At the next step, formulation PDA2 (same as PDA6) was used because the aqueous solution of PDA2 was relatively stable compared with the other formulations, and its drug-release kinetics were relatively sustained.
Cell cytotoxicity of PDA-incorporated nanoparticles
To investigate cytotoxicity of PDA and PDA-incorporated nanoparticles, HaCaT human skin keratinocyte cells were employed, and cytotoxicity was evaluated using MTT cell proliferation assay. As shown in , cell viability was decreased in a dose-dependent manner when HaCaT cells were exposed to PDA itself. On the other hand, when HaCaT cells were exposed to PDA-incorporated nanoparticles, viability of cells was not significantly decreased. These results indicated that intrinsic cytotoxicity of PDA was alleviated. Although the reason for these results was not clear, the sustained-release properties of the nanoparticles might be affected by the alleviated toxicity, and another possibility is better biocompatibility of PGA/GC nanoparticles compared with PDA itself. The mechanistic study of cellular cytotoxicity was investigated using Annexin V staining for apoptosis analysis and PI staining for necrosis analysis. As shown in , both apoptosis and necrosis at PDA treatment was higher than nanoparticle treatment, indicating that nanosized ion-complex formation between PDA and ionic polymer decreased intrinsic cytotoxicity of PDA.
Figure 9 Cytotoxicity of PDA and PDA-incorporated nanoparticles (PDA2 in ) against HaCaT cells. HaCaT cells (1 × 104 cells) were seeded in 96-well plates, and then the cells were exposed to PDA or PDA-incorporated nanoparticles for 3 days. The cell viability was assayed using the MTT colorimetric assay.
Abbreviations: MTT, 3-(4,5-dimethylthiazol-2-yl)-2,5-diphenyltetrazolium bromide; NP, PDA-incorporated nanoparticles; PDA, p-phenylenediamine.
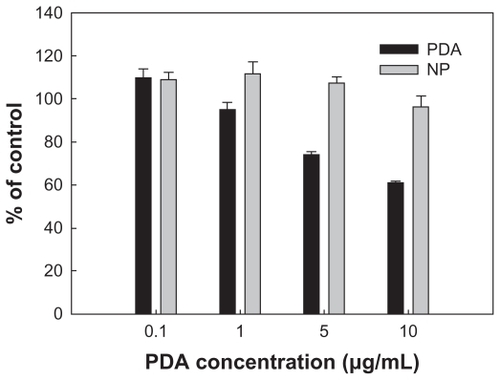
Figure 10 Flow cytometry analysis of PDA and PDA-incorporated nanoparticles (PDA2 in ) against HaCaT cells. FITC-annexin V (Annexin V) and PI were used for apoptosis and necrosis of cells, respectively. Apoptosis or necrosis of cells were analyzed using a FACScan (Becton Dickinson Biosciences, San Jose, CA) flow cytometer.
Abbreviations: FITC, fluorescein isothiocyanate; NP, PDA-incorporated nanoparticles; PDA, p-phenylenediamine; PI, propidium iodide.

Discussion
Nanoparticles have been extensively investigated in the biomedical, cosmetic, and health industries.Citation7–Citation21 In particular, cosmetic application of nanoparticles is regarded as the best technology to upgrade the performance of cosmetic products and to meet the consumer’s needs.Citation19 For example, Popov et al showed that potential application of zinc oxide or titanium oxide nanoparticles for sunscreen has benefit to attenuate ultraviolet light by absorption and scattering.Citation20 Furthermore, the effect of particle size on the potential of titanium oxide nanoparticles as a sunscreen was also reported by other researchers.Citation22 Even though intrinsic toxicity was problematic,Citation23 ingredients based on nanotechnology in cosmetic products are known to have no more risk to human health than larger-sized counterparts.Citation21
Due to hypersensitivity, allergy reaction, and immune response, permanent hair dyes such as PDA and its related chemicals have been widely investigated.Citation1–Citation5 Since PDA or its related chemicals are used more extensively than oxidative hair dyes, their immune-toxicity, carcinogenesis, and genetic toxicity is problematic to human skin keratinocyte cells.Citation4,Citation5 Mosley-Foreman et alCitation3 reported that permanent hair dye revealed cytotoxicity against human skin keratinocyte cells under light irradiation, and no chemical structure-related phototoxicity of derivatives of PDA was found.
This current report describes the preparation of PDA-incorporated nanoparticles using PGA and GC. PDA itself did not form nanoparticles in aqueous solutions (data not shown). Furthermore, PGA itself did not form nanoparticles, and PGA was not clearly dissolved in deionized water, ie, it was simply distributed in the deionized water. However, opaque PGA solution was readily changed into a transparent solution when PDA was added, as shown in . Interestingly, PGA/PDA mixtures were found to form nanoparticles in the aqueous solution based on ion-complex formation between anionic groups of PGA and cationic groups of PDA. Since the formation of these nanoparticles did not require organic solvent or surfactant, nanoparticles based on ion complex between PGA and PDA are very interesting. Nanocomplexes based on polyelectrolytes between anionic compounds and cationic compounds have been extensively investigated by several researchers.Citation24–Citation28 Thünemann and Beyermann reported that retinoic acid as an anionic compound can form ion complexes with cationic polymers such as polyethyleneimine, and these complexes are nanosized carriers.Citation24 Furthermore, they also reported that another cationic polymer, poly(ethylene glycol)/poly(L-lysine) block copolymer, was also employed to form polyion complex micelles. Jeong et al reported that retinoic acid and chitosan can form polyion complex micelles via interaction between amine groups of chitosan and carboxyl groups of retinoic acid, while chitosan itself does not form polymeric micelles.Citation26 In another report, they described nanoparticle formation based on ion complexes between hyaluronic acid and cisplatin.Citation27 Furthermore, they also reported that carboxymethyl (CM) chitosan can form nanoparticles via ion-complex formation between carboxyl groups of CM chitosan and amine groups of doxorubicin.Citation28 As shown in –, the results of this present study showed that PDA-incorporated nanoparticles can be formed by ion complexes between amine groups of PDA and carboxyl groups of PGA. The complexes formed nanoparticles of small size (<1000 nm) and spherical shape. Furthermore, intrinsic cytotoxicity of PDA can be reduced by nanoparticle formation, as shown in and . Even though initial burst release of PDA was observed, as shown in , PDA-incorporated nanoparticles showed sustained release of PDA over 1 week. Even though the reason for the initial burst release of PDA up to 6 hours is not clear at this moment, we assumed that some of the PDA was weakly attached on the surface of the nanoparticles and then these drugs were liberated rapidly. Another reason for this initial-burst phenomenon might be due to the existence of free drug on the surface of the nanoparticles at higher drug concentrations, as shown in (arrow, PDA4). However, no drug crystalline peaks were observed for the other formulations. Therefore, the initial burst release from the nanoparticles must be due to the weak interaction between the drug and the polymer at the nanoparticle surface rather than the existence of free drug. White et al reported that intermittent exposure to low-concentration PDA can be equivalent to a single, higher-dose exposure.Citation29 The authors of this present paper suggest that PDA-incorporated nanoparticles may reduce intrinsic toxicity of PDA and enhance potential application in the cosmetic industry.
Conclusion
PDA-incorporated nanoparticles were prepared based on ion-complex formation between cationic groups of PDA and anionic groups of PGA. To reinforce PDA/PGA ion complexes, GC was added. PDA-incorporated nanoparticles are small in size (<100 nm), and morphological observations showed spherical shapes. FT-IR spectra showed the carboxylic acid peak of PGA decreased with increasing PDA content, indicating that ion complexes were formed between carboxyl groups of PGA and amine groups of PDA. XRD showed intrinsic crystalline peaks of PDA completely disappeared when nanoparticles were formed by ion complexes between PDA, PGA, and GC, indicating that PDA was complexed with PGA and no free drug existed in the formulation. The drug-release experiment showed an initial burst release of PDA and then continuous release over 1 week. Cytotoxicity of PDA-incorporated nanoparticles against HaCaT human skin keratinocyte cells was lower than PDA alone. Furthermore, PDA-incorporated nanoparticles showed reduced apoptosis and necrosis reaction in HaCaT cells.
Acknowledgment
This work was partially supported by a Grant-in-Aid (No. PJ007538) for scientific research from the Ministry for Food, Agriculture, Forestry and Fisheries of Korea.
Disclosure
The authors report no conflicts of interest in this work.
References
- GoebelCCoenraadsPJRotheHElicitation of the immune response to p-phenylenediamine in allergic patients: the role of dose and exposure timeBr J Dermatol20101631205121120795999
- RubinIMDabelsteenSNielsenMMRepeated exposure to hair dye induces regulatory T cells in miceBr J Dermatol201016399299820716223
- Mosley-ForemanCChoiJWangSYuHPhototoxicity of phenylenediamine hair dye chemicals in Salmonella typhimurium TA102 and human skin keratinocytesFood Chem Toxicol2008463780378418940226
- MurataMNishimuraTChenFKawanishiSOxidative DNA damage induced by hair dye components ortho-phenylenediamines and the enhancement by superoxide dismutaseMutat Res200660718419116798066
- ChenFMurataMHirakuYYamashitaNOikawaSKawanishiSDNA damage induced by m-phenylenediamine and its derivative in the presence of copper ionFree Radic Res1998291972059802551
- KreuterJPossibilities of using nanoparticles as carriers for drugs and vaccinesJ Microencapsul198851151273058924
- GrefRMinamitakeYPeracchiaMTTrubetskoyVTorchilinVLangerRBiodegradable long-circulating polymeric nanospheresScience1994263160016038128245
- GulatiNGuptaHParenteral drug delivery: a reviewRecent Pat Drug Deliv Formul2011513314521453250
- TakeuchiHSugiharaHAbsorption of calcitonin in oral and pulmonary administration with polymer-coated liposomesYakugaku Zasshi20101301135114220823671
- SchroeterAEngelbrechtTNeubertRHGoebelASNew nanosized technologies for dermal and transdermal drug delivery. A reviewJ Biomed Nanotechnol2010651152821329045
- du ToitLCPillayVChoonaraYEGovenderTCarmichaelTOcular drug delivery – a look towards nanobioadhesivesExpert Opin Drug Deliv20118719421174606
- Alvarez-RománRNaikAKaliaYNGuyRHFessiHEnhancement of topical delivery from biodegradable nanoparticlesPharm Res2004211818182515553228
- Alvarez-RománRNaikAKaliaYNGuyRHFessiHSkin penetration and distribution of polymeric nanoparticlesJ Control Release200499536215342180
- LademannJRichterHTeichmannANanoparticles – an efficient carrier for drug delivery into the hair folliclesEur J Pharm Biopharm20076615916417169540
- TsujimotoHHaraKTsukadaYEvaluation of the permeability of hair growing ingredient encapsulated PLGA nanospheres to hair follicles and their hair growing effectsBioorg Med Chem Lett2007174771477717658251
- SchneiderMStrackeFHansenSSchaeferUFNanoparticles and their interactions with the dermal barrierDermatoendocrinol2009119720620592791
- HooffGPvan HuizenNAMeestersRJAnalytical investigations of toxic p-phenylenediamine (PPD) levels in clinical urine samples with special focus on MALDI-MS/MSPLoS One20116e2219121829608
- SinghRNalwaHSMedical applications of nanoparticles in biological imaging, cell labeling, antimicrobial agents, and anticancer nanodrugsJ Biomed Nanotechnol2011748950321870454
- WiechersJWMuseeNEngineered inorganic nanoparticles and cosmetics: facts, issues, knowledge gaps and challengesJ Biomed Nanotechnol2010640843121329039
- PopovAPZvyaginAVLademannJDesigning inorganic light-protective skin nanotechnology productsJ Biomed Nanotechnol2010643245121329040
- EpsteinHANanotechnology in cosmetic productsSkinmed2011910911021548515
- WokovichATynerKDoubWSadriehNBuhseLFParticle size determination of sunscreens formulated with various forms of titanium dioxideDrug Dev Ind Pharm2009351180118919555241
- KongBSeogJHGrahamLMLeeSBExperimental considerations on the cytotoxicity of nanoparticlesNanomedicine (Lond)2011692994121793681
- ThünemannAFBeyermannJPolyethyleneimine complexes with retinoic acid: structure, release profiles, and nanoparticlesMacromolecules20003368786885
- ThünemannAFBeyermannJKukulaHPoly(ethylene oxide)- b-poly(L-lysine) complexes with retinoic acidMacromolecules20003359065911
- JeongYIKimSHJungTYPolyion complex micelles composed of all-trans retinoic acid and poly(ethylene glycol)-grafted-chitosanJ Pharm Sci2006952348236016886178
- JeongYIKimSTJinSGCisplatin-incorporated hyaluronic acid nanoparticles based on ion-complex formationJ Pharm Sci2008971268127617674407
- JeongYIJinSGKimIYDoxorubicin-incorporated nanoparticles composed of poly(ethylene glycol)-grafted carboxymethyl chitosan and antitumor activity against glioma cells in vitroColloids Surf B Biointerfaces20107914915520427160
- WhiteJMBasketterDAPeaseCKSandersDAMcFaddenJPIntermittent exposure to low-concentration paraphenylenediamine can be equivalent to single, higher-dose exposureContact Dermatitis20075626226517441847