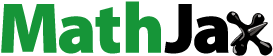
Abstract
Introduction
Doxycycline, a broad-spectrum antibiotic, is the most commonly prescribed antibiotic worldwide for treating infectious diseases. It may be delivered orally or intravenously but can lead to gastrointestinal irritation and local inflammation. For treatment of uterine infections, transcervical administration of doxycycline encapsulated in nanoparticles made of biodegradable chitosan may improve sustained delivery of the drug, thereby minimizing adverse effects and improving drug efficacy.
Methods and materials
As a first step toward assessing this potential, we used an ionic gelation method to synthesize blank and doxycycline-loaded chitosan nanoparticles (DCNPs), which we then characterized in terms of several properties relevant to clinical efficacy: particle size, shape, encapsulation efficiency, antibacterial activity, and in vitro cytotoxicity. Two particle formulations were examined, with one (named DCNP6) containing approximately 1.5 times the crosslinker concentration of the other (DCNP4).
Results
The two formulations produced spherically shaped drug-loaded nanoparticles. The spheres ranged in size from 30 to 220 nm diameter for DCNP4 and 200 to 320 nm diameter for DCNP6. Average encapsulation yield was 53% for DCNP4 and 56% for DCNP6. In terms of drug release, both formulations showed a burst effect within the first 4 to 5 hours, followed by a slow, sustained release for the remainder of the 24-hour monitoring period. The in vitro antibacterial activity against Escherichia coli was high, with both formulations achieving more than 90% inhibition of 4-hour bacterial growth. Cytotoxic effects of the DCNPs on normal human ovarian surface epithelial cells were significantly lower than those of unencapsulated doxycycline. After 5 days, cultures exposed to the unencapsulated antibiotic showed a 61% decrease in cell viability, while cultures exposed to the DCNPs exhibited less than a 10% decrease.
Conclusion
These laboratory results suggest that DCNPs show preliminary promise for possible eventual use in transcervical drug delivery and improved efficacy in the treatment of bacterial uterine infections.
Introduction
According to the Centers for Disease Control and Prevention (CDC), more than 1 million women in the USA experience acute pelvic inflammatory disease (PID), with US$1–2 billion spent annually for treatment of the disease and its sequelae.Citation1–Citation6 The CDC recommends 200 mg of doxycycline, an inexpensive broad-spectrum drug, to be administered for treatment orally or intravenously every 12 hours.Citation7 Doxycycline is used to treat both intracellular and extracellular bacterial infections such as aerobic and anaerobic Gram-positive and Gram-negative bacteria, and other microorganisms such as chlamydia, protozoa, mycoplasma, mycobacteria, and spirochetes.Citation8,Citation9 Due to doxycycline’s antibacterial effects on a wide range of pathogens, it is currently one of the most commonly prescribed antibiotics worldwide for treating infectious diseases.Citation9,Citation10 However, when doxycycline is administered orally or intravenously, it may cause esophageal ulcers, gastrointestinal irritation, and local inflammation, which may in turn lead to premature cessation of treatment.Citation11–Citation13 Furthermore, the use of doxycycline may also result in mechanical scarring of tissues and cavities in the body as well as blood vessels.Citation14–Citation20
In recent years, drug encapsulation and delivery via small particles has garnered increasing interest. Encapsulation may help prevent adverse effects by protecting sensitive tissues from fast drug exposure while also improving drug efficacy by achieving slow, sustained release directly at the infection site. Having patients complete the entire treatment cycle would also increase the likelihood of complete pathogen elimination. These properties suggest that the encapsulation of doxycycline into biodegradable nanoparticles could perhaps be used to eventually improve treatment of PID via direct transcervical drug delivery.
Chitosan is a promising candidate for encapsulating and delivering doxycycline or other drugs directly to an infection site. This naturally occurring cationic polysaccharide possesses muco-adhesive properties that enable its transport across the mucosal membrane.Citation21–Citation26 In addition, it slowly degrades to nontoxic amino sugars that can be completely absorbed by the body. These properties – biocompatibility and biodegradability – are highly desirable for encapsulation materials.Citation26–Citation28 Particle size and co-occurring substances are other important considerations. Encapsulation of doxycycline into chitosan microspheres has been previously accomplished using a water-in-oil emulsion technique, but this approach can introduce oil and other harsh chemicals to the body.Citation29 Chitosan particles prepared by an alternative method may offer a more benign delivery vehicle. Chitosan can also be used to form nanoparticles, which have a higher cellular uptake than microparticles, thus allowing for greater intracellular delivery of the encapsulated (drug) molecule.Citation30–Citation32
Encapsulation methods are chosen in part based on polymer properties, drug hydrophobicity, and desired final particle size. The molecular weight of the chitosan plays a vital role in particle size and formation, as a higher molecular weight produces larger particles.Citation33,Citation34 An ideal method should also ensure drug encapsulation while minimizing drug loss and maintaining pharmacological activity. Commonly used methods for preparing chitosan-based drug delivery systems include emulsion crosslinking,Citation35,Citation36 emulsion-droplet coalescence,Citation28,Citation35 spray drying,Citation37 sieving,Citation35 coacervation/precipitation,Citation29,Citation35,Citation36 and ionic gelation.Citation31,Citation34,Citation36,Citation38,Citation39 Methods such as emulsion crosslinking and emulsion-droplet coalescence involve the use of a harsh crosslinking agent that might induce an unnecessary chemical reaction with the active agents. Spray drying and sieving produce relatively large microparticles, with diameters of approximately 1–10 μm and 543–698 μm, respectively.Citation35 Ionic gelation methods, in contrast, offers the advantage of simplicity with no requirement for complicated equipment as well as electrostatic crosslinking instead of chemical crosslinking, thereby reducing the likelihood of inducing toxic effects with the particles.
In this study, we investigated chitosan nanoparticles as a potential carrier of doxycycline to improve drug delivery and treatment efficacy. The goal was to undertake an initial assessment of particle properties relevant to encapsulated drug delivery through a localized (ie, transcervical) route using a patent-pending device design fabricated by our group. The chosen method for our preparation was ionic gelation. We created and then characterized doxycycline-loaded chitosan nanoparticles (DCNPs) in terms of their morphology (size and shape), drug encapsulation efficiency and release rates, in vitro antibacterial activity, and in vitro cytotoxicity.
Materials and methods
Materials
Doxycycline, phosphate-buffered saline, Millipore water-soluble tetrazolium salts (WST)-1 Cell Proliferation Assay, and acetic acid were obtained from Fisher Scientific (Hampton, NH). Sodium tripolyphosphate (TPP), fetal bovine serum, medium 199, and MCDB 105 medium were supplied by Sigma Chemical Company (St Louis, MO). Partially (75%) deacetylated chitosan (60 kDa) derived from shrimp shells was obtained in powder form from Sigma-Aldrich (St Louis, MO). All other chemicals were of analytical grade and were obtained from a variety of vendors. Eschericha coli (ATCC 25922) was purchased from American Type Culture Collection (Manassas, VA).
Preparation of nanoparticles
The chitosan nanoparticles were prepared using the ionic gelation method described by Clavo et al.Citation38 Chitosan powder was dissolved, 0.2% weight by volume (w/v), in 0.25M acetic acid; this solution was magnetically stirred overnight at a speed of 400 rpm at room temperature. The acetic acid protonates the amine group of the chitosan molecule for a more stable interaction with the crosslinking agent and the drug.Citation33,Citation40 The crosslinker, TPP, was prepared by dissolving the powder in 0.25 M acetic acid at two different concentrations: 0.42% w/v (referred to here as formulation 8 [F8]) and 0.60% w/v (formulation 10 [F10]). In a separate set of experiments, we examined 64 different combinations of solutions and procedural steps and identified these two formulations as consistently producing particles within a predictable formulation-specific size range.
Blank nanoparticles were formed by combining the chitosan and TPP solutions for a total volume of 2 mL; the chitosan-to-TPP ratio was 23:1 for F8 and 16:1 for F10. To initiate ionic gelation (nanoparticle formation), TPP was added dropwise to the stirred chitosan solution and the combined solution was then stirred for an additional hour. Blank nanoparticles prepared using F8 (0.42% w/v TPP) are here referred to as BKCNP4, while blanks prepared using F10 (0.60% w/v TPP) are referred to as BKCNP6. The solution with precipitated nanoparticles was centrifuged at 10,000 rpm for 5 minutes and the resulting supernatant was saved for later analysis of its doxycycline content. The particles in the micro-centrifuge tube were washed/resuspended by adding 2 mL nanopure water and the tube was again centrifuged at 10,000 rpm for 5 minutes. The second supernatant was removed and discarded as preliminary tests had shown that the second supernatant contained no doxycycline residue. Finally, the particles were resuspended in 2 mL nanopure water before further analysis.
A doxycycline stock solution was prepared by dissolving doxycycline powder in nanopure water to achieve a final concentration of 200 mg doxycycline per mL solution.
Drug-loaded chitosan nanoparticles were then prepared according to the procedure outlined above, except that 100 μL of the doxycycline stock solution was added dropwise to the stirred chitosan solution just before the TPP addition. In every batch of DCNP solution, the final doxycycline concentration was 20 mg/mL. Drug-loaded chitosan nanoparticles prepared using 0.42% w/v TPP are here referred to as DCNP4; those prepared with 0.60% w/v TPP are referred to as DCNP6. All analyses of blank particles, drug-loaded particles, and supernatant were initiated within 24 hours of particle preparation.
Nanoparticle characterization
Particle size distributions for blank nanoparticles and DCNPs were determined with a Nanotrac Particle Size Analyzer (Microtrac, Largo, FL), which measures dynamic light scattering by particles in solution. The analyses were performed on samples of nanoparticles suspended in 1 mL of nanopure water. The shapes of the blank particles and DCNPs were examined using a JEM-1400 (Jeol, Tokyo, Japan) transmission electron microscope (TEM). Particles to be used for TEM examination were first dried under vacuum and stored in the dark at 4°C. TEM samples were then prepared by depositing a drop of nanoparticles onto a formvar-coated copper grid, which was allowed to dry by vacuum before TEM analysis.
Encapsulation efficiency
Incorporation of doxycycline into the particles was characterized by measuring the doxycycline contained in the centrifugation supernatant. Since the total amount of drug in each formulation batch was known (2 mL solution with a doxycycline concentration of 20 mg/mL), any doxycycline not found in the supernatant could be assigned to the particles. Doxycycline in the supernatant was quantified using a NanoDrop spectrophotometer (ND-1000; NanoDrop, Wilmington, DE). According to the Beer–Lambert equation, the doxycycline concentration, c, is given by c = A/ɛL, where A is light absorbance at 220 nm wavelength, ɛ is the molar absorptivity coefficient (121.39 M−1 cm−1), and L is the path length (0.01 cm). All measurements were performed in triplicate (n = 3). The encapsulation efficiency (EE, %) was calculated using Equationequation 1(1) .
Assessment of drug release
To determine the rate at which doxycycline was released by the nanoparticles, the particles were resuspended in a drug-free solution that was analyzed for doxycycline content at a predetermined time interval. To begin, dried fresh nanoparticles of known antibiotic content were first resuspended in 2 mL of nanopure water. A small aliquot of this particle-laden solution was then added to phosphate-buffered saline–ethanol solution to produce a final volume of 2 mL with an initial concentration of 100 μg doxycycline per mL. This solution was incubated at 37°C under gentle agitation. At each specified time point (0.5, 1, 2, 3, 4, 5, 6, 7, 8, 16, and 24 hours) thereafter, the sample was centrifuged and the supernatant was isolated and analyzed by Nano-drop spectrophotometry to determine the amount of doxycycline in solution. All measurements were performed in triplicate (n = 3) for each formulation. The percentage of drug released at each time point was calculated according to Equationequation 2(2) .
Assessment of antibacterial activity
To determine the antibacterial activity of the DCNPs, minimum inhibitory concentrations (MICs) and minimum bactericidal concentrations (MBCs) were evaluated. The procedures for both assays were adopted from Lee et al.Citation41 MIC is the lowest concentration of DCNPs that inhibits bacterial growth. For our analyses, a visual turbidimetric method was used. Freshly prepared nanoparticles, blank and drug-loaded, were ultraviolet-sterilized for 10 minutes. The particles were then resuspended in a volume of sterile water sufficient to achieve a final doxycycline concentration of 100 μg/mL. A 500 μL aliquot of this solution with sterilized particles was added to a tube containing Luria-Bertani broth for a total volume of 2 mL. A serial dilution, with a dilution factor of 0.3, was performed for the remaining six tubes. A parallel series of experiments was also run using unencapsulated doxycycline.
Under sterile conditions, the tubes containing particles were inoculated with 1.0 × 105 colony-forming units/mL of E. coli cells in Luria-Bertani broth then incubated at 37°C under agitation for 4 hours. Following the incubation, the tubes were assessed visually for the appearance of turbidity (ie, bacterial growth). Among the tubes that showed no visual turbidity – that is, complete inhibition of visible E. coli growth – the one with the lowest doxycycline concentration was identified as the MIC tube for that series. All MIC tubes were analyzed to assess the amount of bacteria present by measuring optical density of the suspension at 600 nm (OD600) and then calculating the number of bacterial cells present.
The MBC is the minimum concentration of DCNPs that will kill 99% of the bacterial cells initially present. To determine this value, 100 μL aliquots of liquid culture (broth + nanoparticles + bacteria) from each series’ MIC tube and the two tubes prior (ie, containing slightly more doxycycline) were plated and incubated at 37°C overnight. As a positive control, an additional plate was plated with broth plus E. coli (no particles or drug); as a negative control, another plate was plated with broth plus blank particles plus E. coli (no drug). All samples were plated in triplicate. Plates were observed for colony growth, and the plate with the fewest colony colonies was identified as the MBC plate.
Assessment of cytotoxicity
Cytotoxicity of the DCNPs was determined by treating normal human ovarian surface epithelial (OSE) cells with different concentrations of doxycycline-loaded nanoparticles, then monitoring cell viability over the next 5 days. The OSE cells were cultured for 4 to 7 days in flasks containing medium 199/MCDB105 media supplemented with 10% fetal bovine serum. The cells were removed from the flasks and counted, and then 100 μL of medium containing cells was added to the wells of a 96-well plate to give final cell concentrations of 5 × 102, 1.0 × 103, or 2.0 × 103 cells/mL. After 24 hours incubation at 37°C, the cells were treated with blank nanoparticles, DCNP4 (1 and 2 μg/mL), DCNP6 (1 and 2 μg/mL), or unencapsulated doxycycline (1 and 2 μg/mL). The plate was then incubated at 37°C. Bright microscopy was used to examine the cell morphology after treatment just before the assessing for cytotoxicity. Cytotoxicity was assessed on days 1, 3, and 5 by WST-1 assay. WST-1 assay reagent (10 μL) was added to each well, followed by incubation for another 4 hours at 37°C and then spectrophotometric assessment of cell viability. Mitochondrial dehydrogenases produced by viable cells reduce the WST-1 reagent to form formazan dye in an amount directly proportional to the number of metabolically active cells in the well. This dye was quantified using a BioTek Synergy (Winooski, VT) multiplate reader to measure absorbance at 450 nm (reference wavelength was 630 nm). All treatments were assayed in triplicate (n = 3).
Statistical analysis
A two-way analysis of variance was employed to identify statistical differences among the various experimental groups and their corresponding control groups. Experimental groups with P-values of P < 0.05 were considered to be statistically significant.
Results and discussion
Physical characterization of drug-loaded nanoparticles
Blank and DCNPs were successfully prepared using an ionic gelation method. The blank particles, BKCNP4 and BKCNP6, were relatively large, with an average diameter of 4900 and 4450 nm, respectively (data not shown). Both groups of particles were spherical in shape with a narrow particle size distribution. The drug-loaded particles were smaller than their blank counterparts. The DCNP4 particles (), with an average particle diameter of 44.50 nm, were two orders of magnitude smaller than their corresponding blanks and had a wider particle size distribution profile. DCNP4s were spherical in shape with smooth edges (). Similarly, the DCNP6 particles (), with an average diameter of 280 nm, were small compared to their blank counterparts – about 1/15 the size – but were significantly larger than the DCNP4s. In contrast to the DCNP4s, the DCNP6 particles exhibited a very narrow particle size distribution profile. The DCNP6 particles were spherical like the DCNP4s but had edges that were not as smooth ().
Figure 1 Particle size distribution (A and C) for DCNP4 and DPNC6, and transmission electron microscopy images (B and D), for DCNP4 and DPNC6 respectively.
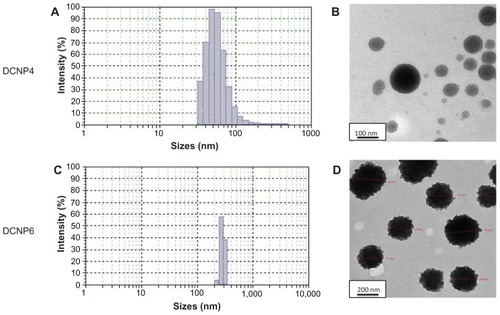
Since the conditions that varied between the two formulations for preparing these particles was the concentration of the TPP crosslinker and the resulting ratio of chitosan to TPP, it can be speculated that the concentration of the crosslinker plays a role in determining particle size. Also, inclusion of the doxycycline in the formulations produced particles significantly smaller than the blanks, which is an interesting finding that our group is currently investigating. Having a narrower particle size distribution profile – ie, particles of more nearly uniform size – is also important. The more similar the particles are in size, the more equally the drug will be distributed among the particles, which will normalize the rate of drug release.
Encapsulation efficiency
Across all batches of DCNP4, the lowest encapsulation efficiency was 22%, the highest was 69%, and the average was 53% ± 19%. For DCNP6, the lowest encapsulation efficiency was 41%, the highest was 68%, and the average was 56% ± 10%. Even though DCNP6 was substantially larger in diameter, there was no significant difference in the amount of drug encapsulated for each type of DCNP.
Drug release
Doxycycline was released from the DCNPs in a burst-effect manner followed by a slow, sustained release (). For DCNP4, the burst effect occurred within the first 5 hours; for DCNP6, within the first 4 hours. Within the first couple of hours after this initial burst, the amount of drug released decreased then was followed by a sustained release for the remaining time. By the end of the 24-hour monitoring period, DCNP6 had released more total drug than had DCNP4. The difference between the amounts of drug released by the two different particle formulations can be attributed to the differences in particle size, with the larger particles (DCNP6) releasing more antibiotic than the smaller ones (DCNP4). A burst effect followed by slow sustained release, as demonstrated by both nanoparticle formulations, is ideal for treating microbial infections such as PID, where delivering the particles in a local (ie, transcervical) manner to the reproductive lumen would provide an increasing amount of doxycycline initially, followed by a reduced amount later, thus increasing the likelihood of total pathogen elimination.
Figure 2 The amount of doxycycline release over a 24-hour period for DCNP4 and DCNP6 both with an initial burst effect within the first 4 to 5 hours followed by a decrease in amount of drug being release then to a slow sustained amount for the remaining hours.
Note: Data shown are the mean ± standard deviation (n = 3).

Antibacterial activity
After 4 hours incubation at 37°C, the drug-loaded nanoparticles’ minimum inhibitory doxycycline concentration, MIC, was 16 μg/mL for DCNP4 and 13 μg/mL for DCNP6. shows the MIC cases for DCNP4 (16 μg/mL) and DCNP6 (13 μg/mL) with unencapsulated doxycycline (Doxy 13 μg/mL) and blank particles serving as controls. For both types of DCNPs, more than 92% E. coli growth inhibition was observed. The minimum bactericidal concentration, MBC, was 48 μg/mL and 40 μg/mL for DCNP4 and DCNP6, respectively. Unencapsulated doxycycline treatments (Doxy), conducted at the same concentrations as the DCNP drug concentrations, resulted in the near-elimination of E. coli. These data suggest that the unencapsulated doxycycline had a higher antibacterial activity than the DCNPs within the 4-hour period. However, because the DCNPs release the doxycycline in a slow and sustained manner, we speculate that the nanoparticles’ antibacterial activity would have been higher if the incubation period had been extended beyond 4 hours.
Figure 3 Inhibitory effects of drug-loaded chitosan nanoparticles on bacterial growth, expressed in terms of percentage of remaining bacteria after 4 hours of treatment. Minimum inhibitory concentration values are as follows: DCNP4 = 16 μg/mL, DCNP6 = 13 μg/mL, and Doxy = 13 μg/mL. The untreated tube was used to define the “100% remaining” (no inhibition) case.
Note: Data shown are the mean ± standard deviation (n = 3).

Cytotoxicity
For the case of initial human OSE cell densities of 5 × 102, no cytotoxicity (relative to the cells-only case) was induced by the 1 μg/mL or 2 μg/mL dosages of either DCNP formulation – in other words, cells treated with the DCNPs for 5 days showed high cell viability (). In fact, a significant increase in cell proliferation relative to the cells-only case was often observed when the OSE cells were treated with blank or doxycycline-loaded nanoparticles. In contrast, unencapsulated doxycycline at the same dosages induced severe cell toxicity: only 39% of the original population remained viable after the 5-day treatment. Cells treated with both dosages of DCNP4 or with BKCNP4 had a higher viability than did untreated cells. For DCNP6, the viability of cells treated with 1 μg/mL was higher than for untreated cells, but cells treated with 2 μg/mL showed lower viability. Nevertheless, the DCNP6 case still showed greater cell viability than did the unencapsulated drug treatments. Data from the wells with 1.0 × 103 and 2.0 × 103 initial cell densities are not presented because of cell overcrowding over the 5-day period.
Figure 4 Five-day cell viability for human ovarian surface epithelial cells exposed to blank nanoparticles, drug-loaded nanoparticles, and unencapsulated doxycycline. A higher formazan absorbance indicates greater cell viability.
Note: Data shown are the mean ± standard deviation (n = 3).

These cytotoxicity results show that encapsulation of doxycycline into the chitosan polymer reduces the toxicity that is normally induced by the unencapsulated drug. The differences between the DCNP4 and DCNP6 cytotoxicity results are possibly due to the differences in their size and the amount of drug released, where the DCNP6s were observed to release more doxycycline than did the DCNP4s. Further exploration and evaluation of the effects of chitosan particles on cell growth is necessary to explain the observed increase in proliferation.
Doxycycline-induced cytotoxicity was confirmed visually by observations of cell morphology following exposure to doxycycline. Cells treated with 1 μg/mL and 2 μg/mL dosages of doxycycline show the morphology of dead cells. The morphological effects of treating with DCNP4 (at 2 μg/mL) and unencapsulated doxycycline (also at 2 μg/mL) are illustrated in . Note that the cells treated with the DCNPs were of the same morphology as the untreated cells further demonstrating that encapsulation of doxycycline into chitosan nanoparticles minimizes adverse effects of the drug.
Conclusion
DCNPs were synthesized and characterized to explore properties that may improve drug delivery and efficacy. Two types of nanoparticles were formulated with differing concentrations of crosslinker: DCNP4 and DCNP6, with the DCNP6 formulation containing approximately 1.5 times more TPP crosslinker. Particle diameters ranged from 30 to 220 nm (DCNP4) and from 200 to 320 nm (DCNP6); average diameters were 44.50 nm (DCNP4) and 280 nm (DCNP6). Both types of drug-loaded chitosan nanoparticles were spherical, with encapsulation efficiencies of approximately 50%. For both formulations, encapsulated doxycycline was released slowly into solution from the particles, with an initial burst effect followed by sustained release over a 24-hour period. Total in vitro release of doxycycline over 24 hours was 10% higher for the larger DCNP6 particles than for DCNP4. Both formulations inhibited the growth of E. coli after 4 hours of incubation. MBC values were <50 μg/mL. The DCNPs induced significantly less apparent cytotoxicity than did the unencapsulated doxycycline, which showed significantly high cytotoxicity against normal OSE cells. These results demonstrate that encapsulation of doxycycline into chitosan nanoparticles minimizes adverse side effects of the drug while also effectively releasing the antibiotic in a slow and sustained manner. Therefore, we have demonstrated that DCNPs have the potential of treating E. coli, a common copathogen in PID, in a slow sustained manner while reducing the cellular toxicity to non-bacterial cells that is normal when doxycycline is used in its unencapsulated form. Our future work is to test these DCNPs in an adequate biofilm model that closely mimics the biofilm of the uterus.
Acknowledgments
This material is based upon work supported by the University South Florida (USF) College of Engineering Interdisciplinary Scholarship Program. We would like to thank the Florida Education Fund McKnight Doctoral Fellowship and the National GEM Consortium PhD Fellowship for their financial support to Natasha Cover. We would also like to thank Dr Don Cameron, Dr Peter Medveckzy, Shara Pantry, and Yvonne Davis from the USF College of Medicine and Regina Easley from the USF College of Marine Science for their help and suggestions.
Disclosure
The authors report no conflicts of interest in this work.
References
- CrossmanSHThe challenge of pelvic inflammatory diseaseAm Fam Physician200673585986416529095
- HaggertyCLNessRNewest approaches to treatment of pelvic inflammatory disease: a review of recent randomized clinical trialsClin Infect Dis200744795396017342647
- WalkerCKWiesenfeldHCAntibiotic therapy for acute pelvic inflammatory disease: the 2006 Centers for Disease Control and Prevention sexually transmitted diseases treatment guidelinesClin Infect Dis200744Suppl 3S111S12217342664
- SweetRRole of bacterial vaginosis in pelvic inflammatory diseaseClin Infect Dis1995202271275
- PetersonHBGalaidEIZenilmanJMPelvic inflammatory disease: review of treatment optionsRev Infect Dis1990126656664
- ReinDBKasslerWJIrwinKLRabieeLDirect cost of pelvic inflammatory disease and its sequelae: decreasing but still substantialObstet Gynecol200095339740210711551
- Centers for Disease Control and PreventionSexually transmitted diseases treatment guidelinesMMWR201059RR–121109
- RiondJRiviereJPharmacology and toxicology of doxycyclineVet Hum Toxicol19883054314433055652
- JoshiNMillerDQDoxycycline revisitedArch Intern Med199715713142114289224219
- CunhaBADomenicoPCunhaCBPharmacodymanics of doxycyclineClin Microbiol Infect20016527027311168126
- GencosmanogluRKurtkaya-YapicierOTiftikciAAvsarETozunNOranESMid-esophageal ulceration and candidiasis-associated distal esophagitis as two distinct clinical patterns of tetracycline or doxycycline-induced esophageal injuryJ Clin Gastroenterol200438648448915220682
- MorrisTJDavisTPDoxycycline-induced esophageal ulceration in the US Military serviceMil Med2000165431631910803009
- TahanVSayrakHBayarNErerBTahanGDaneFDoxycycline-induced ulceration mimicking esophageal cancerCases J20081114418778470
- HeffnerJEStanderferRJTorstveitJUnruhLClinical efficacy of doxycycline for pleurodesisChest19941056174317478205870
- ManssonTTreatment of malignant pleural effusion with doxycyclineScand J Infect Dis Suppl19885329343166542
- RobinsonLAFlemingWHGalgraithTAIntrapleural doxycycline control of malignant pleural effusionsAnn Thorac Surg1993555111511218494419
- HurewitzANLidonicciKWuCLZuckerSHistologic changes of doxycycline pleurodesis in rabbits. Effect of concentration and pHChest19941064124112457924503
- PulsiripunyaCYoungchaiyudPPushpakomRMaranetraNNanaACharoenratanakulSThe efficacy of doxycycline as a pleural sclerosing agent in malignant pleural effusion: a prospective studyRespisology1996116972
- VenugopalPMedical pluerodesisPulmon2007925156
- DaviesRPMcClymontTBoshellDUtlrasound guided chemical pluerodesis with doxycyclineDiagn Ultrasound2005832327
- ZhangHWuSTaoYZangLSuZPreparation and characterization of water-soluble chitosan nanoparticles as protein delivery systemJ Nanomater2009201015
- MuhammedRJuniseVSaraswathiPKrishnanPDilipCDevelopment and characterization of chitosan nanoparticles loaded with isoniazid for the treatment of tuberculosisRes J Pharm Biol Chem Sci201014383390
- PhaechamudTCharoenteeraboonJAntibacterial activity and drug release of the chitosan sponge containing hyclateAAPS Pharm Sci Tech200893829835
- KoJAParkHJHwangSJParkJBLeeJSPreparation and characterization of chitosan microparticles intended for controlled drug deliveryInt J Pharm20022491–216517412433445
- Xi-PengGDa-PingQKai-RongLTaoWPengXMaiKCPreparation and characterization of cationic chitosan-modified poly(D,L-lactide-co-glycolide) copolymer nanospheres as DNA carriersJ Biomater Appl200822435337117494965
- SarmentoBRibeiroAVeigaFFerreiraDDevelopment and characterization of new insulin containing polysaccharide nanoparticleColloids Surf B Biointerfaces200653219320217049214
- GoycooleaFMLolloGRemunan-LopezGQuagliaFAlonsoMJChitosan-alginate blended nanoparticles as car riers for the transmucosal delivery of macromoleculesBiomacromolecules20091071736174319545119
- TokumitsuHIchikawaHFukumoriYChitosan-gadopentetic acid complex nanoparticles for gadolinium neutron-capture therapy of cancer: preparation by novel emulsion-droplet coalescence technique and characterizationPharm Res199916121830183510644070
- ShanmuganathanSShanumugasundaramNAdhirajanNRamyaa-LakshmiTSBabuMPreparation and characterization of chitosan microsphere for doxycycline deliveryCarbohydrate Polymers2007732201211
- MohanrajVJChenYNanoparticle: a reviewTrop J Pharm Res200651561573
- Pinto ReisCNeufeldRJRibeiroAJVeigaFNanoencapsulationIMethods for preparation of drug-loaded polymeric nanoparticlesNanomedicine20062182117292111
- WangJJZengZWXiaoRZRecent advances of chitosan nanoparticles as drug carriersInt J Nanomedicine2011676577421589644
- RacovitaSVasiliuSPopaMLucaCPolysaccharides based on micro-and nanoparticles obtained by ionic gelation and their application as drug delivery systemsRevue Roumaine de Chimie2009549709718
- TiyaboonchaiWChitosan nanoparticles: a promising system for drug deliveryNaresuan Uni J20031135166
- AgnihotriSAMallikarjunaNNAminabhaviTMRecent advances on chitosan-based micro- and nanoparticles in drug deliveryJ Control Release2004100152815491807
- NagpaKKumar-SinghSNath-MishrDChitosan nanoparticles: a promising system in novel drug deliveryChem Pharm Bull201058111423143021048331
- GrenhaASeijoBSerraCRemunan-LopezCChitosan nanoparticle-loaded mannitol microspheres: structure and surface characterizationBiomacromolecules2007872072207917585804
- ClavoPVila-JatoJAlonsoMJEvalution of catonic polymer coated nanocapsules as ocular drug carriersJ Pharma199715314150
- MehrotraANagarwalRCKumar-PanditJLomustine loaded chitosan nanoparticles: characterization and in-vitro cytotoxicity on human lung cancer cell line L13Chem Pharm Bull201159331532021372411
- AlamehMJeanMDeJesusDBuschmannMDMerzoukAChitosanase- based method for RNA isolation from cells transfected with chitosan/siRNA nanocomplexes for real-time RT-PCR in gene silencingInt J Nanomedicine2010547348120957169
- LeeYSJangKAChaJDSynergistic antibacterial effect between silibinin and antibiotics in oral bacteriaJ Biomed Biotechnol2012 article ID 618081