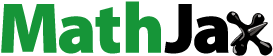
Abstract
Background
The purpose of this study was to develop novel sandwich-structured nanofibrous membranes to provide sustained-release delivery of vancomycin, gentamicin, and lidocaine for repair of infected wounds.
Methods
To prepare the biodegradable membranes, poly(D, L)-lactide-co-glycolide (PLGA), collagen, and various pharmaceuticals, including vancomycin, gentamicin, and lidocaine, were first dissolved in 1,1,1,3,3,3-hexafluoro-2-propanol. They were electrospun into sandwich-structured membranes with PLGA/collagen as the surface layers and PLGA/drugs as the core. An elution method and a high-pressure liquid chromatography assay were used to characterize in vivo and in vitro drug release from the membranes. In addition, repair of infected wounds in rats was studied. Histological examination of epithelialization and granulation at the wound site was also performed.
Results
The biodegradable nanofibrous membranes released large amounts of vancomycin and gentamicin (well above the minimum inhibition concentration) and lidocaine in vivo for more than 3 weeks. A bacterial inhibition test was carried out to determine the relative activity of the antibiotics released. The bioactivity ranged from 40% to 100%. The nanofibrous membranes were functionally active in treating infected wounds, and were very effective as accelerators in early-stage wound healing.
Conclusion
Using the electrospinning technique, we will be able to manufacture biodegradable, biomimetic, nanofibrous, extracellular membranes for long-term delivery of various drugs.
Introduction
Prompt closure of full thickness wounds is critical to survival after severe burns injuries. The risk of infection is of great concern with deep burn wounds, and is likely due to the combination of impaired local immunity with the presence of dead tissue and impaired systemic immunity with such extensive injury.Citation1,Citation2 Development of a wound infection depends on a complex interplay of many factors. If the integrity and protective function of the skin is breached, large quantities of different cell types will enter the wound and initiate an inflammatory response. This is typically characterized by the classic signs of redness, pain, swelling, raised temperature, and fever. Treatment measures for an infected wound include administration of antibiotics, removing necrotic tissue, and cleansing wounds to minimize colonization. Wound dressing is essential for the prevention of infection. An ideal candidate for dressing of infectious wounds should deliver sufficiently high concentration of antibiotics to the wound site; mimic the structure and biological function of native extracellular matrix proteins, which provide support and regulate cellular activities; maintain the normal state of differentiation within the cellular compartment; be biocompatible and/or biodegradable; and have no adverse effects on the surrounding tissue.
Commercial wound dressings that exert their antimicrobial effect by eluting germicidal compounds have been developed to provide sustained release of therapeutic doses of silver ions to the wound. However, silver ions are highly toxic to keratinocytes and fibroblasts, and may delay wound repair if applied indiscriminately to healing tissue areas. In addition, constant wound cleaning and redressing is needed, causing discomfort to patients and requiring substantial nursing input. A biodegradable drug-eluting wound dressing from an electrospun nanofibrous matrix potentially offers several advantages over conventional ones.Citation3–Citation5
Various efforts have been made to develop drug-eluting dressings. Mi et alCitation6 proposed a bilayered chitosan membrane, consisting of a dense skin layer and a sponge-like sublayer for topical delivery of silver sulfadiazine. Peng et alCitation7 developed paracetamol-loaded poly(D,L-lactide) and poly(ethylene glycol)-co-poly(D,L, lactide) fibers that provide sustained drug release in vitro. Elsner and Zilberman and Elsner et alCitation8,Citation9 fabricated antibiotic-eluting composite fibers that consisted of a polyglyconate core and a porous poly(D,L-lactic-co-glycolic acid) (PLGA) shell as basic dressing elements that use freeze-drying of invert emulsions. Teo et alCitation10 developed a three-dimensional polycaprolactone-tricalcium phosphate mesh for delivery of gentamicin sulfate to infected wounds. Meng et alCitation11,Citation12 prepared PLGA/gelatin and PLGA/chitosan nanofibers for delivery of fenbufen via the electrospinning process. Recently, Kontogiannopoulos et alCitation13 prepared electrospun fiber mats containing shikonin and derivatives, using four biocompatible polymers, including cellulose acetate, poly(L-lactide), and 50/50 and 75/25 LA/GA poly(lacide-co-glycolide). Sandwich-structured drug-eluting dressings made of biodegradable nanofibers, with collagen as the surface layers and drugs (antibiotics and anesthetics) at the core, can provide advantages over single-layer dressings. In addition to the delivery of a sustainable antimicrobial concentration to the wound site, the surface layer of electrospun sandwich-structured collagen nanofibers, with its large area and microporous structure, is able to promote wound healing.Citation14–Citation19 Further, the nanofibrous membrane is also important for cell attachment and proliferation in wound healing.Citation20 Nevertheless, there has not been any previous development of sandwich-structured membranes for the delivery of pharmaceuticals.
This study developed biodegradable, sandwich-structured, nanofibrous, drug-eluting membranes (DEM) via electrospinningCitation21 for dressing of infected wounds. In addition to carrying pharmaceuticals, PLGA is nontoxic, elicits a minimal inflammatory response, and can be eventually absorbed without any accumulation in the vital organs.Citation22,Citation23 Collagen is a principal structural component of the extracellular matrix. After electrospinning, the morphology of the electrospun nanofibers was characterized by scanning electron microscopy. The in vitro and in vivo release characteristics of the pharmaceuticals from the membranes was investigated. A bacterial inhibition test was carried out to determine the relative activity of the antibiotics released. In addition, the effect of nanofibrous DEM on repair of infected wounds in rats was examined. Histological examination of epithelialization and granulation at the wound site was also performed.
Materials and methods
Fabrication of nanofibrous membranes
The PLGA used in this study was a commercially available material (Resomer RG 503, Boehringer, Ingelheim, Germany) with a lactide:glycolide ratio of 50:50 and an intrinsic viscosity of 0.4. Type I collagen from bovine Achilles tendon and 1,1,1,3,3,3-hexafluoro-2-propanol (HFIP) were purchased from Sigma-Aldrich (Saint Louis, MO). The drugs used were vancomycin hydrochloride, gentamicin sulfate, and lidocaine hydrochloride (Sigma-Aldrich), all of which were of commercial grade.
The electrospinning apparatus used in this study consisted of a syringe and needle (internal diameter 0.42 mm), a ground electrode, an aluminum sheet, and a high voltage power supply,Citation20 as shown schematically in . The needle was connected to the high voltage supply, which generated positive DC voltages and currents up to 35 kV and 4.16 mA/125 W, respectively. To prepare the membranes, PLGA and collagen (280 mg and 140 mg, respectively) were first dissolved in 1 mL of HFIP, while the PLGA, vancomycin, gentamicin, and lidocaine (960, 140, 140, and 140 mg, respectively) were dissolved in 4 mL of HFIP.
Two types of membranes were prepared, ie, drug-eluting membranes (DEM) and non-drug-eluting membranes (NDEM). For electrospinning of DEM, 1 mL of PLGA/ collagen solution was delivered and electrospun using a syringe pump with a volumetric flow rate of 3.6 mL/hour for the surface layer, followed by electrospinning of 4 mL PLGA/ vancomycin/gentamicin/lidocaine solution with a volumetric flow rate of 1.2 mL/hour for the core layer, and finally by spinning of another 1 mL of PLGA/collagen solution for the second surface layer. Sandwich-structured membranes, with PLGA/collagen as the surface layer and PLGA/drugs at the core, with a thickness of 0.107 mm, were thus obtained. For the preparation of NDEM, 5 mL of PLGA/collagen solution was delivered and electrospun using a syringe pump, with a volumetric flow rate of 3.6 mL/hour, to obtain a membrane 0.110 mm in thickness. The distance between the needle tip and the ground electrode was 12 cm, and the positive voltage applied to the polymer solutions was 17 kV. All electrospinning experiments were carried out at room temperature.
Mechanical properties
The tensile strengths of the PLGA/collagen membranes fabricated were determined. The dumbbell-shaped tensile specimens with gauge lengths of 20 mm and cross-sectional areas of 10 mm × 0.4 mm (width by thickness) were obtained from the nanofibrous membranes using a knife. Tensile tests were performed using a Lloyd tensiometer, along with the ASTM D638 test. A 100 N load cell was used and the crosshead speed was 90 mm/minute.
Standard curve
A high-performance liquid chromatography (HPLC) assay was used to determine standard drug concentration curves. The HPLC analyses were conducted on a Hitachi L-2200 multisolvent delivery system. A Symmetry C8, 3.9 cm × 150 mm HPLC column (Waters, Milford, MA) was used for separation of vancomycin. The mobile phase contained 0.01 mol heptanesulfonic acid (Fisher Scientific UK Ltd, Loughborough, UK) and acetonitrile (85/15, v/v, Mallinckrodt, St Louis, MO). Absorbency was monitored at a wavelength of 280 nm, and the flow rate was 1.4 mL/minute. All experiments were carried out in triplicate and the sample dilutions were performed to bring the unknown concentrations into the range of the standard assay curve. A calibration curve was made for each set of measurements (correlation coefficient > 0.99). The elution product could be specifically identified and quantified with high sensitivity using the HPLC system. Concentrations of eluted gentamicin and lidocaine were also determined using the same HPLC assay.
In vitro release of pharmaceuticals
The release characteristics of vancomycin, gentamicin, and lidocaine from the nanofibrous membrane were determined using the in vitro elution method. Samples with an area of 2 cm × 3 cm, cut from the electrospun membranes, were placed in glass test tubes (one sample per test tube, total number = 3) with 1 mL of phosphate-buffered solution (0.15 mol/L, pH 7.4) in each. The glass test tubes were incubated at 37°C for 24 hours, after which the eluent was collected and analyzed. Fresh phosphate-buffered solution (1 mL) was added for the following 24-hour period, and the procedure was repeated for 30 days. Drug concentrations in the eluents were determined using the standard HPLC assay curve.
Healing of wound infection test
Twelve Sprague-Dawley rats weighing 375 ± 25 g were used in this study. After induction of anesthesia, two full-thickness 2 cm × 2 cm rectangular wounds were cut into each rat’s back, parallel with the vertebral column. All animal procedures received institutional approval, and all animals used in the study were cared for in accordance with the regulations of the National Institute of Health of the Republic of China, Taiwan, under the supervision of a licensed veterinarian. Following the work of Grzybowski et al,Citation24 standard strains of Staphylococcus aureus (ATCC 65389) and Escherichia coli (ATCC 23815), provided by the Bioresource Collection and Research Center of Taiwan, were used in this study. A 10 μL sample of bacteria inoculum was cultured in 5 mL of nutrient broth and grown overnight at 37°C with constant shaking at 200 rpm. The concentration of bacterial suspension was adjusted using ultraviolet-visible spectrophotometry (Opron-3000) at 200–600 nm. The final concentrations of the prepared S. aureus and E. coli media were about 106/μL each. Bacterial suspensions were diluted with 0.9% sterile saline, and the final concentrations were 1 × 106 colony-forming units per 100 μL. The rats then received a topical application of 1 × 106 colony-forming units of both S. aureus and E. coli in 100 μL and were left for 24 hours for infection to develop.Citation25 All rats were divided into three groups, ie, three rats in group A, six rats in group B, and three rats in group C. The electrospun NDEM (PLGA/ collagen matrix) was applied to the infected wounds of each rat in group A, while the DEM (PLGA/collagen/antibiotic) was applied to the infected wounds of each rat in group B. The rats in group C were treated with conventional gauze sponge as the control. No dressings were replaced and no topical drugs were applied during the entire healing process. Intradermal (beneath the dressings) tapping using 19-gauge needle aspiration was performed on days 1, 3, 7, 14, and 21 in three group B rats (wounds treated with DEM) for in vivo release analysis. On postoperative days 7, 14, and 21, macroscopic photographs of the wounds were taken. Further, the wound dressings on the other three rats were removed on postoperative days 7, 14, and 21 for histological examination of epithelialization and granulation.
In vivo release behavior
The concentrations of antibiotics and lidocaine in the tapped fluid were measured by HPLC on a Hitachi L-2200 multisolvent delivery system. All tapping samples were assayed after dilution with phosphate-buffered solution and assessed using the standard assay curve. A calibration curve was made for each set of measurements (correlation coefficient > 0.99).
The relative antibiotic activity of the collected fluid was determined using the disk diffusion method in nutrient broth (beef extract, Peptone, Difco Laboratories, Detroit, MI). Eight microliters of eluent were pipetted onto 6 mm absorption disks. The disks were placed on nutrient agar plates seeded with a layer of S. aureus. The inhibition zones were measured within a micrometer after 16–18 hours of incubation at 35°C. A 50 μg/mL drug solution was used as a reference. The relative activity of the vancomycin released was defined as:
The bioactivity of gentamicin on E. coli was determined by the same method.
Scanning electron microscopic observation
The morphology of the electrospun nanofibers was observed under a scanning electron microscope (Hitachi S-3000N, Japan) after gold coating. The average diameter and size distribution were determined by analyzing the scanning electron microscopic images using a commercial image analysis program (Optimas version 5.22).
Statistics and data analysis
Data were collected from quadruplicate samples and analyzed by one-way analysis of variance. Differences were considered statistically significant at P values <0.05.
Results and discussion
Electrospinning of DEM
In this study, sandwich-structured DEM with PLGA/collagen surface layers and a PLGA/drug core layer were successfully fabricated via the electrospinning technique. During the electrospinning process, a polymeric solution placed inside a syringe is driven out from a metal capillary connected to a high voltage power supply. Nanofibers are collected in the form of a nonwoven matrix on a grounded collector after solvent evaporation. Continuous PLGA, collagen, and pharmaceutical nanofibers were obtained at concentrations that corresponded to the onset of significant chain entanglements in the polymers. The influence of processing parameters on the distribution of the fiber diameters was investigated. It was found that the fiber diameter decreased with the content of drugs and collagen in the blended solutions.Citation20 Furthermore, the diameter of the electrospun fibers increased to some extent with voltage and distance between the needle and its target.
By adopting appropriate process parameters (such as solvent, polymer concentration, and flow rate), electrospun sandwich-structured nanofibrous membranes could be obtained. shows scanning electron micrographs of the electrospun nanofibers under magnification at 20,000×. The surface PLGA/collagen layers () showed a greater diameter distribution than the core PLGA/drug layer (), attributable mainly to the presence of collagen in the blended solutions. The diameters of the spun PLGA/ collagen and PLGA/drug nanofibers ranged from 55 nm to 222 nm and from 185 nm to 314 nm, respectively, and the porosity of the nanofibrous membrane was high.
Figure 2 Scanning electron microscopic images of electrospun, sandwich-structured nanofibers. (A) Top PLGA/collagen layer, (B) PLGA/vancomycin/gentamycin/lidocaine at the core, and (C) bottom PLGA/collagen layer.
Abbreviation: PLGA, poly(D, L)-lactide-co-glycolide.
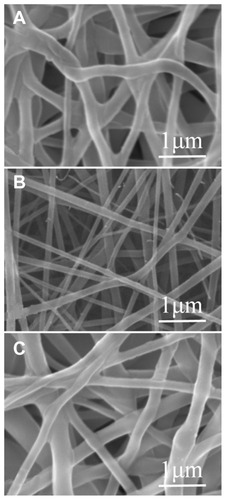
The mechanical properties of the electrospun nanofibrous membranes were determined. shows the measured stress-strain curves for the prepared PLGA/collagen membranes. The tensile strength and elongation at break were measured to be 1.13 MPa and 116%, respectively. The nanofibrous membranes produced by electrospinning were shown to have the mechanical characteristics needed for application as a wound dressing.
In vitro and in vivo drug release
Daily in vitro release curves for vancomycin, gentamicin, and lidocaine are shown in . All drugs showed a triphasic release pattern, with an abrupt initial burst phase on day 1. After the electrospinning process, most of the drugs were dispersed in the bulk of the PLGA matrix; however, some drug particles and crystals might have been located on the surface of the nanofibers. This led to the initial burst of drug release. Following the initial burst, drug release was controlled solely by diffusion. A second peak of release was observed in week 2, and the concentration gradually decreased thereafter. Further, the biodegradable DEM released high concentrations of Gram-positive vancomycin and Gram-negative gentamicin, well above the 90% minimum inhibitory concentration (MIC90)Citation26,Citation27 for 24 and 23 days, respectively. The membrane also eluted lidocaine for 15 days.
Figure 4 In vitro release behavior of vancomycin, gentamicin, and lidocaine from nanofibrous membranes.
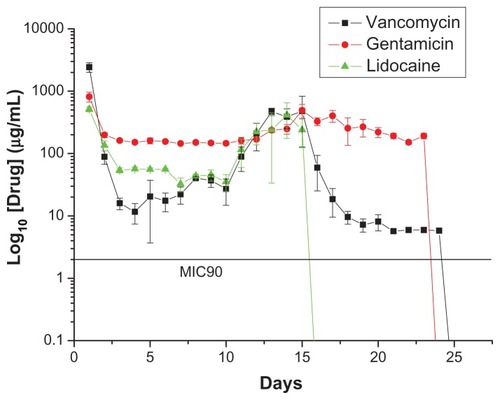
In vivo drug levels were measured over 3 weeks using the HPLC assay, and the concentrations are shown in –. All drugs showed biphasic release and peaked at day 14 (vancomycin 310.87 μg/mL, gentamicin 1729.50 μg/mL, and lidocaine 648.40 μg/mL), and thereafter the cumulative concentration dropped progressively. The in vivo antibiotic concentrations remained well above the IC90 for at least 3 weeks ( and ), and for lidocaine, the concentration was still high at week 3 (). In addition, the bioactivity of the released antibiotics remained high, ranging between 40% and 90% and 45% and 100% for vancomycin and gentamicin, respectively, as shown in and .
Wound healing and histological examination
To test for healing of infected wounds, two full-thickness rectangular wounds were cut into the back of each rat. The rats then underwent topical application of 1 × 106 colony-forming units of S. aureus and E. coli in 100 μL, followed by coverage with DEM, NDEM, or gauze sponge. shows the animals from each group at weeks 1, 2, and 3 after grafting. All four rats in group C (control rats, infected wounds covered only by gauze) died within the first week after grafting. Only rats from group A (wound covered by NDEM) and group B (wound covered by DEM) are shown in the graph.
Figure 8 Appearance of wound healing at weeks 1, 2, and 3 after grafting. (A) PLGA/collagen group and (B) PLGA/collagen with vancomycin, gentamicin, and lidocaine group (rectangular wounds 2 cm × 2 cm were prepared on each rat’s back, as shown in A1 and B1).
Abbreviation: PLGA, poly(D, L)-lactide-co-glycolide.
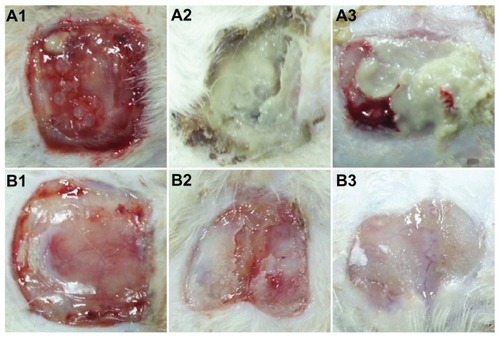
In the first week after grafting, the gross wound appearance in rats with NDEM-covered wounds was similar to that in the rats with DEM-covered wounds (). In the second week, necrotic tissue combined with frank pus formation were noted in rats with NDEM-covered wounds (), and in contrast, clear soft tissue with neovascularization were noted in rats with DEM-covered wounds (). In the third week after grafting, the wound state was worsening in the rats with NDEM-covered wounds (). However, the wound healing process was almost complete in the rats with DEM-covered wounds (). Further, the grafts prepared with DEM scaffolds were well integrated into the surrounding skin. Electrospun DEM appeared to be more effective than NDEM in promoting wound healing. The wound area covered by electrospun DEM decreased to approximately 25% at 21 days.
The images from histological examination shown in demonstrate that the DEM dressings had a better healing effect than NDEM dressings on infected wounds. In the first week after grafting, there was no discernible difference between histological sections of wounds treated by NDEM and DEM dressings (), all showing an ulcerated surface, formation of granulation tissue, and infiltration with inflammatory cells. In the second and third weeks after grafting, the wounds treated with a DEM dressing () were healing, with newly synthesized fibrous tissue and sparse inflammatory cells in the dermis and subcutis, covered by a completely re-epithelialized epidermis. In contrast, the wounds treated with an NDEM dressing () showed incomplete re-epithelialization and prominent inflammatory cell infiltration. This confirms further that electrospun DEM can be a suitable tissue-engineering scaffold for repair of infected wounds and skin regeneration.
Figure 9 Histological images of (A) PLGA/collagen group and (B) PLGA/collagen with vancomycin, gentamicin, and lidocaine group at weeks 1, 2, and 3. (No necrosis was observed in B3).
Abbreviation: PLGA, poly(D, L)-lactide-co-glycolide.

The bactericidal effects of the antibiotics incorporated into the biodegradable membrane far outweigh any negative inherent effects of the membrane itself.Citation28 Local antibiotics and anesthetics have the advantage of delivering high drug concentrations to the precise area required, and the total drug dose applied locally is not normally sufficient to produce toxic systemic effects. Despite the possibility of the antimicrobial and anesthetic agents released from the nanofibers harming viable tissue and delaying cell growth and proliferation during the healing process, the potential impact of the drugs released on cell proliferation gradually diminished in the 2 weeks following grafting ().
As a potential wound dressing scaffold for tissue engineering, the nanofibrous matrix should promote cell growth and physiological function and should be able to maintain normal states of cell differentiation. The presence of collagen in the PLGA/collagen nanofibers increases the wettability of the electrospun matrices, which may promote cell proliferation in the matrices.Citation29 In addition, collagen is a natural extracellular matrix component of many tissues, including skin, bone, tendon, ligament, and other connective tissues that promote wound healing. Despite previous reports of the electrospinning process denaturing the biological and structural properties of natural proteins such as collagen,Citation18 our findings show that the PLGA/collagen nanofibrous matrix remains intact during electrospinning and can act as an excellent scaffold for cell growth and proliferation. Further, the bioactivity of vancomycin and gentamicin remains high after the electrospinning process, indicating that electrospun drug-eluting PLGA/collagen nanofibers are good candidates for dressings to assist in the repair of infected wounds.
Conclusion
In the present study, a sandwich-structured, nanofibrous, drug-eluting matrix produced by electrospinning was formulated for use as a wound dressing. The experimental results show that the biodegradable nanofibrous membranes released high concentrations of vancomycin and gentamicin (well above the MIC90) and lidocaine in vivo for more than 3 weeks. The bioactivity of the antibiotics released ranged from 40% to 100% of relative activity. In addition, repair of infected wounds in rats was studied. Histological examination of epithelialization and granulation at the wound site was also performed. It was found that nanofibrous membranes were functionally active in treating wound infections and were very effective as wound-healing accelerators in the early stages of healing. By using the electrospinning technique, we may be able to manufacture biodegradable, biomimetic, nanofibrous, extracellular membranes for long-term delivery of a range of medicines.
Acknowledgments
This work was supported financially by the National Science Council of Taiwan (grant NSC100-2314-B-182A-010).
Disclosure
The authors report no conflicts of interest in this work.
References
- BesseyPQWound careHerndonDNTotal Burn Care3rd edPhiladelphia, PAElsevier2007
- WardenGDSaffleJRKravitzMA 2-stage technique for excision and grafting of burn woundsJ Trauma198222981037038136
- JayakumarRPrabaharanMNairSVTamuraHNovel chitin and chitosan nanofibers in biomedical applicationsBiotechnol Adv20102814215019913083
- SrinivasanSJayakumarRChennazhiKPLevorsonEJMikosAGNairSVMultiscale fibrous scaffolds in regenerative medicineAdv Polym Sci2012246120
- SillTJvon RecumHAElectrospinning: Applications in drug delivery and tissue engineeringBiomaterials2008291989200618281090
- MiFLWuYBShyuSSControl of wound infections using a bilayer chitosan wound dressing with sustainable antibiotic deliveryJ Biomed Mater Res20025943844911774301
- PengHZhouSGuoTIn vitro degradation and release profiles for electrospun polymeric fibers containing paracetanolColloids Surf B Biointerfaces20086620621218691851
- ElsnerJJZilbermanMAntibiotic-eluting bioresorbable composite fibers for wound healing applications: Microstructures, drug delivery and mechanical propertiesActa Biomater200952872288319416766
- ElsnerJJEgoziDUllmannYBerdicevskyIShefy-PelegAZilbermanMNovel biodegradable composite wound dressings with controlled release of antibiotics: Results in guinea pig burn modelBurns20113789690421466923
- TeoEYOngSYChongMSKPolycaprolactone-based fused deposition modeled mesh for delivery of antibacterial agents to infected woundsBiomaterials20113227928720870283
- MengZXXuXXZhengWPreparation and characterization of electrospun PLGA/gelatin nanofibers as a potential drug delivery systemColloids Surf B Biointerfaces2011849710221227661
- MengZXZhengWLiLZhengYFFabrication, characterization and in vitro drug release behavior of electrospun PLGA/chitosan nanofibrous scaffoldMater Chem Phys2011125606611
- KontogiannopoulosKNAssimopoulouANTsivintzelisIPanayiotouCPapageorgiouVPElectrospun fiber mats containing shikonin and derivatives with potential biomedical applicationsInt J Pharm201140921622821316431
- LiWJLaurencinCTCatersonEJTuanRSKoFKElectrospun nanofibrous structure: a novel scaffold for tissue engineeringJ Biomed Mater Res20026061362111948520
- NohHKLeeSWKimJMElectrospinning of chitin nanofibers: Degradation behavior and cellular response to normal human keratinocytes and fibroblastsBiomaterials2006273934394416574218
- MinBMYouYKimJMLeeSJParkWHFormation of nano-structured poly(lactide-co-glycolic acid)/chitin matrix and its cellular response to normal human keratinocytes and fibroblastsCarbohydr Polym200457285292
- YooCRYeoISParkKEEffect of chitin/silk fibroin nanofibrous bicomponent structures on interaction with human epidermal keratinocytesInt J Biol Macromol20084232433418243300
- PowellHMSuppDMBoyceSTInfluence of electrospun collagen on would contraction of engineered skin substitutesBiomaterials20082983484318054074
- RhoKSJeongLLeeGElectrospinning of collagen nanofibers: Effects on the behavior of normal human keratinocytes and early-stage wound healingBiomaterials2006271452146116143390
- LiuSJKauYCChouCYChenJKWuRCYehWLElectrospun PLGA/collagen nanofibrous membrane as early-stage wound dressingJ Membr Sci20103555359
- TeoWERamakrishnaSA review on electrospinning design and nanofibre assembliesNanotechnology200617R8910619661572
- WilliamsDFBiodegradation of surgical polymersJ Mater Sci19821712331246
- AliSADohertyPJWilliamsDFMechanisms of polymer degradation in implantable devices. 2. Poly(DL-lactic acid)J Biomed Mat Res19932714091418
- GrzybowskiJKolodziejWTrafnyEAStruzynaJA new anti-infective collagen dressing containing antibioticsJ Biomed Mater Res1997361631669261677
- HellmilaMRMattarATaddonioMATopical nanoemulsion therapy reduces bacterial wound infection and inflammation after burn injurySurgery201014849950920189619
- ApplemanMDCitronDMEfficacy of vancomycin and daptomycin against Staphylococcus auerus isolates collected for over 29 yearsDiagn Microbiol Infect Dis20106644144420226335
- ChaRIn vitro activity of cefepime, tigecycline, and gentamicin, and in combination, against extended-spectrum β-lactamase-producing Klebsiella pneumoniae and Escherichia coliPharmacotherapy20082829530018294108
- ZilbermanMElsnerJJAntibiotic-eluting medical devices for various applicationsJ Control Release200813020221518687500
- MatthewsJAWnekGESimpsonDGBowlinGLElectrospinning of collagen nanofibersBiomacromolecules2002323223811888306