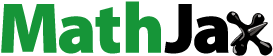
Abstract
Methacrylic-based copolymers in drug-delivery systems demonstrate a pH-sensitive drug-releasing behavior in the colon. In this study, copolymers of methacrylic acid and 2-ethyl hexyl acrylate were prepared using a microemulsion polymerization technique. The purified copolymer was characterized by Fourier transform infrared spectroscopy, proton nuclear magnetic resonance spectroscopy, and differential scanning calorimetry. 5-Fluorouracil (5-FU) was entrapped within methacrylic-based copolymers by a solvent evaporation method. The size of the nanogels formed was characterized by transmission electron microscopy and atomic force microscopy. In vitro drug-release studies using phosphate-buffered saline at different pH levels demonstrated the sustained release of 5-FU and its pH dependence. Cell proliferation assay of a human colon tumor colon cancer cell line (HCT-116) was performed and showed that the nanogels containing 5-FU exhibited considerable cytotoxicity in comparison with free 5-FU. Cell uptake of the nanogels was also monitored using confocal microscopy. Western blot analysis and flow cytometry studies confirmed that the nanogels could be successfully used as an efficient vector for pH-sensitive and controlled delivery of drugs specifically targeted to the colon.
Introduction
In the treatment of colon cancer, pH sensitivity of the polymeric drug-delivery vehicle is an inevitable issue in oral drug-delivery systems (DDSs). Even though 5-Fluorouracil (5-FU) has been shown to be the most effective drug employed for the treatment of colon cancer,Citation1 several factors are known inefficacies, including short biological half-life, poor absorption due to the enzyme dihydropyrimidine dehydrogenase, and nonselective action against healthy cells of the gastrointestinal tract and bone marrow.Citation2,Citation3 In addition, there are variations in the transit time throughout the colon. Moreover, drug release from a polymer-coated tablet can be incomplete when the colon-specific tablet matrix does not readily disintegrate, thus the 5-FU treatment is inadequate. To overcome these limitations and to improve the targeted delivery of 5-FU, the drug needs to be delivered to the colon in nano-sized formulations that have a pH-sensitive polymer matrix.Citation4 Methacrylic-based copolymers are known to demonstrate pH-sensitive swelling behavior and also form hydrogel matrices.
Hydrogels are hydrophilic polymeric three-dimensional networks that exhibit a semisolid morphology and can absorb large amounts of water. The hydrophilicity is due to the presence of ionizable functional groups that account for the characteristics of the hydrogel such as permeability, mechanical stability, and biocompatibility.Citation5 Biodegradable hydrogels have been suggested as attractive drug formulations due to their advantages of biocompatibility, high responsibility for specific degradation, and feasibility of their incorporating the drug into matrices.Citation6 Hydrogel-based devices belong to a group of swelling-controlled DDSs.Citation7,Citation8 The swelling of the hydrogel depends on the pH of the medium. In the case of methacrylic-based hydrogels, the swelling is observed between pH 7.2 and 7.8. Thus, in the colonic environment, they are assumed to exhibit a dynamic swelling behavior and sustained release of the entrapped drug molecules. Methacrylic acid (MA)-based hydrogels are believed to show considerable biocompatibility, as described previously.Citation9,Citation10
The design of an amphiphilic hydrogel-based DDS by incorporating a hydrophobic moiety to the methacrylic-based copolymer will serve as an efficient system, with better loading of hydrophilic drug and high mechanical stability. By oral administration of the drug to the colon, a DDS should be able to bypass the high acidic pH of the stomach (pH 1.5–2.5), the pH of duodenum (pH 6), and then needs to reach the colon at pH 7.6–7.8. The proposed polymer with an ionizable-COOH group shows a pH-sensitive swelling behavior in basic pH.
In the present study, we synthesized a novel copolymeric pH-sensitive DDS for the delivery of 5-FU using MA and 2-ethyl hexyl acrylate (EHA). The synthetic methacrylic acid-ethyl hexyl acrylate (MAEHA) copolymer was characterized using different techniques including Fourier transform infrared spectroscopy (FTIR), proton nuclear magnetic resonance spectroscopy (1H NMR), and differential scanning calorimetry (DSC). The nano-sized hydrogel containing 5-FU was prepared using the MAEHA copolymer using a solvent evaporation technique. The size and morphology of the nanogels were determined using transmission electron microscopy (TEM) and atomic force microscopy (AFM). The thermal behavior of the polymer was also monitored using DSC. In vitro drug release was studied to assess the pH-sensitive drug-release behavior of the nanogels. For a biological evaluation, the HCT-116 human colon cancer cell line was selected. The cellular uptake of these nanogels was studied with a fluorescent dye using confocal microscopy. Controlled release of the drug from the nanogels and its in vitro cytotoxicity when compared to free 5-FU was analyzed using 3-(4, 5-dimethylthiazol-2-yl)-2,5-diphenyltetrazolium bromide (MTT) assay. To confirm the induction of apoptosis in the tumor cell line, poly (ADP-ribose) polymerase (PARP) cleavage was detected by Western blot analysis. The alteration in cell cycle was investigated by flow cytometry.
Materials and methods
Materials
Sodium dodecyl sulfate (SDS), MA, potassium persulfate, EHA, hydroquinone, rhodamine, 5-FU, MTT, and b-actin were all purchased from Sigma-Aldrich (St Louis, MO). Fetal bovine serum was purchased from Gibco (Life Technologies, Carlsbad, CA), Roswell Park Memorial Institute medium (RPMI)-1640 from Invitrogen (Life Technologies, Carlsbad, CA). The enhanced chemiluminescence kit was purchased from GE Healthcare (Little Chalfont, UK) and secondary antibodies (horseradish peroxidase-conjugated anti-mouse) were purchased from Santa Cruz Biotechnology, Inc (Santa Cruz, CA). All organic solvents were of high-performance liquid chromatography grade.
Methods
Synthesis of MAEHA copolymer
MAEHA copolymer was synthesized by a microemulsion polymerization technique using double-deionized water as the dispersion medium.Citation11 The monomers were MA and EHA. SDS was used as the surfactant (10% v/v) and potassium persulfate (3%, v/v) was the initiator for all studied reactions. The monomers EHA (60%–70%) and MA (25%–30%) were taken in different combinations. All reactions were carried out at ~70°C for 2 hours. All reactions were performed with magnetic stirring set at 500 rpm. The polymerization initiator was quenched by adding hydroquinone. The copolymer formed was separated as a precipitate using, ethanol. The residue formed was subsequently separated. The product was purified by dialysis using MEMBRA-CEL® dialysis tubing (22 nm diameter; MWCO 3500, SERVA Electrophoresis GmbH, Heidelberg, Germany), which ensured the removal of all unreacted monomers and sol fraction. A synthetic scheme for the synthesis of the MAEHA copolymer is shown in .
Figure 1 Synthesis of MAEHA copolymer.
Note: Synthesis of MAEHA copolymer using methacrylic acid and 2-ethyl hexyl acrylate as monomers by microemulsion polymerization using KPS as a radical initiator and SDS as the surfactant.
Abbreviations: MAEHA, methacrylic acid-co-2-ethyl hexyl acrylate; KPS, potassium persulfate; SDS, sodium dodecyl sulfate.
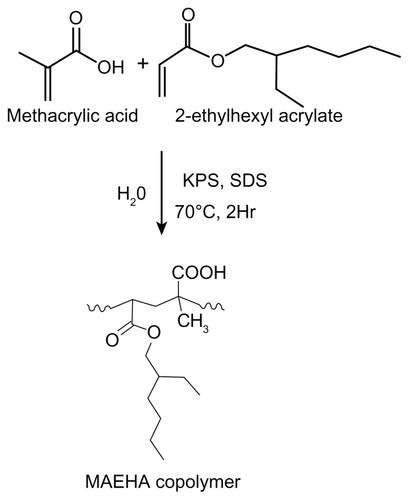
Characterization of copolymer
FTIR spectra
The various compositions of copolymer formed were characterized using FTIR (NicoletTM 5700 spectrometer; Thermo Fisher Scientific, Waltham, MA). The sample aliquots were prepared using potassium bromide pellets. For each sample, eight scans were collected and averaged to reduce the signal to noise ratio. The spectral range covered the mid-infrared region from 4000 to 400 cm−1.
1H NMR
The nuclear magnetic resonance (NMR) spectrum of the polymer was acquired using a Bruker 500 MHz spectrometer (Bruker BioSpin, Billerica, MA) with acetone as the solvent.
DSC analysis
DSC was performed to analyze the polymer matrix. DSC thermograms were obtained using an automatic thermal analyzer system (PyrisTM DSC 6000; PerkinElmer, Waltham, MA). Samples were crimped in standard aluminum pans and heated from 40°C up to 250°C at a rate of 10°C/minute under constant nitrogen purging of 10 mL/minute. An empty pan, sealed similarly to the sample, was used as reference.
Preparation of 5-FU-loaded nanogels
Drug loading was performed using a solvent evaporation technique. Briefly, 5-FU 1% (w/v) was dissolved in water and mixed with different proportions of MAEHA copolymer 5% (w/v) dissolved in acetone. The mixing was carried out with constant vortexing. The resultant solution was then sonicated for 3 minutes in a Sonics® Vibra-CellTM ultrasonic processor (Sonics and Materials, Inc, Newton, CT), and the organic solvent was then evaporated completely by stirring of the emulsion overnight. The hydrogel that formed was then subjected to lyophilization and stored at −20°C. The hypothesized loading chemistry is shown in .
Determination of drug encapsulation efficiency
A known amount of the lyophilized 5-FU-loaded polymer was dissolved in acetone and vigorously vortexed until a clear solution was obtained then kept for 24 hours following filtration through a 0.1 μM membrane filter. The filtrate was determined to have an absorbance of 266 nm using ultraviolet absorbance (Lambda 25; PerkinElmer). The entrapped 5-FU content was determined using the respective ratio between the actual entrapment ratio (AER) and the theoretical entrapment ratio (TER), expressed in terms of amount of 5-FU per weight of nanospheres. The entrapment efficiency can be determined by the following equation:
where AER = measured drug wt/nanosphere wt and TER = Initial drug wt/drug wt and polymer wt.
Characterization of nanogels
TEM analysis
Morphological studies of the nanogels were characterized by TEM (1101-JEM-1011; JEOL Ltd, Tokyo, Japan). The drug-loaded nanogels (0.5% w/v, 0.05% w/v), dissolved in Milli-Q® water (Millipore Corporation, Billerica, MA), were dropped on a copper-coated formvar microscopy grid. The grid was washed with 1 μL Milli-Q water. The sample on the grid surface was allowed to dry at room temperature in a desiccator containing calcium chloride and measurements were taken. TEM pictures were taken using DigitalMicrographTM (Gatan Inc, Pleasanton, CA) and Soft Imaging Viewer software (Olympus Soft Imaging Solutions GmbH, Munster, Germany).
AFM observation
A total of 1 μL of hydrogel was deposited onto a freshly cleaved mica surface. After approximately 15 seconds, the hydrogel on the mica surface was washed with 50 μL of Milli-Q water then the mica was air dried. To study the influence of the drying process on the network structures, some hydrogel samples were placed on mica and then vacuum dried. AFM images were obtained by scanning the mica surface in air with a Nanoscope 3a microscope (Digital Instruments, Santa Barbara, CA) using a silicon cantilever (NCH-10V; Veeco Instruments Inc, Plainview, NY) operated in tapping mode.Citation12
Particle size analysis
The particle size of the MAEHA nanogels was measured by dynamic light scattering (DLS) using a Delsa™ Nano particle size analyzer (Beckman Coulter, Inc, Fullerton, CA) instrument at ambient temperature. The samples were analyzed without prior filtration.
In vitro drug-release studies
The release of 5-FU from the MAEHA copolymer at different pH levels was studied by performing in vitro drug-release studies. A weighed 10 mg amount of the drug-containing hydrogel was dispersed in each of three 50 mL freshly prepared phosphate-buffered saline (PBS) solutions of pH values 2.0, 7.0, and 7.8 respectively, mimicking the different pH conditions of the gastrointestinal tract.Citation13 The reaction mixture was incubated at 37°C and subjected to continuous shaking at 90 rpm using a rotary shaker. The controlled release pattern was observed for 900 minutes.Citation14 It was assumed that the hydrogel showed pH-sensitive release. A 1 mL volume of the reaction mixture was withdrawn at different time intervals and the amount of 5-FU released was estimated at 266 nm using a Lambda 25 ultraviolet-visible spectrophotometer (PerkinElmer).
Cell culture
HCT-116 cells were purchased from American Type Culture Collection (Manassas, VA) and maintained in RPMI-1640 medium supplemented with 10% (v/v) fetal bovine serum and a 1% antibiotic-antimycotic cocktail (Life Technologies) at 37°C under a humidified atmosphere containing 5% carbon dioxide.
Cell cytotoxicity assay
MTT reduction assay was performed to assess cell cytotoxicity. Cells were seeded into a 96-well plate (5000 cells/well) and incubated under standard growth conditions for 24 hours for 60%–70% confluency. Cells were then treated with formulations of 5-FU (1–100 μM) for 24, 48, and 72 hours, respectively. Following the drug treatment periods, the cells were again incubated for an additional 4 hours with 10% v/v MTT (5 mg/mL dissolved in PBS pH 7.4). The media was then removed completely and the formed formazan crystals were dissolved in 100 μL isopropyl alcohol. Absorbance was measured at 570 nm using a Bio-Rad microplate reader, model 680 (Bio-Rad Laboratories, Inc, Hercules, CA). Percent inhibition was calculated using the following formula: [(Average OD of control − Average OD of test)/(Average OD of control) × 100].
Cell uptake studies
HCT-116 cells grown up to 70% confluency were trypsinized and seeded (8 × 104 cell) onto a sterile coverslip and incubated for 24 hours for cell attachment. The media was aspirated completely, and rhodamine-entrapped nanogels were diluted in media and added at a concentration of 10 μmol/L and incubated for 4 hours at 37°C. The cells were counterstained with Hoechst stain (15 minutes, 37°C). At the end of the incubation period, the monolayer cells were washed twice with PBS and fixed in 4% paraformaldehyde for 30 minutes at room temperature. Paraformaldehyde was completely removed by washing twice with PBS. The cells were mounted on a clean glass slide and viewed under a confocal laser scanning microscope (TCS SP2; Leica Microsystems, Wetzlar, Germany) at a magnification of 63×, and subsequent fluorescent images were captured. Similar experiments were also performed for blank nanogels.
Western blot analysis
A total of 2 × 106 cells were seeded in a 100 mm culture dish for 24 hours and 5-FU formulations at different concentrations were assessed for 48 hours. Cells were then lysed, and the total protein content was measured using Bradford’s reagent. An aliquot of 60 μg of total protein was loaded for SDS polyacrylamide gel electrophoresis and immunoblotting was carried out using antibodies specific for PARP. Horseradish peroxidase-conjugated secondary antibodies were used followed by detection using enhanced chemiluminescence.
Cell-cycle analysis
For flow cytometry analysis, 2 × 106 cells were seeded in 100 mm dishes. Free 5-FU, free nanogels, and 5-FU formulations were given at different concentrations for 24 hours. Cells were harvested and fixed with 70% ethanol for 1 hour. The fixed cells were then given ribonuclease A (100 mg/mL) treatment for 1 hour followed by incubation with propidium iodide (10 mg/mL), in the dark, for 15 minutes. Finally, the DNA content of the cells was analyzed using a FACSAria™ 1 special order system (BD Biosciences, San Jose, CA).
Statistics
All measurements were performed in triplicate and results were expressed as arithmetic mean ± standard error of the mean.
Results
Preparation and characterization of MAEHA copolymer
The MAEHA copolymer was successfully prepared using the microemulsion copolymerization technique. The MAEHA in pure form showed good solubility in acetone. The synthesized copolymer was characterized by FTIR and 1H NMR spectra and DSC. The FTIR spectra of the copolymer confirmed the structure of the polymer, as shown in .
Figure 3 FTIR spectra of MAEHA copolymer.
Note: FTIR spectra of MAEHA copolymer was recorded using potassium bromide pellets. Peak a: 1731 cm−1 (ester), 1701 cm−1 (acid); peak b: 3445 cm−1 (−OH); peak c: 1189 cm−1 and 1269 cm−1 (ester); peak d: 2961−2931 cm−1 (ester).
Abbreviations: FTIR, Fourier transform infrared spectroscopy; MAEHA, methacrylic acid-co-2-ethyl hexyl acrylate.
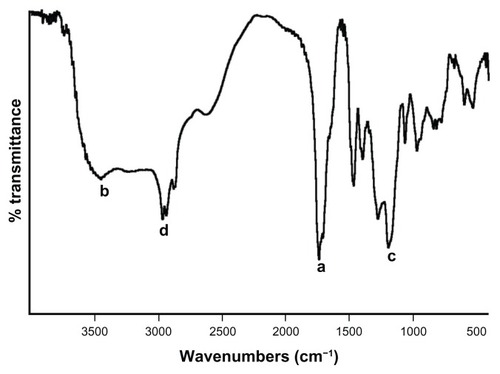
The peak at 1731 cm−1 indicates the presence of an ester group (−COOR) and the 1701 cm−1 peak denotes the presence of an acid group (−COOH) in the copolymer (peak a). The broad peak at 3445 cm−1 shows the presence of an −OH stretching band of the −COOH group (peak b). Peaks at both 1189 cm−1 and 1269 cm−1 depict the stretching of the −CO bond of the ester linkage of EHA (peak c). The methyl −CH− stretching band was depicted at 2961–2931 cm−1 (peak d). The free-radical copolymerization was also confirmed from the 1H NMR data, as shown in .
Figure 4 1H NMR spectra of MAEHA copolymer.
Notes: The presence of methyl (−CH3), methylenic (−CH2-), and tertiary methyl groups were also confirmed by the spectral data (peaks A, B, and C). The NMR spectral peak at 4.0 δ shows the presence of a methylenic group adjacent to the carboxyl group of EHA (peak D). 1H NMR spectra of methacrylic acid-co-2-ethyl hexyl acrylate (MAEHA) copolymer was recorded using acetone as the solvent.
Abbreviations: 1H NMR, proton nuclear magnetic resonance spectroscopy; MAEHA, methacrylic acid-co-2-ethyl hexyl acrylate.
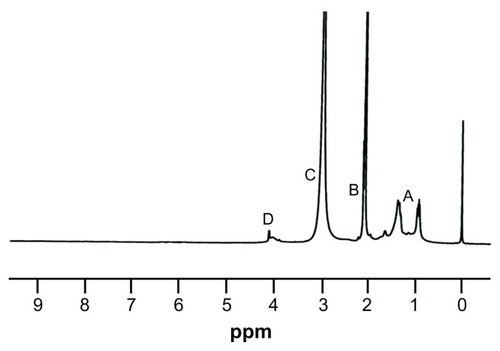
1H NMR data indicates that the synthesis of the copolymer was complete. The absence of a peak at 5.7–6.5 δ supports the fact that the acrylic and methacrylic double bonds of most of the monomer units have undergone the free-radical polymerization to give the desired polymer. The presence of methyl (−CH3), methylenic (−CH2-), and tertiary methyl groups were also confirmed by the spectral data (peaks A, B, and C). The NMR spectral peak at 4.0 δ shows the presence of a methylenic group adjacent to the carboxyl group of EHA (peak D). The DSC of the synthetic polymer shows a glass transition temperature (Tg) value of 115°C.Citation15 From the thermogram, it was determined that the polymer was stable up to 80°C ().
Drug loading and characterization of the 5-FU-loaded nanogels
A high number of drug-loaded nanogels were observed with an encapsulation efficiency of 80%. TEM analysis of the copolymer revealed that the drug-entrapped nanogels ranged in size between 100 and 150 nm. The nanogels were found to be uniformly distributed over the grid and showed homogeneity in size distribution ().
Figure 6 TEM image of MAEHA nanogels.
Notes: For TEM, the sample of MAEHA nanogel suspension in Milli-Q® water was dropped onto formvar-coated copper grids without being negatively stained. Measurements were taken only after the sample had completely dried.
Abbreviations: TEM, transmission electron microscopy; MAEHA, methacrylic acid-co-2-ethyl hexyl acrylate.

AFM images of the drug-entrapped nanogels were further confirmed by the sizes of the nanogels in the range of 100–150 nm. The spherical nanogels showed slight aggregation. Intermolecular hydrogen bonding was found to be responsible for the self-assembly of the polymer chains of MAEHA to form the nanogels with the loaded 5-FU. The hydrophilicity and versatility of the nanogels will be helpful in the controlled release of the drug from the polymer matrix (). On DLS analysis, the drug-entrapped nanogels showed a mean diameter of 150 nm (). The homogeneity of the nanogels was also supported by the DLS data. The polydispersity index was found to be 0.100.
Figure 7 AFM image of MAEHA nanogels. (A and C) Phase images; (B and D) amplitude images.
Notes: For AFM, the sample of 1 μL of MAEHA nanogel suspension was dropped onto freshly cleaved mica surface and washed with 50 μL of Milli-Q® water. Measurements were taken only after the sample had completely dried.
Abbreviations: AFM, atomic force microscopy; MAEHA, methacrylic acid-co-2-ethyl hexyl acrylate.
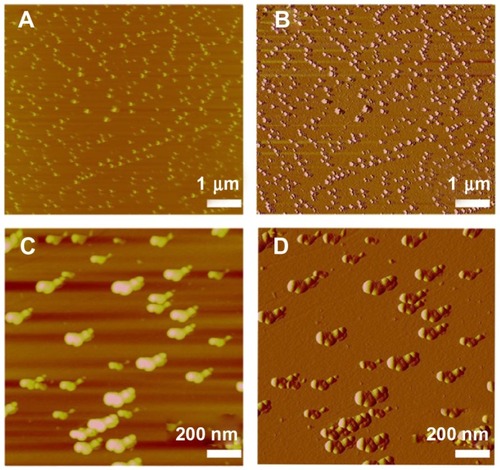
Figure 8 Dynamic light scattering curve showing the particle size of the methacrylic acid-co-2-ethyl hexyl acrylate nanogels.
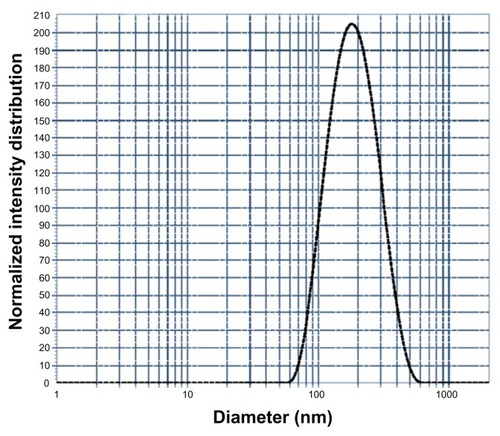
To assess the release pattern of 5-FU from the nanogels, in vitro kinetics studies were performed. The major aim of the in vitro drug-release studies was to illustrate the pH-sensitive drug-release properties of the copolymer of MAEHA. Kinetics studies clearly indicated that the MAEHA copolymer showed pH-sensitive drug-release behavior. The MAEHA copolymer showed a good release pattern at basic pH. During the initial 60 minutes, there was a burst release of drug from the nanogels. The release pattern observed in all the combinations at different pH followed Peppas’ modelCitation8 of drug release. The release pattern is shown in . In acidic pH, a low concentration of drug was released, indicative of the fact that the stability of the nanogels was considerable for the controlled release. The drug release from the nanogels was shown to increase as the pH of the buffer was increased. At basic pH, it was observed that the nanogels showed a sustained release pattern over time.
Figure 9 In vitro drug-release profile from the MAEHA nanogels.
Notes: Release pattern of the drug 5-FU from MAEHA nanogels in phosphate-buffered saline at pH 7.8, pH 7.0, and pH 2.0 at 37°C. All measurements were done in triplicate. The results are expressed as arithmetic mean ± standard error of the mean.
Abbreviations: MAEHA, methacrylic acid-co-2-ethyl hexyl acrylate; 5-FU, 5-Fluorouracil.
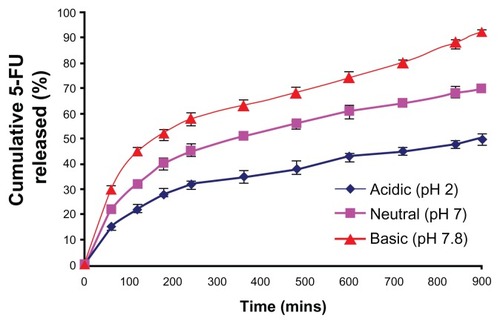
5-FU-loaded nanogels showed significant cytotoxicity
MTT assay revealed that 5-FU-entrapped nanogels exhibited higher cytotoxicity than that of free 5-FU over a range of experimental concentrations in the HCT-116 cell line. When these cells were exposed to nanogels for 24 hours, significant cytotoxicity was observed (44.1% inhibition at 100 μM concentration) over that of free 5-FU. The biocompatibility of the free nanogels was also illustrated by the MTT results, as they were found significantly nontoxic, even after 72 hours. Forty-eight-hour MTT data showed the inhibition was 56.27% for nanogels with 5-FU, twice the value of the free drug. After 72 hours of incubation, the nanogels showed highest inhibition of 64.75% for 100 μM, as shown in .
Figure 10 MTT analysis of MAEHA nanogels with 5-FU on HCT-116 cells at (A) 24, (B) 48, and (C) 72 hours on incubation at the concentrations indicated.
Abbreviations: MTT, 3-(4, 5-dimethylthiazol-2-yl)-2,5-diphenyltetrazolium bromide; MAEHA, methacrylic acid-co-2-ethyl hexyl acrylate; 5-FU, 5-Fluorouracil; HCT, human colon tumor.
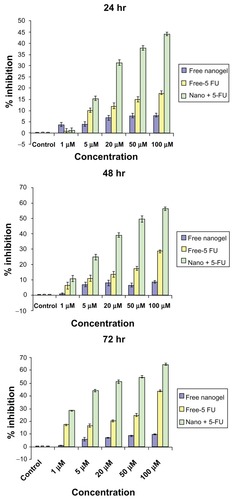
The cellular uptake of the nanogels was monitored using confocal microscopy. The results show that the nanogels with rhodamine were internalized by the HCT-116 cells at as early as 4 hours of incubation. The internalized particles were seen as red fluorescence of rhodamine in the cell cytoplasm. The internalization of the nanogels was further substantiated by the images taken after 4 hours of incubation ().
Figure 11 Cellular uptake studies of MAEHA nanogels.
Notes: Top row: confocal images of HCT-116 cells after 4 hours incubation with rhodamine-entrapped MAEHA nanogels: bright field, rhodamine, Hoescht stain, merged image. Bottom row: confocal images of HCT-116 cells after 4 hours incubation with blank MAEHA nanogels: bright field, rhodamine, Hoescht stain, merged image.
Abbreviations: MAEHA, methacrylic acid-co-2-ethyl hexyl acrylate; HCT, human colon tumor.
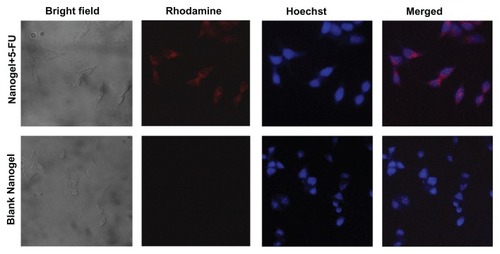
Western blotting for PARP cleavage was performed to characterize apoptotic cell death. Cleavage of PARP, a DNA repairing protein, is a hallmark of apoptosis.Citation16 Immunoblot analysis showed that the intensity of the 89 kDa cleaved PARP band had increased considerably in a dose-dependent manner (25, 50, and 80 μM of 5-FU nanogels; ). Since PARP cleavage is a marker of apoptosis, these results show the efficiency of 5-FU nanogels to cause cell death.Citation17
Figure 12 Immunoblot analysis and densitometry graph showing PARP. (A) Comparison of PARP cleavage induced by 25, 50, and 80 μM of MAEHA nanogels with 5-FU in HCT-116 cells after incubation for 24 hours. (B) Densitometry graph showing the intensity of the bands.
Notes: All measurements were done in triplicate. Results are expressed as arithmetic mean ± standard error of the mean.
Abbreviations: PARP, poly (ADP-ribose) polymerase; 5-FU, 5-Fluorouracil; MAEHA, methacrylic acid-co-2-ethyl hexyl acrylate.
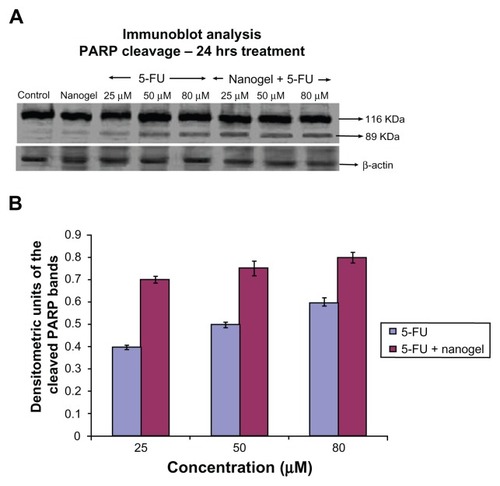
Cell-cycle analysis was performed to identify whether the 5-FU nanogels caused any cell-cycle arrest. Free 5-FU at a lower concentration caused an S-phase arrest; however, as the dose increased, the arrest was seen to shift to G1 phase.Citation18 When treating the cells with the 5-FU-nanogel formulation in a dose-dependent manner, the cell-cycle arrest was maintained at S-phase (25, 50, 75, and 100 mM) ().
Figure 13 Fluorescein-activated cell-sorting analysis. (A) Cell-cycle analysis of HCT-116 cells: (left) control and (right) blank nanogels. (B) Cell-cycle analysis of HCT-116 cells of free 5-FU and MAEHA nanogels with 5-FU in concentrations 25, 50, 75, and 100 μM.
Abbreviations: HCT, human colon tumor; 5-FU, 5-Fluorouracil; MAEHA, methacrylic acid-co-2-ethyl hexyl acrylate.
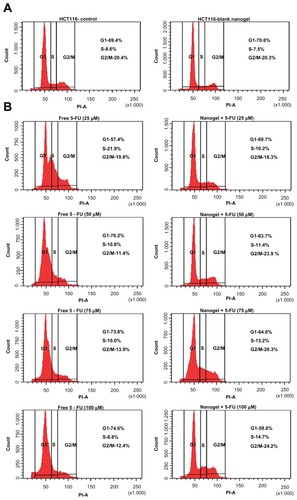
Discussion
In recent years, considerable attention has been given to the field of advanced biomaterials, including nanobiomaterials and smart hydrogels.Citation19–Citation21 Our study presents the preparation and evaluation of 5-FU-loaded pH-sensitive MAEHA nanogels as a therapeutic agent for cancer. The highly efficient but nonspecific cancer drug 5-FU was used in this study. Thus, our main objective was to synthesize highly stable 5-FU-loaded MAEHA nanogels and evaluate their cytotoxicity in cancer cells. The hypothesis was that 5-FU-loaded MAEHA nanogels would impart a cytotoxic effect on cancer cells by releasing the drug in a controlled manner, inducing apoptosis.
The synthesis of the MAEHA copolymer was confirmed by FTIR and NMR spectra, as shown in and , which clearly depict the formation of the copolymer. Use of a modified solvent evaporation technique led to the formation of MAEHA nanogels in the size range of 100–150 nm. The pH-sensitive drug-release profile of the 5-FU-MAEHA nanogels was able to substantiate the sustained release pattern of 5-FU.
Our study clearly demonstrates that 5-FU-MAEHA nanogels can induce cell death in cancer cell lines at a lower dose than that of the free drug. This is an important observation because cancer cell death has been considered the target of chemotherapeutic agents in a variety of cancer research.Citation22,Citation23 Apoptosis is a process of an essential tissue homeostasis, thus is regarded as the ideal way to inhibit cancer cell growth.Citation24 Therefore, the induction of cancer cell death by 5-FU-MAEHA nanogels can be viewed as a potential new approach in cancer treatment. Many studies have demonstrated that the cell death in cancer cells induced by anticancer drugs occurs in a dose- and time-dependent manner.Citation25–Citation28 Our data are in agreement with this fact, showing that the 5-FU-MAEHA nanogels with higher concentrations of 5-FU can induce cancer cell death within a short period. Additional studies using Western blotting and fluorescein-activated cell-sorting analysis showed the induction of apoptosis, confirming the efficacy and the mechanism by which the 5-FU-MAEHA nanogels induce cell death.
Conclusion
This study has shown that 5-FU-loaded MAEHA nanogels are an efficient DDS with a diverse pH-responsive mechanism. Even though many pH-sensitive DDSs have been previously reported,Citation4,Citation8,Citation9 the MAEHA nanogel system presented here is novel. The synthesis of amphiphilic polymer MAEHA with desired properties proved to be an efficient vector for the entrapment of hydrophilic anticancer drugs. Further, the method used to load the drug into the polymeric nanogels is straightforward. The pH sensitivity of the nanogels will be key in site-specific targeting of 5-FU to the colon. Complete cellular internalization of the biocompatible nanogels was also observed. The high rate of cytotoxicity resulting from the induction of apoptosis is an added advantage in the use of MAEHA nanogels as an effective DDS. This biocompatible DDS is believed to accumulate in the tumor microenvironment and bring about cell death by apoptosis.
Acknowledgments
The authors are thankful to the National Institute of Interdisciplinary Sciences and Technology, Kerala, for NMR studies; the Indian Institute of Science Education and Research Trivandrum for AFM analysis; the Department of Biotechnology, New Delhi, for providing the financial assistance for research work; and the Council of Scientific and Industrial Research, New Delhi, for the Junior Research Fellowship awarded to Ashwani Kumar N.
Disclosure
The authors report no conflicts of interest in this work.
References
- Van CutsemEPeetersMVerslypeCFilezLHaustermansKJanssensJThe medical treatment of colorectal cancer: actual status and new developmentsHepatogastroenterology1999462670971610370599
- FataFRonIGKemenyNO’ReillyEKlimstraDKelsenDP5-fluorouracil-induced small bowel toxicity in patients with colorectal carcinomaCancer19998671129113410506695
- Di PaoloADanesiRFalconeARelationship between 5-fluorouracil disposition, toxicity and dihydropyrimidine dehydrogenase activity in cancer patientsAnn Oncol20011291301130611697844
- LamprechtAYamamotoHTakeuchiHKawashimaYMicrosphere design for the colonic delivery of 5-fluorouracilJ Control Release200390331332212880698
- KimISKimSHChoCSDrug release from pH-sensitive interpenetrating polymer networks hydrogel based on poly (ethylene glycol) macromer and poly (acrylic acid) prepared by UV cured methodArch Pharm Res19961911822
- GanderBGurnyRDoelkerEPeppasNAEffect of polymeric network structure on drug release from cross-linked poly(vinyl alcohol) micromatricesPharm Res1989675785842508077
- ColomboPSantiPBettiniRBrazelCSDrug release from swelling-controlled systemsWiseDLHandbook of Pharmaceutical Controlled Release TechnologyNew York, NYMarcel Dekker2000183209
- KimBLa FlammeKPeppasNADynamic swelling behavior of pH-sensitive anionic hydrogels uses for protein deliveryJ Appl Polym Sci200389616061613
- WangKXuXLiuTTSynthesis and characterization of biodegradable pH-sensitive hydrogel based on poly(ɛ-caprolactone), methacrylic acid, and Pluronic (L35)Carbohydr Polym2010793755761
- De GrootCJVan LuynMJVan Dijk-WolthuisWNIn vitro biocompatibility of biodegradable dextran-based hydrogels tested with human fibroblastsBiomaterials200122111197120311336291
- OttoDPVoslooHCLiebenbergWde VilliersMMDevelopment of microporous drug releasing film cast from artificial nanosized latexes of poly(styrene-co-methyl methacrylate) or poly(styrene-co-ethyl methacrylate)Eur J Pharm Biopharm20086931121113418417330
- ZhaoYYokoiHTanakaMKinoshitaTTanTSelf-assembled pH-responsive hydrogels composed of the RATEA16 peptideBiomacromolecules2008961511151818498190
- FallingborgJChristensenLIngeman-NielsenMJacobsenBAbildgaardKRasmussenHHpH-Profile and regional transit times of the normal gut measured by a radiotelemetry deviceAliment Pharmacol Ther1989366056132518873
- YangLChuJSFixJAColon-specific drug delivery: new approaches and in vitro/in vivo evaluationInt J Pharm20022351–211511879735
- PenzelERiegerJSchneiderHAThe glass transition temperature of random copolymers: 1. Experimental data and the Gordon-Taylor equationPolymer1997382325337
- Simbulan-RosenthalCMRosenthalDSIyerSBoularesAHSmulsonMETransient poly(ADP-ribosyl)ation of nuclear proteins and role of poly(ADP-ribose) polymerase in the early stages of apoptosisJ Biol Chem19982732213703137129593711
- BoularesAHYakovlevAGIvanovaVRole of poly(ADP-ribose) polymerase (PARP) cleavage in apoptosis. Caspase 3-resistant PARP mutant increases rates of apoptosis in transfected cellsJ Biol Chem199827433229322294010438458
- GuoXGoesslEJinGCell cycle perturbation and acquired 5-fluorouracil chemoresistanceAnticancer Res2008281A91418383818
- Miquelard-GarnierGDemouresSCretonCHourdetDSynthesis and rheological behavior of new hydrophobically modified hydrogels with tunable propertiesMacromolecules2006392381288139
- SoppimathKSAminabhaviTMKulkarniARRudzinskiWEBiodegradable polymeric nanoparticles as drug delivery devicesJ Control Release2001701–212011166403
- NairKLJagadeeshanSNairSAKumarGSBiological evaluation of 5-fluorouracil nanoparticles for cancer chemotherapy and its dependence on the carrier, PLGAInt J Nanomedicine201161685169721980233
- NaganeMPanGWeddleJJDixitVMCaveneeWKHuangHJIncreased death receptor 5 expression by chemotherapeutic agents in human gliomas causes synergistic cytotoxicity with tumor necrosis factor-related apoptosis-inducing ligand in vitro and in vivoCancer Res200060484785310706092
- LoweSWRuleyHEJacksTHousmanDEp53-dependent apoptosis modulates the cytotoxicity of anticancer agentsCell19937469579678402885
- EvanGIVousdenKHProliferation, cell cycle and apoptosis in cancerNature2001411683534234811357141
- PintoACMoreiraJNSimoesSCiprofloxacin sensitizes hormone-refractory prostate cancer cell lines to doxorubicin and docetaxel treatment on a schedule-dependent mannerCancer Chemother Pharmacol200964344545419104815
- Di SalvatoreMOrlandiABagalàCAnti-tumour and anti-angiogenetic effects of zoledronic acid on human non-small-cell lung cancer cell lineCell Prolif201144213914621401755
- ParveenSMitraMKrishnakumarSSahooSKEnhanced antiproliferative activity of carboplatin-loaded chitosan-alginate nanoparticles in a retinoblastoma cell lineActa Biomater2010683120313120149903
- YoshikawaRKusunokiMYanagiHDual antitumor effects of 5-fluorouracil on the cell cycle in colorectal carcinoma cells: a novel target mechanism concept for pharmacokinetic modulating chemotherapyCancer Res20016131029103711221829