Abstract
This article explores the novel gold nanoparticle–enhanced photodynamic therapy of methylene blue against recalcitrant pathogenic Candida albicans biofilm. Physiochemical (X-ray diffraction, ultraviolet-visible absorption, photon cross-correlation, FTIR, and fluorescence spectroscopy) and electron microscopy techniques were used to characterize gold nanoparticles as well as gold nanoparticle–methylene blue conjugate. A 38.2-J/cm2 energy density of 660-nm diode laser was applied for activation of gold nanoparticle–methylene blue conjugate and methylene blue against C. albicans biofilm and cells. Antibiofilm assays, confocal laser scanning, and electron microscopy were used to investigate the effects of the conjugate. Physical characteristics of the gold nanoparticles (21 ± 2.5 nm and 0.2 mg/mL) and methylene blue (20 μg/mL) conjugation were confirmed by physicochemical and electron microscopy techniques. Antibiofilm assays and microscopic studies showed significant reduction of biofilm and adverse effect against Candida cells in the presence of conjugate. Fluorescence spectroscopic study confirmed type I photo toxicity against biofilm. Gold nanoparticle conjugate–mediated photodynamic therapy may be used against nosocomially acquired refractory Candida albicans biofilm.
Introduction
Biofilms are spatially structured heteromorphic microbial communities ensconced in exopolymeric matrix material.Citation1,Citation2 It has been shown that a substantial amount of microbial infections occur through biofilm formation.Citation3 Candida albicans is a frequently isolated fungal species from infections and recurrently associated with biofilm formation. Citation4 It is generally found as a harmless ubiquitous commensal species in normal microbiota of humans, such as in the gastrointestinal and genitourinary tracts.Citation5 However, under conditions of immune dysfunction, colonizing C. albicans possess the capacity to opportunistically cause life-threatening infections in immunocompromised patients.Citation6,Citation7 Since last century, Candida biofilm has played an indispensable role in health care-related infections. C. albicans is currently regarded as the fourth- and third-leading cause of hospital-acquired bloodstream and urinary tract infections, respectively.Citation8 In the US, candidemia has become recalcitrant and the fourth-leading hospital-acquired infection.Citation9
Candida biofilm is one of the main causes of clinical repercussions through encounters with such implanted biomaterials as intravascular catheters, pacemakers, prostheses, stents, shunts, urinary catheters, and orthopedic implants. Hence, these implants serve as colonies as well as inseminating reservoirs of further Candida infections.Citation8
Biofilm is highly resistant against drug molecules as compared to planktonic cells.Citation10 Despite a growing antifungal armamentarium, recalcitrant Candida biofilm presents multiple complex factors against antimicrobial agents, and these multifactorial phenomena need to be further unraveled. Among the most important factors are biofilm matrix or exopolmeric substance (EPS), high density of cells in biofilm, presence of presister cells, and drug-efflux pumps.Citation11–Citation15
Ambient matrix or EPS produced by sessile cells of biofilm recently gained the spotlight and imparting the impregnation of drug molecules and putative charge barrier.Citation16,Citation17 Recently, photodynamic therapy (PDT) emerged as an alternative to conventional treatment of infections caused by C. albicans.Citation18,Citation19 In PDT, photosensitizing drugs render the type I and type II phototoxicity in the presence of specific wavelengths of light.Citation20 Irradiation of photosensitizers with appropriate wavelengths of light in the presence of molecular oxygen produces highly cytotoxic reactive oxygen species such as hydroxyl free radicals (type I phototoxicity) and singlet oxygen (type II phototoxicity).Citation21 Photosensitizer thiazine dyes such as methylene blue (MB), toluidine blue O (TBO), and pthalocyanine have been shown to be phototoxic against Pseudomonas aeruginosa and Porphyromonas gingivalis biofilm.Citation22–Citation24 Recently, it has also been reported that MB also has fungicidal effects against C. albicans.Citation18,Citation19
Colloidal gold nanoparticles (GNPs) have resilient properties compared to other inorganic nanoparticles, such as unique plasmon-resonance optical properties and a bioconjugation surface for molecular probes. These unique properties have drawn attention with a view to developing a totally different method in the field of nanomedicine.Citation25 GNP has been used as a carrier of antitumor drugs, antibodies, antibiotics, and other drugs for selective killing of diseased cells and microbes.Citation26–Citation28 GNP per se did not have any antimicrobial activity, but when coupled with antibiotics showed significant antimicrobial effect.Citation28,Citation29
Cationic thiazine dyes such as TBO and MB showed strong interaction with GNP by increased ultraviolet (UV)- visible absorption.Citation30 It has been reported that TBO shows enhanced antibacterial properties when coupled with GNP as compared to TBO alone.Citation31 Hence we initiated this study to explore novel Candida antibiofilm activity by amalgamating the photocytotoxic properties of MB and antimicrobial enhancer properties of GNP.
Materials and methods
Synthesis of gold nanoparticles
GNPs were synthesized in colloidal form by a modified Turkevich–Frens method.Citation32,Citation33 First, 1 mM HAuCl4 (Sigma-Aldrich, St Louis, MO) was mixed with 20 mL distilled water and kept on a hot plate in stirring condition at about 80°C, then 1% of trisodium citrate dehydrate (Na3C6O7 · 2H2O; Sigma-Aldrich) reducing agent was added to the solution in stirring condition. Gradually, the color of the solution changed from transparent to red, and after 10 minutes it changed to a deep-red wine color, indicating colloidal GNP formation.
Spectroscopic characterization of synthesized gold nanoparticles
UV-visible spectra of GNP were taken using a double-beam UV-visible spectrophotometer (PerkinElmer, Boston, MA) with wavelength ranging from 400 to 700 nm in Milli-Q solvent. The X-ray powder diffraction (XRD) pattern was recorded using a Rigaku (Tokyo, Japan) Miniflex X-ray diffractometer with Cu Kα radiation (λ = 1.54060 Å) in 2θ ranging from 30° to 80°. GNP hydrodynamic particle size distribution analysis was done by a particle size analyzer (Nanophox; Sympatec, Clausthal-Zellerfeld, Germany) based on photon cross-correlation spectroscopy.
Growth conditions of organism
C. albicans (ATCC 90028) was grown in yeast peptone dextrose medium 1% yeast extract, 2% peptone, 2% dextrose (HiMedia, Mumbai, India). Batches of medium (20 mL in 250-mL Erlenmeyer flasks) were inoculated with freshly grown C. albicans in yeast peptone dextrose agar plates and incubated overnight in an orbital shaker (200 rpm) at 30°C under aerobic conditions. Cells were harvested and washed twice in sterile phosphate buffer saline (PBS) (pH 7.4). Cells were resuspended in Roswell Park Memorial Institute (RPMI) 1640 supplemented with L-glutamine and buffered with morpholinepropanesulfonic acid (HiMedia) and adjusted to the desired density after measurement with a hematocytometer.
Determination of minimum inhibitory concentration (MIC) and minimum fungicidal concentration (MFC)
The photosensitizer, MB, was purchased from Sigma-Aldrich, and stock solution (1.0 mg/mL) was made in PBS (10 mM phosphate buffer, 2.7 mM potassium chloride, 137 mM sodium chloride; pH 7.4). A twofold serial dilution of the MB (initial concentration 1.0 mg/mL) was performed. For the GNP–MB conjugate, after twofold serial dilution of MB, a constant concentration of GNP (0.2 mg/mL) was added to each diluted solution. Each inoculum was prepared in normal saline, and density was adjusted to a 0.5 McFarland standard and diluted to 1:100 for the broth microdilution procedure. After treatment with irradiation at 12 hours, microtiter plates continue to incubate at 37°C and MIC was recorded after 24 hours. MFC was determined by subculturing the test dilutions on to a fresh solid medium and incubating for a further 24 hours. The highest dilution that yielded no fungal growth on solid medium was taken as MFC.
Spectroscopic measurements of GNP–MB conjugate
A double-beam UV-visible spectrophotometer (PerkinElmer) was used to characterize the synthesized GNP and measure the mode of interaction of GNP with MB photosensitizer. Scanning was in the 400–700-nm wavelength range. Fluorescence spectra were recorded on a Hitachi (Tokyo, Japan) F-4500X fluorescence spectrometer controlled by a personal computer data-processing unit. The excitation was done at 600 nm and the emission spectra from 500 nm to 800 nm were collected. All excitation and emission slits were set at 1.5 nm.
FTIR measurement of the MB and GNP–MB conjugate was carried out with a Nicolet Magna 750 FT-IR spectrophotometer (DTGS detector, Ni-chrome source and KBr beam splitter; Thermo Fisher Scientific, Waltham, MA) with a total of 100 scans and resolution of 16 cm−1 at a range of 400–4000 cm−1, using silver bromide windows at room temperature.
Photosensitization of MB and GNP–MB conjugate
MB and GNP–MB conjugate were activated or photosensitized during treatment against Candida biofilm and C. albicans cells. Semiconductor AlGaInP diode laser (ML101J27; Mitsubishi, Tokyo, Japan) with 660-nm wavelength light source and output power of 120 mW was used for photosensitization. A laser illuminated area of 0.12 cm2 with energy density of 38.2 J/cm2 was used for 40 seconds for both MB and conjugate activation. In all Candida antibiofilm and antimicrobial assays, MB and GNP–MB conjugate were treated and activated at 6 and 18 hours during 12- and 24-hour incubation periods, respectively.
Candida biofilm formation
Biofilms were produced on commercially available presterilized, polystyrene, flat-bottom 96-well microtiter plates. At first, 100 μL of a standardized C. albicans cell suspension (107 cells/mL in RPMI 1640) was transferred into each well of a microtiter plate, and the plate was incubated for 1.5 hours at 37°C in a shaker at 75 rpm to allow for adherence to the surface of the wells. Three wells of each microtiter plate were used as control in an identical fashion, except that no Candida suspensions were added. Following the adhesion phase, the cell suspensions were aspirated and each well was washed twice with 150 μL of PBS to remove loosely adherent cells. A total of 100 μL of RPMI 1640 was then transferred into each of the washed wells with a pipette, and the plates were incubated at 37°C in a shaker at 75 rpm. The biofilm was allowed to develop for 24 hours and used in different assays. All assays were carried out on three different occasions in triplicate.
Crystal violet–staining assay
Control, GNP, MB and GNP–MB conjugate–treated Candida biofilm were assessed by microdilution method and quantified by crystal violet (HiMedia) assay, as described earlier.Citation34,Citation35 Treated and control biofilm-coated wells of microtiter plates were washed twice with 200 μL of PBS and then air-dried for 45 minutes. Then, each of the washed wells was stained with 110 μL of 0.4% aqueous crystal violet solution for 45 minutes. Afterwards, each well was washed four times with 350 μL of sterile distilled water and immediately destained with 200 μL of 95% ethanol. After 45 minutes of destaining, 100 μL of destaining solution was transferred to a new well and the amount of the crystal violet stain in the destaining solution measured with a microtiter plate reader (iMark; Bio- Rad, Hercules, California) at 595 nm. The absorbance for the controls was subtracted from the test values to minimize background interference.
XTT biofilm reduction assay
This assay was performed as described previously.Citation36 2,3- Bis (2-methoxy-4-nitro-5-sulfophenyl)-5-([phenylamino] carbonyl)-2H-tetrazolium hydroxide (XTT) (Sigma-Aldrich) was dissolved in PBS at a final concentration of 1 mg/L. The solution was filter sterilized using a 0.22-mm pore-size filter and stored at −70°C until required. Menadione (Sigma-Aldrich) solution (0.4 mM) was also prepared and filtered immediately before each assay. Prior to each assay, XTT solution was thawed and mixed with menadione solution at a volume ratio of 20:1. The adherent cells in treated and control Candida biofilm, as previously described in crystal violet assay, were washed four times with 200 μL of PBS to remove loosely adherent or planktonic cells. Afterwards, 158 μL of PBS, 40 μL of XTT, and 2 μL of menadione inoculated each of the prewashed wells. After incubation in the dark for 4 hours at 37°C, 100 μL of the solution was transferred to a new well and a colorimetric change in the solution measured using a microtiter plate reader (iMark) at 490 nm.
Electron microscopy of GNP, GNP–MB conjugate, Candida biofilm and Candida cells
Transmission electron microscopy (TEM) was used to determine the actual size, shape, and pattern of arrangement of synthesized GNP and GNP–MB conjugate. TEM was also used to measure the thickness of the MB layer around GNP. Colloidal GNP and GNP–MB conjugate droplets were placed on a copper grid and dried in desiccators before viewing under a field-emission electron microscope (JEM-2100F; Jeol, Tokyo, Japan) at 120 kV.
For Candida cells, TEM is the standard method used, as described elsewhere.Citation37 Control and treated culture material was removed by centrifugation at 1000 rpm for 10 minutes and the suspended pellet again centrifuged with PBS (pH 7.4). Secondary fixation was done in 1% osmium tetroxide for 2–3 hours at 4°C and washed with PBS (pH 7.4). Samples were then completely dehydrated by serially washing with ethanol and embedded in araldite CY 212 (Taab, Aldermaston, UK) resin for making the cell-pellet blocks. Ultrathin sections of cells were stained with uranyl acetate and lead citrate and observed under the JEM-2100F microscope at 120 kV.
The effects of GNP–MB conjugate on the morphogenesis of C. albicans cells in Candida biofilm were observed by scanning electron microscopy (SEM), examination as described elsewhere.Citation38 For SEM, C. albicans cells were inoculated on sterile glass cover-slip disks in 12-well cell culture plates (CoStar, Bethesda, MD) for 12 and 24 hours at 37°C by dispensing a standardized cell suspension (2 mL of a suspension containing 106 cells/mL in RPMI 1640) onto appropriate spherical glass disks. Meanwhile, during inoculation, Candida cells were pretreated at 6 and 18 hours with activated GNP–MB conjugate. The disks were removed after 12 and 24 hours and washed three times in sterile PBS. The resultant samples were fixed with 2% formaldehyde and 2.5% glutaraldehyde in PBS (pH 7.4) overnight, rinsed three times with PBS, and dehydrated in absolute ethanol series (70% for 10 minutes, 95% for 10 minutes, and 100% for 20 minutes). Samples were dried in desiccators prior to sputter coating with gold and observed by SEM (EVO 40; Zeiss, Jena, Germany) at 20 kV.
Confocal laser scanning microscopy (CLSM)
CLSM of Candida biofilm cells was performed as described elsewhere with slight modification.Citation39 CLSM was used to illustrate the effect of GNP and photosensitized or activated GNP–MB conjugate on Candida cells during Candida biofilm formation. Biofilm-forming Candida cells were grown on glass cover slips by adding 4 mL of standardized C. albicans strain ATCC 90028 in 12-well cell culture plates (CoStar). After 12 hours, GNP, GNP–MB-treated and control Candida cells on the glass cover slips were removed, transferred to new six-well culture plates, and incubated for 45 minutes at 37°C in 4 mL of PBS containing the following fluorescent dyes as molecular probes: concanavalin A ([Con A] Alexa Fluor 488 conjugated, 25 μg/mL; Invitrogen, Carlsbad, CA), FUN-1 (Fungolight 10 μM; Invitrogen) and 4,6-diamidino-2-phenylindole (DAPI, 2.5 μg/mL, Sigma-Aldrich). The excitation and emission wavelengths for FUN-1 are 543 nm and 560 nm, respectively, whereas the excitation and emission wavelengths for Con A are 488 nm and 505 nm, respectively. DAPI has an excitation wavelength of 358 nm and an emission wavelength of 461 nm of light. After incubation with the dyes, the disks were flipped on glass plates and the stained Candida biofilms were observed with a FluoView FV1000 (Olympus, Tokyo, Japan) confocal laser scanning microscope equipped with argon and HeNe lasers.
Statistical analysis
The effect of MB and GNP–MB conjugate on Candida biofilm formation was compared with control biofilms (without any supplement) and analyzed using Student’s t-test. Data with P-values <0.05 were considered statistically significant.
Results
Characterization of GNP
GNPs showed a strong absorption band in the visible range due to surface plasmon resonance. Surface plasmon resonance is caused by the motion of free electrons in the conduction band caused by interaction with an electromagnetic field.Citation40 When the frequency of the light wave became resonant with the electron motion, strong absorption was observed, which is the origin of colloidal colour.Citation41,Citation42 In the UV-visible spectrum of GNP, nanoparticles absorb light at around 534 nm, which is a visible range of red color ().Citation43 shows a typical powder XRD pattern of GNPs and confirmed the XRD peaks 111, 200, 220, and 311, which are in good agreement with previously reported data.Citation44,Citation45 GNP size (21 ± 2.5 nm) and hydrodynamic mean diameter (22.4 nm) were analyzed and measured by TEM and cross-correlation spectroscopy instruments, respectively (, respectively). It was observed that particles were ellipsoidal and quite stable as their sizes and shape remained constant after synthesis.
Figure 1 Ultraviolet-visible absorption spectra of GNP, MB, and GNP–MB conjugate.
Notes: GNP has signature spectra at 534 nm due to surface plasmon resonance. MB shows absorption peaks at 662 nm and 613 nm. With the increase of MB concentration in constant concentration of GNP (0.2 mg/mL), absorption peaks of GNP successively vanished at 534 nm, and new enhanced absorption peaks formed at 662 nm and 613 nm. Coloration pattern is shown in inset. Test tubes 1, 2, 3, and 4 show MB of 2.50, 5.00, 10.00 and 20.00 μg/mL concentrations, respectively. Test tubes 5 and 6 show GNP (0.2 mg/mL) and GNP–MB (20.00 μg/mL MB), respectively.
Abbreviations: GNP, gold nanoparticle; MB, methylene blue.
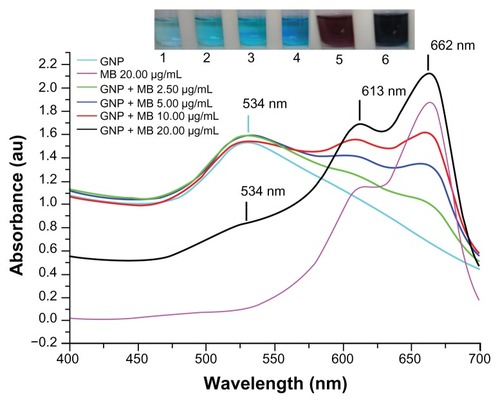
Figure 2 X-ray powder diffraction pattern by Cu Kα radiation (λ = 1.54060 Å) in 2θ ranging from 30° to 80°.

Figure 3 (A–C) Transmission electron microscopy images of GNP and GNP–MB (20 μg/mL; MB) conjugate. (C) Synthesized and clearly spherical colloidal GNPs with average size 21 ± 2.5 nm. (B) MB-conjugated GNPs and coating of MB around GNP are shown by black arrows. (A) The 2.52-nm thickness of MB coating around GNP. Photon cross-correlation spectra of GNP and GNP–MB (20 μg/mL; MB) conjugate are shown in (D and E) respectively. (D) Hydrodynamic mean diameter of GNP around 22.4 nm. (E) Hydrodynamic mean diameter of GNP–MB conjugate around 26.5 nm.
Abbreviations: GNP, gold nanoparticle; MB, methylene blue.
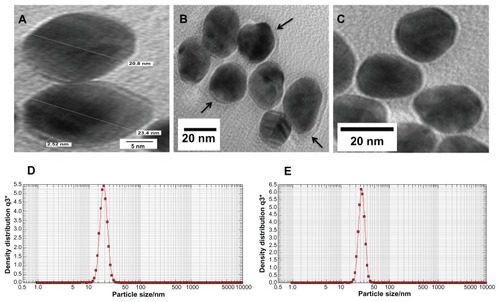
Minimum fungicidal and inhibitory effect of MB and GNP–MB conjugate
GNP showed no minimum inhibitory effect on Candida cells after serial dilution (initial concentration 0.2 mg/mL) on microtiter plate. MB showed MIC and MFC of 62.5 μg/mL and 125.0 μg/mL, respectively, whereas GNP–MB conjugate showed MIC and MFC of 31.2 μg/mL and 62.5 μg/mL, respectively. GNP–MB conjugate showed twofold higher activities against C. albicans than MB alone.
Physicochemical interaction of MB with GNP
The maximum absorption spectrum of GNP measured by UV-visible spectroscopy was at 534 nm due to surface resonance around the GNP. MB showed a primary maximum absorption peak at 662 nm and secondary absorption peak at 613 nm. After gradual addition of MB concentration up to 20 μg/mL in the presence of a constant concentration of GNP (0.2 mg/mL), there was a remarkable decrease in the GNP absorption peak at 534 nm and new peaks formed at 613 nm and 662 nm (). The UV-visible absorption result suggests that MB cationic dye either absorbed on GNP or GNP aggregated after addition of MB.
Further FTIR spectroscopy, photon cross-correlation spectroscopy (particle-size analysis), and TEM were conducted to determine the absorption of MB (20 μg/mL) on GNP and aggregation of GNPs. The FTIR spectra of GNP–MB conjugate as compared to MB shows a sharp peak near 1600 cm−1. A blunt peak of MB near 3250 cm−1 became much more intense near 3298 cm−1 in GNP–MB conjugate. Additional suppressed and broadened bands are observed near 2698 cm−1 for GNP–MB conjugate as compared to MB ().
Figure 4 Percent transmittance Fourier transform infrared spectroscopy spectra of MB (20 μg/mL) and GNP–MB (20 μg/mL; MB) conjugate.
Notes: GNPs in GNP–MB conjugate show characteristic transmittance peak at 654 cm−1. GNP–MB conjugate shows the prominent peak difference as compared to MB, shown by black arrows.
Abbreviations: GNP, gold nanoparticle; MB, methylene blue.
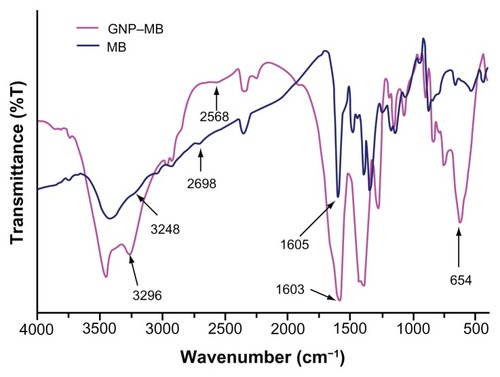
GNP mean diameter was measured by photon cross-correlation spectroscopy (particle-size analyzer) and was found to be 22.4 nm (), whereas after addition of MB (20 μg/mL), GNP size increased to 26.5 nm (). Further, GNP–MB size analyzed by TEM was observed to be 23 ± 2 nm (). Comparative studies of photon cross-correlation measurement () showed that there was no aggregation of GNPs on addition of MB.
Fluorescence spectroscopy was used to determine the enhancement or quenching of MB after conjugation with GNP (). Fluorescence spectra of MB (20 μg/mL) showed maximum fluorescence peak at 684 nm on excitation at 660 nm, as described elsewhere.Citation46 GNP (0.2 mg/mL) has a maximum fluorescence peak of 665 nm on excitation at 660 nm. A remarkable quenching in fluorescence of MB was observed on addition of GNP (0.2 mg/mL) at 684 nm.
Figure 5 Fluorescence emission spectra of GNP (0.2 mg/mL), MB (20 μg/mL), and GNP–MB (20 μg/mL; MB) conjugate after excitation at 660 nm.
Notes: In the presence of GNP (0.2 mg/mL), the fluorescence emission peak of MB quenches at 684 nm. In inset, GNP shows a maximum fluorescent emission peak at 665 nm.
Abbreviations: GNP, gold nanoparticle; MB, methylene blue.
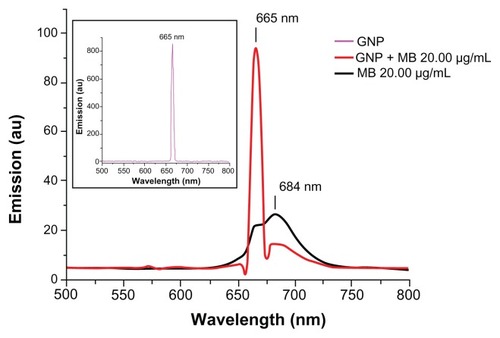
Quantitation of Candida biofilm formation
The antibiofilm activities of MB and GNP–MB were observed by using crystal violet and XTT biofilm reduction assays. Crystal violet assay has been used widely to measure biofilm formation because of its amenability to large screening procedures.Citation47 Crystal violet dye was used to measure percent inhibition on biofilm formation in the presence of different compounds because it affects diffusion as well as morphological and physiological differences in individual cells that influence dye binding.Citation48 A crystal violet assay revealed a maximum percent inhibition of Candida biofilm formation after 24 hours’ incubation, recorded as 63.2% and 82.2% by MB (20 μg/mL) and GNP–MB (20 μg/mL; MB) conjugate, respectively (). We used another tetrazolium salt (XTT) reduction assay for quantitative measurement of Candida biofilm formation. This assay is the most commonly used test to estimate viable biofilm growth and examine the impact of biofilm therapies. XTT assay is a more sensitive technique for the study of antifungal activity. In XTT assay, maximum percent inhibition during 24-hour treatment was found to be 81.9% and 95.4% for MB (20 μg/mL) and GNP–MB (20 μg/mL; MB) conjugate, respectively (). GNP-treated biofilms showed negligible reduction of biofilm as compared to control in both assays.
Figure 6 Crystal violet biofilm reduction assay bar plot represents the reduced biofilm optical density measurement at 595 nm.
Notes: Here, GNP (0.2 mg/mL) optical density shows the negligible inhibition of Candida biofilm as compared to control. With the increment of MB concentration in GNB-MB conjugate (1.75 μg/mL to 20 μg/mL; MB), biofilm or optical density of biofilm significantly decreases in GNP–MB-treated biofilm, in comparision to MB-treated biofilm.
Abbreviations: GNP, gold nanoparticle; MB, methylene blue.

Figure 7 Bar plot represents the XTT biofilm reduction assay at 490 nm.
Notes: Here, GNP (0.2 mg/mL)-treated and control biofilm show a negligible difference in optical density. With increments in MB and its concentration in GNP–MB conjugate (1.75 μg/mL to 20 μg/mL; MB), biofilm or optical density of biofilm significantly decreases in GNP–MB-treated biofilm as compared to MB-treated biofilm.
Abbreviations: GNP, gold nanoparticle; MB, methylene blue.
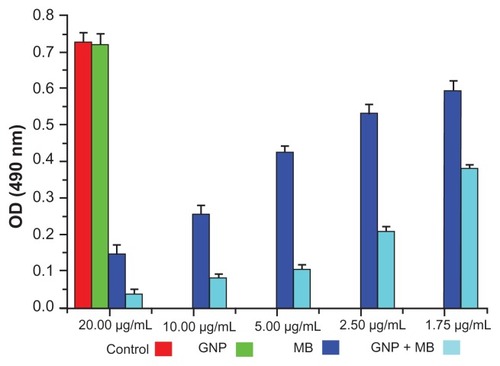
CSLM and electron microscopic exploration of MB–GNP effects on Candida cells and biofilm
SEM is important to determine the overall morphology of Candida cells and biofilm in three dimensions. Control, GNP (0.2 mg/mL), and GNP–MB (20 μg/mL; MB) treated biofilms were grown for 12 hours and 24 hours (, respectively). There was no significant difference in GNP-treated () and control biofilms (), shown as spherical yeast-type cells and elongated hyphae (black arrows in , respectively), whereas GNP–MB-treated biofilms showed a detrimental effect, wherein Candida cells and biofilms shrank, completely ruptured (shown by black arrows in ), and aggregated as irregularly shaped material (shown by red arrows in ).
Figure 8 (a–t) Morphological, cytological, and cytometabolic effects of GNP–MB (20 μg/mL; MB) conjugate on Candida biofilm. Here, morphology of Candida biofilm showed by scanning electron micrographs (a–d) 12-hour-grown biofilm; (e–h) 24-hour-grown biofilm); (b and f) GNP-treated Candida biofilm; (a and e) control. (c, d, g and h) Effects of GNP–MB conjugate on Candida biofilm. (j–l) Cytological effects of GNP–MB conjugate in 12-hour-grown Candida cells represented by transmission electron micrograph; (i) control; (M–T) Candida cytological perturbations in 12-hour-grown Candida cells viewed by confocal scanning micrograph; (o, p, s and t) GNP–MB treated; (n and r) GNP-treated; (m and q) control. Bars in (a–T) are 2 μM and 1 μM, respectively.
Abbreviations: GNP, gold nanoparticle; MB, methylene blue.
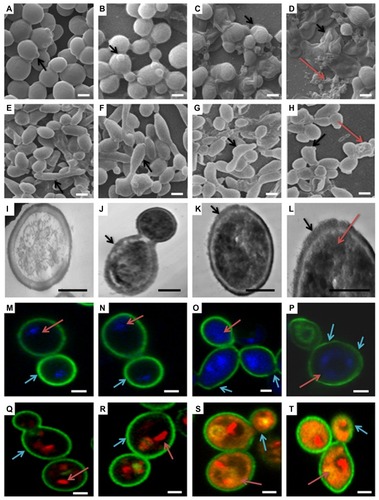
TEM was performed to further substantiate the effect of GNP–MB (20 μg/mL; MB) conjugate in the 12-hour-grown Candida cells. In TEM, control cell () showed intact cell wall integrity and cell organelles, whereas in the presence of GNP–MB, cell wall integrity was lost (shown by black arrows in ), and GNP–MB was shown in the dark-black cells (shown by red arrow in panel 8L). CLSM ultimately supported the TEM and SEM micrographs by revealing cellular metabolic activity, viability, and cell wall integrity, using FUN-1, DAPI, and Con A fluorescent dyes, respectively.
FUN-1 is transformed from a yellow to an orange-red fluorescent cylindrical or rodlike intravacuolar structure by metabolically active or viable cells, and Con-A is transformed to green fluorescence by binding to glucose and mannose residues of cell wall polysaccharides.Citation49,Citation50 DAPI intercalates between base pairs of DNA, specifically AT-rich regions of minor grooves, and gives blue fluorescence, which represents the polar cellular nucleus.Citation51 GNP (0.2 mg/mL)-treated Candida cells () have similar cytological features as compared to control cells (). Red arrows in , show intact vacuole in orange-red fluorescent cylindrical intravacuolar structure by FUN-1 Fluor probe. In , cyan arrows show the thick intact cell wall in green by cell wall–specific Fluor probe Con A. Red arrows in , show the intact polar nucleus by blue DAPI fluorescent probe. After GNP–MB (20 μg/mL; MB) treatment, FUN-1 probe showed cytosolic diffused yellow and orange fluorescence, which confirmed vacuolar disintegration and fragmentation in metabolic nonviable cells (red arrows in ) and loss of cell wall integrity by fading and thinning of Con-A green Fluor, represented by cyan arrows in . Conjugate-treated cells showed nuclear fragmentation by sprinkled and scattered DAPI blue Fluor in cytosol (red arrows in ).
Discussion
C. albicans is one of the foremost causes of nosocomial infection, which adheres and forms a matrix-like structure around the surface of prosthetic devices and implants such as joint replacements, heart valves, and intravascular or urinary catheters.Citation8 This matrix-like structure or biofilm shows a distinct phenotypic and chemical composition, which is not vulnerable to antimicrobial agents as compared to planktonic cells.Citation10 It became imperative to remove these biofilm-attached implants and devices, otherwise they may disseminate candidiasis infection.Citation52
Besides the multifactorial phenomenon,Citation11–Citation15 the unique properties of Candida biofilm matrix materials thwart antimicrobial activity against well-ensconced Candida cells as compared to planktonic cells. The chemical composition of ECM of Candida biofilm has not been not fully elucidated. Recently, it has been shown that a structure component of matrix and cell wall called β-1,3-glucans binds to antifungal drugs (fluconazole and amphotericin B) and minimizes antifungal activity against Candida cells.Citation16 Another putative factor that may attribute resistance to biofilms is negatively charged chemical composition in EPS, restricting the penetration of positively charged drug molecules.Citation17
In the last two decades, nanobiotechnologists have gained more interest in inorganic nanoparticles, mostly metallic nanoparticles that are now used for bioconjugation and biological imaging in drug delivery and diagnostic applications.Citation53,Citation54 Metallic nanoparticles have a large surface area, and this helps the interaction of a broad gamut of drug molecules.Citation55
Nobel metallic nanoparticles such as colloidal GNPs are attracting significant interest as carriers of various payloads towards the target. These payloads are antitumor agents and other drugs.Citation26,Citation56 GNPs have some unique chemical and physical properties that make them efficient carriers and enhancers. GNP has remarkable surface plasmon resonance for imaging and fluorescence enhancing or quenching of attached drugs or dyes.Citation57,Citation58
We performed the most efficient and straightforward synthesis of GNPs by using a modified Turkevich–Frens method,Citation32,Citation33 producing colloidal GNPs of around 20 nm. These synthesized GNPs were characterized by maximum-absorption spectra using UV-visible spectroscopy (). XRD of synthesized GNP confirmed that the GNPs were in good agreement with previously reported data ().Citation44,Citation45 Photon cross-correlation spectroscopy (particle-size analyser) () and TEM findings () bolstered GNP size (22.4 and 21 ± 2.5 nm, respectively). Here, we used appropriate and optimum size and concentration (21 ± 2.5 nm and 0.2 mg/mL) of GNP, which is compatible with mammalian cells, as described elsewhere.Citation59,Citation60 GNP (0.2 mg/mL) did not show any antibiofilm ( and ; crystal violet and XTT biofilm reduction assay) and fungicidal (MIC) activity against Candida biofilm and Candida cells. It was earlier described that GNPs do not affect bacterial growth, but when coupled with vancomycin they show antibacterial activity.Citation28
These charged stabilized nanoparticles have been attached or bioconjugated with a variety of photosensitizers and antibiotics.Citation61,Citation30 Earlier studies have been performed against pathogenic microbes by amalgamating the properties of both colloidal GNP and thiazine dyes such as MB and TBO as a GNP–thiazine conjugate. In one of the studies, GNP conjugated with TBO showed effective PDT against Staphylococcus aureus and enhanced the bactericidal effect up to 90%–99%.Citation31 Recently, in another study, GNP-conjugated biodegradable and biocompatible poly(lactic-co-glycolic acid) PGLA with MB embedded showed effective antimicrobial PDT against Enterococcus faecalis-infected tooth root canal.Citation62
Many studies have demonstrated MB-mediated PDT against bacterial and fungal infections.Citation63,Citation64 It was described that MB (0.05 mg/mL) inhibits 80%–90% of Candida cells upon treatment of 683-nm diode laser light irradiation with a 28 J/cm2 energy dose.Citation18 In another study, 0.05 mg/mL MB increased membrane permeability and reduced Candida growth with irradiation of 684-nm light diode laser with 28 J/cm2 energy dose.Citation65 In our study, diode laser of 660-nm light with 38.2 J/cm2 energy dose in the presence of MB inhibited the Candida cells’ growth (MIC; 62.5 μg/mL) and showed 125.0 μg/mL fungicidal activity. This result is comparable with previously described results, but there is some inconsistency in previously described PDT protocols because of varying factors that influence the results of PDT. These factors are media in which particular strains are grown, type of strain, time of exposure, concentration of photosensitizer, and energy dose of laser.Citation66
Most studies of GNP-conjugated photosensitizer have been performed on planktonic or suspended microbes, but these types of conjugate were never applied against microbial biofilm. This is the first study to characterize GNP–MB conjugation for examining antibiofilm activity against Candida biofilm. In the present study, we used a subconcentration of MB photosensitizer (20 μg/mL) and 660-nm diode laser with 38.2 J/cm2 energy against Candida biofilm, which has been reported as nonlethal against mammalian host cells.Citation67
The spectroscopic techniques and TEM were used to determine the type of interaction between MB and GNP. In UV-absorption spectroscopy, with incremental MB concentration the plasmon resonance peak of constant concentration of GNP (0.2 mg/mL) decreases and new absorption peaks appear. The absorption peak of GNP–MB (20 μg/mL; MB) was significantly higher as compared with MB (20 μg/mL) alone (). This new absorption peak may be attributed to aggregation of GNPs or interaction between GNP and MB. Further, three types of studies were performed to elucidate the UV-absorption finding. In the first study, FTIR spectroscopy of GNP–MB (20 μg/mL; MB) shows the percent transmission peaks near 1600 cm−1 and 3200 cm−1, indicating the presence of aromatic rings and primary amine (N–H), respectively, as compared to FTIR peaks of MB (20 μg/mL).Citation68,Citation69 In addition, the absence of an S–H vibration band of MB (near 2698 cm−1) in GNP–MB conjugate indicates deprotonation and coordination with GNP ().Citation70 In a second study, hydrodynamic mean diameter of GNP and conjugate was measured by photon correlation spectroscopy where GNP–MB (20 μg/mL; MB) conjugate had a mean diameter of 26.5 nm as compared to colloidal GNP (22.4 nm). This minimal average size difference (~4 nm) confirmed that there was no aggregation of GNP in the presence of maximum absorbance at 20-μg/mL concentration of MB (). In the third study, TEM images showed that synthesized colloidal GNPs are of an ellipsoid shape, with 21 ± 2.5-nm average size (), and in the presence of MB (20 μg/mL) a layer of 2 ± 0.5 nm was formed around GNP (). TEM image () shows there is no aggregation of GNP–MB conjugate at MB (20 μg/mL) experimental concentration. These three studies evidently substantiate GNP–MB interaction. Furthermore, FTIR and absorption studies propound the aurophilic coordination bond to GNP through sulphur atoms of MB and electrostatic interaction between GNP and MB, respectively.
Preliminary in vitro antimicrobial studies of GNP, MB, and GNP–MB conjugate were carried out with MIC and MFC assay, wherein MIC and MFC of GNP–MB conjugate were found to be 31.2 μg/mL and 62.5 μg/mL, respectively, as compared to MIC and MFC of MB at 62.5 μg/mL and 125.0 μg/mL, respectively. GNP did not demonstrate any antifungal activity at initial constant experimental concentration (0.2 mg/mL). Inhibitory and fungicidal studies were instigated to investigate antifungal activity against Candida biofilm, wherein XTT biofilm reduction () and crystal violet reduction assay () demonstrated 95.4% and 82.2% reduction of Candida biofilm by GNP–MB conjugate, respectively, as compared to MB, which reduced the biofilm by 81.9% and 63.2%, respectively.
Three-dimensional morphological studies by SEM clearly illustrate the GNP–MB-treated Candida cells in 12- and 24-hour-grown biofilm, which are deformed, shrunken, completely ruptured, and aggregated in the form of irregularly shaped materials ().
Further, TEM and CLSM capture the cytological and cytometabolical alteration in Candida cells after GNP–MB conjugate treatment. Our TEM micrograph shows that the treated cells lost their cell wall integrity and increased GNP–MB conjugate permeability; as a consequence, GNP–MB conjugate penetrated the Candida cells and thus caused cytological perturbation (). FUN-1, Con-A, and DAPI fluorescent probes in CLSM micrographs illustrate the decrease of metabolic viability (), loss of cell wall integration (), and fragmentation of nucleus or degradation of nuclear DNA (), respectively.
Our fluorescence spectroscopic study investigated type of reactive species or molecules produced by GNP–MB conjugate in PDT that are responsible for morphological as well as cytological perturbation of Candida cells. On 660-nm excitation, GNP (0.2 mg/mL) quenched the weak fluorescence of MB at 684 nm () and acted as a quencher. It was previously reported that GNP acts as a quencher as well as enhancer of fluorophores, and these two opposite effects are due to various factors, as described elsewhere. Citation71 Primarily, GNP-mediated quenching of fluorophore is caused by two phenomena, nonradiative energy transfer from fluorophore to GNP and collision dynamics between GNP and fluorophore, as described earlier.Citation72,Citation73 In conjugate, MB relaxes to ground state by nonradiative energy decay and forms free radical species such as hydroxyls and superoxides, as described previously.Citation31 Consequently, this free hydroxyl radical production addresses the type I phototoxicity, as described earlier.Citation21
Conclusion
This study investigated antimicrobial and antibiofilm activity of GNP–MB conjugate against Candida biofilm. SEM, TEM, and CLSM micrographs show the detrimental effects of conjugate, such as perturbation of Candida biofilm, cell wall integrity, nuclear disintegration, and metabolic viability. Quenching of fluorescence of MB in GNP–MB conjugate confirms type I phototoxicity against Candida biofilm by producing hydroxyl free radicals, as shown in the proposed model (). This is the first time we have developed an alternative, efficient, and novel GNP–MB-mediated Candida antibiofilm PDT approach. Microbial biofilm is one of the most important factors in the worldwide increase of multiple drug-resistant pathogens. Moreover, Candida biofilm on medical implants and prosthetic devices necessitates immediate replacement and thus increased treatment cost. Therefore, this novel approach may be a good alternative to control infection in hospital and clinical settings.
Figure 9 Schematic overview of conjugation of photosensitizer MB with GNP.
Notes: Specific 660-nm wavelength of diode laser transits MB of GNP–MB conjugate to an excited state. Nonradiative energy decay by GNP of MB causes the transition to ground state, which results in the formation of hydroxyl free radicals in the presence of molecular oxygen and substrates around the vicinity of the conjugate (type I phototoxicity).
Abbreviations: GNP, gold nanoparticle; MB, methylene blue.
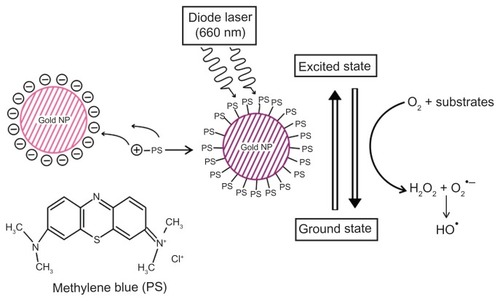
Acknowledgments
The authors would like to acknowledge Drs Gajender Saini and Ruchita Pal, Advanced Instrumentation Research Facility, JNU, for providing TEM and SEM instrumental support. The authors would also like to acknowledge DBT, government of India, for the support and internal facilities of the department. This work was supported by internal funds of Biotechnology Unit, AMU and DBT grant, BT/PR11453/BID/07/296/2009 to AUK.
Disclosure
The authors report no conflicts of interest in this work
References
- DonlanRMBiofilms: microbial life on surfacesEmerg Infect Dis2002888189012194761
- CostertonJWLewandowskiZCaldwellDEKorberDRLappin-ScottHMMicrobial biofilmsAnnu Rev Microbiol1995497117458561477
- DouglasLJMedical importance of biofilms in Candida infectionsRev Iberoam Micol20021913914312825991
- DouglasLJCandida biofilms and their role in infectionTrends Microbiol200311303612526852
- MacCallumDCandida albicans: New Insights in Infection, Disease and TreatementKavanaghKNew Insights in Medical MycologyDordrechtSpringer2007
- PfallerMADiekemaDJEpidemiology of invasive candidiasis: a persistent public health problemClin Microbiol Rev20072013316317223626
- FidelPLJrCandida-host interactions in HIV disease: relationships in oropharyngeal candidiasisAdv Dent Res200619808416672555
- KojicEMDarouicheROCandida infections of medical devicesClin Microbiol Rev20041725526715084500
- EdmondMBWallaceSEMcClishDKPfallerMAJonesRNWenzelRPNosocomial bloodstream infections in United States hospitals: a three-year analysisClin Infect Dis19992923924410476719
- AslamSEffect of antibacterials on biofilmsAm J Infect Control200836S17519084156
- BaillieGSDouglasLJMatrix polymers of Candida biofilms and their possible role in biofilm resistance to antifungal agentsJ Antimicrob Chemother20004639740310980166
- BaillieGSDouglasLJEffect of growth rate on resistance of Candida albicans biofilms to antifungal agentsAntimicrob Agents Chemother199842190019059687381
- LaFleurMDKumamotoCALewisKCandida albicans biofilms produce antifungal-tolerant persister cellsAntimicrob Agents Chemother2006503839384616923951
- RamageGBachmannSPattersonTFWickesBLLopez-RibotJLInvestigation of multidrug efflux pumps in relation to fluconazole resistance in Candida albicans biofilmsJ Antimicrob Chemother20024997398012039889
- KuhnDMGhannoumMACandida biofilms: antifungal resistance and emerging therapeutic optionsCurr Opin Investig Drugs20045186197
- VediyappanGRossignolTd’EnfertCInteraction of Candida albicans biofilms with antifungals: transcriptional response and binding of antifungals to beta-glucansAntimicrob Agents Chemother2010542096211120194705
- HugoWBRussellADHugo and Russell’s Pharmaceutical Microbiology7th edMaldenBlackwell Science2004
- MuninEGiroldoLMAlvesLPCostaMSStudy of germ tube formation by Candida albicans after photodynamic antimicrobial chemotherapy (PACT)J Photochem Photobiol B200788162017566757
- PeloiLSSoaresRRBiondoCESouzaVRHiokaNKimuraEPhotodynamic effect of light-emitting diode light on cell growth inhibition induced by methylene blueJ Biosci20083323123718535357
- MacDonaldIDoughertyTJBasic principles of photodynamic therapyJ Porphyr Phthalocyanines20015105129
- CastanoAPDemidovaTNHamblinMRMechanisms in photodynamic therapy: part one – photosensitizers, photochemistry and cellular localizationPhotodiagnosis Photodyn Ther20041279293
- WoodSNattressBKirkhamJAn in vitro study of the use of photodynamic therapy for the treatment of natural oral plaque biofilms formed in vivoJ Photochem Photobiol B1999501710443029
- SoukosNSSocranskySSMulhollandSELeeSDoukasAGPhotomechanical drug delivery into bacterial biofilmsPharm Res20001740540910870983
- BhattiMMacRobertAMeghjiSHendersonBWilsonMA study of the uptake of toluidine blue O by Porphyromonas gingivalis and the mechanism of lethal photosensitizationJ Photochem Photobiol199868370376
- HuMChenJLiZYGold nanostructures: engineering their plasmonic properties for biomedical applicationsChem Soc Rev2006351084109417057837
- HanGGhoshPRotelloVMFunctionalized gold nanoparticles for drug deliveryNanomedicine2007211312317716197
- PissuwanDValenzuelaSMMillerCMCortieMBA golden bullet? Selective targeting of Toxoplasma gondii tachyzoites using antibody-functionalized gold nanorodsNano Lett200773808381218034505
- HuangWCTsaiPJChenYCFunctional gold nanoparticles as photothermal agents for selective-killing of pathogenic bacteriaNanomedicine (Lond)2007277778718095845
- WilliamsDNEhrmanSHHolomanTRPEvaluation of the microbial growth response to inorganic nanoparticlesJ Nanobiotechnology20064316507102
- NarbandNUppalMDunnillCWHyettGWilsonMParkinIPThe interaction between gold nanoparticles and cationic and anionic dyes: enhanced UV-visible absorptionPhys Chem Chem Phys200911105131051819890539
- NarbandNTubbySParkinIPGold nanoparticles enhance the toluidine blue-induced lethal photosensitisation of Staphylococcus aureusCurr Nanosci20084409414
- TurkevichJStevensonPCHillierJA study of the nucleation and growth processes in the synthesis of colloidal goldDiscuss Faraday Soc1951115575
- FrensGControlled nucleation for the regulation of the particle size in monodisperse gold suspensionsNature19732412022
- TobudicSKratzerCLassniggAGraningerWPresterEIn vitro activity of antifungal combinations against Candida albicans biofilmsJ Antimicrob Chemother20106527127419996142
- DjordjevicDWiedmannMMcLandsboroughLAMicrotiter plate assay for assessment of Listeria monocytogenes biofilm formationAppl Environ Microbiol2002682950295812039754
- JinYZhangTSamaranayakeYHFangHHPYipHKSamaranayakeLPThe use of new probes and stains for improved assessment of cell viability and extracellular polymeric substances in Candida albicans biofilmsMycopathologia200515935336015883718
- OsumiMThe ultrastructure of yeast: cell wall structure and formationMicron1998292072339684351
- HawserSPDouglasLJBiofilm formation by Candida species on the surface of catheter materials in vitroInfect Immun1994629159218112864
- ChandraJKuhnDMMukherjeePKHoyerLLGhannoumMABiofilm formation by the pathogen C. albicans: development, architecture, and drug resistanceJ Bacteriol20011835385539411514524
- LinkSEl-SayedMAShape and size dependence of radiative, nonradiative and photothermal properties of gold nanocrystalsInt Rev Phys Chem200019409453
- NoginovMAZhuGBahouraMThe effect of gain and absorption on surface plasmons in metal nanoparticlesAppl Phys B200686455460
- AmendolaVMeneghettiMSize evaluation of gold nanoparticles by UV-vis spectroscopyJ Phys Chem C200911342774285
- DongSTangCZhouHZhaoHPhotochemical synthesis of gold nanoparticles by the sunlight radiation using a seeding approachGold Bull20043734
- LuoYFormation of submicrometer-scale gold nanoparticle aggregates and their self–organization into “supracrystals”Colloid J200769391393
- HuJWangZLiJGold nanoparticles with special shapes: controlled synthesis, surface-enhanced Raman scattering, and the application in biodetectionSensors2007732993311
- LeeSKMillsANovel photochemistry of leuco-methylene blueChem Commun20031823662367
- PittsBHamiltonMAZelverNStewartPSA microtiterplate screening method for biofilm disinfection and removalJ Microbiol Methods20035426927612782382
- NiuCGilbertESColorimetric method for identifying plant essential oil components that affect biofilm formation and structureAppl Environ Microbiol2004706951695615574886
- MillardPJRothBLYueSTHauglandRPDevelopment of the FUN-1 family of fluorescent probes for vacuole labeling and viability testing of yeastsAppl Environ Microbiol199763289729059212436
- MandalDKBrewerCFDifferences in the binding affinities of dimeric concanavalin A (including acetyl and succinyl derivatives) and tetrameric concanavalin A with large oligomannose-type glycopeptidesBiochemistry199318511651208494887
- DarzynkiewiczZTraganosFKapuscinskiJStaiano-CoicoMelamedMRAccessibility of DNA in situ to various fluorochromes: relationship to chromatin changes during erythroid differentiation of Friend leukemia cellsCytometry198453553636468176
- DonlanRMCostertonJWBiofilms: survival mechanisms of clinically relevant microorganismsClin Microbiol Rev20021516719311932229
- DanielMCAstrucDGold nanoparticles: assembly, aupramolecular chemistry, quantum-size-related properties, and applications toward biology, catalysis, and nanotechnologyChem Rev200410429334614719978
- ParakWJGerionDPellegrinoTBiological applications of colloidal nanocrystalsNanotechnology200314R1527
- HolisterPWeenerJWRomas VasCHarperTNanoparticles: Technology White Papers 3IlkleyCientific2003
- LiJWangXWangCThe enhancement effect of gold nanoparticles in drug delivery and as biomarkers of drug-resistant cancer cellsChem Med Chem2007237437817206735
- TamFGoodrichGPJohnsonBRHalasNJPlasmonic enhancement of molecular fluorescenceNano Lett2007749650117256995
- GlommWRFunctionalized gold nanoparticles for applications in bionanotechnologyJ Dispers Sci Technol200526389414
- ConnorEEMwamukaJGoleAMurphyCJWyattMDGold nanoparticles are taken up by human cells but do not cause acute cytotoxicitySmall2005132532717193451
- PernodetNFangXSunYAdverse effects of citrate/gold nanoparticles on human dermal fibroblastsSmall2006676677317193121
- BuryginGLKhlebtsovBNShantrokhaANDykmanLABogatyrevVAKhlebtsovNGOn the enhanced antibacterial activity of antibiotics mixed with gold nanoparticlesNanoscale Res Lett2009479480120596384
- PagonisTCChenJFontanaCRNanoparticle-based endodontic antimicrobial photodynamic therapyJ Endod20103632232820113801
- UsachevaMNTeichertMCBielMAComparison of the methylene blue and toluidine blue photobactericidal efficacy against gram-positive and gram-negative microorganismsLasers Surg Med20012916517311553906
- de SouzaSCJunqueiraJCBalducciIKoga-ItoCYMuninEJorgeAOCPhotosensitization of different Candida species by low power laser lightJ Photochem Photobiol B200683343816413196
- GiroldoLMFelipeMPde OliveiraMAMuninEAlvesLPCostaMSPhotodynamic antimicrobial chemotherapy (PACT) with methylene blue increases membrane permeability inCandida albicansLasers Med Sci20092410911218157564
- WilsonMMiaNSensitization of Candida albicans to killing by low-power laser lightJ Oral Pathol199322354357
- XuYEndodontic antimicrobial photodynamic therapy:safety assessment in mammalian cell culturesJ Endod2009351567157219840649
- TsaiSWLiawJWHsuFYChenYYLyuMJYehMHSurface- modified gold nanoparticles with folic acid as optical probes for cellular imagingSensors2008866606673
- AndersonKYoungSJiangHPlasma enhanced copolymerization of amino acid and synthetic monomersLangmuir2012281833184522176716
- ZhangXWangSShenQThe electrochemical behavior of p-aminophenol at a ω-mercaptopropionic acid self-assembled gold electrodeMicrochimica Acta20051493742
- KangKAWangJJasinskiJBAchilefuSFluorescence manipulation by gold nanoparticles: from complete quenching to extensive enhancementJ Nanobiotechnology201191621569249
- DulkeithEMorteaniACNiedereichholzTFluorescence quenching of dye molecules near gold nanoparticles: radiative and nonradiative effectsPhys Rev Lett20028920300212443474
- DubertretBCalameMLibchaberAJSingle-mismatch detection using gold-quenched fluorescent oligonucleotidesNat Biotechnol20011936537011283596