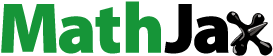
Abstract
Objective
Brucine was encapsulated into stealth liposomes using the ammonium sulfate gradient method to improve therapeutic index.
Materials and methods
Four brucine stealth liposomal formulations were prepared, which were made from different phosphatidylcholines (PCs) with different phase transition temperatures (Tm). The PCs used were soy phosphatidylcholine (SPC), dipalmitoyl phosphatidylcholine (DPPC), hydrogenated soy phosphatidylcholine (HSPC), and distearoyl phosphatidylcholine (DSPC). The stabilities, pharmacokinetics, and toxicities of these liposomal formulations were evaluated and compared.
Results
Size, zeta potential, and entrapment efficiency of brucine-loaded stealth liposomes (BSL) were not influenced by PC composition. In vitro release studies revealed that drug release rate increased with decreased Tm of PCs, especially with the presence of rat plasma. After intravenous administration, the area under the curve (AUC) values of BSL-SPC, BSL-DPPC, BSL-HSPC, and BSL-DSPC in plasma were 7.71, 9.24, 53.83, and 56.83-fold as large as that of free brucine, respectively. The LD50 values of brucine solution, BSL-SPC, BSL-DPPC, BSL-HSPC, and BSL-DSPC following intravenous injection were 13.17, 37.30, 37.69, 51.18, and 52.86 mg/kg, respectively. It was found in calcein retention experiments that the order of calcein retention in rat plasma was SPC < DPPC << HSPC < DSPC stealth liposomes.
Conclusion
PC composition could exert significant influence on the stabilities, pharmacokinetics, and toxicities of brucine-loaded stealth liposomes. DSPC or HSPC with Tm above 50°C should be used to prepare the stealth liposomal formulation for the intravenous delivery of brucine. However, it was found in the present paper that the pharmacokinetics and toxicity of BSL were not influenced by the PC composition when the Tm of the PC was in the range of −20°C to 41°C.
Introduction
Brucine, originally isolated by Pelletier and Caventou in 1819 from nux-vomica,Citation1 is a white, odorless, crystalline solid with a molecular weight of 394.45. Antiproliferative and cytotoxic effects of brucine have been reported in HepG2,Citation2 SMMC-7721Citation3 hepatoma cells, and multiple myeloma RPMI 8226 cell lines.Citation4 Further studyCitation5 revealed that brucine produced time- and dose-dependent inhibition of MCF-7 cells in vitro and Ehrlich ascites carcinoma tumors in vivo, and that, furthermore, it could reduce peritoneal angiogenesis and microvessel density in vivo. Although brucine has been shown to possess an impressive preclinical profile in the pharmacological studies, severe central nervous system toxicity is the major obstacle to its clinical application. There is a narrow margin of safety between a therapeutic and a toxic dose. Higher doses of brucine will cause such symptoms as violent convulsion, significant increase in blood pressure, and even lethal poisoning.Citation6
Liposomes have previously been used as carriers for antitumor drugs, and they have shown a significant effect in decreasing the extent and types of nonspecific toxicities. In addition, liposomes also increase the amount of drug that can be effectively delivered to the tumor. In the early 1990s, grafting polyethylene glycol (PEG) onto liposomes was invented to avoid the uptake of the mononuclear phagocyte system, resulting in improved pharmacokinetics in vivo. The term “stealth” was applied to these PEGylated liposomes because of their ability to evade interception by the mononuclear phagocyte system, much in the same way the stealth bomber is able to evade radar. The main properties of stealth liposomes are their significantly improved pharmacokinetics and thus enhanced residence in the blood.Citation7 If brucine is encapsulated into the stealth liposomes, it mainly stays in the blood circulation since stealth liposomes cannot pass the biomembrane directly. Therefore, the distribution of brucine into the brain can be avoided and the acute toxicity of brucine can be reduced subsequently.
It is evident that the phosphatidylcholine (PC) used to prepare liposomes has a significant effect on the stability of the resulting liposomes. In the early 1980s, it was demonstrated that liposomes obtained from a PC with a high transition temperature (Tm) were more stable in the blood than liposomes prepared from a PC with a low Tm.Citation8 PC is an important consideration with liposome stability, and the state of liposomes at physiological temperature, for instance, can be altered by the inclusion of PCs with different Tms.
For stealth liposomes, different PCs possessing different Tms have been used to prepare liposome formulations. Hydrogenated soy phosphatidylcholine (HSPC, Tm =50°C) was recently used to make flavopiridol-loaded stealth liposomesCitation9 and its in vivo pharmacokinetics were significantly improved compared to the free drug. Dipalmitoyl phosphatidylcholine (DPPC) was used to prepare gemcitabine-loaded stealth liposomes and their antitumor activity at 5 mg/kg was equal to the free drug at the dose of 50 mg/kg.Citation10 CKD-602, a camptothecin analogue, was encapsulated into stealth liposomes composed of distearoyl phosphatidylcholine (DSPC). Compared to the free drug, the AUC in plasma after intravenous administration of CKD-602-containing DPPC stealth liposomes increased about 22-fold.Citation11
Since stealth liposomes composed of different PCs with different Tms were all reported to improve in vivo behavior, it is necessary to evaluate the effect of PC on the stability, pharmacokinetic behavior, and toxicity of brucine-loaded stealth liposomes.
As for brucine, the stability of stealth liposomes is the key factor which is directly associated with toxicity. If the stealth liposomes are instable in the blood circulation, brucine can be rapidly leaked from the carrier and distribution into the brain cannot be avoided. Therefore, PC composition seems to have a significant effect on the toxicity of brucine stealth liposomes following intravenous administration.
In the present paper, four different PCs – soy phosphatidylcholine (SPC), DPPC, HSPC, and distearoyl phosphatidylcholine (DSPC) – with Tm in the range of −20°C to 55°C were used to prepare brucine-loaded stealth liposomes (BSL). Their stabilities, pharmacokinetics, and acute toxicities were investigated and compared.
Materials and methods
Materials
SPC, DPPC, HSPC, DSPC, and 1,2-distearoyl-sn-glycero-3-phosphoethanolamine- N-(carbonyl-methoxypolyethyleneglycol-2000) (DSPE-PEG2000) were purchased from Lipoid Corp (Ludwigshafen, Germany). Cholesterol was purchased from Huixing Biochemistry Reagent Company (Shanghai, China). Reference substances of brucine and the internal standard (IS) huperzine A were supplied by the National Institute for the Control of Pharmaceutical and Biological Products (Beijing, China). The purities of brucine (95.9%) and huperzine A (98.0%) were determined by high-performance liquid chromatography (HPLC) analysis. Brucine and calcein were obtained from Sigma-Aldrich (St Louis, MO). Sephadex G-50 was purchased from Pharmacia Biotech (Uppsala, Sweden). HPLC-grade acetonitrile was obtained from Tedia Company Inc. (Fairfield, OH). All other reagents were of analytical grade.
Animals
Male Sprague-Dawley rats, weighing 200–250 g, were purchased from Shanghai Slac Laboratory Animal Co, Ltd (Shanghai, China). Toxicity experiments were performed with male ICR mice, weighing 18–22 g, also obtained from the Slac Laboratory Animal Co, Ltd. The animals were acclimatized for at least 1 week in a 12-hour light/dark cycle with free access to standard food and water. According to the requirement of the National Act on the Use of Experimental Animals (People’s Republic of China), the protocols of the animal experiments were approved by the Animal Ethics Committee of Nanjing University of Chinese Medicine.
Preparation of liposomes
The BSLs were prepared by the ammonium sulfate gradient loading method as described previously.Citation12 Briefly, the mixture of PC, cholesterol and DSPE-PEG2000 at a molar ratio of 15:5:1 were dissolved in 2 mL ethanol and then injected into a solution of 5 mL 200 mol/L ammonium sulfate under magnetic stirring at 60°C. The ethanol was evaporated to no odor under vacuum, and then water was added to adjust the volume of final liposome suspension to 5 mL. To get homogenous liposome suspensions, liposomes were ultrasonically treated for 10 minutes, using a JY92-2 probe ultrasonicator (Xinzhi Biotechnology Co, Ltd, Ningbo, China) equipped with a tapered microtip. The resultant liposomes were filtered through a 0.22 μm micropore filter to remove the titanium fragments. The transmembrane ammonium sulfate gradient was created by four consecutive dialysis exchanges (2 hours at a time) against 100 mL of phosphate buffer solution (PBS) (pH = 7.4) at room temperature. Brucine in powder form in a drug/lipid mass ratio of 1:12 was added to liposomes at 60°C for 20 minutes.
A total of four formulations were prepared which were named BSL-SPC, BSL-DPPC, BSL-HSPC, and BSL-DSPC. The main difference among these formulations was PC composition (see for more information).
Figure 1 The characterization of brucine-loaded stealth liposomes with different PC composition.
Abbreviations: PC, phosphatidylcholine; BSL, brucine-loaded stealth liposomes; SPC, soy phosphatidylcholine; DPPC, dipalmitoyl phosphatidylcholine; HSPC, hydrogenated soy phosphatidylcholine; DSPC, distearoyl phosphatidylcholine.
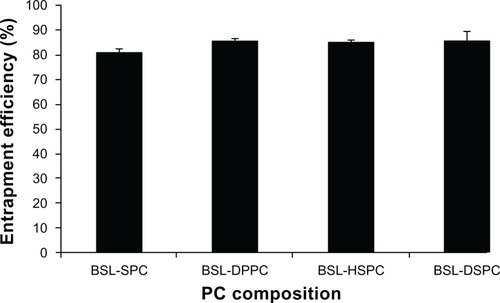
Vesicle size and zeta potential measurement
The particle sizes and zeta potentials of BSLs were evaluated in triplicate by dynamic light scattering (DLS), using a Zeta Potential Analyzer (Brookhaven Instruments Corporation, Holtsville, NY).
Entrapment efficiency
The entrapment efficiency (EE) was calculated by the percentage of brucine encapsulated in stealth liposomes relative to the total amount of brucine (encapsulated and free) in stealth liposome suspension. To determine the total amount of brucine in stealth liposome suspension, 0.5 mL brucine stealth liposome was disrupted by the addition of 2 mL ethanol-isopropanol (1:4, v/v) to form a clear solution. The concentration of brucine was assayed by UV spectroscopy (TU1800S, Puxi Instrument Company, Beijing, China) at 264 nm compared to a standard curve.
To completely remove nonentrapped drug, BSLs were passed through a Sephadex G50 column (1 × 27 cm; Pharmacia Biotech Corp., Uppsala, Sweden) equilibrated with PBS (pH 7.4). The resultant PBS fraction containing the stealth liposomes free of nonentrapped brucine was collected and the entrapped brucine concentration was determined as described above.
EE was calculated using the following equation:
HPLC analysis of brucine in PBS
An HPLC system (Shimadzu Corporation, Kyoto, Japan) consisting of a LC-20AT pump and a SPD-20A UV-VIS detector was used for the assay of brucine. The mobile phase consisted of acetonitrile and buffer (10 mm sodium heptane sulfonate and 20 mm potassium dihydrogen phosphate, pH adjusted to 2.8 with 10% phosphonic acid). The ratio of acetonitrile/buffer (v/v) was adjusted to 24:76. Separation was carried out at 35°C using a reverse-phase C18 column (Lichrospher, 5 μm, 4.6 mm × 250 mm, Hanbang Corp, Huaian, China). The detection wavelength was 264 nm and a flow rate of 1.0 mL/minute was employed. A sample volume of 20 μL was injected.
Calibration curves of brucine in PBS were linear in the range of 0.4–12.8 mg/L. The regression coefficient was 0.9994. Intra-day and inter-day precisions of brucine were no more than 1.50%.
Drug release study
The in vitro release of BSLs with different PC composition was analyzed according to the published method.Citation12 Non-entrapped drug was removed from BSLs over a Sephadex G-50 column as described above before an in vitro release test. The BSL suspension (drug content: 2 mg) was placed in a dialysis bag with a molecular weight cut-off of 10,000. The dialysis bag was suspended in 100 mL isotonic PBS (pH 7.4) which was incubated at 37°C under constant rotation at 500 rpm. At scheduled time intervals, aliquot samples were withdrawn and assayed for brucine content by HPLC as described above. The volume of dissolution medium was maintained at 100 mL throughout the experiment. To evaluate the effect of plasma albumin on drug release from stealth liposomes, brucine stealth liposome suspension combined with fresh rat plasma (1:1, v/v) was placed in the dialysis bag.
In vivo pharmacokinetic studies
Rats were randomized into groups of five and six. Five groups of rats were given a single dose of 2.5 mg/kg brucine solution (dissolved in PBS), BSL-SPC, BSL-DPPC, BSL-HSPC, and BSL-DSPC by tail vein injection. At predetermined intervals (5, 10, 20, 40, 60, 90, 120, 180, 240, and 360 minutes), the rats were anaesthetized using ether and blood samples were collected from the retro-orbital plexus into heparinized microfuge tubes. The blood samples were then immediately centrifuged at 10,000 rpm for 2 minutes to obtain the plasma samples, which were then stored at −20°C until HPLC analysis.
Sample preparation
Plasma samples were treated using the liquid-liquid extraction step described previously.Citation13 Briefly, 100 μL plasma were spiked with 10 μL IS (40 μg/mL huperzine A solution in methanol). 10 μL of aqueous ammonia was added and the samples were basified. 3 mL n-hexane-dichloromethane-isopropanol (65:30:5, v/v/v) was then added. The mixture was vortex-mixed for 3 minutes and subsequently centrifuged for 10 minutes at 4000 rpm at ambient temperature. The organic layer was transferred into a glass tube and the residue was re-extracted in a similar manner. The combined organic layer was evaporated at 50°C under a gentle stream of nitrogen gas, until a completely dried residue was left. The residue was reconstituted in 100 μL methanol and centrifuged for 5 minutes at 12,000 rpm. Finally, 50 μL of the supernatant were injected into HPLC system for analysis.
HPLC analysis of plasma samples
HPLC analysis of treated plasma samples were performed using a HPLC-UV system (Shimadzu Corporation, Kyoto, Japan) consisting of a LC-20AT pump and a SPD-20A UV detector. Data was collected by a HPLC chromatography workstation (N2000, Intelligence and Information Institute of Zhejiang University, Hangzhou, China). Chromatographic separation was achieved on a Kromasil C18 analytical column (4.6 mm × 250 mm, 5 μm, Kromasil, Sweden) coupled with a C18 guard cartridge (4.6 mm × 10 mm, 5 μm, Hanbang Corp), maintained at 35°C. The mobile phase consisted of 24% acetonitrile and 76% buffer (isometric mixture of 10 mM sodium heptane sulfonate and 20 mM potassium dihydrogen phosphate, the pH value was adjusted to 2.8 with 10% phosphoric acid). The prepared mobile phase was filtered using a vacuum filter system equipped with 0.45 μm filter and delivered at a flow rate of 1.0 mL/minute. The detection wavelength was set at 264 nm.
Statistical analysis and calculation of pharmacokinetic parameters
The chromatographic data were automatically processed to obtain the peak-area ratios of compound to IS and fitted to a weighted (1/C2) linear regression relationship. The pharmacokinetic parameters were calculated using non-compartmental analysis of data. The data are presented as means with standard deviations (SD) for the individual groups. Statistically significant differences were assessed by Student’s t-test with the level of statistical significance set at P < 0.05.
The following pharmacokinetic parameters were calculated using noncompartmental analysis of data (Drug and Statistics version 2.0 Program, Shanghai, China): the area under the plasma concentration–time curve from time zero to time infinity (AUC0–∞), the area under the plasma concentration–time curve from time zero to the last time point (AUC0–t), the total body clearance (Cl), the mean residence time (MRT), the terminal elimination half-life (t½) and the apparent volume of distribution (V).
Acute toxicity test
Brucine was dissolved in pH 7.4 PBS and the resulting brucine solution was obtained. BSLs with different PC compositions had concentrations of 2.0 mg/mL and EEs higher than 80%. The maximal and minimal lethal doses were preliminarily estimated. The dosages for determination of the median lethal dose (LD50) were then calculated and intravenously administered to five dosage groups (10 mice each). All mice were observed for general symptoms. The number of dead mice was recorded. LD95, LD50, and LD5 values were calculated using the Bliss method.Citation14
Evaluation of membrane permeability by calcein release measurements in vitro
Because the release of calcein from liposomes can be determined without the use of dialysis bags, calcein is usually used to evaluate membrane stability. Calcein-loaded liposomes with different PC compositions were prepared by the thin film hydration method.Citation15 The mixture of PC, cholesterol, and DSPE-PEG2000 at a molar ratio of 15:5:1 was dissolved in chloroform in a round-bottom flask. The solvent was evaporated using a rotary evaporator to form a thin layer of lipid in the flask. 5 mL of 90 mM calcein (pH 7.4) was added and the lipid mixture was hydrated at 60°C for 40 minutes to form multilamellar vesicles (MLV). MLVs were then sonicated using a JY92-2 probe ultrasonicator (Xinzhi Biotechnology Co, Ltd, Ningbo, China) equipped with a tapered microtip. The resulting liposomes were filtered through a 0.22 μm micropore filter to remove the titanium fragments. Unencapsulated calcein was removed by gel filtration using Sephadex G50 column (1 × 27 cm) with pH7.4 phosphate buffer saline (PBS) as eluent at a flow rate of 1 mL/minute.
Calcein-loaded liposomes (100 μL) were added to 2.9 mL rat plasma/PBS medium (1:1) and incubated at 37°C under constant stirring. At predetermined time points, aliquot samples were withdrawn and diluted. After rapid cooling in an ice-bath, the fluorescence of the sample was measured in a fluorimeter (F96S; Lengguang, Shanghai, China) using 490 nm excitation and 515 nm emission wavelengths. Complete release of calcein was achieved by the addition of Triton X-100 (Amresco LLC, Solon, OH) (final concentration, 1%). The percentage of calcein release at different time points was calculated using the following equation:
Where Ft is the fluorescence at time point, F0 is the initial fluorescence of calcein liposomes at the start of the incubation time, and F100 is the fluorescence of Triton X-100 treated samples.
Results and discussion
Characterization of brucine-loaded stealth liposomes
The particle sizes of BSL-SPC, BSL-DPPC, BSL-HSPC, and BSL-DSPC are shown in . The PC composition had little effect on the size of BSLs. The polydispersity index was lower than 0.25 for all BSLs, indicating that liposome population was homogenous in size. The results of zeta potential measurements revealed that four liposomal formulations were all electric neutral.
The effect of PC composition on EE of stealth liposomes at a drug-to-lipid mass ratio of 1:12 is shown in . The EE of BSLs was not influenced by PC composition and all EEs were higher than 80%.
Figure 2 Effect of phosphatidylcholine composition on entrapment efficiency of brucine-loaded stealth liposomes.
Note: n = 3.
Abbreviations: BSL, brucine-loaded stealth liposomes; SPC, soy phosphatidylcholine; DPPC, dipalmitoyl phosphatidylcholine; HSPC, hydrogenated soy phosphatidylcholine; DSPC, distearoyl phosphatidylcholine.

Drug release in vitro
HPLC chromatograms are shown in . The in vitro drug release profiles are shown in and . Without the existence of rat plasma, the drug release ratios of BSL-SPC, BSL-DPPC, BSL-HSPC, and BSL-DSPC in 10 hours were 10.52% ± 0.47%, 13.25% ± 0.81%, 6.08% ± 1.25%, and 6.43% ± 1.55%, respectively. With the presence of rat plasma, the release of brucine was accelerated dramatically and a large extent of drug release was observed. The retention of the loaded brucine in the stealth liposomes was more significantly dependent on PC compositions with different Tm. Most encapsulated brucine (80.92% ± 1.94%) had been released from BSL-SPC. In comparison, the other three BSLs improved drug retention significantly. For BSLs with higher Tm, only 30.71% ± 5.23%, 15.56% ± 1.04%, and 17.34% ± 0.95% released from BSL-DPPC, BSL-HSPC, and BSL-DSPC at 10 hours, respectively. It was evident that the extent of drug retention in BSLs corresponded to the Tm of PC, even though the release of BSL-DPPC was markedly higher than compared to BSL-SPC in the initial 4 hours.
Figure 3 HPLC chromatographs of brucine in PBS.
Note: Peak 1 represents brucine.
Abbreviations: HPLC, high-performance liquid chromatography; PBS, phosphate buffer solution.
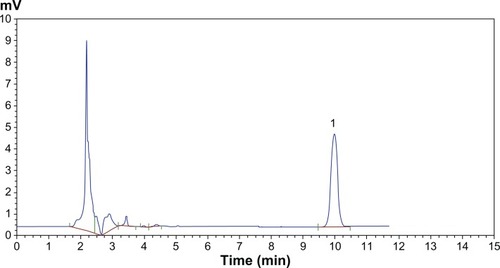
Figure 4 In vitro release profiles of brucine from brucine-loaded stealth liposomes with different phase transition temperature.
Notes: mean ± SD; n = 3.
Abbreviations: BSL, brucine-loaded stealth liposomes; SPC, soy phosphatidylcholine; DPPC, dipalmitoyl phosphatidylcholine; HSPC, hydrogenated soy phosphatidylcholine; DSPC, distearoyl phosphatidylcholine; SD, standard deviation.
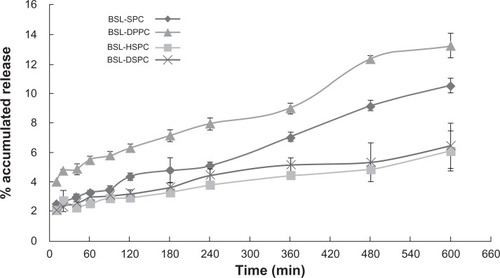
Figure 5 In vitro release profiles of brucine from brucine-loaded stealth liposomes with different phase transition temperature in the presence of rat plasma.
Notes: mean ± SD, n = 3.
Abbreviations: BSL, brucine-loaded stealth liposomes; SPC, soy phosphatidylcholine; DPPC, dipalmitoyl phosphatidylcholine; HSPC, hydrogenated soy phosphatidylcholine; DSPC, distearoyl phosphatidylcholine; SD, standard deviation.
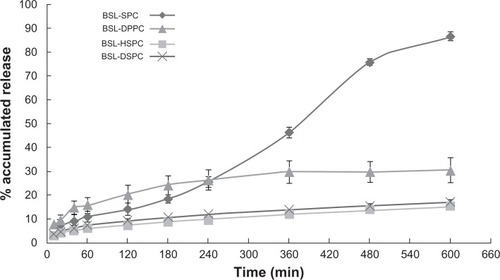
For stealth liposomes, the effect of lipid composition on the stability in vitro was investigated. Irinotecan was encapsulated into HSPC, DSPC, or egg phosphatidylcholine (EPC)-based stealth liposomes and the drug leakage profiles of these liposome formulations were compared.Citation16 Stealth liposomes that contained EPC of a lower Tm (approximately −20°C) were found to be highly unstable as about 95% irinotecan was leaked in 240 minutes. In contrast, liposomes that were composed of lipids with higher Tm (approximately 50°C–60°C), HSPC and DSPC, were significantly more stable under the experimental condition. Only 50%–60% of the encapsulated drug was leaked in 240 minutes. It was concluded that the lipid membranes consisting of PCs with lower Tm exhibit higher fluidity compared to membranes consisting of PCs with higher Tm.
It should be noted that preparation methods influence the release profiles as well. For brucine-loaded HSPC liposomes prepared by ethanol-dripping method and ammonium sulfate gradient loading method, there were about 68%Citation17 and 6% brucine (in the present paper) released into PBS after 10 hours.
In the present study, the stability of BSLs with different PC compositions was examined by drug release kinetics in vitro. The results of the drug release test without the presence of rat plasma revealed that nearly 85%–95% of the encapsulated drug was retained inside the liposomes in 10 hours. One possible explanation for the good drug retention is that brucine forms a gel-like precipitate with ammonium sulfate as doxorubicin does because of the lower solubility of the brucine sulfate salt formed in the intra-liposomal aqueous phase. It was well known that serum components destabilize liposomal membranes.Citation18 Phospholipids exchange and transfer to lipoproteins, which can destabilize and disintegrate liposomes. Therefore, with the presence of rat plasma, the release of brucine was significantly increased. Compared with BSL-SPC, the other three BSLs were proved to possess better drug retention. The good stability of BSLs with higher Tm may be attributed to the enhanced bilayer rigidity. Thus BSLs with higher Tm showed a significant decrease in release rate and an increase in resistance to release. However, if Tm was higher than 50°C, the PC composition effect on the stability of BSL was no longer significant.
In vivo pharmacokinetic studies
The semi-logarithmic plasma concentration-time profiles of brucine following intravenous administration of 2.5 mg/kg brucine solution and four BSLs are shown in . The pharmacokinetic parameters are summarized in . As seen in and , after encapsulation into stealth liposomes, the pharmacokinetics of brucine were significantly improved. In comparison with free brucine, the AUC0–ts of BSL-SPC, BSL-DPPC, BSL-HSPC, and BSL-DSPC increased 7.71-, 9.24-, 53.83-, and 56.83-fold, respectively. The t½ values of BSLs were also increased. It was obvious that the pharmacokinetics of BSL-SPC and BSL-DPPC were similar and no statistical significance was found in all pharmacokinetic parameters of these two groups as shown in . It appeared that the pharmacokinetic characteristics of BSL were not influenced by the PC composition when the Tm of the PC was in the range of −20°C to 41°C. The pharmacokinetics of BSL-HSPC and BSL-DSPC with higher Tm were also similar. Compared to BSL-SPC and BSL-DPPC, BSL-HSPC and BSL-DSPC significantly increased AUC and remarkably reduced Cl (P < 0.05). Therefore, the pharmacokinetics of BSL seemed to be improved significantly with the increase of the Tm in the PC from 41°C to 50°C.
Table 1 Pharmacokinetic parameters of brucine after intravenous administration at a single dose of 2.5 mg/kg of brucine solution or brucine-loaded stealth liposomes with different phase transition temperature in rats
Figure 6 Plasma concentration-time profiles of brucine after intravenous administration at a single dose of 2.5 mg/kg of brucine solution or brucine-loaded stealth liposomes with different phase transition temperature in rats.
Note: n = 6.
Abbreviations: BSL, brucine-loaded stealth liposomes; SPC, soy phosphatidylcholine; DPPC, dipalmitoyl phosphatidylcholine; HSPC, hydrogenated soy phosphatidylcholine; DSPC, distearoyl phosphatidylcholine.
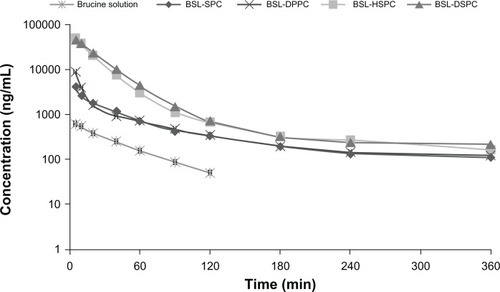
The relationship between the Tm of PC and the AUC0-t of the corresponding BSLs after intravenous administration were shown in . When the Tm of PC was higher than 50°C, the AUC of BSL composed of the corresponding PC was significantly increased compared to that of BSL with Tm under 41°C.
Figure 7 Relationship between the phase transition temperature of PC and the AUC0-t of brucine-loaded stealth liposomes composed of the corresponding PC after intravenous administration at a single dose of 2.5 mg/kg to rats.
Note: n = 6.
Abbreviations: PC, phosphatidylcholine; AUC0-t, area under the curve from time 0 to time (t).

Although in vitro stability of stealth liposomes was intensively studied, the effect of PC composition on in vivo stability should also be investigated and revealed. The results of pharmacokinetics demonstrated that the AUC of BSLs with Tm above 50°C was significantly increased compared to those with Tm below 41°C. Therefore, for the development of BSL for the treatment of tumors, DSPC or HSPC with higher Tm should be taken into consideration. The higher concentration of BSLs in the bloodstream, as demonstrated by plasma AUC measurements, could lead to a higher brucine accumulation in the tumor by enhanced permeation and retention effects after intravenous administration.
It was surprising that MRT values of BSL-HSPC and BSL-DSPC were found to be lower than that of BSL-SPC. But the pharmacokinetic results were in accordance with the drug release profiles in rat plasma. It was generally assumed that the macrophage-resistant property of stealth liposomes is due to suppression in surface opsonization and protein absorption. However, evidence showed that stealth liposomes were also prone to opsonization particularly by the opsonic components of the complement system.Citation19 Although opsonization was significantly restricted in the initial phase after intravenous administration, protein absorption might not be avoided in the later phase. Therefore, the acceleration of clearance from the blood might lead to the reduced MRT values. However, because the liposomes are mainly uptaken by the liver and spleen, not including the brain, the toxicity of BSLs will not be affected.
Acute toxicity test
shows a comparison of the acute toxicity of free brucine and different BSL formulations. The intravenous LD50 of brucine solution was 13.17 mg/kg, while the LD50 values of BSL-SPC, BSL-DPPC, BSL-HSPC, and BSL-DSPC were 37.30, 37.69, 51.18, and 52.86 mg/kg, respectively.
Table 2 Toxic values (Bliss method) of intravenously administrated brucine solution or brucine-loaded stealth liposomes in mice
Severe central nervous system toxicity is the major obstacle to the clinical application of brucine. If it is encapsulated into the stealth liposomes, brucine mainly stays in the blood circulation since stealth liposomes cannot pass the biomembrane directly. Therefore, the distribution of brucine into the brain can be avoided and the acute toxicity of brucine can be reduced subsequently. However, if brucine can be easily released from the liposomes with lower Tm in the bloodstream, it can pass through the blood-brain barrier completely and rapidly. And the toxicity of BSL was increased subsequently.
It was obvious that the toxicity of brucine was significantly reduced after encapsulation into stealth liposomes. In addition, although plasma concentrations of brucine after intravenous administration of BSL-HSPC and BSL-DSPC were much higher than those after intravenous administration of BSL-SPC and BSL-DPPC, the LD50 values were significantly increased. It demonstrated that brucine was mostly encapsulated into stealth liposomes in plasma due to higher stability of HSPC or DSPC stealth liposomes.
Evaluation of membrane permeability by calcein release measurements in vitro
The release of calcein was determined fluorometically by measuring the increase of the fluorescence signal as a result of de-quenching. This has usually been used to characterize the membrane permeability of liposomes. A 90 mM solution of the fluorescent dye calcein was encapsulated into the liposomes. The concentration of the encapsulated dye is above its self-quenching concentration (12 μM).Citation15 Consequently, the fluorescence is only observable upon dilution of the dye due to its release. This enabled monitoring of the release of entrapped material in situ by measuring the increase in the fluorescence signal intensity. The calcein release profiles from stealth liposomes with different PC composition are shown in . At 10 hours, 77.18% ± 3.35% calcein were released from SPC stealth liposomes. For liposomes with higher Tm, only 46.85% ± 0.57%, 11.82% ± 2.53%, and 8.39% ± 2.55% calcein were released from DPPC, HSPC, and DSPC stealth liposomes at 10 hours, respectively. The order of calcein retention in 50% rat plasma was SPC < DPPC << HSPC < DSPC stealth liposomes. The results were in accordance with the results of in vitro brucine release tests.
Figure 8 Calcein release from BSLs with different PC composition in rat plasma: PBS (1:1) at 37°C monitored at different time intervals in the range from 5 to 600 minutes.
Notes: mean ± SD; n = 3.
Abbreviations: BSL, brucine-loaded stealth liposomes; PC, phosphatidylcholine; PBS, phosphate buffered solution; SPC, soy phosphatidylcholine; DPPC, dipalmitoyl phosphatidylcholine; HSPC, hydrogenated soy phosphatidylcholine; DSPC, distearoyl phosphatidylcholine; SD, standard deviation.

Conclusion
In the present paper, the effects of PC (with different Tm) composition on stabilities, pharmacokinetics, and toxicities of BSLs were investigated. It was found that BSLs composed of PC with Tm above 50°C were more stable in vitro and in vivo. But if the Tm of the PC was lower than 41°C, the resulting BSL-SPC and BSL-DPPC were unstable in plasma in vitro and the AUC in vivo was less than one-fifth of that of BSL-HSPC or BSL-DSPC. The toxicity of BSL was in direct relationship with the PC composition. BSL-HSPC and BSL-DSPC with Tm above 50°C possessed lower toxicity compared to BSL-SPC and BSL-DPPC. In summary, our study indicated that DSPC or HSPC with Tm above 50°C should be used to prepare the stealth liposomal formulation for the targeted delivery of brucine because of high stability.
Acknowledgements
This work was financially supported by the National Nature Science Foundation of China (No 30701111, 81001644) and Guangdong Nature Science Foundation (10451040701004643).
Disclosure
The authors report no conflicts of interest. The authors alone are responsible for the content and writing of this paper.
References
- PhilippeGAngenotLTitsMFrédérichMAbout the toxicity of some Strychnos species and their alkaloidsToxicon200444440541615302523
- DengXKYinWLiWDThe anti-tumor effects of alkaloids from the seeds of Strychnos nux-vomica on HepG2 cells and its possible mechanismJ Ethnopharmacol2006106217918616442763
- YinWDengXKYinFZZhangXCCaiBCThe cytotoxicity induced by brucine from the seed of Strychnos nux-vomica proceeds via apoptosis and is mediated by cyclooxygenase 2 and caspase 3 in SMMC 7721 cellsFood Chem Toxicol20074591700170817449162
- RaoPSRamanadhamMPrasadMNAnti-proliferative and cytotoxic effects of Strychnos nux-vomica root extract on human multiple myeloma cell line – RPMI 8226Food Chem Toxicol200947228328819027818
- AgrawalSSSaraswatiSMathurRPandeyMCytotoxic and antitumor effects of brucine on Ehrlich ascites tumor and human cell lineLife Sci2011895–614715821684292
- MaloneMHSt John-AllanKMBejarEBrucine lethality in miceJ Ethnopharmacol19923532952971347799
- ZhangLHanLSunXGaoDQinJWangJThe use of PEGylated liposomes to prolong the circulation lifetime of salvianolic acid BFitoterapia2252012 [Epub ahead of print.]
- SeniorJGregoriadisGIs half-life of circulating liposomes determined by changes in their permeability?FEBS Lett198214511091146897042
- YangXZhaoXPhelpsMAA novel liposomal formulation of flavopiridolInt J Pharm20093651–217017418778761
- PaolinoDCoscoDRacanicchiLGemcitabine-loaded PEGylated unilamellar liposomes vs GEMZAR: biodistribution, pharmacokinetic features and in vivo antitumor activityJ Control Release2010144214415020184929
- ZamboniWCStrychorSJosephEPlasma, tumor, and tissue distribution of STEALTH liposomal CKD-602 (S-CKD602) and nonliposomal CKD-602 in mice bearing A375 human melanoma xenograftsClin Cancer Res200713237217722318056203
- ChenJLinAChenZAmmonium sulfate gradient loading of brucine into liposomes: effect of phospholipid composition on entrapment efficiency and physicochemical properties in vitroDrug Dev Ind Pharm201036324525319678739
- ChenJXiaoHLHuRRPharmacokinetics of brucine after intravenous and oral administration to ratsFitoterapia20118281302130821958965
- RosielloAPEssignmannJMWoganGNRapid and accurate determination of the median lethal dose (LD50) and its error with a small computerJ Toxicol Environ Health19773797809599579
- DjanashviliKten HagenTLBlangéRSchipperDPetersJAKoningGADevelopment of a liposomal delivery system for temperature-triggered release of a tumor targeting agent, Ln(III)-DOTA-phenylboronateBioorg Med Chem20111931123113020624680
- ChouTHChenSCChuIMEffect of composition on the stability of liposomal irinotecan prepared by a pH gradient methodJ Biosci Bioeng200395440540816233428
- QinXQYuanYLiuCSPreparation of liposomal brucine and its pharmaceutical/pharmacodynamic characterizationActa Pharmacol Sin200728111851185817959038
- MercadalMDomingoJCBermudezMMoraMDe MadariagaMAN-palmitoylphosphatidylethanolamine stabilizes liposomes in the presence of human serum: effect of lipidic composition and system characterizationBiochim Biophys Acta1995123522812887756336
- MoghimiSMSzebeniJStealth liposomes and long circulating nanoparticles: critical issues in pharmacokinetics, opsonization and protein-binding propertiesProg Lipid Res200342646347814559067