Abstract
A significant need exists for orthopedic implants that can intrinsically resist bacterial colonization. In this study, three biomaterials that are used in spinal implants – titanium (Ti), polyether-ether-ketone (PEEK), and silicon nitride (Si3N4) – were tested to understand their respective susceptibility to bacterial infection with Staphylococcus epidermidis, Staphlococcus aureus, Pseudomonas aeruginosa, Escherichia coli and Enterococcus. Specifically, the surface chemistry, wettability, and nanostructured topography of respective biomaterials, and the effects on bacterial biofilm formation, colonization, and growth were investigated. Ti and PEEK were received with as-machined surfaces; both materials are hydrophobic, with net negative surface charges. Two surface finishes of Si3N4 were examined: as-fired and polished. In contrast to Ti and PEEK, the surface of Si3N4 is hydrophilic, with a net positive charge. A decreased biofilm formation was found, as well as fewer live bacteria on both the as-fired and polished Si3N4. These differences may reflect differential surface chemistry and surface nanostructure properties between the biomaterials tested. Because protein adsorption on material surfaces affects bacterial adhesion, the adsorption of fibronectin, vitronectin, and laminin on Ti, PEEK, and Si3N4 were also examined. Significantly greater amounts of these proteins adhered to Si3N4 than to Ti or PEEK. The findings of this study suggest that surface properties of biomaterials lead to differential adsorption of physiologic proteins, and that this phenomenon could explain the observed in-vitro differences in bacterial affinity for the respective biomaterials. Intrinsic biomaterial properties as they relate to resistance to bacterial colonization may reflect a novel strategy toward designing future orthopedic implants.
Introduction
In the year 2008, an estimated 413,171 spinal fusion operations, 436,736 primary total hip arthroplasty (THA), and 680,839 primary total knee arthroplasty (TKA) procedures were performed in the United States alone,Citation1 leading to an approximately US$13 billion market for orthopedic implants.Citation2 Future demand for such implants is expected to rise. When otherwise well functioning orthopedic implants become colonized with bacteria, significant patient morbidity can follow. The reason is that bacteria adhere to implant surfaces and are resistant to host immune mechanisms and systemic antibiotics. Treatment of such implant-related infections requires extensive repeat surgery, implant extrication, extended duration of systemic antibiotic therapy, bone loss, and substantial cost, suffering, and disability.
Implant-related infections occur in spine surgery between 2.6% and 3.8% of the time.Citation3 In hip replacement surgery, deep infection occurs at 1.63% at 2-years post-operatively,Citation4 and the figures for knee replacement are about 1.55%.Citation5 These numbers underestimate the true incidence of infection because of difficulties related to clinical diagnoses, and a paucity of credible data from epidemiological surveys. Mortality rates from complications related to deep prosthetic infections in THA and TKA are reportedly between 2.7% and 18%,Citation6 and infection is the most common reason for repeat surgery after otherwise successful prosthetic joint replacement.Citation7 The incidence of infection in THA and TKA may be increasing, probably due to improved detection and also because of evolving antibiotic-resistant bacteria strains. Treatment costs related to infected orthopedic implants can range from 1.52 to 1.76 times the cost of the original surgery.Citation8 While orthopedic implants are deemed expensive, their costs are easily overwhelmed by the overall treatment cost of implant-related infections.Citation9 Therefore, there is considerable interest in reducing the risk of infections related to orthopedic implants.
In addition to total joint replacement and spinal devices, orthopedic implants are also manufactured as screws, plates, and percutaneously implanted pins to treat fractures; bacterial contamination of such can lead to poor bone healing.Citation10 Unless acute implant infections can be overcome expeditiously, adjacent tissues become colonized with bacteria,Citation11–Citation13 and the risk of chronic bone infection (osteomyelitis) increases.Citation13,Citation14 Osteomyelitis can complicate fracture treatment with metal external fixator devices up to 4% of the time.Citation11–Citation13 Ultimately, orthopedic infections lead to implant loosening, nonhealing of fractures, and device failures.Citation12
Bacteria present in the bloodstream and body tissues can usually be cleared by host immune mechanisms and antibiotic treatment.Citation15 However, once bacteria colonize implant surfaces by expressing a biofilm layer, they become relatively impervious to such nonsurgical measures. Biofilm production is accompanied by changes in gene expression and growth rates such that host immune mechanisms are rendered ineffective, leading to a chronically infected environment around the implant.Citation15–Citation18 A related clinical concern pertains to the development of antibiotic-resistant strains of bacteria, including S. aureus and S. epidermidis, which have been well documented in the clinical orthopedic setting.Citation19,Citation20 Any material science-derived strategy that discourages bacterial colonization of implant surfaces will therefore be of value in reducing the need for systemic antibiotic therapy and surgical removal of infected implants.Citation20
Changing implant surface texture from a micron-sized to a nanometer-sized topography affects osteoblast adhesionCitation21–Citation26 and subsequent cellular function, while decreasing competitive fibroblast activity.Citation27,Citation28 Nanoroughened titanium (Ti) made by electron-beam evaporationCitation29 and nanotubular and nanotextured Ti created by anodization can enhance osteoblast adhesion and function (such as alkaline phosphatase synthesis, calcium deposition, and collagen secretion) when compared with micron nanosmooth control surfaces.Citation30 Increased protein adsorption on nanotextured Ti surfaces is correlated with improved osteoblast function.Citation31–Citation33 The authors have previously shown that by selectively engineering the surface topography of a biomaterial, bacteria adhesion can be decreased.Citation34,Citation35
Because silicon nitride (Si3N4) is used in spinal reconstructive surgery today, the adhesion of multiple bacteria species onto polished and nanostructured versions of this biomaterial was examined, using two other orthopedic biomaterials (Ti and poly-ether-ether-ketone [PEEK]) as controls. The null hypothesis was that bacterial adhesion and surface protein adsorption (fibronectin, vitronectin, and laminin) would not differ between these materials. Gram-positive S. epidermidis and S. aureus, and Gram-negative P. aeruginosa, E. coli, and Enterococcus were tested because these strains are commonly implicated in orthopedic implant infections.Citation19
Materials and methods
Biomaterials
Si3N4 was supplied by Amedica Corporation (Salt Lake City, UT) in two surface morphologies; with an “as-fired” surface that has nanostructured features, and a smooth polished surface, respectively. Biomedical grade 4 titanium (Fisher Scientific, Continental Steel and Tube Co, Fort Lauderdale, FL) and PEEK Optima® (Invibio, Lancashire, United Kingdom) were obtained with typical machined surfaces. The surfaces of these materials were characterized for morphology and roughness using scanning electron microscopy (SEM) (using an LEO 1530 VP FE-4800 field-emission scanning electron microscope [Zeiss, Peabody, MA]). Specimens of 1 cm × 1 cm dimensions first underwent sessile water-drop tests to assess material wetting characteristics using a KRÜSS easy drop contact angle instrument connected to the drop shape analysis program (version 1.8) (KRÜSS GmbH, Hamburg, Germany) in accordance with an ASTM standard (D7334-08).Citation36 Prior to bacterial exposure, all samples underwent sterilization with ultra violet light exposure for 24 hours on all sides, and roughness characterization with standard SEM.
Bacteria studies
Bacteria were inoculated (105) onto the material surfaces for 4, 24, 48, and 72 hours. S. epidermidis, P. aeruginosa, S. aureus, E. coli, and Enterococcus were obtained from American Type Culture Collection (Manassas, VA) (strains 35984, 25668, 25923, 26, and 6569, respectively). The dry pellet was rehydrated in 6 mL of Luria broth (LB) consisting of 10 g tryptone, 5 g yeast extract, and 5 g NaCl per liter double-distilled water with the pH adjusted to 7.4 (Sigma-Aldrich, St Louis, MO). Fetal bovine serum (FBS) (HyClone; Thermo Scientific, Waltham, MA) at 10% concentration was added to the LB, and the bacteria solution was agitated under standard cell conditions (5% CO2/95% humidified air at 37°C) for 24 hours until the stationary phase was reached. The second passage of bacteria was diluted at a ratio of 1:200 into fresh LB supplemented with 10% FBS and incubated until it reached the stationary phase. The second passage was then frozen in one part LB and 10% FBS and one part glycerol (Sigma-Aldrich) and stored at −18°C. All experiments were conducted from this frozen stock. One day before bacterial seeding for experiments, a sterile 10 μL loop was used to withdraw bacteria from the frozen stock and to inoculate a centrifuge tube with 3 mL of fresh LB supplemented with 10% FBS.
Bacterial function was determined by crystal violet staining and a live/dead assay (Molecular Probes®, Life Technologies, Carlsbad, CA) using previously described methods.Citation35 For the live/dead assay, at the end of the prescribed time period, substrates were rinsed twice with Tris-buffered saline (TBS) comprised of 42 mM Tris-HCl, 8 mM Tris base, and 0.15 M NaCl (Sigma-Aldrich) and then incubated for 15 minutes with the BacLight™ Live/Dead solution (Life Technologies) dissolved in TBS at the concentration recommended by the manufacturer. Substrates were then rinsed twice with TBS and placed into a 50% glycerol solution in TBS prior to imaging. Bacteria were visualized and counted in situ using a Leica DM5500 B fluorescence microscope with image analysis software captured using a Retiga™ 4000R camera (QImaging, Surrey, BC, Canada).
Protein adsorption
Standard fibronectin, vitronectin, and laminin enzyme-linked immunosorbent assays (ELISA) were performed after soaking material samples in bacterial media (LB supplemented with 10% FBS) used above for 20 minutes, 1 hour, and 4 hours, as previously described.Citation35 Substrates were placed in a standard 24-well culture plate and immersed in 1 mL of LB supplemented with and without 10% FBS for 20 minutes, 1 hour, and 4 hours at 37°C in 5% CO2/95% humidified air. After rinsing in phosphate-buffered saline (PBS), areas that did not absorb proteins were blocked and incubated for 1 hour in bovine serum albumin, BSA (2 wt% in PBS, Sigma-Aldrich). Substrates were again rinsed twice with PBS before either fibronectin, vitronectin, or laminin were directly linked respectively with primary rabbit antibovine fibronectin, anti-vitronectin, or anti-laminin (EMD Millipore, Billerica, MA) at a concentration of 6 μg/mL in 1% BSA for 1 hour at 37°C in 5% CO2/95% humidified air. After rinsing three times with 0.05% Tween 20® (AkzoNobel, Amsterdam, The Netherlands) for 5 minutes with each rinse, the samples were further incubated for 1 hour with a secondary goat anti-rabbit conjugated with horseradish peroxidase (HRP) (Bio-Rad Laboratories, Hercules, CA) at a concentration of 10 μg/mL in 1% BSA. Following triple rinsing with 0.05% Tween 20 for 5 minutes per rinse, surface-adsorbed protein was measured with an ABTS substrate kit (Vector Laboratories, Burlingame, CA) that reacted only with the HRP. Light adsorbance was measured at 405 nm on a spectrophotometer and analyzed with computer software. The average adsorbance was subtracted by the average adsorbance obtained from the negative controls soaked in LB with no FBS. ELISA was performed in duplicate and repeated three times per substrate.
Statistical analysis
Each experiment was done in triplicate with new bacteria, media, and samples. Time series data for biofilm formation, bacteria colonization, and growth were curve-fit to either linear or exponential functions. Hypothesis testing was completed using regression analysis and confidence intervals in accordance with techniques previously described.Citation37 Paired comparison t-tests were used to assess significance for the protein adsorption studies; a P-value of <0.05 was deemed statistically significant.
Results
Comparison of materials
Relevant properties of the three biomaterials tested are compared in . Si3N4 has a protective surface layer that is composed of charged SiNH3+, SiOH2+, SiO−, and neutral SiNH2 and SiOH groups.Citation38 The presence of the amine groups leads to a high isoelectric point (between 8 and 9) and an overall net positive surface charge.Citation38,Citation39 Ti has a native oxide layer (TiO2) which has an isoelectric point of about 4.5 and therefore a negative surface charge at pH 7.Citation40 PEEK surfaces have polymeric chains ending in –OH groups, that yield an isoelectric point of about 4.5 and a negative surface charge.Citation41 Si3N4 has the lowest wetting angle and greater hydrophilicity when compared with PEEK or Ti ().
Table 1 Comparative properties of medical grades of Si3N4, ASTMCitation35 grade 4 Ti, and PEEK
SEM images of biomaterial surfaces are illustrated in and . Of the materials tested, PEEK and Ti had machined surfaces, while Si3N4 was in as-fired and polished formulations. SEM images of PEEK and Ti show a micron-rough surface typical of machined materials. The as-fired Si3N4 had nanostructured surface features with a larger total surface area, as shown in the high magnification images (). The as-fired Si3N4 surface morphology reflects the natural condition of this material subsequent to densification by sintering and hot-isostatic pressing.Citation42 The surface is composed of randomly oriented acicular protruding grains typically less than 1 μm in cross-section, yielding a unique nanotexture (). Note that individual hexagonal grains of Si3N4 have definitive linear facets (in cross-section) and sharp corners at the termination of the acicular grains, which are typically less than 100 nm. These unique features may play a role in interaction with bacteria, resulting in their lysis. This is contrasted to the highly polished Si3N4 surface of in which the acicular asperities were removed.
Figure 1 Scanning electron microscopy surface microstructures of PEEK Optima®, Ti, and Si3N4: (A1) PEEK 1000×; (A2) PEEK 5000×; (B1) Ti 1000×; (B2) Ti 5000×; (C1) Si3N4 1000×; (C2) Si3N4 5000×.
Abbreviation: PEEK, poly-ether-ether-ketone.
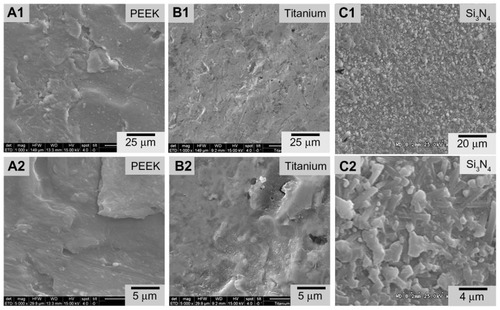
Figure 2 Scanning electron microscopy surface microstructure of polished and as-fired Si3N4: (A) polished surface 20,000×; (B) as-fired surface 20,000×.
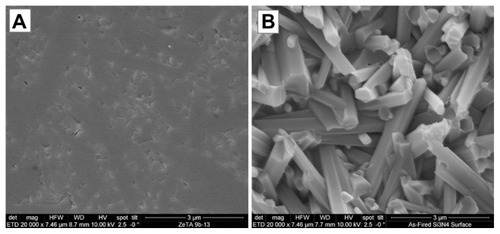
Surface roughness measurements derived from atomic force microscopy (AFM) measurements are summarized in . Results were 25 nm, 10 nm, 3 nm, and 1 nm for the asfired Si3N4, polished Si3N4, Ti, and PEEK, respectively. These AFM surface roughness values differ from those that could be obtained using contact profilometry because the areal sampling size of AFM is small compared with contact profilometry. It is obvious from a comparison of the features in that the micron scale roughness of Si3N4 is similar to that of Ti and PEEK, although there are notable topographical differences. However, the AFM measurements were made over fractions of nanometers. In effect, AFM is measuring the nanoroughness of the materials inbetween micron-sized surface features. These results show that as-fired Si3N4, polished Si3N4, Ti, and PEEK have markedly different surface chemistries and topographies that could affect bacterial and protein adhesion.
Bacteria studies
shows biofilm formation for each bacterial species, and shows corresponding live bacteria counts at each time interval. Except for Enterococcus, trends in biofilm formation were similar for all bacteria. Exponential growth of biofilm was noted on PEEK when exposed to S. epidermidis, S. aureus, P. aeruginosa, and E. coli, whereas linear growth was observed for Si3N4 and Ti. Independent of time points, the lowest biofilm formation occurred on the as-fired Si3N4, followed by polished Si3N4. These differences were statistically significant (P < 0.05) for bacterial exposure times > 24 hours. Also, with the exception of Enterococcus, biofilm formation was significantly lower (P < 0.05) on Ti compared with PEEK, for time periods > 48 hours. PEEK demonstrated the highest biofilm affinity, with values that were 5–16 times greater than those for as-fired Si3N4, 1.5–10 times more than for polished Si3N4, and between 1 and 6.7 times higher than for Ti.
Figure 3 Biofilm formation: (A) Staphylococcus epidermidis, (B) S. aureus, (C) Pseudomonas aeruginosa, (D) Escherichia coli, (E) Enterococcus.
Note: Dashed lines represent confidence intervals at P < 0.05.
Abbreviations: SN, Si3N4; PEEK, poly-ether-ether-ketone.
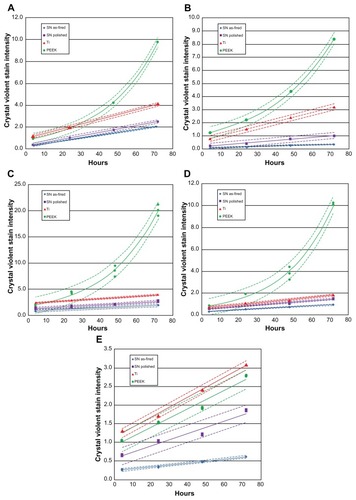
Figure 4 Count of live bacteria: (A) Staphylococcus epidermidis, (B) S. aureus, (C) Pseudomonas aeruginosa, (D) Escherichia coli, (E) Enterococcus.
Note: Dashed lines represent confidence intervals at P < 0.05.
Abbreviations: SN, Si3N4; PEEK, poly-ether-ether-ketone.
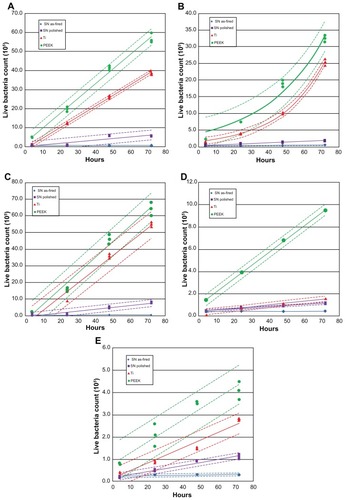
Live bacteria counts were the highest for PEEK at all time periods, followed by Ti, polished Si3N4, and then asfired Si3N4 (). These differences were statistically significant (P < 0.05) for all bacterial species tested, and at all time periods > 48 hours. Live bacteria manifested on PEEK were between 8 and 30 times the bacteria found on as-fired Si3N4. These results were particularly remarkable for P. aeruginosa, which is a virulent and difficult microbe to eliminate from polymeric implants.Citation43
To summarize, less bacterial activity was manifested on Si3N4 than on either Ti or PEEK, probably because of the surface chemistry and nanostructure differences between these materials. Substantially lower loads of S. epidermidis, S. aureus, P. aeruginosa, E. coli, and Enterococcus bacteria were measured on Si3N4 surfaces than Ti or PEEK, for up to 72 hours of incubation.
Protein adsorption studies
shows the relative absorbance of fibronectin, vitronectin, and laminin at 20-, 60-, and 240-minute intervals. Fibronectin and vitronectin adsorption was significantly greater on Si3N4 (whether polished or as-fired) when compared with PEEK or Ti at all time intervals (P < 0.01). As-fired Si3N4 showed a higher affinity for these two proteins than polished Si3N4. Laminin adsorption did not differ significantly between the biomaterials at the 20-minute time interval. At 60 minutes, both Si3N4 surfaces showed significant increased laminin adsorption (P < 0.01) when compared with Ti or PEEK. At 240 minutes, as-fired Si3N4 was the only surface that showed statistically more laminin adsorption (P < 0.01) than all other materials.
Figure 5 Protein adsorption: (A) 20 minutes, (B) 60 minutes, (C) 240 minutes.
Notes: *P < 0.01 compared with all others; **P < 0.05 compared with PEEK.
Abbreviations: Arb, arbitrary; SN, Si3N4; PEEK, poly-ether-ether-ketone.
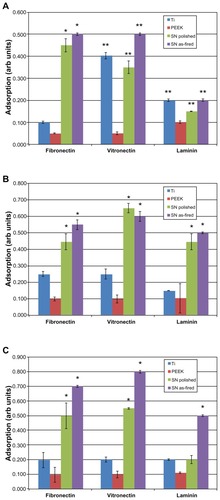
To summarize, Ti and PEEK proved inferior in terms of protein adsorption when compared with as-fired and polished Si3N4 surfaces. As-fired Si3N4 provided the highest protein adsorption platform among the materials tested. Surface protein adsorption is relevant because previous studies have correlated increased vitronectin and fibronectin adsorption to decreased bacterial activity.Citation35
Discussion
Bacterial infection of orthopedic implants is a complex, multifactorial process that is influenced by bacterial properties, the presence of serum proteins, fluid flow around the implant, implant surface chemistry and morphology, host immune variables, and probably other variables.Citation44 Infection can arise from inadvertent contamination of an implant, contagion from the surgical staff, bacteria arising from the patient’s skin or mucus membranes, unrecognized infection elsewhere in the body, ineffectively applied surgical disinfectants, and sepsis acquired from others.Citation45 Most of these risk factors can be mitigated by appropriate nosocomial hygiene practices, and through the use of perioperative antibiotic prophylaxis.Citation3,Citation6,Citation9,Citation46,Citation47 However, no strategy has proven effective in completely eliminating the risk of infections related to implant surgery. Strategies to discourage bacterial adhesion to implants have included antibacterial surface coatings and treatments, and the development and use of nanostructured surfaces, or a combination of these methods.
Surface coatings and treatments
Surface coatings or surface treatments on implants can resist pathogen adhesion or release chemicals that invade bacterial biofilm. Silver ions have been investigated as a surface antiseptic agent, although the exact mechanism for silver ion toxicity on bacteria is unclear.Citation48 Silver can be incorporated into polymeric or inorganic coatings,Citation48,Citation49 and while its anti-infective properties have been known for generations, it continues to drive patent innovation.Citation50,Citation51 Other coatings have included pre-loaded formulations of antibiotics like vancomycin or gentamicin, or antiseptic agents like chlorohexidine and chloroxylenol.Citation52 Antibacterial polymeric functionalized coatings which incorporate hyaluronic acid and chitosan have been investigated thoroughly.Citation52–Citation57 Xerogel polymers that have been modified to release nitric oxide are effective in inhibiting the adhesion of S. aureus, S. epidermidis, and E. coli.Citation58 A limitation of all such coatings is the transient duration of effectiveness, ie, once the coating dissipates, so does any antibacterial effect.
Surface modifications of biomaterials can provide a long-term shield against bacterial infection of implants. Yoshinari et al modified the surface of Ti and studied bacterial adhesion of P. gingivalis and Aggregatibacter actinomycetemcomitans using ion implantation of Ca+, N+, and F+, ion-beam deposition of Ag, Sn, Zn, and Pt, ion plating of TiN and Al2O3, and anodic oxidation formation of TiO2.Citation59 Control materials included polished, sand-blasted, and striated Ti. They found a general trend toward higher bacterial adhesion on blasted and Ca+ implanted Ti. A follow-up study by these authors also showed that F+ implanted surfaces resisted initial bacterial adhesion in the absence of fluorine leaching from the surface; the antibacterial effect probably related to metal-fluorine complexes at the surface.Citation60 Katsikogianni et al found otherwise: S. epidermidis activity on polymers with and without fluorine showed that those containing fluorine increased bacteria attachment.Citation61 Raulio et al also investigated fluorine, and found that by coating stainless steel with fluoropolymers, it was possible to reduce biofilm formation of several bacteria strains, including S. epidermidis.Citation62
Li et al performed a comparative study using glass and metal-oxides applied as thin films.Citation63 These included three uncoated glass surfaces and combinations of Co-Fe-Cr, Ti-Fe-O, SnO2, SnO2-F, SnO2-Sb, Al2O3, and Fe2O3 thin films applied to glass substrates. After measuring material hydrophilicity, zeta potential, and surface energy, the authors tested them against eight strains of bacteria, and found that hydrophobicity and total surface energy (rather than material chemistry) led to increased bacterial adhesion. Hydrophilic surfaces had the fewest number of adherent bacteria, and increasing the concentration of surface ions encouraged binding for both Gram-positive (Bacillus subtilis) and Gram-negative (two P. aeruginosa strains, three E. coli strains, and two Burkholderia cepacia strains) bacteria.
Conversion of Ti surfaces to TiO2 via anodic oxidation, electrophoretic deposition, chemical vapor deposition, ion implantation, and plasma spraying has been examined as an anti-infective surface modification strategy, especially when coupled with photocatalytic activation of TiO2.Citation64 Orthopedic implants made of porous Ti are commonly coated with hydroxyapatite to promote osteointegration, but this strategy may lead to increased harboring of S. aureus bacteria, more severe infection, and less osteointegration when compared with uncoated Ti.Citation65,Citation66
The results of this present study show that Si3N4 has intrinsic bacteriostatic properties, whether in an as-fired or smooth-surface morphology. This study did not elucidate the precise mechanism of Si3N4 anti-infectivity, but results suggest that material hydrophilicity and surface chemistry may contribute to less biofilm formation () and lower bacteria counts () on Si3N4 when compared with Ti or PEEK. These differences were observed for all bacteria, regardless of Gram-positive or Gram-negative strains. Hydrophilic Si3N4 surfaces were probably less conducive to bacterial adhesion, when compared with hydrophobic surfaces where water displacement is not required for microbial adherence.Citation44 Consistent with this observation, the inert and highly hydrophobic characteristics of PEEK promoted bacterial adhesion and inhibited protein adsorption when compared with Si3N4. Other authors have supported these observations as they relate to PEEK and Ti.Citation67,Citation68
The anti-infectivity of Si3N4 may also relate to surface chemistry, and the authors’ observations are consistent with previous data with polymeric coatings containing chitosan, a material similar to Si3N4 in terms of a net positive charge and the presence of amine groups at the surface.Citation52–Citation56 The interaction of these groups with negatively charged bacteria reportedly leads to membrane disruption and lysis in chitosan-containing polymers.Citation56 However, further research is required to confirm whether or not this is a dominant operative mechanism for Si3N4.
Nanostructured surfaces
Nanotechnology is of interest in enhancing the surface characteristics and improving the performance of orthopedic implants. Increasing micron-level surface roughness of biomedical implants correlates with increased bacterial adhesionCitation44 and enhanced osteointegration.Citation69 The competition between bacteria, bone cells, and serum proteins has been characterized as a “race to the surface.”Citation10 However, surface roughness is only one of several variables that influence bacterial adhesion and protein adsorption on an implant. For instance, without specifically distinguishing changes in surface roughness, surface chemistry, or crystallinity, Truong et al showed that nanoscale roughening of ultrafine grained Ti led to greater attachment of S. aureus and P. aeruginosa, when compared with smooth surfaces.Citation70 Conversely, Singh et al found increased bacterial adhesion and biofilm formation on Ti surfaces with Ra (roughness average) values < 20 nm, and increased protein adsorption at Ra values between 16 and 32 nm; further increases in surface roughness were associated with reduced pathogen activity. Citation71 In contrast, Hilbert et al could not correlate bacterial adhesion, colonization, and growth with changes in surface finish ranging from 0.1 to 0.90 μm,Citation72 and Flint et al found that bacterial adhesion could not be related to surface roughness at all.Citation73 Thus, surface roughness alone may not fully explain the differences in bacterial adhesion and protein adsorption.
Anselme et al reviewed the factors that affect the interaction of cells and bacteria with nanostructured surfaces. Citation74 In addition to surface roughness, detailed surface topography, including the size, shape, orientation, distance, and organization of surface nanofeatures were identified as important variables. Whitehead et al further supported this view when they found higher bacterial counts on substrates that contained 2 μm diameter pits as opposed to those in the range of 0.5 μm.Citation75 Xu et al compared bacterial adhesion between two polyurethane surfaces of the same chemistry; one was smooth and the other contained oriented protrusions or pillars (400 nm diameter × 650 nm height).Citation76 They found biofilm formation significantly less for both S. epidermidis and S. aureus when using the highly textured polyurethane. Dalby et al examined the response of fibroblasts to nanocolumn structures (100 nm diameter × 160–170 nm height) in polymethylmethacrylate (PMMA) in comparison with smooth substrates and demonstrated decreased cell adhesion using the materials with nanocolumns.Citation77 Bagno et al showed that the number of protruding peaks (ie, peak density) was positively correlated with osteoblast adhesion.Citation78 Similar topographical effects on bacterial adhesion and protein adsorption were observed by these authors for Ti, when conventional (smooth) surfaces were compared to nanoroughened, nanotextured, and nanotubular variations. The authors also reported decreased in-vitro activity of S. aureus, S. epidermidis, and P. aeruginosa on nanorough surfaces prepared by electron-beam evaporation, but an opposite result for nanotubular and nanorough surfaces prepared via anodization.Citation35 However, all three of the nanoprepared Ti surfaces demonstrated increased adsorption of fibronectin in comparison with conventional Ti. A subsequent study showed reduced adhesion of macrophages on anodized Ti with nanotextured and nanotubular structures.Citation79
Engineered surfaces with topographical features in the nanometer range may affect cell behavior while reducing bacterial adhesion, but these factors alone do not sufficiently predict cellular response. Other variables related to surface energy, surface charge, and chemistry can also inhibit bacterial colonization while enhancing protein adsorption and conformation, leading to improved osteoblast adhesion and tissue growth.Citation31–Citation33 Biomaterial surfaces with nanorough features are associated with greater vitronectin and fibronectin adsorption that, in turn, are related to decreased bacterial function on the surface.Citation31–Citation33,Citation35,Citation80
The acicular grain structure on the as-fired surface of Si3N4 is composed of randomly oriented columnar grains that are typically 200–700 nm in diameter, and that can protrude above the surface up to about 3 μm. This as-fired surface is not only significantly rougher than Ti, PEEK, or polished Si3N4 () but its nanotopography is fundamentally different, as suggested by AFM surface measurements. Even though the PEEK and titanium samples were as-machined, they had significantly smoother surfaces at the nanolevel than either as-fired or polished Si3N4. Consequently, bacterial and protein adherence to the biomaterials differed in the present experiments. While this study did not elucidate the precise mechanisms, it is probable that the observed behavior of as-fired Si3N4 is related to nanocolumns, protrusions, and peaks described in previous studies for polyurethane, PMMA, and Ti surfaces.Citation76–Citation78 In summary, the present authors believe it is the totality of silicon nitride’s nano-topography – including its protruding acicular grains with their definitive hexagonal features and sharp grain ends – combined with its surface chemistry (ie, positive surface charge and the presence of amine groups) which provides Si3N4 with its unique antibacterial and protein adsorption characteristics.
Conclusion
This study examined the behavior of Ti, PEEK, and Si3N4 when respective materials were exposed to five different bacterial species for up to 72 hours. Polished and as-fired surface formulations of Si3N4 were tested. Decreased biofilm formation and bacterial colonization were demonstrated on both as-fired and polished Si3N4 in comparison with Ti or PEEK, under in-vitro incubation for time periods of up to 72 hours. Si3N4 resisted bacterial proliferation under these conditions, despite the absence of antibiotic pharmaceutical agents. Differential protein adsorption on Ti, PEEK, and Si3N4 surfaces was also demonstrated, such that fibronectin, vitronectin, and laminin adsorbed preferentially onto Si3N4 when compared with Ti or PEEK, for time periods of up to 4 hours. Previous studies have shown that such differences in protein affinity for biomaterial surfaces may explain, at least in part, differences in biomaterial susceptibility to bacterial infection. The unique surface chemistry and nanostructured features of Si3N4 may contribute to the favorable antibacterial properties of this bioceramic material, and support further investigation into the use of Si3N4 as a material platform for orthopedic implants.
Acknowledgments
The authors thank Khalid A Sethi, MD, FACS, and Christine Ann Snyder, PA, of the Southern New York NeuroSurgical Group, P.C., for their assistance in performing the studies, and David Bohrer for efforts in initiating this work. The authors also express their appreciation to Bryan McEntire, Chief Technology Officer, Alan Lakshminarayanan PhD, Senior Director of Research and Development, Ryan Bock PhD, Research Scientist, all of Amedica Corporation (Salt Lake City, UT); and Steven C Friedman, Senior Editor, Department of Orthopedic Surgery, University of Missouri, for their kind assistance with the manuscript.
Disclosure
B Sonny Bal is advisory surgeon to Amedica, developer of synthetic silicon nitride for orthopedic applications, and serves on the Board of Directors of Amedica, Salt Lake City, UT. Co-authors Deborah Gorth, Sabrina Puckett, Batur Ercan, Thomas J Webster, and Mohamed N Rahaman have no disclosures for this article.
References
- RajaeeSSBaeHWKanimLEDelamarterRBSpinal fusion in the United States: analysis of trends from 1998 to 2008Spine2012371677621311399
- MendenhallSHip and knee implant reviewOrthopedic Network News2011223466469
- CollinsIWilson-MacDonaldJChamiGThe diagnosis and management of infection following instrumented spinal fusionEur Spine J200817344545018075763
- OngKLKurtzSMLauEProsthetic joint infection risk after total hip arthroplasty in the Medicare populationJ Arthroplasty200924Suppl 610510919493644
- KurtzSMOngKLProsthetic joint infection risk after TKA in the Medicare populationClin Orthop Relat Res2010468525619669386
- MatarWYJafariSMRestrepoCPreventing infection in total joint arthroplastyJ Bone Joint Surg Am201092Suppl364621123590
- GardnerJGioeTJTatmanPCan this prosthesis be saved? Implant salvage attempts in infected primary TKAClin Orthop Relat Res2011469497097620544317
- KurtzSMLauESchmierJInfection burden for hip and knee arthroplasty in the United StatesJ Arthroplasty200823798499118534466
- DarouicheROTreatment of infections associated with surgical implantsN Engl J Med2004350141422142915070792
- GristinaAGBiomaterial-centered infection: microbial adhesion versus tissue integrationScience19872374822158815953629258
- MoroniAVanniniFMoscaMGianniniSState of the art review: techniques to avoid pin loosening and infection in external fixationJ Orthop Trauma200216318919511880783
- MahanJSeligsonDHenrySHynesPDobbinsJFactors in pin tract infectionsOrthopedics19911433053082020629
- ZlowodzkiMPrakashJSAggarwalNKExternal fixation of complex femoral shaft fracturesInt Orthop200731340941316909254
- GreenSRipleyMChronic osteomyelitis in pin tracksJ Bone Joint Surg Am1984667109210986384221
- CostertonJWStewartPSGreenbergEPBacterial biofilms: a common cause of persistent infectionsScience199928454181318132210334980
- RimondiniLFiniMGiardinoRThe microbial infection of biomaterials: a challenge for clinicians and researchers. A short reviewJ Appl Biomater Biomech20053111020799234
- FuxCCostertonJStewartPStoodleyPSurvival strategies of infectious biofilmsTrends Microbiol2005131344015639630
- DonlanRMBiofilms and device-associated infectionsEmerg Infect Dis20017227728111294723
- BaqueroFGram-positive resistance: challenge for the development of new antibioticsJ Antimicrob Chemother199739Suppl A169511055
- CampocciaDMontanaroLArciolaCRThe significance of infection related to orthopedic devices and issues of antibiotic resistanceBiomaterials200627112331233916364434
- WebsterTJEjioforJUIncreased osteoblast adhesion on nanophase metals: Ti, Ti6Al4V, and CoCrMoBiomaterials200425194731473915120519
- WebsterTJSiegelRWBiziosROsteoblast adhesion on nanophase ceramicsBiomaterials199920131221122710395391
- WebsterTJErgunCDoremusRHSiegelRWBiziosREnhanced functions of osteoblasts on nanophase ceramicsBiomaterials200021171803181010905463
- WebsterTJErgunCDoremusRHSiegelRWBiziosREnhanced osteoclast-like cell functions on nanophase ceramicsBiomaterials200122111327133311336305
- EjioforJWebsterTBone cell adhesion on titanium implants with nanoscale surface featuresInt J Powder Metallurgy20044024353
- WardBCWebsterTJIncreased functions of osteoblasts on nanophase metalsMater Sci Eng C Mater Biol Appl2007273575578
- MillerDCVanceRJThapaAWebsterTJHaberstrohKMComparison of fibroblast and vascular cell adhesion to nano-structured poly(lactic-co-glycolic acid) filmsAppl Bionics Biomech20052117
- CohenALiu-SynderPStoreyDWebsterTJDecreased fibroblast and increased osteoblast functions on ionic plasma deposited nanostructured Ti coatingsNanoscale Res Lett200728385390
- PuckettSParetaRWebsterTJNano rough micron patterned titanium for directing osteoblast morphology and adhesionInt J Nanomedicine20083222924118686782
- YaoCSlamovichEBWebsterTJEnhanced osteoblast functions on anodized titanium with nanotube-like structuresJ Biomed Mater Res A200885115716617688267
- WooKMChenVJMaPXNano-fibrous scaffolding architecture selectively enhances protein adsorption contributing to cell attachmentJ Biomed Mater Res A200367253153714566795
- KhangaDKimSYLiu-SnyderPEnhanced fibronectin adsorption on carbon nanotube/poly(carbonate) urethane: Independent role of surface nano-roughness and associated surface energyBiomaterials200728324756476817706277
- WebsterTJErgunCDoremusRHSiegelRWBiziosRSpecific proteins mediate enhanced osteoblast adhesion on nanophase ceramicsJ Biomed Mater Res200051347548310880091
- ColonGWardBCWebsterTJIncreased osteoblast and decreased Staphylococcus epidermidis functions on nanophase ZnO and TiO2J Biomed Mater Res A200678359560416752397
- PuckettSDTaylorERaimondoTWebsterTJThe relationship between the nanostructure of titanium surfaces and bacterial attachmentBiomaterials201031470671319879645
- ASTM D7334-08 Standard Practice for Surface Wettability of Coatings, Substrates, and Pigments by Advancing Contact Angle Measurement2008
- BrownAMA step-by-step guide to non-linear regression analysis of experimental data using a Microsoft Excel spreadsheetComput Methods Programs Biomed200165319120011339981
- MezzasalmaSCharacterization of silicon nitride surface in water and acid environment: a general approach to the colloidal suspensionsJ Colloid Interface Sci19961802413420
- LewisJAColloidal processing of ceramicsJ Am Ceram Soc2000591023412359
- RoesslerSZimmermannRScharnweberDCharacterization of oxide layers on Ti6Al4V and titanium by streaming potential and streaming current measurementsColloids Surf B Biointerfaces2002264387395
- MaJWangCLiangCHColloidal and electrophoretic behavior of polymer particulates in suspensionMater Sci Eng C Mater Biol Appl2007274886889
- KimSBaikSHot isostatic pressing of sintered silicon nitrideJ Am Ceram Soc199174717351738
- BartonAJSagersRDPittWGBacterial adhesion to orthopedic implant polymersJ Biomed Mater Res19963034034108698704
- KatsikogianniMMissirlisYFConcise review of mechanisms of bacterial adhesion to biomaterials and of techniques used in estimating bacteria-material interactionsEur Cell Mater20048375715593018
- RosenthalVDMakiDGJamulitratSInternational Nosocomial Infection Control Consortium (INICC) report, data summary for 2003–2008, issued June 2009Am J Infect Control201038295104e220176284
- ZimmerliWTrampuzAOchsnerPEProsthetic-joint infectionsN Engl J Med2004351161645165415483283
- SenthiSMunroJTPittoRPInfection in total hip replacement: meta-analysisInt Orthop201135225326021085957
- KnetschMLWKooleLHNew strategies in the development of antimicrobial coatings: the example of increasing usage of silver and silver nanoparticlesPolymers201131340366
- HuangH-LChangY-YLaiM-CAntibacterial TaN-Ag coatings on titanium dental implantsSurf Coat Technol2010205516361641
- ZieglerGGollwitzerHHeidenauFMitteimeierWStenzelFAntiinfectious, biocompatible titanium coating for implants, and method for the production thereof US Patent 7,906,1323152011
- NeumannH-GPrinzCMethod for producing an anti-infective coating on implants US Patent Application Publication 2012/0024712222012
- ZhaoLChuPKZhangYWuZAntibacterial coatings on titanium implantsJ Biomed Mater Res B Appl Biomater200991147048019637369
- HamiltonVYuanYRigneyDBone cell attachment and growth on well-characterized chitosan filmsPolym Int2007104641647
- ShiZNeohKGKangETPohCWangWBacterial adhesion and osteoblast function on titanium with surface-grafted chitosan and immobilized RGD peptideJ Biomed Mater Res A200886486587218041731
- JouC-HYuanLLinSBiocompatibility and antibacterial activity of chitosan and hyaluronic acid immobilized polyester fibersJ Appl Polym Sci2007104220225
- IkedaTHirayamaHYamaguchiHTazukeSWatanabeMPolycationic biocides with pendant active groups: molecular weight dependence of antibacterial activityAntimicrob Agents Chemother19863011321353092730
- ChuaP-HNeohK-GKangE-TWangWSurface functionalization of titanium with hyaluronic acid/chitosan polyelectrolyte multilayers and RGD for promoting osteoblast functions and inhibiting bacterial adhesionBiomaterials200829101412142118190959
- CharvilleGHetrickEGeerCReduced bacterial adhesion to fibrinogen-coated substrates via nitric oxide releaseBiomaterials200829304039404418657857
- YoshinariMOdaYKatoTOkudaKHirayamaAInfluence of surface modifications to titanium on oral bacterial adhesion in vitroJ Biomed Mater Res200052238839410951380
- YoshinariMOdaYKatoTInfluence of surface modifications to titanium on antibacterial activity in vitroBiomaterials2001221211085377
- KatsikogianniMSpiliopoulouIDowlingDPMissirlisYFAdhesion of slime producing Staphylococcus epidermidis strains to PVC and diamond-like carbon/silver/fluorinated coatingsJ Mater Sci Mater Med200617867968916897160
- RaulioMJärnMAholaJMicrobe repelling coated stainless steel analysed by field emission scanning electron microscopy and physicochemical methodsJ Ind Microbiol Biotechnol200835775176018379832
- LiBLoganBEBacterial adhesion to glass and metal-oxide surfacesColloids Surf B Biointerfaces2004362819015261011
- VisaiLDe NardoLPuntaCTitanium oxide antibacterial surfaces in biomedical devicesInt J Artif Organs201134992994622094576
- VogelyHOosterbosCPutsEEffects of hydroxyapatite coating on Ti6Al4V implant site infection in a rabbit tibial modelJ Orthop Res20001848549310937638
- OosterbosCVogelyHNijhofMOsseointegration of hydroxy-apatiteöcoated and noncoated Ti6Al4V implants in the presence of local infection: a comparative histomorphometrical study in rabbitsJ Biomed Mater Res20026033934711920656
- BriemDStrametzSSchröderKResponse of primary fibroblasts and osteoblasts to plasma treated polyetheretherketone (PEEK) surfacesJ Mater Sci Med2005167671677
- Olivares-NavarreteRGittensRSchneiderJOsteoblasts exhibit a more differentiated phenotype and increased bone morphogenetic protein production on titanium alloy substrates than on polyether-ether-ketoneSpine J201212326527222424980
- SchwartzZRazPZhaoGEffect of micrometer-scale roughness of the surface of Ti6Al4V pedicle screws in vitro and in vivoJ Bone Joint Surg Am200890112485249818978418
- TruongVKLapovokREstrinbYSThe influence of nano-scale surface roughness on bacterial adhesion to ultrafine-grained titaniumBiomaterials201031133674368320163851
- SinghAVVyasVPatilRQuantitative characterization of the influence of the nanoscale morphology of nanostructured surfaces on bacterial adhesion and biofilm formationPloS One201169e2502921966403
- HilbertLRBagge-RavnDKoldJGramLInfluence of surface roughness of stainless steel on microbial adhesion and corrosion resistenceInt Biodeterior Biodegradation2003523175185
- FlintSHBrooksJDBremerPJProperties of the stainless steel substrate influencing the adhesion of thermo-resistant streptococciJ Food Eng2000434235242
- AnselmeKDavidsonPPopaMThe interaction of cells and bacteria with surfaces structured at the nanometre scaleActa Biomater20106103824384620371386
- WhiteheadKAColligonJVerranJRetention of microbial cells in substratum surface features of micrometer and sub-micrometer dimensionsColloids Surf B Biointerfaces2005412–312913815737538
- XuL-CSiedleckiCSubmicron-textured biomaterial surface reduces staphylococcal bacterial adhesion and biofilm formationActa Biomater201281728121884831
- DalbyMJRiehleMOSutherlandDSAgheliHCurtisASGFibroblast response to a controlled nanoenvironment produced by colloidal lithographyJ Biomed Mater Res A200469231432215058004
- BagnoAGenoveseMLuchiniAContact profilometry and correspondence analysis to correlate surface properties and cell adhesion in vitro of uncoated and coated Ti and Ti6Al4V disksBiomaterials200425122437244514741609
- RajyalakshmiAErcanBBalasubramanianKWebsterTJReduced adhesion of macrophages on anodized titanium with select nanotube surface featuresInt J Nanomedicine201161765177121980239
- AnagnostouFDebetAPavon-DjavidGOsteoblast functions on functionalized PMMA-based polymers exhibiting Staphylococcus aureus adhesion inhibitionBiomaterials200627213912391916564569