Abstract
Biocompatibility and biomechanical stability are two of the main obstacles limiting the effectiveness of vascular scaffolds. To improve the biomechanical stability and biocompatibility of these scaffolds, we created a heparin-nanomodified acellular bovine jugular vein scaffold by alternating linkage of heparin and dihydroxy-iron via self-assembly. Features of the scaffold were evaluated in vitro and in vivo. Heparin was firmly linked to and formed nanoscale coatings around the fibers of the scaffold, and the amount of heparin linked was about 808 ± 86 μg/cm2 (101 ± 11 USP/cm2) per assembly cycle. The scaffolds showed significantly strengthened biomechanical stability with sustained release of heparin for several weeks in vitro. Importantly, the modified scaffolds showed significantly reduced platelet adhesion, stimulated proliferation of endothelial cells in vitro, and reduced calcification in a subcutaneous implantation rat model in vivo. Heparin nanomodification improves the biocompatibility and biomechanical stability of vascular scaffolds.
Introduction
Vascular grafts have been used widely in cardiovascular disease, cancer, trauma, organ transplantation, and microsurgical reconstruction. Vascular graft substitutes include autologous vessels, vascular allografts, vascular xenografts, and synthetic vascular grafts.Citation1,Citation2 Autologous vessels and vascular allografts are ideal alternative vessels, but an insufficient supply of donor vessels limits their widespread clinical application.Citation3 Synthetic vascular grafts perform well in large vessel reconstruction, and there is a plentiful supply, but low graft patency greatly limits surgical utilization of small-caliber synthetic grafts because of thrombosis.Citation1,Citation4
We recently reported on in vivo use of decellularized bovine jugular vein (BJV) scaffolds to reconstruct pulmonary and right ventricular tissue in a dog model, with potential regeneration.Citation5–Citation7 The acellular vascular scaffolds were able to retain the tensile strength and unique extracellular matrix composition of native vessels, and thus could serve as an alternative blood vessel.Citation8,Citation9 Acellular BJV is a biological xenograft with a retained natural valve, and glutaraldehyde-treated BJV (Contegra®, Medtronic Inc, Minneapolis, MN) provides a promising substitute for surgical valvular reconstruction of the right ventricular outflow tract.Citation10–Citation12 However, poor biocompatibility and biomechanical stability of acellular BJV scaffolds, including poor endothelialization, thrombus formation, aneurysm formation, and calcification, limit the clinical effectiveness of these scaffolds.Citation10–Citation12
Poor biocompatibility can induce thrombogenicity. Thrombus formation is one reason for transplant failure.Citation5–Citation7,Citation13 To prevent early thrombosis after vascular xenograft implantation, systemic anticoagulant therapy is commonly used and this increases the risk of bleeding complications.Citation13 A thromboresistant surface or endothelialization of a vascular graft can effectively prevent thrombosis. Establishing a thromboresistant surface is crucial to prevent formation of blood clots in the initial phase of graft implantation.Citation14
Poor biomechanical stability can induce aneurysms. Formation of an aneurysm is another reason for transplant failure because of poor biomechanical stability of xenografts.Citation10–Citation12 Glutaraldehyde is used to improve the stability of a xenograft, but this procedure increases the cytotoxicity of xenografts and induces thrombogenicity.Citation10–Citation12
Heparin is a conventional anticoagulant and can be linked to the surface of a vascular graft.Citation15 This approach can reduce thrombus formation without bleeding complications and also provide a substrate to bind growth factors, including basic fibroblast growth factor, epithelial growth factor, and vascular endothelial growth factor, which accelerate endothelialization and regeneration.Citation14,Citation16–Citation18 Heparin can be linked to an extracellular matrix using a crosslinker such as glutaraldehyde, 1-(3-dimethylaminopropyl)-3-ethylcarbodiimide hydrochloride (EDC), or chitosan, but the amount of heparin linked is small, and long-term results are not ideal.Citation14,Citation19–Citation21 Much effort has been devoted to improving the antithrombogenicity of xenogeneic skin grafts by surface modification using anticoagulant molecules. Dai et al reported that complex multilayers of iron polysaccharide, which are used to modify a nitinol surface, provide a stable thromboresistant surface with good biocompatibility.Citation22
In the present study, our main objective was to develop a decellularized vascular scaffold with heparin nanomodification, and to improve the biocompatibility and biomechanical stability of acellular vascular scaffolds. Stable heparin-nanomodified scaffolds were created by alternating linkage of heparin and dihydroxy-iron (DHI) to the scaffolds via layer-by-layer self-assembly. Furthermore, we evaluated the biocompatibility and biomechanical stability of the modified vascular scaffold in vitro and in vivo, using an unmodified counterpart as a control. Heparin-nanomodified scaffolds could provide a stable antithrombogenic surface and significantly improve biomechanical stability and biocompatibility compared with unmodified scaffolds. The results of this study suggest a new method for developing tissue-engineered vascular grafts.
Materials and methods
Materials and reagents
Heparin sodium (molecular weight 10,000–15,000, 125 USP/mg) was purchased from Sigma Ltd (St Louis, MO) and ferric chloride hexahydrate (FeCl3 · 6H2O, molecular weight 270.8) was obtained from Tianda Chemical (Tianjing, China).
Preparation of DHI ([Fe(OH)2]+) solution was as follows. FeCl3 · 6H2O was dissolved in deionized water, and its pH was slowly adjusted to 3.0 with NaOH solution while stirring (molar ratio of NaOH to FeCl3 is 2 to 1). Its concentration was then adjusted to 0.05 mol/L with deionized water.
Preparation of acellular BJV scaffolds was as follows. BJVs from 300–500 kg cattle beasts were obtained from a local slaughterhouse and stored in chilled normal saline. The loose connective tissue and fat on the outer surface of the BJVs was removed in the laboratory. The multistep detergent-enzymatic decellularization procedure has been described in detail previously.Citation5–Citation7
Modification procedure
Acellular BJV scaffolds were modified as follows. First, the acellular BJV scaffolds were trimmed to the desired shape and size, immersed in 0.05 mol/L DHI solution for 10 minutes at room temperature, and then rinsed three times with 0.9% NaCl solution for 5 minutes. Next, the scaffolds were immersed in 5 mg/mL heparin solution containing 0.9% NaCl for 5 minutes at room temperature, followed by washing three times with 0.9% NaCl solution for 5 minutes. Subsequently, the scaffolds were immersed in 0.05 mol/L DHI solution for 5 minutes at room temperature. Finally, after completion of the desired number of cycles, the scaffolds were removed, rinsed with 0.9% NaCl solution, and stored in 5 mg/mL heparin solution containing 0.9% NaCl at 4°C for further use.
Quantification of linked heparin
The toluidine blue colorimetric method was used to measure the initial and residual concentration of heparin solution before and after each assembly cycle, and the amount of linked heparin was calculated.Citation23,Citation24 In brief, acellular BJV scaffolds were cut into square sheets (1 cm × 1 cm, n = 12) and modified as described above. The initial concentration of heparin solution was detected by the toluidine blue colorimetric method first, then the residual concentration of heparin solution was detected after the sheets were removed from the heparin solution during each assembly cycle. Absorbance was recorded at 630 nm using an ultraviolet spectrophotometer (UV-2450 Shimadzu Corporation, Tokyo, Japan), and the amount of linked heparin per cm2 per cycle was calculated. The amount of linked heparin was calculated as (the initial concentration of heparin solution – the residual concentration of heparin solution) × the volume of heparin solution.
Surface ultrastructure
Scanning electron microscopy (SEM, Nova NanoSEM 230, FEI Company, Hillsboro, OR) was used to evaluate the variances in surface ultrastructure between unmodified and heparin/DHI-modified BJV scaffolds. In brief, acellular BJV scaffolds were cut into vessel sheets (1 cm × 1 cm) and modified by one, four, seven, ten, and 13 cycles of heparin/DHI coating as described earlier, with unmodified BJV scaffolds were used as the control. All samples were fixed in 4% glutaraldehyde for 24 hours, rinsed in phosphate-buffered saline, postfixed in 1% OsO4 for 2 hours, followed by a brief wash with distilled water and then dehydration with graded ethanol. The samples were then critical-point dried, mounted luminal side up, sputter-coated with gold, and examined using SEM (JEOL-6490 LV, JEOL, Tokyo, Japan).
Histological characteristics
Linked heparin in tissue sections can be stained with toluidine blue and visualized using light microscopy.Citation14,Citation25 Briefly, the formaldehyde-fixed BJV scaffold samples modified or not modified by seven cycles heparin/DHI were embedded in paraffin and cut into slices. The histological sections were deparaffinized and hydrated routinely, then stained with 0.1% toluidine blue for 10 minutes. Next, they were dehydrated and mounted in a routine manner. Photographs were taken under a light microscope (Nikon E600, Tokyo, Japan).
Washing test
To study the binding force of the heparin/DHI coating on the surface of the BJVs, the shaken-wash model was used, which mimics resting heart rate, body temperature, and exposure of the heparin/DHI coatings to fluid flow stress.Citation22 In brief, acellular BJV scaffolds were cut into square sheets (1 cm × 1 cm, 10 samples each) and modified using four, seven, and ten cycles of heparin/DHI as described above. Each sheet was subjected to a tangential shaking wash in 40 mL of phosphate-buffered saline at 37°C, and 0.2 mL samples of the washed solution were removed after washing for one day and one, 2, 4, 6, and 8 weeks. After removal, the heparin concentration in the samples was determined using the toluidine blue colorimetric method. Absorbance was recorded as described above, and the cumulative amount of heparin released was calculated. The ultrastructure of the luminal surface of the BJV scaffold modified by seven cycles of heparin/DHI was examined after 8 weeks of washing as described above.
Biomechanical stability
A tensile test was used to evaluate the biomechanical stability of the heparin/DHI-modified scaffolds.Citation6,Citation7 Briefly, 20 pieces of acellular BJV scaffolds which were more than 1 cm away from the vein valve sinus were cut transversely into six portions. Five portions of scaffolds were transversely cut into vessel sheets (1 cm × 4 cm) and modified by one, four, seven, ten, and 13 cycles of heparin/DHI as described above, and the other scaffolds were used as controls. The elastic modulus, maximum load, and maximum tensile stress of the modified and unmodified scaffolds were tested using a tensile test machine (Instron Co, Norwood, MA).
Antithrombogenic activity in vitro
The activated partial thrombin time (APTT) and prothrombin time are used widely to evaluate the antithrombogenic activity of biomaterials.Citation22 In brief, BJV scaffolds modified by seven cycles of heparin/DHI were cut into square sheets (1 cm × 1 cm), and every sheet was washed in 40 mL of phosphate-buffered saline at 37°C under 80 rpm shaking conditions. The sheets were removed after one day and one, 4, 6, and 8 weeks of washing (eight samples each), and then rinsed three times with phosphate-buffered saline for 3 minutes. Unmodified BJV scaffolds were also cut into sheets (n = 8) and used as controls. Each sheet was then placed carefully in a tube containing 1 mL of citrate-anticoagulated blood (obtained from volunteer donors) at 37°C for 60 minutes, and the blood was next centrifuged for 15 minutes at 3000 rpm to obtain the plasma. The APTT and prothrombin time of the resulting plasma were detected using an automatic blood coagulation analyzer (CA6000, Sysmex Ltd, Kobe, Japan).
Platelet adhesion in vitro
The biocompatibility of biomaterials can be investigated by evaluation of platelet adhesion.Citation22,Citation26 Briefly, platelet-rich plasma was generated by centrifugation for 15 minutes at 750 rpm. Scaffolds modified by seven cycles of heparin/DHI were then cut into square sheets (1 cm × 1 cm, n = 6), and every sheet was positioned in contact with 1 mL of platelet-rich plasma for 3 hours in a humidified 5% CO2 air atmosphere at 37°C. Unmodified scaffold sheets (n = 6) were used as the control. The specimens were fixed in 4% glutaraldehyde solution for 24 hours, then washed in phosphate-buffered saline three times and dehydrated using graded ethanol. Next, the specimens were dried in a vacuum dryer, sputter-coated with gold, and observed using SEM.
Cytotoxicity assay in vitro
An in vitro cytotoxicity assay was used to evaluate the cytotoxicity of the BJV scaffolds modified with heparin/DHI. In brief, BJV scaffolds modified with seven cycles of heparin/DHI were sheared into 1.0 cm diameter disks (n = 48) and sterilized using gamma-ray irradiation at 20 KGy. Human EA.hy926 endothelial cells (1 × 105 cells) from a spontaneously transformed human umbilical vein endothelial cell line (ScienCell Co, San Diego, CA) were seeded onto the luminal surface of the modified BJV scaffolds in 48-well culture plates.Citation18,Citation27 The disks were incubated in a humidified atmosphere of 5% CO2 and 95% air at 37°C for one, 4, 7, and 10 days (12 disks each). Unmodified BJVs (n = 48) were used as the control. Dulbecco’s Modified Eagle’s Medium (Hyclone Ltd, Logan, UT) supplemented with 10% fetal bovine serum (Hyclone Ltd) was used as the culture medium and changed routinely every 2 days.
At the end of the culture period, each group of disks was divided into two portions. One portion was subjected to MTT assay as follows. The disks were incubated for 4 hours in serum-free medium with 0.8 mg/mL MTT (Sigma Ltd), then washed briefly with phosphate-buffered saline, followed by shaking in 1 mL of dimethyl sulfoxide (Sigma Ltd) for 10 minutes. Next, 0.2 mL samples of the resulting solution were transferred into 96-well plates, and absorbance was recorded at 560 nm using a microplate spectrophotometer (Bio-Tek Instruments Inc, Winooski, VT). The other portion of the disks was assayed by light microscopy. For this examination, the disks were stained with DioC18 solution (Beyotime Institute of Biotechnology, Haimen, China) for 10 minutes, and photographs were taken under a fluorescence microscope (E600, Nikon Corporation, Tokyo, Japan).Citation28
Anticalcification in a rat model
Subcutaneous implantation was used to evaluate calcification in the BJV scaffolds modified using heparin/DHI. Briefly, 20 Sprague-Dawley rats (140–150 g) were obtained from the animal department of Central South University. Sheets of BJV scaffolds (1 cm × 1 cm, n = 20) modified using seven cycles of heparin/DHI or not (control scaffold sheets) were subcutaneously implanted symmetrically on both sides of the rat spine and retrieved after 30 days (n = 10) or 60 days (n = 10). Rat handling complied with the requirements for care and use of animals according to National Institutes of Health and Chinese guidelines. The samples retrieved were dried to a constant weight in a desiccator oven and hydrolyzed with HNO3 (10 mL, 0.75 mol/L) at 70°C for 15 hours. After centrifugation (15 minutes, 3000 rpm), the fluid was removed and diluted, and the calcium content was determined using a fast sequential atomic absorption spectrometer (Varian AA240FS, Varian Inc, Palo Alto, CA).Citation29
Statistical analysis
All values are expressed as the mean ± standard deviation. The statistical analysis was performed using analysis of variance followed by the Student-Newman-Keuls test to compare the groups in a pairwise manner. The statistical analysis was performed using the Statistical Package for Social Sciences, Windows version 18.0 (SPSS Inc, Chicago, IL). A P < 0.05 was considered to be statistically significant.
Results
Gross appearance
The gross appearance of the scaffolds is shown in and . The vessel walls and valves with unmodified BJV scaffolds () and the scaffolds modified by heparin/DHI () had a similar shape, flexibility, and texture. However, the color of the luminal and outer surfaces of the BJV scaffolds modified by heparin/DHI changed from white to golden yellow, whereas the middle layer of the modified scaffolds remained white (). The intensity of the golden yellow color appeared to increase with an increase in the number of assembly cycles ().
Quantification of linked heparin
We detected the initial and residual concentrations of heparin solution using the toluidine blue colorimetric method and calculated the amount of linked heparin. The results show that the average amount of linked heparin was about 808 ± 86 μg/cm2 (101 ± 11 USP/cm2) per cycle, and the mean amount of linked heparin was about 3.23 ± 0.34, 5.46 ± 0.60, and 8.80 ± 0.86 mg/cm2 after four, seven, and ten cycles, respectively.
Surface ultrastructure and histological characteristics
SEM images of the ultrastructure of the luminal surface () showed that heparin and DHI were linked to and formed nanoscale coatings around the fibrils of the scaffolds. The coatings of the BJV scaffolds modified by four, seven, and ten cycles of heparin/DHI were uniform, but the coatings of scaffolds modified by 13 cycles of heparin/DHI were not uniform. We compared the diameters of fibrils from the BJV scaffolds modified by heparin/DHI with those of their unmodified counterparts, and the results show that the thickness of the heparin/DHI coatings increased with an increase in the number of assembly cycles; the thickness of one-cycle heparin/DHI coating was about 25 nm, and the thicknesses of BJV scaffolds modified by four, seven, and ten cycles of heparin/DHI coating were about 95, 160, and 260 nm, respectively. However, if assembly involved more than seven cycles, adjacent fibrils could be fused with each other by the heparin/DHI complexes.
Figure 3 Luminal surface ultrastructure of scaffolds. (A) SEM image of unmodified scaffold (10,000×). (B) SEM image of a scaffold modified by one cycle of heparin/DHI (10,000×). (C) SEM image of scaffold modified by four cycles of heparin/DHI (10,000×). (D) SEM image of scaffold modified by seven cycles of heparin/DHI (10,000×). (E) SEM image of scaffold modified by ten cycles of heparin/DHI (10,000×). (F) SEM image of scaffold modified by one cycle of heparin/DHI (20,000×).
Abbreviations: DHI, dihydroxy-iron; SEM, scanning electron microscope.
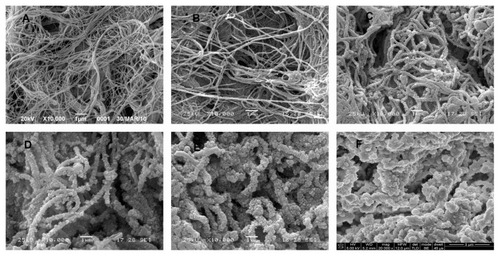
Photographs of sections stained using toluidine blue () showed that both the outer and luminal layers of the heparin/DHI-modified scaffolds () were stained deeply, whereas the middle layers of BJV scaffolds modified by heparin/DHI were stained lightly but more deeply than in their unmodified counterparts ().
Figure 4 Toluidine blue staining of scaffolds (200×). (A) Toluidine blue staining shows the outer and middle layers of BJV scaffolds modified by heparin/DHI stained by toluidine blue, with the outer layer stained more deeply than the middle layer. The arrows indicate positive staining. (B) Toluidine blue staining shows that the unmodified BJV scaffolds could not be stained by toluidine blue.
Abbreviations: BJV, bovine jugular vein; DHI, dihydroxy-iron.
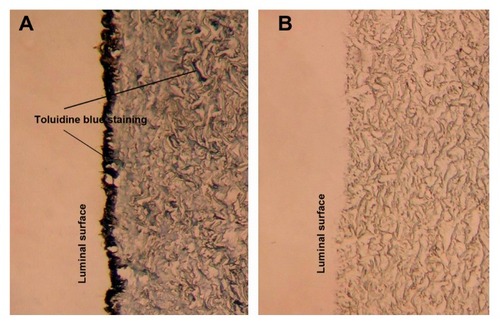
Stability of heparin/DHI coatings
The modified scaffolds still retained their golden yellow color after 8 weeks of washing. The ultrastructure of the luminal surface of the scaffolds modified by heparin/DHI after 8 weeks of washing is shown in . There was a large amount of heparin left in the scaffold, and the remains of the heparin/DHI coating could be found around the fibrils. Cumulative release of heparin is shown in . The results indicate that the heparin/DHI coating released heparin in a sustained manner for at least 8 weeks. There was no significant difference in the amount of heparin released between the scaffolds modified by heparin/DHI using different cycles.
Table 1 Cumulative release of heparin from heparin/DHI modified scaffolds (n = 10)
Strengthened biomechanical stability
The results of the tensile test are shown in and . Compared with the unmodified scaffolds, the elastic modulus, maximum load, and maximum tensile stress of the heparin/DHI-modified scaffolds were significantly increased. There were significant differences between the unmodified and heparin/DHI-modified scaffold groups (P < 0.01). The biomechanical stability of the modified scaffolds increased with an increase in the number of assembly cycles. However, biomechanical stability was not significantly increased after ten cycles.
Table 2 Biomechanical stability of unmodified and heparin/DHI-modified scaffolds (n = 20)
Figure 6 Line graphs showing tensile test results.
Note: Tensile curves show that the elastic modulus, maximum load, and maximum tensile stress of heparin/DHI-modified scaffolds increased along with an increase in the number of assembly cycles, but with no significant increase after ten cycles of nanomodification.
Abbreviation: DHI, dihydroxy-iron.
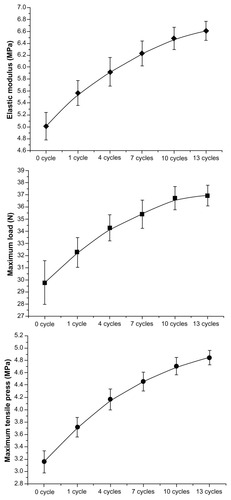
Increased antithrombogenic activity
The results of the coagulation activity assay are shown in . The prothrombin time and APTT of the unmodified scaffolds were within the normal reference range, but above the upper limit of the normal range in all the heparin/DHI-modified scaffold groups. It is important to note that the prothrombin time and APTT of the modified sheets removed after one day and one week of washing were above the upper detection limit of the blood coagulation analyzer (>120 seconds for prothrombin time and >180 seconds for APTT). There was a significant difference in antithrombogenic activity between the unmodified and heparin/DHI-modified scaffold groups, regardless of washing duration (P < 0.01).
Table 3 Prothrombin time and APTT of unmodified and heparin/DHI-modified scaffolds (n = 8)
Reduced platelet adhesion
SEM images of platelets adhered to the luminal surface () indicate that the heparin/DHI-modified scaffolds drastically decreased surface platelet adhesion compared with their unmodified counterparts. Large numbers of platelets adhered to and aggregated in some regions of the unmodified sheets (), but few platelets were found on the luminal surfaces of the heparin/DHI-modified sheets (). Mean platelet counts per 10,000 μm2 area for the heparin/DHI-modified and unmodified scaffolds were 8 ± 4 and 48 ± 16, respectively. The difference between the two groups was significant (P < 0.01).
Stimulated proliferation of endothelial cells
Photographs of DioC18 staining are shown in . The results of this assay show that human EA.hy926 endothelial cells could adhere to and proliferate on the luminal surface of both heparin/DHI-modified and unmodified scaffolds. The number of endothelial cells on the luminal surface of a heparin/DHI-modified sheet () was less than the number on the luminal surface of an unmodified sheet () after one day of incubation. After 4 days of incubation, the number of endothelial cells on the luminal surface of the modified sheet () was very similar to that on the surface of its unmodified counterpart (). However, the number of endothelial cells on the luminal surface of the unmodified scaffold () was less than the number on the luminal surface of the heparin/DHI-modified scaffold sheet () after incubation for 7 and 10 days. In particular, at 10 days of incubation, the morphology of the endothelial cells on the luminal surface of the unmodified scaffold sheets changed to being smaller and thinner than that on the heparin/DHI-modified scaffold sheets.
Figure 8 DioC18 staining of endothelial cells on the luminal surface of scaffolds (200×). (A-D) are photographs of endothelial cells on the luminal surface of unmodified scaffolds after incubation for one, 4, 7 and 10 days, respectively. (E-H) are photographs of endothelial cells on the luminal surface of heparin/DHI-modified scaffolds after incubation for one, 4, 7 and 10 days, respectively.
Abbreviation: DHI, dihydroxy-iron.
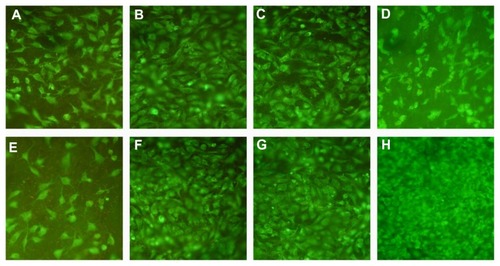
The MTT assay showed similar results. Mean OD values were 0.21 ± 0.04, 0.39 ± 0.03, 0.58 ± 0.0,6 and 0.65 ± 0.08 for heparin/DHI-modified sheets after incubation for one, 4, 7, and 10 days, respectively. Corresponding mean OD values for the unmodified sheets were 0.32 ± 0.03, 0.40 ± 0.04, 0.38 ± 0.05, and 0.28 ± 0.08 after incubation for one, 4, 7, and 10 days, respectively. There were significant differences between OD values obtained for unmodified and heparin/DHI-modified scaffolds after incubation for one, 7, and 10 days (P < 0.01).
Reduced calcification
The modified scaffolds retrieved after 30 or 60 days retained their golden yellow color (). Mean calcium content () after 30 days of incubation for the heparin/DHI-modified and unmodified BJV scaffolds was 8.5 ± 1.9 and 46.6 ± 3.7 μg/mg dry weight, respectively, and at 60 days was 12.5 ± 6.8 and 112.6 ± 16.9 μg/mg dry weight. There was a significant difference in calcium content between the heparin/DHI-modified and unmodified scaffolds at both time points (P < 0.01).
Discussion
In the current study, a stable heparin/DHI-nanomodified acellular vascular xenograft scaffold was developed by alternating dative covalent linkage of DHI ions and heparin molecules onto BJV scaffolds using layer-by-layer self-assembly. The mechanisms for establishing heparin/DHI complexes may be as follows. Iron ion, an essential trace element in the human body, is a typical transition metal cation that accepts electron pairs. DHI ions are formed when FeCl3 solution mixes slowly with NaOH solution, and the chemical equation is Fe3+ + 2OH− = [Fe(OH)2]+. Heparin, a highly sulfated linear polysaccharide, contains large numbers of hydroxyl, amino, carboxyl, sulfonyl, and other groups, and these groups are typical Lewis bases that donate electron pairs.Citation15,Citation30,Citation31 Positively charged DHI ions can be combined with negatively charged sulfonic or carboxylic acids of heparin molecules by ionic bonds, and they can also be chemically bonded with the carboxyl, sulfonyl, hydroxyl, or amino groups of heparin molecules by dative covalent bonds. Hence, heparin/DHI complexes are formed when heparin molecules and DHI ions chemically bond with each other. The extracellular matrix, which consists of collagen fibers, elastic fibers, fibronectin, laminin, glycosaminoglycan, and proteoglycan, also contains large numbers of carboxyl, hydroxyl, amino, and other groups that can donate electron pairs.Citation15,Citation32 As described above, DHI ions can also be chemically bonded to the extracellular matrix by dative covalent bonds.
The self-assembly procedure may occur as follows. Firstly, when acellular BJV scaffolds are immersed in DHI solution, DHI ions are chemically combined with the carboxyl, hydroxyl, or amino groups of the extracellular matrix by dative covalent bonds and linked to the scaffolds. Secondly, when the scaffolds are immersed in heparin solution, the carboxyl, sulfonyl, hydroxyl, or amino groups on the heparin molecules are chemically combined with the DHI ions linked into the extracellular matrix by dative covalent bonds, and heparin molecules are linked onto the scaffolds. Thirdly, when the scaffolds are immersed in DHI solution again, DHI ions are chemically combined with the remaining carboxyl, sulfonyl, hydroxyl, or amino groups of the linked heparin molecules in the extracellular matrix and linked to the scaffolds. Finally, after completion of several cycles, large numbers of heparin molecules and DHI ions are alternately linked to scaffolds and form heparin/DHI complex coatings around the fibrils.
In this study, quantification showed that the average amount of linked heparin was about 808 ± 86 μg/cm2 (101 ± 11 USP/cm2) per assembly cycle, and the total amount of linked heparin was about 8.80 ± 0.86 mg/cm2 (1040 ± 110 USP/cm2) after ten cycles. In contrast, only 6.21 ± 2.03 USP/cm2 of heparin was linked to the extracellular matrix when EDC was used as the crosslinker.Citation14 These results indicate that more heparin molecules are linked to the extracellular matrix when using DHI than when using EDC. Our recent test results show that the average amount of linked iron ion was about 178 ± 46 μg/cm2 per assembly cycle.Citation33 Iron ion is an essential trace element used by the human body to produce red blood cells, and DHI is nontoxic to humans.
Heparin/DHI coatings could be visualized directly using SEM, which suggests that a large number of heparin molecules were linked to the scaffolds. SEM images of the luminal surface ultrastructure show that the thickness of a one-cycle heparin/DHI coating was about 25 nm, suggesting that heparin/DHI complex coatings are nanoscale films.Citation34,Citation35 Increasing the number of assembly cycles should increase the thickness of the heparin/DHI coating, and if the number of assembly cycles increased enough, the heparin/DHI complexes would fill up the spaces between adjacent fibrils and form a microscale coating on the surface of the scaffold.
The current results show that both the outer and luminal layers of the heparin/DHI-modified vascular scaffold were stained deeply by toluidine blue, whereas the middle layer of the modified scaffold was stained lightly but more deeply than the unmodified scaffolds. These observations suggest that the heparin molecules were mainly linked to the surface of the scaffolds, with a little heparin linked to the middle layer.
The results of the tensile test show that the elastic modulus, maximum load, and maximum tensile stress in the heparin/DHI-modified scaffolds were increased significantly compared with unmodified scaffolds. Our findings suggest that nanomodification with heparin/DHI increased the biomechanical stability of the scaffold, which might be because the heparin/DHI complexes form crossbridges and crosslink adjacent fibrils. However, there was no significant difference in biomechanical stability between scaffolds modified by ten and 13 cycles of heparin/DHI, indicating that the crosslinked heparin/DHI complexes have limited biomechanical stability. Increasing the number of assembly cycles beyond ten may not increase the biomechanical stability of the scaffold further. The modified scaffold was stiffer than its unmodified counterpart, but softer than the glutaraldehyde-treated scaffold.
The modified scaffolds used in this study released heparin in a sustained manner during 8 weeks of incubation in phosphate-buffered saline. The amount of heparin released from a 1 cm2 area of scaffold sheets modified by four, seven, and ten cycles of heparin/DHI after 8 weeks of washing was approximately 1.88 mg (58%), 1.91 mg (35%), and 2.01 mg (23%), respectively. These results indicate that the heparin/DHI complexes were firmly linked to the BJV scaffolds, and more linked heparin enabled heparin release for a longer period of time. The prothrombin time and APTT of the modified BJV sheets after washing for one day and 8 weeks were above the upper limit of the normal reference ranges, indicating that heparin/DHI-modified BJV scaffolds retained antithrombogenic activity for at least 8 weeks. Therefore, modification with heparin/DHI complexes could provide a stable antithrombogenic surface for acellular BJV scaffolds and effectively resist thrombus formation. All these observations indicate that heparin/DHI-modified scaffolds might be more antithrombogenic than unmodified scaffolds.
Platelet adhesion increases the likelihood of mural thrombosis, so less platelet adhesion means less thrombogenicity. Compared with the unmodified scaffolds, adhesion of platelets to the surface of the heparin/DHI-modified scaffolds was dramatically decreased in this study, suggesting that the modified scaffolds should have better antithrombogenicity than the unmodified scaffolds.
It has been reported that iron ions and heparin molecules can protect biomaterials from calcification.Citation36–Citation38 We obtained similar results in our experiment. The calcium content of the heparin/DHI-modified scaffolds decreased significantly compared with that in the unmodified scaffolds, suggesting that the modified BJV scaffolds may reduce calcification.
In the current study, the number of endothelial cells on the surface of the heparin/DHI-modified scaffold sheets was less than the number on the surface of the unmodified sheets in the early period of culture, but endothelial cells could grow and proliferate on the luminal surface of the modified scaffolds more rapidly, and the number of endothelial cells on the surface of heparin/DHI-modified scaffolds was greater than that on the surface of the unmodified scaffolds after 10 days of incubation. Furthermore, growth of endothelial cells on the unmodified scaffolds had slowed down and stopped by 10 days of incubation. These findings indicate that nanomodification using heparin/DHI decreased adherence of endothelial cells in the initial period, but once adhered, these cells grew and proliferated rapidly on the luminal surface in vitro. Further, the heparin/DHI complexes were not cytotoxic.
Heparin molecules and DHI ions can be alternately linked to acellular vascular scaffolds and have four main actions. First, heparin can reduce the thrombogenicity of vascular xenografts and prevent formation of blood clots on the surface after implantation.Citation14 Second, coating the graft with heparin provides a substrate to bind growth factors, such as basic fibroblast growth factor, epithelial growth factor, and vascular endothelial growth factor, that may accelerate endothelialization and regeneration.Citation18,Citation39 Third, DHI ions and heparin molecules can reduce calcification of biomaterials, and this may minimize calcification in vascular xenografts. Fourth, heparin/DHI complexes can crosslink adjacent fibrils and strengthen the biomechanical stability of acellular tissue-engineered scaffolds.
The amount of heparin linked to the scaffolds increases with an increase in the number of assembly cycles. However, the optimal number of assembly cycles and amount of heparin that needs to be linked for maximum efficacy are not clear. Although heparin inhibits smooth muscle cell proliferation and neointimal formation, resists inflammation, and has other beneficial effects, it is not clear whether heparin/DHI-modified acellular vascular scaffolds have these same properties.Citation16,Citation40,Citation41 These issues still need further evaluation, and additional studies with heparin/DHI-nanomodified tissue-engineered vascular grafts implanted in animals are in progress.
Conclusion
We have created heparin-nanomodified acellular vascular xenografts by alternating dative covalent linkage of heparin and DHI on acellular BJV scaffolds via layer-by-layer self-assembly. Our initial results show that acellular vascular scaffolds modified with heparin/DHI complexes have excellent antithrombogenicity and biomechanical stability, resist calcification, decrease platelet adhesion, and stimulate proliferation of endothelial cells without cytotoxicity. This study suggests a new method for developing tissue-engineered vascular grafts. However, these results are preliminary, and further in vivo studies in animals with long-term implantation of these heparin/DHI-modified grafts are needed.
Acknowledgments
This project was supported financially by the National 863 Plan for the People’s Republic of China (2007AA071900), the National Natural Science Foundation of China (81071275), and the Natural Science Foundation of the Jiang’xi Province of China (20122BAB205098).
Disclosure
The authors report no conflicts of interest in this work.
References
- TorikaiKIchikawaHHirakawaKA self-renewing, tissue-engineered vascular graft for arterial reconstructionThorac Cardiovasc Surg20081363745
- TeebkenOEHaverichATissue engineering of small diameter vascular graftsEur J Vasc Endovasc Surg20022347548512093061
- CooperGJUnderwoodMJDeverallPBArterial and venous conduits for coronary artery bypass: a current reviewEur J Cardiothorac Surg1996101291408664004
- Luong-vanEGrondahILChuaKNLeongKWNurcombeVCoolSMControlled release of heparin from poly (epsilon-caprolactone) electrospun fibersBiomaterials2006272042205016305806
- LuWDYuFLWuZSSuperior vena cava reconstruction using bovine jugular vein conduitEur J Cardiothorac Surg20073281681717768061
- LuWDZhangMWuZSHuTHDecellularized and photooxidatively crosslinked bovine jugular veins as potential tissue engineering scaffoldsInteract Cardiovasc Thorac Surg2009830131519074454
- LuWDZhangMWuZSThe performance of photooxidatively crosslinked acellular bovine jugular vein conduits in the reconstruction of connections between pulmonary arteries and right ventriclesBiomaterials2010312934294320053442
- SchmidtCEBaierJMAcellular vascular tissues: natural biomaterials for tissue repair and tissue engineeringBiomaterials2000212215223111026628
- TschoekeBFlanaganTCCornelissenADevelopment of a composite degradable/nondegradable tissue-engineered vascular graftArtif Organs20083280080918684200
- RastanAJWaltherTDaehnertIBovine jugular vein conduit for right ventricular outflow tract reconstruction: evaluation of risk factors for mid-term outcomeAnn Thorac Surg2006821308131516996925
- OzkanSAkayTHGultekinBSezginATokelKAslamaciSXenograft transplantation in congenital cardiac surgery at Baskent Iniversity: midterm resultsTransplant Proc2007391250125417524946
- FioreACRuzmetovMHuynhDComparison of bovine jugular vein with pulmonary homograft conduits in children less than 2 years of ageEur J Cardiothoracic Surg201038318325
- CrikisSCowanPJd’ApiceAJIntravascular thrombosis in discordant xenotransplantationTransplantation2006821119112317102759
- LiaoDWangXLinPHYaoQChenCJCovalent linkage of heparin provides a stable anti-coagulation surface of decellularized porcine arteriesJ Cell Mol Med2009132736274319040421
- RabensteinDLHeparin and heparan sulfate: structure and functionNat Prod Rep20021931233112137280
- WangXNChenCZYangMGuYJImplantation of decellularized small-caliber vascular xenografts with and without surface heparin treatmentArtif Organs2007319910417298398
- BasmadjianDSeftonMVRelationship between release rate and surface concentration for heparinized materialsJ Biomed Mater Res1983175095186863352
- TanQTangHHuJControlled release of chitosan/heparin nanoparticle-delivered VEGF enhances regeneration of decellularized tissue-engineered scaffoldsInt J Nanomedicine2011692994221720505
- LeeWKParkKDHanDKSuhHParkJCKimYHHeparinized bovine pericardium as a novel cardiovascular bioprosthesisBiomaterials2000212323223011026639
- WissinkMJBeerninkRPieperJSImmobilization of heparin to EDC/NHS-crosslinked collagen. Characterization and in vitro evaluationBiomaterials20012215116311101159
- YuSHWuYBMiFLShyuSSPolysaccharide-based artificial extracellular matrix: preparation and characterization of three-dimensional, macroporous chitosan, and heparin composite scaffoldJ Appl Polym Sci200810936393644
- LiuMYueXDaiZNovel thrombo-resistant coating based on iron-polysaccharide complex multilayersACS Appl Mater Interfaces2009111312320355762
- BalamuruganKKalaichelvanADIsolation and identification of heparin like substances from the body tissue of conus musicusInt J Pharm Tech Res2009116501653
- AholaMSSäilynojaESRaitavuoMHVaahtioMMSalonenJIYli-UrpoAUIn vitro release of heparin from silica xerogelsBiomaterials2001222163217011432596
- SmithPKMalliaAKHermansonGTColorimetric method for the assay of heparin content in immobilized heparin preparationsAnal Biochem19801094664737224172
- OkrójWWalkowiak-PrzybyłoMRośniak-BakKKlimekLWalkowiakBComparison of microscopic methods for evaluating platelet adhesion to biomaterial surfacesActa Bioeng Biomech2009114549
- EmeisJJEdgellCJFibrinolytic properties of a human endothelial hybrid cell line (Ea.hy 926)Blood198871166916753130908
- ZhaoXKimJCezarCAActive scaffolds for on-demand drug and cell deliveryProc Natl Acad Sci U S A2011108677221149682
- PettenazzoEValenteMThieneGOctanediol treatment of glutaraldehyde fixed bovine pericardium: evidence of anticalcification efficacy in the subcutaneous rat modelEur J Cardiothorac Surg20083441842218550382
- NugentMAHeparin sequencing brings structure to the function of complex oligosaccharidesProc Natl Acad Sci U S A200097103011030310984527
- SasisekharanSVenkataramanGHeparin and heparan sulfate: biosynthesis, structure and functionCurr Opin Chem Biol2000462663111102866
- WagenseilJEMechamRPVascular extracellular matrix and arterial mechanicsPhysiol Rev20098995798919584318
- TaoYHuTWuZTangHHuYTanQHeparin-iron complex multilayer nanomodification improves hemocompatibility of decellular xenograftZhong Nan Da Xue Xue Bao Yi Xue Ban201237260266 Chinese22561507
- Von der MarkKParkJBauerSSchmukiPNanoscale engineering of biomimetic surfaces: cues from the extracellular matrixCell Tissue Res201033913115319898872
- ShekaranAGarciaAJNanoscale engineering of extracellular matrix-mimetic bioadhesive surfaces and implants for tissue engineeringBiochim Biophys Acta2011181035036020435097
- BaldwinMTCiesligaBLBarkasiLDWebbCLLong-term anticalcification effect of Fe3+ in rat subdermal implants of glutaraldehyde preserved bovine pericardiumASAIO Trans199137170172
- ValdesTIMoussyFA ferric chloride pre-treatment to prevent calcification of Nafion membrane used for implantable biosensorsBiosens Bioelectron19991457958511459103
- VasudevSCMosesLRSharmaCPCovalently bonded heparin to alter the pericardial calcificationArtif Cells Blood Substit Immobil Biotechnol20002824125310852675
- SinghSWuBMDunnJCThe enhancement of VEGF-mediated angiogenesis by polycaprolactone scaffolds with surface cross-linked heparinBiomaterials2011322059206921147501
- GargHGMrabatHYuLEffect of carboxyl-reduced heparin on the growth inhibition of bovine pulmonary artery smooth muscle cellsCarbohydr Res20103451084108720399420
- BeamishJAGeyerLCHaq-SiddiqiNAKottke-MarchantKMarchantREThe effects of heparin releasing hydrogels on vascular smooth muscle cell phenotypeBiomaterials2009306286629419709740