Abstract
This research was conducted to formulate biodegradable itraconazole (ITZ)-loaded d-a-tocopheryl polyethylene glycol 1000 succinate-b-poly(e-caprolactone-ran-glycolide) (TPGS-b-(PCL-ran-PGA); TPP) nanoparticles (NPs) (designed as ITZ-loaded TPP NPs) to improve antifungal efficacy. ITZ-loaded TPP NPs were prepared by a modified double-emulsion method, and their size distribution, morphology, zeta potential, drug encapsulation efficiency, drug-release profile, and antifungal effects were characterized. The cytotoxicity of ITZ-loaded-TPP NPs on HeLa cells and fibroblasts was measured using the 3-(4,5-dimethylthiazol-2-yl)-2,5-diphenyltetrazolium bromide (MTT) method. The in vivo antifungal activity of ITZ-loaded-TPP NPs was examined in mice by administrating 5×105 colony forming units of Candida albicans through the tail vein. The survival rate and survival time of the mice was observed. The fungal count and pathology of lung tissue was analyzed. The data showed that ITZ-loaded-TPP NPs have size of 265±5.8 nm, zeta potential of −31±0.5 mV, high encapsulation efficiency (95%), and extended drug-release profile. ITZ-loaded-TPP NPs at a high concentration of 25 mg/mL had no cytotoxicity on HeLa cells and fibroblasts. Furthermore, ITZ-loaded-TPP NPs achieved a higher level of antifungal activity both in vitro and in vivo. The survival rate and duration was higher in mice treated by ITZ-loaded-TPP NPs than in the other groups (P<0.05). In conclusion, ITZ-loaded-TPP NPs significantly improved ITZ bioavailability by increasing its aqueous dispersibility and extending the duration of drug release, thereby improving the antifungal efficacy of the ITZ agent.
Introduction
Fungal diseases tend to occur most often as opportunistic infections in patients with a weakened immune system, such as patients with human immunodeficiency virus infection, diabetes mellitus, or leukemia, or in patients who received immunosuppressive therapy or chemotherapy.Citation1 The symptoms of fungal infection vary with location within the body and the type of infection. Some fungal infections can be mild. However, some types of fungal infections, such as Candida spp., can result in serious complications or even death.Citation2 Currently, many kinds of new drugs, such as polyenes (amphotericin B), triazoles (fluconazole, itraconazole, voriconazole, posaconazole) or echinocandins (caspofungin, micafungin, anidulafungin), have been specifically developed to inhibit fungal infection.Citation3–Citation5 Among these drugs, itraconazole (ITZ) is one of the most promising for the prophylaxis of opportunistic fungal infections in patients, due to its excellent ability to inhibit a wide range of superficial and deep fungal infections.Citation6 ITZ can be used to treat fungal infections via oral or intravenous administration.Citation7 However, ITZ has low bioavailability via oral administration owing to its very low aqueous solubility of approximately 1 ng/mL at pH 7.0.Citation8,Citation9 The current commercial intravenous formulation of ITZ, formulated with a high concentration of hydroxypropyl-beta-cyclodextrin, has been found to have some side effects, including diarrhea.Citation10–Citation13 Therefore, less-toxic, solvent-free formulations of ITZ for effective antifungal therapy are urgently needed for clinical administration.
Recently, polymeric nanoparticles (NPs) have been widely investigated as drug carriers due to their ability to effectively deliver water-insoluble drugs, reduce the drug blood concentration fluctuations, and obtain persistent drug release.Citation14 In the past decade, several biodegradable aliphatic polyesters, such as poly(e-caprolactone) (PCL) and polyglycolide (PGA) have been approved by the US Food and Drug Administration for use in the field for drug delivery.Citation15,Citation16 However, the high crystallinity of PCL results in poor soft tissue compatibility, lower biodegradability, and a slower degradation rate than PGA. PGA is also not a perfect material for a NP drug delivery system because of its high melting temperature of about 220°C, high crystallinity of 45%–55%, and insolubility in common solvent.Citation16–Citation18 These drawbacks have hampered the application of PCL and PGA in NP drug delivery systems.
To solve these problems, we have previously synthesized a novel diblock copolymer, d-a-tocopheryl polyethylene glycol 1000 succinate-b-poly(e-caprolactone-ran-glycolide) [TPGS-b-(PCL-ran-PGA)] using PCL, PGA, and d-α-tocopheryl polyethylene glycol 1000 succinate (TPGS) by ring-opening polymerization reaction.Citation19 We found that TPGS-b-(PCL-ran-PGA) (TPP)-based NPs has better biocompatibility, biodegradability, dispersibility, drug encapsulation efficiency, and drug-release profile than PGA and PCL NPs alone.Citation19 In this study, we prepared the ITZ-loaded TPP NPs by a modified double-emulsion method and investigated the NP size, morphology, drug-release profile, and antifungal effects in vitro and in vivo to explore this novel antifungal agent.
Materials and methods
Materials
TPGS, glycolide (1,4-Dioxane-2,5-dione), polyvinyl alcohol (80% hydrolyzed), 3-(4,5-dimethylthiazol-2-yl)-2,5-diphenyltetrazolium bromide (MTT), ITZ, poly(lactic-co-glycolic acid) (PLGA) (50:50) and stannous octoate were purchased from Sigma-Aldrich (St Louis, MO, USA). ε-Caprolactone was from Acros Organics (Geel, Belgium). Acetonitrile and methanol were purchased from EM Science (ChromAR, HPLC grade; Mallinckrodt Baker, USA). Dulbecco’s Modified Eagle’s Medium (DMEM) and fetal bovine serum were from Invitrogen (Thermo Fisher Scientific, Waltham, MA, USA). All other agents were of analytical grade or higher quality and were commercially available. High-purity water was prepared using a Milli-Q plus System (Millipore Corporation, Breford, USA). All experiments were performed in strict accordance with the Guide for the Care and Use of Laboratory Animals as adopted by the China National Institutes of Health, and the animal experiments were approved by the Administrative Committee on Animal Research in the Graduate School at Shenzhen, Tsinghua University (No TSUSZ20130012).
Preparation of ITZ-loaded TPP NPs
TPP polymers were synthesized according to a previously described method.Citation19 Then ITZ-loaded TPP NPs were prepared by a modified double emulsion method. Briefly, 100 mg TPP polymers and 6.4 mg ITZ were fully dissolved in 10 mL dichloromethane (DCM). The formed solution was poured into 50 mL of 3% (w/v) polyvinyl alcohol solution under vigorous stirring. The mixture was sonicated for 10 minutes with pulses of 10 seconds on and 10 seconds off at 600 W output to form oil/water emulsion. DCM was then allowed to evaporate overnight under continuous stirring at 500 rpm. The suspension was then dialyzed in a Regenerated Cellulose Dialysis Membrane (Spectra/Por 6, molecular weight cutoff =1,000; Spectrum, Houston, TX, USA) to remove the emulsifier and unencapsulated ITZ. The dialysis bag was put into ultrapure water under gentle stirring for 12 hours, with water changed every 4 hours. The resulting suspension was freeze-dried at −50°C at a reduced pressure of 0.13 Pa for 48 hours. The resulting NPs were stored at −80°C for further analysis. The preparation of ITZ-loaded PLGA NPs was similar to the methods described above.Citation19 Empty TPP NPs were also prepared via the same procedure, with the exception that no ITZ was added to the DCM.
NP characterization
The surface morphology, size, zeta potential, drug encapsulation efficiency (EE) and drug release profile of nanoparticles are pivotal characterizations, which determine the functions.
Surface morphology
Samples were dropped onto double-sided sticky tape on the stub. The particles were then coated with a platinum layer using a JFC-1300 automatic fine platinum coater (JEOL, Tokyo, Japan) for 30 seconds in a vacuum. The surface morphologies of the NPs were observed by a field emission scanning electron microscope (JSM-6700F system; JEOL).
Particle size and zeta potential
Particle size and size distribution were measured by dynamic light scattering (Brookhaven Instruments Corporation, Holtsville, NY, USA). Zeta potential of the NPs was determined by using laser Doppler anemometry (Zetasizer Nano ZS90; Malvern Instruments LTD, Malvern, UK). Each measurement was performed in triplicate.
Encapsulation efficiency
Fifteen milligrams of ITZ was dissolved in 5 mL 95:5 (v/v) acetonitrile:water solution and diluted in 10-fold series (0, 0.003, 0.03, 0.3, 3 mg/mL). Then the ITZ standard curve was prepared by measuring the light absorption value at 300 nm with a spectrophotometer (UV 1601; Shimadzu, Kyoto, Japan). The amount of ITZ encapsulated in the NPs was calculated by dissolving 5 mg of the freeze-dried NPs in 5 mL of acetonitrile:water solution, and ITZ concentration was determined according to the standard curve. The ITZ encapsulation efficiency was defined as the ratio of the amount of ITZ encapsulated in the NPs to that theoretically added in the fabrication process.
In vitro release of ITZ from NPs
Thirty milligrams of ITZ-loaded NPs was suspended in 10 mL of phosphate-buffered saline (PBS) release buffer (containing 0.05% w/v Tween 80, pH 7.4).Citation19 The dispersion was then aliquoted into ten tubes and shaken at 37°C and 150 rpm. At appropriate intervals, the supernatant was collected by centrifuging the tube at 15,000 rpm for 30 minutes. The ITZ concentration in the supernatant was determined as described in the encapsulation efficiency (EE) method.
Cytotoxicity assay
The cytotoxicity of ITZ-loaded TPP NPs was evaluated using the MTT assay. Briefly, HeLa cells or fibroblasts were seeded at a density of 5×103 cells/well in 100 μL culture medium into a 96-well plate and incubated overnight. The cells were incubated with different concentrations of NPs for 48 hours and 72 hours. At indicated time points, the culture medium was replaced with 100 μL MTT (5 mg/mL, in DMEM medium) and the plates were incubated at 37°C for another 4 hours; thereafter, MTT was removed and DMSO was added to completely dissolve the formazan crystals. Absorbance at 570 nm was measured with a microplate reader (Varioskan Flash; Thermo Fisher Scientific).
In vitro antifungal effects
The in vitro antifungal activity was tested using the paper-plate technique. Briefly, 10 μL of conidial suspension at 1×105 spores/mL of Candida albicans (ATCC 10231) was spread over the glucose agar plate; 5 mm diameter filter paper was made with a sterile borer and was sterilized. Three pieces of sterile filter paper were placed on the solidified agar layer. Four different treatments (10 μL) were dropped onto the papers: PBS, ITZ-loaded PLGA NP (equivalent to 0.3 mg/mL ITZ), ITZ-free TPP NP suspensions at 10 mg/mL, and ITZ-loaded TPP NP suspensions at 10 mg/mL (equivalent to 0.3 mg/mL of ITZ), respectively. The plates were then incubated at 28°C for 7 days. The plates were photographed and the inhibitory zones were measured every day.
In vivo antifungal effects
Survival test of mice after treatment
BALB/c mice were randomly divided into five groups with ten animals each group: group 1 (noninfected group with no treatment); group 2 (infected group with PBS treatment); group 3 (infected group with ITZ-loaded PLGA NPs treatment); group 4 (infected group with ITZ-free TPP NPs treatment); group 5 (infected group with ITZ-loaded TPP NPs treatment). Mice were challenged intravenously via the tail vein with 50 μL of 1×107 colony forming units (CFU)/mL C. albicans, and then the mice were given corresponding administration with 100 μL relevant solution (100 μL ITZ-loaded PLGA NP or ITZ-loaded TPP NP suspension is equivalent to 0.03 mg ITZ) via tail vein 12 hours after challenge of C. albicans. On day 3 (48 hours after first administration), the mice were given corresponding administration once again, and the behaviors and lifetime of mice were observed every day. In this animal experiment, we begin to calculate the survival of mice after the first administration of drug. All surviving mice were euthanized on day 16.
Fungi count test and pathological examination
On day 3, three mice of each group were euthanized by cervical dislocation 48 hours after the first administration. We aseptically removed their lungs for our experiments because the size of NPs is about 260 nm, which is easily retained in lungs, and their lungs were severely damaged. The right lung was rinsed and homogenized in sterile PBS with a tissue grinder. Then, the suspension was diluted to 100 multiples and was cultured on a glucose agar plate. The CFUs were counted to determine the CFU/g of tissue (ie, colony forming units per gram of lung tissue). These colonies were further analyzed by yeast morphology assay and 18S rDNA polymerase chain reaction analysis. In addition, the left lung tissue was embedded with paraffin and sectioned at 5 μm. Hematoxylin/eosin staining was performed to observe the pathological change.
Statistical analysis
Raw data were analyzed using the SPSS statistical software (version 16.0, IBM Corporation, Armonk, NY, USA). All data are expressed as means ± standard deviation of three independent experiments. Differences between paired groups were analyzed by analysis of variance. P<0.05 was considered statistically significant.
Results
Size, zeta potential, and drug encapsulation efficiency
Size distribution, zeta potential, drug-loading content and encapsulation efficiency are summarized in . As shown in , TPP NPs have a diameter of about 265 nm. Although there is a little difference between the NP size as detected by scanning electron microscopy and dynamic light scattering, the difference was not significant. Zeta potential is a marker of stability of NPs in suspension. The detection by laser Doppler anemometry showed that the zeta potential of TPP NPs was −31 mV, which contributed to the dispersivity and stability of the NPs. The amount of ITZ encapsulated in the TPP and PLGA NPs was 10.04% and 9.7%, respectively, and the drug encapsulation efficiency of both NPs was up to 95%.
Table 1 Characterization of NPs
Surface morphology
shows the field emission scanning electron microscopy image of the ITZ-loaded TPP NPs. The NPs seemed to be around 260 nm in diameter with a smooth surface within the resolution level. This size is consistent with the result described above.
In vitro drug release
The drug-release profile of the ITZ-loaded NPs in the first 12 days is shown in . The kinetics curve of ITZ-loaded NPs displays a biphase feature. The ITZ release from the TPP NPs was about 23.75% and 56% of the encapsulated drug in the first 3 days and after 12 days, respectively, suggesting that TPP NPs can serve as a drug reservoir to realize the long-acting antifungal effects of ITZ, similar to ITZ-loaded PLGA NPs. However, the ITZ release from TPP NPs was more rapid than that from PLGA NPs, although the difference was not significant (P>0.05).
Figure 2 In vitro release profiles of ITZ from loaded TPP NPs and PLGA NPs.
Notes: There was no significant difference (P>0.05) between ITZ-loaded TPP NPs and ITZ-loaded PLGA NPs. However, the release efficacy of ITZ-loaded TPP NPs was higher than that of ITZ-loaded PLGA NPs at all indicated times. The release curve has biphase kinetics: at the initial phase, the release is burst-like; then the release is relatively stable. The release of ITZ-loaded TPP NPs and ITZ-loaded PLGA NPs was up to 50% at day 8 and day 10, respectively. The experiment was performed in PBS at 37°C.
Abbreviations: ITZ, itraconazole; NP, nanoparticle; PBS, phosphate-buffered saline; PLGA, poly(lactic-co-glycolic acid); TPP, TPGS-b-(PCL-ran-PGA).
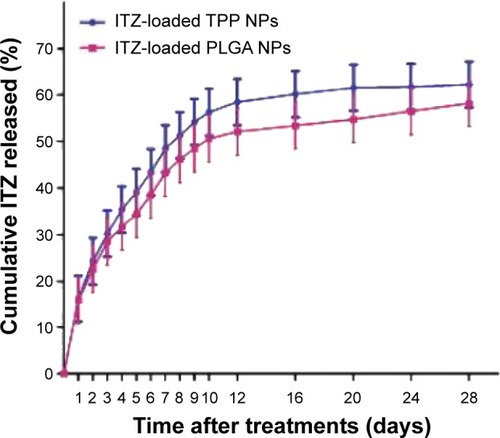
Cytotoxicity of ITZ-loaded TPP NPs
To determine whether ITZ-loaded TPP NPs are cytotoxic for cells, the in vitro cell viability of ITZ-loaded TPP NPs was evaluated by the MTT assay using HeLa cells and fibroblasts. As shown in , the ITZ-loaded TPP NPs had no obvious cytotoxic activity at 0.25 mg/mL and 25 mg/mL concentrations after 24 hours and 48 hours incubation, suggesting that the ITZ-loaded TPP NPs are safe for use.
Figure 3 Viability of HeLa cells and fibroblasts treated with ITZ-loaded TPP NPs.
Notes: The results showed that ITZ-loaded TPP NPs had no cytotoxic activity on HeLa cells and fibroblasts at dosages of 0.25 mg/mL and 25 mg/mL at 48 hours and 72 hours.
Abbreviations: ITZ, itraconazole; NP, nanoparticle; PBS, phosphate-buffered saline; TPP, TPGS-b-(PCL-ran-PGA).

The in vitro antifungal activity
The antifungal activity of four different treatments (PBS control, ITZ-loaded PLGA NPs, ITZ-free TPP NPs, and ITZ-loaded TPP NPs) were tested by paper-plate technique, and the diameters of inhibition zones were measured. ITZ-free TPP NPs had no visible inhibitory activity against fungal growth () compared with the PBS control (). ITZ-loaded PLGA showed moderate antifungal effect, with inhibition-zone diameters of 9.43±0.25 mm on day 3, 10.61±0.15 mm on day 5, and 11.85±0.25 mm on day 7, indicating that the diameters of the inhibition zone of agent application did increase as time progressed (). In particular, ITZ-loaded TPP NPs showed superior antifungal efficacy compared to ITZ-loaded PLGA NPs, with inhibition zone diameters of 11.43±0.25 mm on day 3, 13.67±0.15 mm on day 5, and 15.32±0.21 mm on day 7 (), which present clear inhibition zones beyond the areas of application ().
Table 2 Diameters of the inhibition zones of drug application on day 7
Figure 4 Antifungal activity of four different treatments (PBS control, ITZ-loaded PLGA NPs, ITZ-free TPP NPs, and ITZ-loaded TPP NPs).
Notes: Paper-plate growth inhibition of Candida albicans treated with (A) ITZ-free TPP NPs; (B) PBS; (C) ITZ-loaded PLGA NPs; and (D) ITZ-loaded TPP NPs. The results showed that ITZ-free TPP NPs and PBS had no inhibitory effect on C. albicans, whereas ITZ-loaded TPP NPs and ITZ-loaded PLGA NPs could inhibit the growth of C. albicans. In addition, the inhibitory zone of ITZ-loaded TPP NPs and ITZ-loaded PLGA NPs showed that the inhibitory effect of ITZ-loaded TPP NPs on C. albicans was superior than that of ITZ-loaded PLGA NPs, which probably results from the drug release difference of ITZ-loaded TPP NPs and ITZ-loaded PLGA NPs.
Abbreviations: ITZ, itraconazole; NP, nanoparticle; PBS, phosphate-buffered saline; PLGA, poly(lactic-co-glycolic acid); TPP, TPGS-b-(PCL-ran-PGA).

The in vivo antifungal activity
To evaluate the antifungal activity of ITZ-loaded TPP NPs in vivo, mice were challenged intravenously via the tail vein with 50 μL of 1×107 CFU/mL C. albicans and then administered PBS, ITZ-loaded PLGA NPs, ITZ-free-TPP NPs, and ITZ-loaded TPP NPs. On day 3, 48 hours after the first administration, the lung tissue of three mice in each group was homogenized for the fungi count test and the CFU/g of tissue was calculated. As shown in , the group with ITZ-loaded PLGA NPs treatment had a significantly lower value of 1.6×103 CFU/g of tissue compared with those of the PBS control group and ITZ-free TPP NPs group (P<0.01). In particular, treatment of ITZ-loaded TPP NPs showed the best inhibitory efficacy against C. albicans growth in lung of mice, with a value of 1.2×102 CFU/g, much less than 2.4×106 CFU/g in mice treated by ITZ-free TPP NPs. C. albicans could be detected in lung of mice treated by ITZ-loaded PLGA NPs, but it was rarely detected in mice treated with ITZ-loaded TPP NPs. The mice treated with ITZ-free NPs tended to gather and be torpid, and to have slower responses and lower activity levels. Their lung tissues displayed blood dots, and their pulmonary alveoli contained red cells and a large amount of inflammatory cells (). Meanwhile, mice in the ITZ-loaded PLGA NPs group only exhibited a pathological behavior. The lung epidermis cells were impaired, pulmonary alveoli contained red cells, and less inflammatory-cell infiltration compared with the PBS and ITZ-free NPs groups (). It is encouraging that mice treated with ITZ-loaded TPP NPs displayed normal behavior and that their lung tissues had normal pathological features (). The survival rate and duration of mice has been observed and the data indicated that ITZ-loaded TPP NPs had the best protective effects against fungal infection in mice ().
Table 3 The CFU/g lung tissue of mice treated by different agents (n=3)
Figure 5 The pathological change in lung tissue for mice treated with different administrations.
Notes: (A) Normal mouse, untreated. (B) Infected group with PBS treatment. (C) Infected group with ITZ-loaded PLGA treatment. (D) Infected group with ITZ-free TPP NPs treatment. (E) Infected group with ITZ-loaded TPP NPs treatment.
Abbreviations: ITZ, itraconazole; NP, nanoparticle; PBS, phosphate-buffered saline; PLGA, poly(lactic-co-glycolic acid); TPP, TPGS-b-(PCL-ran-PGA).
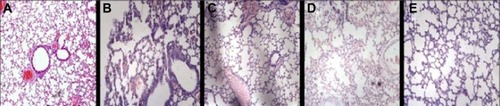
Figure 6 The survival test curves for mice under different treatments (n=10/group).
Notes: All PBS-treated mice and mice treated with ITZ-free TPP NPs died within 6 days. In contrast, 80% of the mice survived after administration of ITZ-loaded TPP NPs and 30% survived after treatment with ITZ-loaded PLGA NPs; the survival time was more than 15 days. In this animal experiment, all surviving mice were euthanized on day 16.
Abbreviations: ITZ, itraconazole; NP, nanoparticle; PBS, phosphate-buffered saline; PLGA, poly(lactic-co-glycolic acid); TPP, TPGS-b-(PCL-ran-PGA).
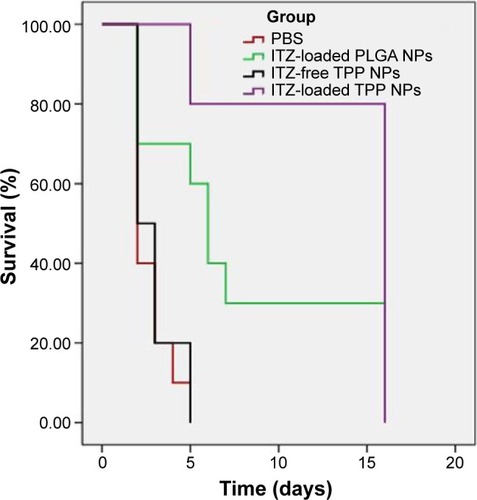
Discussion
Polymer-based NPs have been widely investigated as a drug-delivery system, especially for hydrophobic drugs.Citation20 However, the biodegradable polymers NPs that have been approved by the US Food and Drug Adminstration, such as PCL and PLGA, have poor compatibility and a too-slow degradation rate in vivo owing to their compaction structure.Citation16–Citation18 Several newly synthesized polymers have been developed to improve NP drug-delivery systems. In our previous study, a novel polymer, TPP, was synthesized and confirmed to have better advantage in biocompatibility, biodegradability, dispersibility, docetaxel-encapsulation efficiency, and docetaxel-release profile.Citation19
In this work, the ITZ-loaded NPs were prepared using the TPP material for antifungal infection. Our results showed that ITZ-to-polymer ratios at 5:1 can obtain low particle size of about 265 nm with high encapsulation efficiency of 95%. Previous research reported that TPGS addition in the manufacture of NPs can promote the EE of water-insoluble drugs and decrease the size of NPs; thus, the low particle size and high EE of ITZ-loaded TPP NPs may be caused by the self-emulsifying function of TPGS.Citation21 In addition, TPGS has been widely used as an emulsifier in the manufacture of NPs, offering additional steric stabilization effect while avoiding aggregation of NPs in the colloidal system, which can promote the stability of the particles.Citation22 Zeta potential is an important physicochemical parameter that influences stability of the NPs. Similar positive or negative zeta potential values can prevent aggregation of the nanoparticles and ensure good redispersion.Citation23 The zeta potential of ITZ-loaded TPP NPs is −31 mV, indicating that it is desirable result.
The antifungal activity of the developed NPs formulation was further examined in vitro by the paper-plate test. ITZ-loaded TPP NPs demonstrated an enhanced inhibitory effect compared with ITZ-loaded-PLGA NPs ( and ). The enhanced antifungal activity may be attributed to the following reasons. First, TPP NPs were expected to improve the dispersing of ITZ in water. As previously mentioned, ITZ is a water-insoluble drug, which results in its low bioavailability. Since TPP NPs are hydrophilic and small in size, this facilitates their contact with the fungus on the plate surface and leads to faster drug internalization. Secondly, TPP NPs constitute a slow-release drug delivery system and can prevent drugs from degradation, similar to PLGA-based vectors (). While ITZ is consumed or degraded, the fungus near the point of drug application continues to grow. The sustained release of ITZ from TPP NPs can provide a constant inhibition on fungal growth, resulting in larger inhibition zones as observed over the time of the study. This will help reduce frequencies of drug administration, therefore reducing the side effects.
The in vivo study also demonstrated that ITZ-loaded TPP NPs have a better therapeutic effect for fungal infection than ITZ-loaded PLGA NPs ( and ). The mice treated with ITZ-loaded TPP NPs had better behaviors and lower death rates than the mice in the ITZ-loaded PLGA NPs group. In this work, we did not compare the antifungal efficacy of ITZ-loaded TPP NPs to ITZ alone. Instead, we compared the antifungal efficacy of ITZ-loaded TPP NPs to ITZ-loaded PLGA NPs. It has been reported by Patel et alCitation24 that the antifungal efficacy of ITZ-loaded PLGA NPs is superior to ITZ alone. Based on these facts, we think that the application of ITZ-loaded TPP NPs is better than the traditional ITZ treatment. Lung is the most common target organ of fungal infection in some cases, such as AIDS patients.Citation25,Citation26 Because previous studies have reported that NPs with a size of 100–1,000 nm are able to be retained in the lung,Citation27,Citation28 it is highly likely that ITZ-loaded TPP NPs could accumulate in the lung tissue. In addition, the release of TPGS from NPs may play some role. Detailed mechanism needs further investigation.
Conclusion
We developed the ITZ-loaded TPP NPs with appropriate particle size, high EE, and an extended drug-release profile. The ITZ-loaded TPP NPs have been proven to be an effective means for enhancing the antifungal effects of ITZ. ITZ-loaded TPP NPs provide a better choice for patients with fungal diseases when traditional antifungal agents are not available or do not work.
Acknowledgments
This research was supported by the National Natural Science Foundation of China (No 81072123) and the Clinical Medical Research Project of Wuhan Health and Family Planning Commission (WX13A01). The animal experiments were approved by the Administrative Committee on Animal Research in Graduate School at Shenzhen, Tsinghua University (No TSUSZ20130012).
Disclosure
The authors report no conflicts of interest in this work.
References
- PaganoLAkovaMDimopoulosGHerbrechtRDrgonaLBlijlevensNRisk assessment and prognostic factors for mould-related diseases in immunocompromised patientsJ Antimicrob Chemother201166Suppl 1i5i1421177404
- EnochDALudlamHABrownNMInvasive fungal infections: a review of epidemiology and management optionsJ Med Microbiol200655Pt 780981816772406
- JungSHLimDHJungSHAmphotericin B-entrapping lipid nanoparticles and their in vitro and in vivo characteristicsEur J Pharm Sci2009373–431332019491021
- OddsFCBrownAJGowNAAntifungal agents: mechanisms of actionTrends Microbiol200311627227912823944
- SabatelliFPatelRMannPAIn vitro activities of posaconazole, fluconazole, itraconazole, voriconazole, and amphotericin B against a large collection of clinically important molds and yeastsAntimicrob Agents Chemother20065062009201516723559
- PandaNKItraconazole – a potent antifungal drugIndian J Otolaryngol Head Neck Surg199749329329423119315
- WillemsLvan der GeestRde BeuleKItraconazole oral solution and intravenous formulations: a review of pharmacokinetics and pharmacodynamicsJ Clin Pharm Ther200126315916911422598
- PeetersJNeeskensPTollenaereJPVan RemoorterePBrewsterMECharacterization of the interaction of 2-hydroxypropyl-b-cyclodextrin with itraconazole at pH 2, 4, and 7J Pharm Sci20029161414142212115841
- De BeuleKItraconazole: pharmacology, clinical experience and future developmentInt J Antimicrob Agents19966317518118611706
- AkkarAMüllerRHIntravenous itraconazole emulsions produced by SolEmuls technologyEur J Pharm Biopharm2003561293612837478
- ChenWGuBWangHPanJLuWHouHDevelopment and evaluation of novel itraconazole-loaded intravenous nanoparticlesInt J Pharm20083621–213314018585448
- YiYYoonHJKimBOA mixed polymeric micellar formulation of itraconazole: characteristics, toxicity and pharmacokineticsJ Control Release20071171596717097755
- GouldSScottRC2-Hydroxypropyl-beta-cyclodextrin (HP-beta-CD): a toxicology reviewFood Chem Toxicol200543101451145916018907
- PanyamJLabhasetwarVBiodegradable nanoparticles for drug and gene delivery to cells and tissueAdv Drug Deliv Rev200355332934712628320
- MundargiRCSrirangarajanSAgnihotriSADevelopment and evaluation of novel biodegradable microspheres based on poly(d,l-lactide-co-glycolide) and poly(epsilon-caprolactone) for controlled delivery of doxycycline in the treatment of human periodontal pocket: in vitro and in vivo studiesJ Control Release20071191596817331611
- VertMDegradable and bioresorbable polymers in surgery and in pharmacology: beliefs and factsJ Mater Sci Mater Med200920243744618815731
- AndrianoKPDanielsAUSmutzWPWyattRWPreliminary biocompatibility screening of several biodegradable phosphate fiber reinforced polymersJ Appl Biomater19934111210148351
- SunHMeiLSongCCuiXWangPThe in vivo degradation, absorption and excretion of PCL-based implantBiomaterials20062791735174016198413
- HuangLChenHZhengYNanoformulation of d-α-tocopheryl polyethylene glycol 1000 succinate-b-poly(ε-caprolactone-ran-glycolide) diblock copolymer for breast cancer therapyIntegr Biol (Camb)2011310993100221938302
- MatsumuraYKataokaKPreclinical and clinical studies of anticancer agent-incorporating polymer micellesCancer Sci2009100457257919462526
- RuanGFengSSLiQTEffects of material hydrophobicity on physical properties of polymeric microspheres formed by double emulsion processJ Control Release200284315116012468218
- MuLFengSSVitamin E TPGS used as emulsifier in the solvent evaporation/extraction technique for fabrication of polymeric nanospheres for controlled release of paclitaxel (Taxol)J Control Release2002801–312914411943393
- MainardesRMEvangelistaRCPLGA nanoparticles containing praziquantel: effect of formulation variables on size distributionInt J Pharm20052901–213714415664139
- PatelNRDamannKLeonardiCSabliovCMItraconazole-loaded poly(lactic-co-glycolic) acid nanoparticles for improved antifungal activityNanomedicine (Lond)2010571037105020874019
- PachecoPVenturaASBrancoTGonçalvesLCarvalhoCClinical experience in invasive fungal infectionsClin Drug Investig201333Suppl 1S23S26
- GazzoniFFSeveroLCMarchioriEFungal diseases mimicking primary lung cancer: radiologic-pathologic correlationMycoses201457419720824147761
- BorakBBiernatPPreschaABaszczukAPlutaJIn vivo study on the biodistribution of silica particles in the bodies of ratsAdv Clin Exp Med2012211131823214294
- WanakulePLiuGWFleuryATRoyKNano-inside-micro: disease-responsive microgels with encapsulated nanoparticles for intracellular drug delivery to the deep lungJ Control Release2012162242943722841795