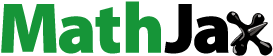
Abstract
A sensitive electrochemical immunosensor was developed for detection of alpha-fetoprotein (AFP) based on a three-dimensional nanostructure gold electrode using a facile, rapid, “green” square-wave oxidation-reduction cycle technique. The resulting three-dimensional gold nanocomposites were characterized by scanning electron microscopy and cyclic voltammetry. A “sandwich-type” detection strategy using an electrochemical immunosensor was employed. Under optimal conditions, a good linear relationship between the current response signal and the AFP concentrations was observed in the range of 10–50 ng/mL with a detection limit of 3 pg/mL. This new immunosensor showed a fast amperometric response and high sensitivity and selectivity. It was successfully used to determine AFP in a human serum sample with a relative standard deviation of <5% (n=5). The proposed immunosensor represents a significant step toward practical application in clinical diagnosis and monitoring of prognosis.
Introduction
Immunoassays based on specific recognition between an antigen and an antibody have been used successfully in a number of fields, including medical detection, processing quality control, and environmental monitoring.Citation1–Citation3 Traditional immunoassay methods have included the radioimmunoassay, enzyme-linked immunosorbent assay, and electrochemiluminescence immunoassay. In spite of their good sensitivity, the radioimmunoassay significantly increases the radioactivity hazard for laboratory workers and the enzyme-linked immunosorbent assay is tedious and time-consuming. Newer methods, including surface plasmon resonance,Citation4 quartz crystal microbalance,Citation5 and opticalCitation6 and electrochemical methods,Citation7 have attracted considerable interest for immunoassays due to their rapid recognition and simple fabrication. Among these methods, the electrochemical immunoassay has received much attention in view of its high sensitivity and low cost.Citation7,Citation8
The crucial step in construction of an electrochemical immunosensor is efficient immobilization of biomolecules onto the electrode surface.Citation9 New opportunities for fabrication of highly sensitive electrochemical immunosensors have been opened up by the advent of nanomaterials and nanotechnologies.Citation10 Advances in biomolecular detection have included use of nanomaterials, such as nanotubes, nanoparticles, and nanowires, as a medium of signal amplification.Citation11–Citation13 In recent years, due to its interesting properties, including a large surface area, superior conductivity, biocompatibility, and high stability, nanoporous gold has attracted considerable attention. Several methods, including electrochemical deposition,Citation14 electrochemical erosion,Citation15 “direct freezing”,Citation16 a voltage-induced dimension change method,Citation17 a “dealloying process”,Citation18–Citation20 template synthesis,Citation21,Citation22 and repetitive square-wave oxidation-reduction cycle (SWORC)Citation23 were been used to prepare a three-dimensional gold (3D-Au) nanostructure. Due to the time-saving, easy, and controllable preparation of nanoporous gold, SWORC is very attractive among these methods.Citation24,Citation25
Alpha-fetoprotein (AFP) is normally synthesized by the liver, yolk sac, and gastrointestinal tract in the human fetus. Plasma levels of AFP decrease rapidly after birth and reach normal adult levels by the age of 8–12 months. Elevated serum AFP levels in adults are significant in the diagnosis of ataxia-telangiectasia syndrome, hereditary tyrosinemia, cirrhosis, alcoholic hepatitis, hepatocellular carcinoma, and viral hepatitis, and are also useful for monitoring the effectiveness of surgical and chemotherapeutic management of hepatomas and germ cell neoplasms.Citation8 AFP testing is currently performed using the radioimmunoassay and enzyme-linked immunosorbent assay.Citation26,Citation27 However, there are some limitations, such as radiation hazards and a long analysis time. Therefore, it is important to explore new routes for detection of AFP.
In the present study, a facile electrochemical immunosensor based on a 3D-Au electrode was developed for detection of AFP. The 3D-Au electrode was prepared by a simple, rapid, and “green” SWORC approach. The 3D-Au electrode was then used as a substrate for construction of a “sandwich-type” AFP immunosensor. This new immunosensor showed a fast amperometric response, high sensitivity, and selectivity, and was used to assay of AFP in a real serum sample with a satisfactory result. The proposed immunosensor represents a significant step towards practical application in clinical diagnosis and prognostication.
Materials and methods
Chemicals and materials
3-mercaptopropionic acid, nitric acid, sulfuric acid, potassium permanganate, ethanol, N-hydroxysuccinimide, and 1-ethyl-3-(3-dimethylamino propyl) carbodiimide hydrochloride (EDC) were provided by Sinopharm Chemical Reagent Co Ltd (Shanghai, People’s Republic of China). Bovine serum albumin was sourced from Sigma-Aldrich (St Louis, MO, USA). 3,3′,5,5′-tetramethylbenzidine (TMB) substrate (K-blue low activity substrate) was purchased from Neogen (Lansing, MI, USA). Human serum samples were obtained from the First Affiliated Hospital of Fujian Medical University. All chemicals were of analytical reagent grade and were used without further purification. All solutions were prepared with Milli Q water (18 MΩ·cm resistivity) from a Millipore system (Billerica, MA, USA).
Mouse anti-AFP monoclonal antibody (8.556 mg/mL) and horseradish peroxidase (HRP)-conjugated mouse anti-AFP monoclonal antibody (13.34 mg/mL), AFP standard solution, and cancer antigen 125, 153, and 199 standard solutions were from Zhengzhou Biocell Biotechnology Co Ltd (Zhengzhou, People’s Republic of China). The antibody dilution was 10 mM phosphate-buffered saline (PBS) containing 0.08 M NaCl (pH 7.4). The antibodies were aliquoted and stored at −20°C. The purified antibodies were diluted upon use in PBS suitable for the immobilizing and capture reactions.
Apparatus
All electrochemical measurements were performed on a CHI 760D Electrochemical Workstation (CH Instruments, Austin, TX, USA). Electrochemical experiments were carried out with a conventional three-electrode system comprising a 3D-Au working electrode, an Ag/AgCl (with saturated KCl) reference electrode, and a platinum wire auxiliary electrode. Cyclic voltammetry was carried out at a scan rate of 100 mV per second. Amperometric detection was performed at a fixed potential of 100 mV. Steady-state currents were recorded within 100 seconds. Scanning electron microscopic images were obtained using an S-4800 instrument (Hitachi, Tokyo, Japan). Atomic force microscopic images were acquired using an SPM-Multimode 8 system (Bruker, Santa Barbara, CA, USA) with Nanoscope Analysis version 1.40.0.0 software. All the images presented in this paper were acquired using ScanAsyst mode under ambient conditions.
Preparation of 3D-Au electrode
The method used to prepare the 3D-Au electrode was the same as that described in our previous reports.Citation24,Citation25 Briefly, before each experiment, a gold electrode (2 mm in diameter) was polished with 1.0, 0.3, and 0.05 μm alumina powder. The gold electrode was then cleaned ultrasonically sequentially and thoroughly in ethanol and deionized water. The nanoporous gold electrode was electrochemically cleaned in fresh 0.5 M H2SO4 solution by potential scanning between −0.35 V and 1.5 V until a cyclic reproducible voltammogram was obtained to remove any possible impurities on the surfaceCitation28 and the electrode was rinsed with doubly distilled water, then the electroabsorption charge of the O adatoms between the rough gold electrode and the flat gold electrode was checked.
Fabrication of immunosensor
The cleaned 3D-Au electrode was first immersed in an aqueous solution of 5 mM 3-mercaptopropionic acid for 24 hours at room temperature. After thorough rinsing with deionized water to remove the physically adsorbed 3-mercaptopropionic acid, the nanoporous gold electrode was immersed in 10 mM PBS (pH 7.4) with 20 mg/mL of EDC and 10 mg/mL of N-hydroxysuccinimide for 40 minutes. The activated 3D-Au electrode was thoroughly rinsed with 10 mM PBS (pH 7.4). Next, 5 μL of capture antibody (mouse monoclonal antibody directed against AFP, 0.5 mg/mL solution) was dropped onto the electrode surface and incubated overnight to yield sensing interfaces. A blocking treatment aimed at preventing nonspecific responses during incubation of the sample was carried out by casting 10 μL of 5% (v/w) bovine serum albumin at room temperature for 1 hour, followed by careful washing in 10 mM PBS (pH 7.4). Thereafter, 5 μL of AFP antigen solution at different concentrations were added onto the electrode surface and incubated for 1 hour, and the modified electrode was then rinsed extensively to remove unbound antigen molecules. Next, 5 μL of detection antibody (HRP-conjugated mouse monoclonal antibody directed against AFP, 0.02 mg/mL) was dropped onto the modified electrode surface and incubated for 1 hour, after which the electrode was washed and ready for measurement. A schematic illustration of the stepwise preparation of the immunosensor is shown in .
Figure 1 Schematic representation of the fabrication procedure for an immunosensor based on a 3D-Au electrode.
Abbreviations: 3D, three-dimensional; Au, gold; BSA, bovine serum albumin; EDC, 1-ethyl-3-(3-dimethylamino propyl) carbodiimide hydrochloride; MPA, 3-mercaptopropionic acid; NHS, N-hydroxysuccinimide; SWORC, square-wave oxidation-reduction cycle; HRP, horseradish peroxidase.
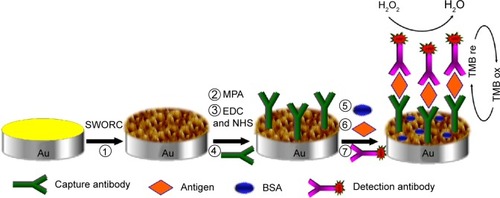
Electrochemical measurement
Electrochemical measurements, including cyclic voltammetry and amperometric i-t curve studies, were performed using a commercial TMB substrate solution containing a certain percentage of H2O2. Cyclic voltammetry was carried out at a scan rate of 100 mV per second. Amperometric detection was performed at 100 mV. Steady-state current was usually reached within 100 seconds. The data for the condition, optimization, and calibration curves was the average of three measurements.
Results and discussion
Preparation of 3D-Au electrode
shows scanning electron microscopic images of the same gold electrode before () and after () pretreatment with SWORC at a frequency of 8,000 Hz for 5 minutes. As shown in , the 3D-Au electrode surface was divided into two layers. The bottom layer was a brain-like nanoporous structure revealing the presence of small grains and channels about 120 nm in width, and the upper layer was a cotton wool-like nanostructure. The brain-like structure shown in the scanning electron microscopic images had been reported previously as being characteristic of the gold overlayers resulting from the electroreduction process.Citation29 The atomic force microscopic images () also revealed a difference between the surface structure of flat gold and that of 3D-Au. The surface of 3D-Au showed a rounded cap columnar-type structure composed of many grains. It should be noted that some large grains (120–150 nm) were formed by small subunits (40–60 nm). Some channels surrounded by a grain-like structure can also be observed in , and the width of channels was about 120 nm.
Figure 2 (A) Scanning electron microscopic images of the flat gold electrode and (B) after SWORC pretreatment. Insert is enlarged view of the image in circle. (C) ScanAsyst-mode atomic force microscopic image of flat gold electrode and (D) a 3D-Au electrode. (E) Cyclic voltammograms of a nanoporous gold electrode (curve b) and a flat gold electrode (curve a) with the same geometric area in a solution of 0.5 M H2SO4 at a scan rate of 100 mV per second.
Abbreviations: 3D, three-dimensional; Au, gold; SWORC, square-wave oxidation-reduction cycle.

The 3D-Au electrode was electrochemically characterized in 0.5 M H2SO4 at a scan rate of 100 mV per seconds (, curve b). For comparison with the nanoporous gold electrode, the voltammogram for a bare flat gold electrode with the same geometric surface area is also presented (, curve a). The real surface area of the 3D-Au electrode was approximately 31 times that of the flat gold electrode, assuming that the reduction of the monolayer of gold oxide requires 386 μC/cm2, and the roughness factor (ratio of the real surface area to the geometric surface area) is about 114.Citation21
Electrochemical responses of immunosensor based on 3D-Au electrode
shows the i-t curve for the capture antibody or capture antibody/antigen/detection antibody sandwich-modified biosensor at different electrodes in TMB solution. As shown in , curve b was the i-t curve for the capture antibody-modified 3D-Au electrode after the antigen and detection antibody were immobilized on the surface of the 3D-Au electrode by immunoreaction. The current value (curve d) increased significantly, and the difference in current value between curve d and curve b was 3.07 μA. This indicates that the sandwich structure was formed upon immunoreaction, and the HRP-conjugated detection antibody was bound onto the 3D-Au electrode surface to produce the current signal. Notably, because of the site of HRP redox, which was shielded within insulating peptide backbones, the site of HRP redox did not directly exchange electrons with the electrode.Citation30,Citation31 Therefore, small redox molecule TMB can easily diffuse in and out of the redox site of HRP as an electron shuttle, and coupling with the redox reaction of TMB, the catalytic reduction of H2O2 occurs on the electrode.Citation30,Citation31 Thus, the HRP modified on the surface of 3D-Au electrode could efficiently catalyze reduction reactions of H2O2 with the redox reaction of TMB, leading to significant amplification of current.Citation31,Citation32 However, due to the absence of the detection antibody, this catalytic reduction current on the capture antibody-modified 3D-Au electrode was not obvious. The phenomenon at the flat gold electrode surface (, curves a and c) was also similar, but the current signals decreased significantly and the difference in current value between the two curves was only 0.63 μA. Compared with the flat gold electrode, the current difference of the 3D-Au electrode was about 4.9 times higher, demonstrating that the 3D-Au-based biosensor could significantly improve the electrocatalytic activity.
Figure 3 The i-t curves corresponding to capture antibody-modified flat gold electrode (A), capture antibody-modified 3D-Au electrode (B), capture antibody/antigen/detection antibody sandwich-modified flat gold electrode (C), and capture antibody/antigen/detection antibody sandwich-modified 3D-Au electrode (D).
Abbreviations: 3D, three-dimensional; Au, gold.

Optimization of experimental conditions
The concentration of solutions used for incubation of the capture antibody and detection antibody dramatically influenced the performance of the immunosensors. On the basis of our preliminary experiments, we set three concentrations of the solutions used for incubation of both antibodies by evaluating its effect on the blank signal. The concentration levels explored were 0.1, 0.5, and 1.0 mg/mL for the capture antibody and 0.01, 0.05, and 0.1 mg/mL for the detection antibody. As shown in , when the concentration of the detection antibody was fixed at 0.02 mg/mL and 0.05 mg/mL, respectively, the blank signal decreased significantly with increasing concentration of the solution used for incubation of the capture antibody, whereas an opposite trend was observed for the detection antibody when concentrations of the capture antibody were fixed at 0.5 mg/mL and 1.0 mg/mL, respectively. This phenomenon could be explained by the fact that a high concentration of the capture antibody solution allowed complete and regular functionalization of the nanoporous gold substrate, thus preventing nonspecific binding of detection antibody. Conversely, an excessive concentration of the solution used for incubation of the detection antibody could promote its nonspecific binding. Further experiments carried out by incubating the sensors with blank solution and with 10 ng/mL of AFP solution indicated that the signal arising from the AFP solution did not significantly decrease, even when the concentration of the detection solution was reduced to 0.02 mg/mL. On the basis of these results, we fixed the concentrations of the incubating solution at 0.5 and 0.02 mg/mL for the capture and detection antibodies, respectively.
The effects of incubation temperature and incubation time on the current value for antibody and antigen reaction were also studied, as shown in , respectively. The results indicate that the optimal reaction temperature was 37°C and the optimal reaction time was 60 minutes. As a result, an incubation temperature of 37°C, a reaction time of 60 minutes, and incubating solution concentrations of 0.5 mg/mL for the capture antibody and 0.02 mg/mL for the detection antibody were selected for immunoassay of the AFP antigen.
Specificity of immunosensor
Specificity is an important parameter that influences the performance of an immunosensor in practical application. To evaluate the specificity of the presented immunosensor toward the target AFP analyte, the system was challenged with other biomarkers, including cancer antigens 153, 199, and 125. showed the histograms corresponding to the current intensity arising from immunoreaction of the AFP antibody with different proteins. As indicated in , the amperometric current intensity for the target AFP increased markedly after immunoreaction with AFP antigen as compared with the other antigens and the blank solution. Meanwhile, the presence of sample matrix components did not obviously change the current intensity of the developed immunosensor toward the target AFP. The results clearly indicate the high specificity of the developed immunosensor.
Figure 4 Amperometric response of the immunosensor to different antigens with the same concentration of 20 ng/mL.
Notes: (A) 20 ng/mL AFP antigen, (B) mixed sample containing 20 ng/mL AFP antigen, 20 IU/mL CA153 antigen, CA199 antigen, and CA125 antigen, (C) 20 IU/mL CA153 antigen, (D) 20 IU/mL CA199 antigen, (E) 20 IU/mL CA125 antigen, and (F) blank solution (not containing AFP antigen).
Abbreviation: AFP, alpha-fetoprotein.

Sensitivity of fabricated immunosensor
In order to examine the sensitivity of the 3D-Au-based biosensor, amperometric signals were measured before and after immunoreaction with the AFP antigen at different concentrations. shows an increase in the currents with increasing AFP antigen concentration in the range of 1–50 ng/mL. Under optimal conditions, a good linear relationship was observed between the current signal and the logarithmic value of the antigen concentration (shown in ) in the range of 10–50 ng/mL. The linear regression equation was
with a correlation coefficient 0.9950. The lowest detection limit of 3 pg/mL AFP antigen could be estimated using 3σ (where σ is the standard deviation of the blank solution, n=5). According to the linear equation, the concentration of AFP antigen could be detected quantitatively, and lower serum AFP levels could also be detected by appropriate dilution with pH 7.4 PBS.
Figure 5 Current-time curves for the capture antibody-modified 3D-Au electrode before and after incubation with different concentrations of AFP antigen in TMB solution. (A) Blank solution. Curves b-i represent 0.01, 0.05, 0.1, 1.0, 5.0, 10.0, 20.0, and 50.0 ng/mL AFP antigen, respectively. (B) Linear relationship between currents and logarithmic AFP antigen concentration (from 10 pg/mL to 50 ng/mL).
Abbreviation: AFP, alpha-fetoprotein.

To further elucidate the advantage of the developed electrochemical immunosensor, the sensitivity and linear range of the immunosensor were compared with other AFP electrochemical immunosensors.Citation22,Citation33–Citation38 As shown in , the sensitivity and linear range of the electrochemical immunosensor are comparable. Moreover, preparation of the 3D-Au electrode is more simple, more economical, and greener than the other nanocomposites.
Table 1 Comparison of different electrochemical immunosensors
Precision, reproducibility, and stability of AFP immunosensor
The precision of the electrochemical immunosensors was evaluated by calculating the intra-assay and interassay variation coefficients. The intra-assay precision of the immunosensor was evaluated by assaying one AFP level for three repeated measurements, whereas the interassay precision was estimated by measuring one AFP level with three immunosensors prepared independently under the same experimental conditions. The intra-assay and interassay variation coefficients obtained from 10 ng/mL AFP were 4.56% and 6.84%, respectively, indicating acceptable precision and reproducibility. Further, the stability of the electrochemical immunosensor was evaluated. The immunosensors were stored at 4°C. The amperometric currents were measured every 3–5 days using the same immunosensors. The currents were 97.3%, 94.9%, and 91.8% of the initial current at days 10, 20, and 30. The immunosensor was regenerated by rinsing with 0.1 M glycine-HCl (pH 2.2) stripping buffer for 3 minutes to dissociate the antigen-antibody complex.Citation39 The renewed immunosensor could restore 96% of the initial value after five assay runs, indicating high reusability and stability.
Application of immunosensor for human AFP levels
The AFP levels obtained in a human serum sample using the proposed immunosensor are shown in , which compares the results with those obtained from the Roche electrochemiluminescence immunoassay analyzer performed by the First Affiliated Hospital of Fujian Medical University. As shown in , the results obtained with the immunosensor are in good agreement with those measured by the electrochemiluminescence immunoassay analyzer. The relative standard deviation less than 5% in five experiments were in the acceptable range. Thus, this method has potential application for detection of AFP levels in clinical samples.
Table 2 Comparison of developed immunosensor and ECL immunoassay analyzer in detection of serum AFP levels (n=5)
Conclusion
In this work, an electrochemical biosensor was developed based on a 3D-Au electrode, and was prepared by a simple, economical, and controllable approach known as SWORC. Due to the superior conductivity and large surface area of the 3D-Au electrode, the developed immunosensor showed excellent sensitivity and selectivity for detection of AFP antigens. Compared with other electrochemical immunosensors,Citation22,Citation33–Citation38 the selectivity, sensitivity, and linear range of the developed immunosensor is competitive. Further, the procedure used to prepare the 3D-Au electrode is more simple, economical, and greener than the alternative nanocomposites. This new immunosensor was also used to assay AFP levels in a human serum sample and satisfactory results were obtained. This proposed method is stable, versatile, and highly sensitive, and should have wide application in the diagnosis and monitoring of cancers or other immunoassay for non-tumor biomarkers such as immunoglobulin, glycosylated hemoglobin, and troponin.
Acknowledgments
The authors gratefully acknowledge the financial support of the National High Technology and Development of China (2012AA022604), the National Natural Science Foundation of China (21275028, 81301520), the Natural Science Foundation of Fujian Province (2014J05088), the rolling subsidy scheme of Outstanding Youth Science Fund Project of Fujian province (2014J07009), the Scientific Research Major Program of Fujian Medical University (09ZD013), the Research Fund for the Doctoral Program of Higher Education of China (20123518110001), the Fujian Provincial Important Science and Technology Foundation (2011R1007-2) sponsored by the Medical Elite Cultivation Program of Fujian, People’s Republic of China (2013-ZQN-JC-5), the Science and Technology Plan Project, General Administration of Quality Supervision (2014IK060), the Foundation of Fujian Provincial Department of Education (JA11110, JA12130), and the Key Clinical Specialty Discipline Construction Program of Fujian, People’s Republic of China.
Supplementary material
Figure S1 (A) Effect of the concentration of the solutions used on incubation of capture and detection antibodies on the blank signal acquired after incubation with AFP-free solution. (B) Effect of incubation temperature on antigen-antibody interaction. (C) Effect of antigen-antibody incubation time on response of amperometric current.
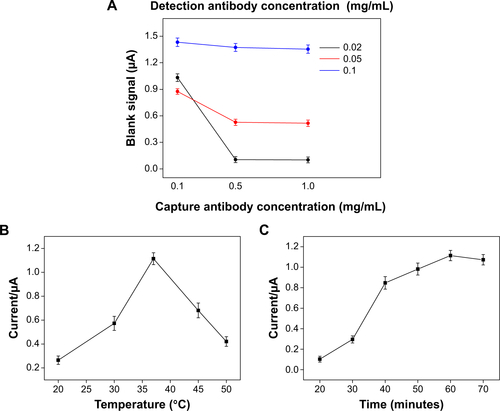
Disclosure
The authors report no conflicts of interest in this work.
References
- LiuGWangSLiuJSongDAn electrochemical immunosensor based on chemical assembly of vertically aligned carbon nanotubes on carbon substrates for direct detection of the pesticide endosulfan in environmental waterAnal Chem20128493921392822448910
- LiuJZanardiSPowersSSumanMDevelopment and practical application in the cereal food industry of a rapid and quantitative lateral flow immunoassay for deoxynivalenolFood Control20122618891
- Al-KhafajiQAMHarrisMTombelliSAn electrochemical immunoassay for HER2 detectionElectroanalysis2012244735742
- UludagYTothillIECancer biomarker detection in serum samples using surface plasmon resonance and quartz crystal microbalance sensors with nanoparticle signal amplificationAnal Chem201284145898590422681722
- KimYJRahmanMMLeeJJUltrasensitive and label-free detection of annexin A3 based on quartz crystal microbalanceSens Actuators B Chem2013177172177
- WengerBKugelbreyKGaoHSigristHVoirinGAu-labeled antibodies to enhance the sensitivity of a refractometric immunoassay: detection of cocaineBiosens Bioelectron2012341949922342363
- ZhangYDaiWJLiuFUltrasensitive electrochemiluminescent immunosensor based on dual signal amplification strategy of gold nanoparticles-dotted graphene composites and CdTe quantum dots coated silica nanoparticlesAnal Bioanal Chem2013405144921492923508581
- LiuLXingYZhangHLiuRLiuHXiaNAmplified voltammetric detection of glycoproteins using 4-mercaptophenylboronic acid/biotin-modified multifunctional gold nanoparticles as labelsInt J Nanomedicine2014912619262624920899
- DempseyEDiamondDCollierADevelopment of a biosensor for endocrine disrupting compounds based on tyrosinase entrapped within a poly(thionine) filmBiosens Bioelectron200420236737715308243
- HahmJLieberCMDirect ultrasensitive electrical detection of DNA and DNA sequence variations using nanowire nanosensorsNano Lett2004415154
- SánchezSPumeraMFàbregasECarbon nanotube/polysulfone screen-printed electrochemical immunosensorBiosens Bioelectron200723333234017560102
- RahmanMASonJIWonMSShimYBGold nanoparticles doped conducting polymer nanorod electrodes: ferrocene catalyzed aptamer-based thrombin immunosensorAnal Chem200981166604661120337374
- BangarMAShiraleDJChenWMyungNVMulchandaniASingle conducting polymer nanowire chemiresistive label-free immunosensor for cancer biomarkerAnal Chem20098162168217519281260
- LiFHanXPLiuSFDevelopment of an electrochemical DNA biosensor with a high sensitivity of fM by dendritic gold nanostructure modified electrodeBiosens Bioelectron20112652619262521146393
- DengYPHuangWChenXLiZLFacile fabrication of nanoporous gold film electrodesElectrochem Commun2008105810813
- ZhangHFHussainIBrustMButlerMFRannardSPCooperAIAligned two- and three-dimensional structures by directional freezing of polymers and nanoparticlesNat Mater200541078779316184171
- WeissmüllerJViswanathRNKramerDZimmerPWürschumRGleiterHCharge-induced reversible strain in a metalScience2003300561731231512690195
- ZielasekVJürgensBSchulzCGold catalysts: nanoporous gold foamsAngew Chem Int Ed2006454882418244
- LangXYGuoHChenLYNovel nanoporous Au-Pd alloy with high catalytic activity and excellent electrochemical stabilityJ Phys Chem C2010114626002603
- HuKCLanDXLiXMZhangSElectrochemical DNA biosensor based on nanoporous gold electrode and multifunctional encoded DNA-Au bio bar codesAnal Chem200880239124913019551936
- GaoWXiaXHXuJJChenHYThree-dimensionally ordered macroporous gold structure as an efficient matrix for solid-state electrochemiluminescence of Ru(bpy)32+/TPA system with high sensitivityJ Phys Chem C2007111131221312219
- LiYSongYYYangCXiaXHHydrogen bubble dynamic template synthesis of porous gold for nonenzymatic electrochemical detection of glucoseElectrochem Commun200795981988
- GameroMParienteFLorenzoEAlonsoCNanostructured rough gold electrodes for the development of lactate oxidase-based biosensorsBiosens Bioelectron20102592038204420171079
- ZhongGXLiuALChenXHElectrochemical biosensor based on nanoporous gold electrode for detection of PML/RARα fusion geneBiosens Bioelectron20112693812381721420849
- ZhongGXLiuALXuXWDetection of femtomolar level osteosarcoma-related gene via a chronocoulometric DNA biosensor based on nanostructure gold electrodeInt J Nanomedicine2012752753622334782
- ShimJHYoonDLHanSIs serum alpha-fetoprotein useful for predicting recurrence and mortality specific to hepatocellular carcinoma after hepatectomy? A test based on propensity scores and competing risks analysisAnn Surg Oncol201219123687369622644512
- HoHKPokSStreitSFibroblast growth factor receptor 4 regulates proliferation, anti-apoptosis and alpha-fetoprotein secretion during hepatocellular carcinoma progression and represents a potential target for therapeutic interventionJ Hepatol200950111812719008009
- FanCHPlaxcoKWHeegerAJElectrochemical interrogation of conformational changes as a reagentless method for the sequence-specific detection of DNAProc Nat Acad Sci U S A20031001691349137
- VazquezLBartolomeABaroAMSTM-SEM combination study on the electrochemical growth mechanism and structure of gold overlayers: a quantitative approach to electrochemical metal surface rougheningSurf Sci19892151–2171189
- LiuGWanYGauVAn enzyme-based e-DNA sensor for sequence-specific detection of femtomolar DNA targetsJ Am Chem Soc2008130216820682518459781
- Fanjul-BoladoPGonzalez-GarciaMBCosta-GarciaAAmperometric detection in TMB/HRP-based assaysAnal Bioanal Chem2005382229730215891870
- DasAHechtMHPeroxidase activity of de novo heme proteins immobilized on electrodesJ Inorg Biochem200710111–121820182617765314
- YangSJiaWZQianQYZhouYGXiaXHSimple approach for efficient encapsulation of enzyme in silica matrix with retained bioactivityAnal Chem20098193478348419354263
- WuYMXuWJWangYYuanYLYuanRSilver-graphene oxide nanocomposites as redox probes for electrochemical determination of α-1-fetoproteinElectrochim Acta201388135140
- ParkJYLeeYSKimBHParkSMLabel-free detection of antibody-antigen interactions on (R)-lipo-diaza-18-crown-6 self-assembled monolayer modified gold electrodesAnal Chem200880134986499318505271
- HuangKJLiJWuYYLiuYMAmperometric immunobiosensor for α-fetoprotein using Au nanoparticles/chitosan/TiO(2)-graphene composite based platformBioelectrochemistry201390182323165290
- LiuSZhangJTuWBaoJDaiZUsing ruthenium polypyridyl functionalized ZnO mesocrystals and gold nanoparticle dotted graphene composite for biological recognition and electrochemiluminescence biosensingNanoscale2014642419242524435065
- ZhaoLLiSHeJTianGWeiQLiHEnzyme-free electrochemical immunosensor configured with Au-Pd nanocrystals and N-doped graphene sheets for sensitive detection of AFPBiosens Bioelectron20134922222523770392
- LinJWeiZZhangHShaoMSensitive immunosensor for the label-free determination of tumor marker based on carbon nanotubes/mesoporous silica and graphene modified electrodeBiosens Bioelectron20134134234723010015