Abstract
Therapeutic interventions based on the transplantation of stem and progenitor cells have garnered increasing interest. This interest is fueled by successful preclinical studies for indications in many diseases, including the cardiovascular, central nervous, and musculoskeletal system. Further progress in this field is contingent upon access to techniques that facilitate an unambiguous identification and characterization of grafted cells. Such methods are invaluable for optimization of cell delivery, improvement of cell survival, and assessment of the functional integration of grafted cells. Following is a focused overview of the currently available cell detection and tracking methodologies that covers the entire spectrum from pre- to postmortem cell identification.
Introduction
Cell transplantation has been explored as a new clinical approach to repair injured tissue. Recently, the therapeutic properties of a variety of cell types have been studied extensively, and it is now clear that cells delivered to remote sites home to the area of injury and stimulate repair and regeneration of the damaged tissue. However, to gain some insight into exogenous cell migration, tissue localization, and the level of engraftment, the cells require labeling and subsequent tracking. Moreover, cell-tracking studies require a label that it is uniquely distinguishable and biologically stable for the quantitative analysis of dynamic processes in living cells. A variety of methods have been developed to identify transplanted cells premortem (in vivo) and postmortem (ex vivo). These methods rely on various contrast mechanisms and most require some premodification of the cells of interest. In this review, we describe a broad range of techniques that are suitable for cell-tracking studies, from methods applicable solely for postmortem microscopy assessment to those that facilitate longitudinal cell-tracking in live animals and from physical cell labeling methods to the use of reporter genes or strategies that do not require exogenous labeling, but are based on the detection of inherent marker labels, such as the Y chromosome, newly replicating DNA, or natural mutations.
Premortem cell detection techniques
SPECT and PET imaging
Radioactive substances have been employed in medical practice for many years. While the resolution of single-photon emission computed tomography (SPECT) and positron emission tomography (PET) is relatively low, nuclear medicine compensates with an outstanding sensitivity at the whole-body level. One of the main differences between the SPECT and PET techniques is the type of radioisotopes. In SPECT, radioisotopes directly emit gamma waves, whereas in PET imaging, the nuclei of the radioactive element first generate a positron, which annihilates after contact with the electron from the body, producing two collinear gamma rays that are then detected coincidently. Technetium-99m, Indium-111, and Gallium-67 are the most commonly used radiometal isotopes in SPECT, while Galium-68, Copper-64, Yttrium-86, and Zirconium-89 are usually applied in PET. Moreover, there are several nonmetallic radionuclides that could be utilized for PET, including 18F, 11C, 13N, 15O, and 124I. Most of the radioisotopes mentioned earlier have been adapted for oncological diagnosis and therapy. Before application, they are conjugated with an adequate chelator (eg, DOTA, DTPA, TETA) and/or a targeting molecule (antibody or small protein) in order to obtain thermodynamic stability, chemical inertness, and optimal biodistribution in the body.Citation1,Citation2 The labeling of cells with radioisotopes is a simple and very efficient procedure, but preparation of radioisotopes is complex and costly, including access to highly special-ized equipment at the site of application, since, due to rapid decay, the labels cannot be prepared in advance. While the compounds bearing radioisotopes are easily internalized by cells from the high-concentration incubation medium, the radioisotopes can leak.Citation3 Kim et alCitation4 used a direct labeling method for rat adipose-derived stem cells, with a radioactive 124I-HIB-label. PET imaging combined with computed tomography enabled the identification of cells after transplantation in the myocardium for more than 9 days. This technique also provided additional information about the survival rate of transplanted cells. The radioactivity level was inversely proportional to that of apoptosis of transplanted cells.Citation4 However, in general, the fast decay of radioactivity is another obstacle allowing for cell imaging only acutely after transplantation. The half-life of SPECT radioisotopes ranges from hours to a few days, and it is even shorter for the 18F used for PET imaging. In addition, the diagnostic/therapeutic centers must be equipped with highly specialized instruments. The doses applied clinically spread throughout the body, thus carrying minimal risk for individual cells. In contrast, the accumulation of radioisotopes in labeled cells can be potentially destructive, as it is for thyroid cells treated with radioiodine.Citation5,Citation6 Despite the drawbacks, radioisotopes are actively involved in stem cell tracking both in the preclinical setting and in clinical trials.Citation7,Citation8
Radioisotopes can be used for direct labeling of cells, but also can be conjugated with ligands of specific receptors present in a particular type of cell. A properly constructed compound can identify the transplanted stem cells inside the host body. Tarantal et al,Citation9 showed that injection of 89Zr conjugated to human-specific CD45 antibody into the circulatory system of Rhesus monkeys facilitated imaging of the biodistribution of grafted human stem cells with PET. Another interesting application of noninvasive PET imaging was geared at differentiation of cancer stem cells from healthy brain cells. The CD133 epitope being a promising cancer stem cell marker was used for that purpose. Radioactive tracer 64Cu-NOTA conjugated to CD133 antibody was shown to successfully detect cancer stem cells in both subcutaneous and intracranial tumors.Citation10 Another example of that system consists of a somatostatin receptor and a derivative of the somatostatin inhibitor, [111In]DTPA-octreotide (Octreoscan), which is approved in the United States and Europe for the diagnosis of tumors with high endogenous somatostatin receptor expression, such as breast cancers, pituitary tumors, or pheochromocytomas, but such technology has not been used for the detection of transplanted cells as yet.Citation11
A PET reporter gene/probe system was described for the first time by Tjuvajev et alCitation12 in 1995. It was based on the use of a reporter gene together with a reporter probe. There are three main classes of reporter genes: enzymes, receptors, and transporters. The reporter gene can be introduced in an expressive vector into transplanted cells, and its product accumulates inside the cells. Then, the product of the reporter gene interacts selectively with radioactive molecules that are delivered in the reporter probe, which results in the selective accumulation of the probe in transplanted cells. These cells can then be detected using PET. An example of this kind of system is an in vivo tracing of transplanted rat bone marrow mesenchymal stem cells (MSCs) transfected with the recombinant adenovirus vector, Ad5-hERL-IRES-VEGF (Ad-EIV), in the muscle of a rat host. Ad-EIV carries a reporter gene (hERL) coding the human estrogen receptor ligand-binding domain (hERL). After intravenous tail vein injection of a radiolabeled, biologically inactive, estrogen analog, 16α-[18F] fluoro-17β-estradiol (18F-FES), the radioactive signal originating from the grafted cells was visible with PET.Citation13 The engraftment of human and rat hematopoietic stem cells in the rat was successfully observed in vivo using 1-(2-deoxy-2-18fluoro-β-L-arabinofuranosyl)-5-methyluracil and deoxycytidine kinase as a reporter gene. This kinase carries a mutation within the active site.Citation14 The use of another kinase – the herpes simplex virus thymidine kinase (sr39TK) – as a reporter gene was shown to be very beneficial in PET imaging of transplanted, genetically modified human hematopoietic stem cells in rats. The radioactive signal from stem cells engineered to express sr39TK could be recorded after the infusion of [18F]-FHBG. Moreover, sr39TK is also a suicide gene, and, after administration of gancyclovir, the cells that express its active form undergo apoptosis. The possibility to induce fast and selective destruction of a transplanted, genetically modified stem cell population is quite important in the context of possible clinical application, alleviating to some extent safety concerns related to insertional mutagenesis and cell transformation.Citation15 The human sodium iodide symporter (hNIS) is another reporter gene that is increasingly used. It has demonstrated success in monitoring adenoviral-based gene delivery.Citation16 The expression of hNIS has been easily achieved in tumor cells, and the subsequent application of the radioactive 188Re probe proved to be theranostic.Citation17 Moreover, the tumor-homing property of MSCs has been used for tumor-selective radionuclide accumulation via hNIS expression, with positive therapeutic effects.Citation18 The hNIS has been also used in the field of regenerative medicine to determine the viability of transplanted cells, and has shown less variable results, and thus, a superior profile compared to eGFP (enhanced green fluorescent protein) for in vivo imaging.Citation19,Citation20 The observation of stem cells labeled with radiotracers can not only provide researchers with information about the fate of these cells, but can also enable optimization of the delivery route and technique.Citation21 The increasing requirement for a detailed visualization of stem cells often leads to the development of multimodal approaches. Some newly introduced radioisotopes, such as 52Mn, can also be visualized by both PET and magnetic resonance imaging (MRI) scanners.Citation22 However, the application of radioactive tracers for regenerative medicine carries the risk of not only radiation-induced cell death, but also mutagenesis, which could potentially result in tumor formation, a very grim complication even in a very delayed fashion. In addition, the crossing of radionuclides through the intact blood–brain barrier, as present in restorative neurotransplantation, has not been studied as yet.
X-ray and US imaging
X-ray-based fluoroscopy and computer tomography, as well as ultrasonography, are extensively exploited modalities in clinical imaging. X-ray-based methods of cellular imaging work by the absorption of X-rays by contrast agents, which are detected by various 2D and 3D detectors. Ultrasonography depends on the recording of echoes of ultrasonic waves. Heavy elements are the preferred cellular labels for X-ray imaging, while bubbles are the most frequently used contrast agents for ultrasonography. Unfortunately, even heavy elements and bubbles in cell-loadable quantities are difficult to detect with current state-of-the-art detectors. Thus, indirect approaches have been tested to support cell transplantation with these modalities, including, for example, coencapsulation of cells with bromine compounds.Citation23,Citation24 Another proposed option is the suspension of cells within a tantalum-labeled scaffold (hydrogel).Citation25 Microbubbles can be easily internalized by stem cells, thus enabling their localization within internal organs, but such an approach is not useful for cell imaging within the central nervous system due to the low bone permeability of ultrasonic pulses.Citation26 What is of interest is the current use of extracellular bubbles to facilitate cell homing to injured tissues after intravascular delivery.Citation27,Citation28
Relaxation-based MR contrast agents
In vivo tracking of stem cells with MRI based on relaxation requires prelabeling of cells with special compounds that can change the water relaxation time and/or magnetic susceptibility, and then, determining the location of these compounds based on the image intensity. MRI contrast agents can be divided into two main groups: exogenous and endogenous.
Metal-based compounds are primary among the exogenous-based labeling strategies. Metallic marker tags can be primarily based on iron, manganese, and gadolinium. They can be divided into two main groups. The first group includes MRI contrast agents that affect the longitudinal relaxation time, T1, where the spin lattice relaxation time is generated. T1-weighted contrast agents involve gadolinium (Gd3+) and manganese (Mn3+) chelates, where the mode of action is based on the decrease of the T1 relaxation time. In practice, highly intense T1-weighted images are produced with positive contrast. Contrast agents for stem cell labeling based on Gd elements include chelated Gd–lipid nanoparticles, Gd-chelated dextran nanoparticles, and Gd-DOTA–peptide complexes, and gadolinium oxide nanoparticles.Citation29–Citation32 However, the use of gadolinium nanoparticles in stem cell tracking is plagued by significant risk of cytotoxicity, which may compromise cell function or survival. It is also unclear how these compounds are metabolized in labeled cells.Citation33 There is a potential threat that the toxic, free inorganic Gd3+ could be released and influence cell metabolism of the labeled cells as was reported in the case of the rat spinal cord cultured neurons, where free Gd3+ distorted the activity of K+–Cl− membrane cotransporter.Citation34
Among the contrast agents with manganese compounds as an MRI signal source, MnCl2, and mesoporous and hollow MnO nanoparticles have been employed.Citation35–Citation37 An interesting study was performed with the systemic administration of MnCl2 that made possible simultaneous monitoring and elimination of human embryonic stem cell-derived teratoma cells.Citation38 While a T1-positive contrast agent is more desirable, it is also characterized by lower sensitivity than T2* contrast agents, which capitalize on the magnetic field inhomogeneity produced by iron-oxide-based compounds. The second main group of the metallic contrast agents are those related to transverse relaxation time, T2, where the spin–spin relaxation time is reduced. T2-weighted contrast agents are primarily superparamagnetic iron nanoparticles (SPIOs). The most common use for stem cell labeling is related to Fe3O4 magnetite nanoparticles, which are composed of nonstoichiometric Fe2+ and Fe3+ oxides.Citation39,Citation40
Several types of SPIOs can be used for stem cell imaging, such as citrate-coated or dextran-coated SPIOs and ferumoxides.Citation41,Citation42 Ferumoxides are composed of iron particles of approximately 5 nm in size, but with a hydrodynamic diameter of approximately 80–150 nm, where the iron crystals are covered with a dextran layer. Some ferumoxides are combined with poly-L-lysine.Citation43 Ultrasmall SPIOs (USPIOs), whose diameter size fluctuates approximately 35 nm, are another type of SPIOs. This type of contrast agent has been used successfully for adult rat neural stem cell labeling and tracking.Citation44
A commercially available Feraheme® (ferumoxytol, AMAG Pharmaceuticals, Waltham, MA, USA) is an iron oxide with a hydrophilic carboxydextran coat.Citation45 The overall colloidal particle size is approximately 17–31 nm in diameter. Ferumoxytol was found to be useful in human neural stem cell tracking.Citation46 For instance, the meso-2,3-dimercaptosuccinic acid-coated iron nanoparticles were used for efficient adipose-derived stem cell labeling, thereby increasing the spectrum of possible uses for different coating materials.Citation47 An elegant method of simultaneous MRI and fluorescence imaging is achieved by using Molday ION Rhodamine B nanoparticles, where 50 nm magnetite-based nanocolloidals are also labeled with the fluorochrome Rhodamine B, with a 565–620 nm emission wavelength.Citation48 A similar approach was employed in bone marrow-derived stem progenitor cells, where cells were labeled with the T2 contrast agent ferumoxide and a fluorescent tissue marker.Citation49 Ferumoxide has also been successfully used in intraventricular delivery in the pediatric patient with global ischemia (), and long-term observation did not reveal negative consequences.Citation50 Microsized paramagnetic iron oxide nanoparticles are another type of MRI contrast agent, in which the diameter of nanoparticles is approximately 1 µm.Citation51 For instance, iron nanoparticles were covered with a divinylbenzene polymer to detect the migration of bone marrow-derived stromal cells in a stroke model.Citation52 Despite the numerous examples of its use, similar to Gd-based contrast agents, SPIO labeling does not seem to be neutral for labeled cells. SPIO-based stem cell labeling as a method is presently not FDA (US Food and Drug Administration) approved, because the molecular mechanisms of SPIO actions are not clearly understood as yet. So, there are still concerns about the uncertain side effects on cell function, as well as the possible negative influence on the fate of the labeled cells.Citation53 For instance, labeling with SPIOs could impair functional properties like migration capacity and colony formation abilities of labeled MSCs.Citation54 However, there are FDA-approved SPIO formulations, but each clinical application needs Institutional Review Board’s approval for specific SPIO formulation and cell type to be used. In summary, despite the fact that several different MRI contrast agents exist and safety issues are not still resolved, the most popular T2 and T1 MRI-based stem cell labeling and in vivo tracking agents are SPIOs and gadolinium oxide nanoparticles.Citation33 There are some data that indicate that the use of dual T1 and T2 MRI contrast agents could improve cell detection accuracy.Citation31 SPIO-based agents are the only MR contrast agents that have so far been used for clinical (stem) cell tracking.Citation55
Figure 1 Imaging of SPIO-labeled autologous cord blood derived cells in a patient with global cerebral ischemia.
Notes: (A) Volume rendering of MRI data of the patient’s head obtained 24 hours posttransplantation. Semiautomatic segmentation is based on pixel intensity, showing the projection of the ventricular system (green) and the distribution of the SPIO signal from the transplanted cells within the occipital horn of the right ventricle (red). Note the supine configuration of the head, corresponding to positioning during surgery. The route and trajectory of cell transplantation via the frontal horn is represented by the needle. (B) Posterior-superior view of the patient’s head, emphasizing the location of the hypointense SPIO signal from autologous cord blood-derived cells transplanted within the occipital horn. (C) T2*-weighted image with an orthogonal view centered on the cellular SPIO signal in the occipital horn (white arrowhead). (D–I) Sagittal T2*-weighted MRI scans showing a longitudinal dispersion of SPIO signal within the occipital horn (white arrowheads); (D) pretransplantation, (E) 24 hours posttransplantation (PT), (F) 7 days PT, (G) 2 months PT, (H) 4 months PT, and (I) 33 months PT.
Abbreviations: SPIO, superparamagnetic iron nanoparticle; MRI, magnetic resonance imaging.
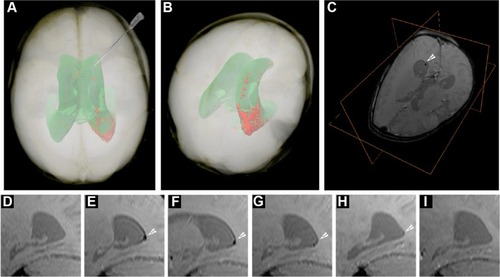
Apart from the metallic elements approach for MRI, stem cell labeling with the use of nonmetallic elements, such as fluorine, can be efficiently implemented, despite the lower sensitivity compared to iron-based nanoparticles.Citation56–Citation58 In addition to exogenous substances for MR labeling, endogenous MR tracer tags can be specified. An interesting technical solution for the MR labeling is a genetic-based strategy. Bengtsson et alCitation59 reported that in β-galactosidase-engineered bone marrow cells, β-galactosidase activity in the presence of a special compound – S-Gal™ (Sigma-Aldrich, St Louis, MO, USA) – resulted in enhanced T2 MR contrast imaging. In another biological MRI approach, cells of interest were transfected with genes encoding proteins that were, in turn, able to bind iron particles, such as ferritin, transferrin receptors, and iron transporters derived from magnetotactic bacteria for the biomineralization of iron.Citation60–Citation63 In addition, stem cell surfaces could be engineered by artificial antigens with subsequent detection by SPIO-coupled antibodies.Citation64
Chemical exchange saturation transfer (CEST) MRI
MRI has been used extensively over the last 3 decades for anatomical, functional, and dynamic imaging. Rapid improvements in MRI instrumentation and techniques have led to increased spatial resolution.Citation65 The MRI contrast can be further enhanced by the expression of certain proteins (encoded by genes, termed reporter genes) that increase the MRI contrast. For instance, these proteins can be involved in iron metabolism and storage, and can act as enzymes that convert certain compounds to paramagnetic contrast agents.Citation66–Citation71
(Paramagnetic) chemical exchange saturation transfer ([PARA]CEST) is a novel approach for generating MRI contrast, in which the dynamic exchange process between an exchangeable proton and the surrounding water protons is used to amplify the desired contrast.Citation72–Citation74 One advantage of CEST is that the magnetization of different protons can be specifically saturated at different resonance frequencies, enabling the in vivo detection of multiple targets simultaneously.Citation75 This rapidly evolving approach to creating contrast has been applied to detect temperature changes, pH, enzyme activity, metal ions, and metabolites like glycogen and glucose, and glycosaminoglycan.Citation76–Citation87
One potential application of such reporter genes is in regenerative medicine, where cells (eg, stem, progenitors, or immune cells) are transplanted into patients to repair a damaged tissue. Since the fate of these cells after transplantation is mostly unknown, it would be greatly beneficial if the cells could be tagged. Ideally, the “tag” should be a protein or an enzyme that is expressed only in the transplanted cells and for as long as the cells are viable. Therefore, reporter genes based on CEST MRI have an advantage, since they are bioorganic and biocompatible and are constitutively and continuously expressed by the cell. The first generation of a CEST-based reporter gene was a synthetic gene that encodes a lysine-rich protein. This reporter was used to distinguish glioma cells that overexpress the transgene from control cells in vivo in an animal model.Citation88 Along the same lines, a synthetic gene was used to encode to an artificial protein that could sense cellular signaling.Citation89 These studies were followed by a reengineering of the human protamine 1 (hPRM-1) gene as a CEST-based reporter. Since the hPRM-1 is a human protein, which is normally only expressed in sperm cells, it has a very low background signal in the body. Moreover, this is a human protein, and, therefore, would not be expected to trigger an immune reaction. Protamine was also used to monitor sustained drug release. Recently, CEST was applied to detect the activity of the theranostic enzymes, cytosine deaminase, carboxypeptidase G2, and herpes simplex virus type-1 thymidine kinase (HSV1-TK) ().Citation80,Citation90–Citation93 In these cases, the reporter can be used both for tagging the cells, if an imaging probe is used, and also as a suicide gene if a different compound (a prodrug) is used. Thus, such a theranostic gene has an additive value, especially where cells are needed to eradicate tumors. Unlike other labeling techniques, which have a very limited selection of compounds that can be used as imaging agents, CEST MRI that relies on bioorganic compounds allows almost endless possibilities for probe selection and design. Therefore, this technology holds great promise for the next generation of diagnostic imaging tools.
Figure 2 High-resolution MRI images.
Notes: Brain has two tumors, a control (wt) and a glioma expressing a recombinant MRI reporter HSV1-TK, highlighted using CEST imaging.
Abbreviations: MRI, magnetic resonance imaging; HSV1-TK, herpes simplex virus type-1 thymidine kinase; CEST, chemical exchange saturation transfer; h, hour.

Bioluminescent imaging (BLI)
BLI is an attractive approach for tracking transplanted cells, as it specifically, and with relatively high sensitivity, reports on the viability of cells expressing a BLI reporter gene. In addition, this technique with the use of specific promoters, eg, Oct4 could potentially be used to observe alterations of gene expression in cultured or transplanted stem cells.Citation94 This imaging modality relies on stable expression of the reporter gene in the target cells, and such expression can be induced using one of the well-established molecular biology techniques, eg, lentiviral transduction, or with the use of primary cells isolated from transgenic, light-producing animals.Citation95–Citation100 Several BLI reporter genes have been isolated, including the North American firefly (Photinus pyralis; FLuc), jellyfish (Aequorea), sea pansy (Renilla; RLuc), corals (Tenilla), the click beetle (Pyrophorus plagiophthalamus), and several bacterial species (Vibrio fischeri, Vibrio harveyi); however, for cell tracking purposes, FLuc is, by far, most widely used.Citation101 The variety of currently available luciferase types with different substrate specificities opens a new possibility to observe two or more cell lines simultaneously. Leng et alCitation102 adapted this idea to show the therapeutic effect of transplanted MSC in a model of human breast cancer. In this study, MSCs engineered to express RLuc were injected in mice bearing Fluc-expressing tumors, and due to different enzyme substrates, it enabled simultaneous detection of both MSCs and tumor cells. That imaging paradigm revealed the inhibition of cancer progression induced by the presence of MSC. This example showed that dual luciferase imaging enables investigation of the interaction between cell populations.Citation102
The principle of BLI is based on the luciferase reaction that requires an enzyme (firefly luciferase), its substrate (D-luciferin or coelenterazine), ATP, and oxygen. This enzyme-catalyzed oxidation results in oxyluciferin, a product that, when decaying, emits photons. Photons are detected by specialized charge-coupled device (CCD) cameras that convert photons into electrons. The noise of the system is reduced by supercooling the CCD camera and mounting the camera in a light-tight chamber (). The sensitivity of this imaging modality is dependent on several factors, including the optical properties and expression level of the reporter gene, the depth of labeled cells within the body, and the sensitivity of the detection device.Citation103 The major challenge of in vivo BLI is that emitted photons must pass through the tissues and have to be detected outside the body. For that reason, detection of cells implanted superficially is much more effective than are deeper targets. As a general rule, the signal drops approximately tenfold for each 10 mm of tissue depth.Citation104 In order to address the problem of tissue attenuation that limits sensitivity, significant effort has been directed toward improving the optical properties of imaging probes. The potential strategies include developing reporter genes with red-shifted emission profiles of photons known to have better tissue penetration, or developing new generations of substrates, such as CycLuc1, which offers significantly better pharmacokinetics and effectively results in an improved imaging signal.Citation96,Citation105 Another technique with the potential to improve detection of luciferase-expressing cells is based on the modification of ABC-transporter activity. These proteins are involved in the removal of xenobiotics from cells. The result of their inhibition is a higher accumulation of D-luciferin inside cells, and thus, an increased availability of substrate for the luciferase enzyme.Citation106 An important advancement in BLI was the development of image processing algorithms for extracting spatial information from the imaging signal, referred to as optical tomography.Citation107 Another quite interesting alternative is the use of bioluminescent luciferase from Gaussia princeps (GLuc), which is naturally secreted from cells in an active form. GLuc is present in body fluids. After the addition of the substrate to urine or serum, the measurement of the bioluminescent signal can provide information about the condition and viability of the transplanted cells.Citation108
Figure 3 Schematic outline of bioluminescence cells creation and their identification by BLI method in host body after transplantation.
Abbreviation: BLI, bioluminescent imaging.
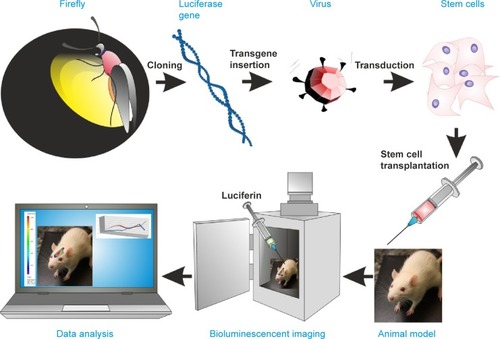
Overall, the advantages of BLI are robust reporter genes, effective and nontoxic substrates, and low cost and high throughput. These qualities make it an excellent and broadly used imaging modality for monitoring the survival of transplanted cells in small animals.Citation109 The disadvantages of this technique include low spatial resolution, and susceptibility to the dynamic changes in light scattering and absorption properties of the tissue (bleeding, vascularization, pigmentation, etc), which can compromise the accuracy of the quantitative analysis of the photon signal.
Optoacoustic/photoacoustic imaging
Optoacoustic imaging is based on the phenomenon of a transverse wave of light (photons generated by a short laser pulse or less expensive high-power LED [light-emitting diode]) hitting specific molecules and causing their transient thermal expansion, which, in turn, generates an acoustic wave sensed by external detectors, such as microphones or piezoelectric tools ().Citation110 Thus, the stimulation is the same as for fluorescent imaging, but the detected signal is based on an acoustic wave, which can be a huge advantage. In fluorescent imaging, the energy of photons generated by a fluorescent lamp or a laser is sufficient for relatively deep penetration to tissues, and the limiting factor is the energy of reflected photons, which is insufficient to reach detectors. In optoacoustic imaging, this limitation is overcome by the detection of an acoustic wave, which has dramatically better penetration of tissues. The lack of tissue autofluorescence is an additional advantage of optoacoustic technology. While fluorescence provides a scattering contrast, optoacoustic technology provides an absorption contrast.Citation111,Citation112 Several systems have been developed for optoacoustic imaging. Portable, hand-held imaging probes can reach 1.5 cm in depth at a speed of 10 volumetric frames per second and a spatial resolution of 200 µm.Citation113 Photoacoustic microscopy provides high-resolution images of tissue slices, can be combined with multiphoton microscopy, and can also be used for in vivo photoacoustic imaging.Citation115 The recent development of optoacoustic devices with tomographic capabilities, based on multispectral immixing (multispectral optoacoustic tomography – MSOT), has fueled even more interest in this imaging modality.Citation116–Citation118 This was made possible because of the relatively high yield of the acoustic signal. An MSOT device has just come on the market (http://www.ithera-medical.com).
Figure 4 Depiction of the mechanism of optoacoustic imaging.
Note: Copyright © 2015. Reproduced with permission from iThera Medical, (http://www.ithera-medical.com/technology/msot-principle.html).Citation114

Optoacoustic imaging can be virtually always applied to fluorescence imaging, including reporter genes and probes.Citation118,Citation119 The resolution of images from infrared fluorescent protein-labeled cells has been found to be 10× higher (100 µm) compared to fluorescence molecular tomography.Citation119 However, the applicability goes beyond fluorescence, with the recognition of gold as a strong generator of an optoacoustic signal.Citation119–Citation121 Gold nanoparticles, nanorods, and nanocages are widely available and biocompatible; thus, they have been increasingly used as an optoacoustic contrast agent.Citation122–Citation124 The utility of optoacoustic imaging has been shown to be advantageous not only for cell labeling, but also for the imaging of biomaterial scaffolds ().Citation19
Table 1 Summary of most commonly used techniques for premortem identification of transplanted cells
Postmortem cell identification
Immunohistochemical and genetic techniques
Thymidine analog markers
Various thymidine analogs can be used effectively to label proliferating cells. Among these, 5-bromo-2′-deoxyuridine (BrdU) is extensively used for labeling cells through its incorporation into newly synthesized DNA. BrdU applied to donor cells before transplantation could be used to trace their migration and identify the destination and accumulation in the host tissues.Citation125–Citation127 However, this method has a number of limitations and pitfalls. It has been shown that BrdU can be transmitted from the labeled cells into the neighboring cells of the recipient, leading to incorrect results by identifying the host cells as transplanted cells.Citation128 Furthermore, due to proliferation of the originally labeled cells, the interpretation of a positive signal is often merely guesswork due to the label dilution when BrdU is transferred to the daughter cells.
A new thymidine analog, 5-ethynyl-2-deoxyuridine (EdU), has been introduced for tracking transplanted cells.Citation129 While BrdU leaks from the labeled cells, EdU overcomes this difficulty since it is covalently bound to DNA and persists in the nucleus of tracked cells.Citation130 It was shown that EdU labeling did not interfere with in vitro cell proliferation, differentiation, cytokine secretion, or migratory response.Citation131 EdU-tracked cells are detected by a chemical reaction using an azide-conjugated fluor (red or green Alexa Fluors) that binds to the EdU alkyne moiety. However, sometimes “false-positive” staining occurs since certain types of unlabeled cells, eg, bone marrow cells, reveal a positive reaction with Alexa-azide fluor in the absence of an alkyne label.Citation132
Species-specific markers
In the context of preclinical studies in xenogeneic transplantation, it is possible to use species-specific markers to distinguish donor cells from surrounding host cells. Thus, exogenous cells of human origin, engrafted within rodent tissues, could be identified using antibodies specific for human antigens. Recently, successful attempts at species-specific immunolabeling include antibodies to anti-human mitochondria, anti-human nuclei, or anti-human neuron-specific enolase.Citation133–Citation137
Y chromosome markers
Localization of donor-derived cells in the sex-mismatched transplant recipients can be detected by chromosome fluorescence in situ hybridization (FISH). Y chromosome-specific probes for FISH have been used to determine male cells transplanted into female recipients in both animals and humans.Citation138,Citation139 This method could be used to identify transplanted cells for a long period of time, regardless of their differentiation into different cell phenotypes.Citation140,Citation141 Double staining of tissue sections by Y chromosome FISH and immunohistochemistry enables monitoring of the path taken by transplanted male cells and their fate toward maturation.Citation142,Citation143 When FISH is combined with nuclear dyes, eg, DAPI (4′6-diamidino-2-phenylindole) or ethidium bromide counterstaining to highlight the nucleus, the nuclear localization of the Y chromosome probe could be confirmed, thus eliminating false-positive results.
Fluorescent dyes
The widespread use of fluorescence-based microscopy resulted from the many available fluorophores that could be used to label cells. Fluorophores are divided into exogenous dyes that interact with different cellular components or endogenous fluorescent proteins constitutively produced by cells. The density of staining and localization of fluoro-phores is influenced by their properties and the target cells being labeled. Fluorescent dyes are suitable for attaching to the cell structures of interest and for controlled localization and expression levels.
Cell membrane dyes
Lipophilic carbocyanine fluorescent dyes that bind to the cell membrane lipid bilayer have been widely used due to their low cytotoxicity. Membrane dyes can be used to visualize the donor cells in the host tissues or to assess the proliferation of transplanted cells after their division. Commonly used are PKH lipophilic dyes, eg, PKH26 used to track stem cells derived from the different sources.Citation134,Citation144–Citation146 These dyes stain the whole plasma membrane of cells through lateral diffusion, then spread into intracellular compartments.Citation147 Recently, PKH dyes have been validated for their applicability to stain microvesicles isolated from MSC.Citation148
Other types of carbocyanine lipophilic membrane dyes, such as the chloromethyl-dialky-carbocyanine (CM-DiI) series, have been recently used to track multiple cell populations.Citation149,Citation150 These dyes have a number of advantages. They heavily intercalate within the lipid layer of the cell membrane, providing strong, highly photostable and long-lasting staining.Citation151 Most importantly, these dyes have not been reported to be cytotoxic, even at high concentrations. However, CM-DiI dyes reveal decreased detection after mitotic cell division when the fluorescence of daughter cells appears to be halved compared to that of the mother cells.Citation152 More importantly, the most significant problem with lipo-philic membrane dyes is their transfer between the cells. The acquisition of PKH and CM-DiI dyes by neighboring cells, mostly tissue macrophages, has been shown, particularly if the labeled cells were dead.Citation153,Citation154 Although, even if there are no dead cells among the transplanted cell population, the noncovalently bound DiI can dissociate from labeled donor cells and get picked up by the host cells.Citation155
Cytoplasmic and nuclear dyes
Cytoplasmic and nuclear dyes seem to be very efficient for cell tracking; however, they can affect different cellular functions. There are several fluorescent dyes permeable through the cell membrane. Among these, CFDA-SE (carboxyfluo-rescein diacetate succinimidyl ester) and CFSE (carboxyfluo-rescein succinimidyl ester) are frequently used.Citation156–Citation159 These dyes penetrate the cells where they are metabolized to amine-reactive chemicals, which then covalently bind to cytosolic cell components and are retained within the cytoplasm for a long period of time in vitro.Citation147 However, CFDA-SE and CFSE are easily photobleached and their fluorescence decreases rapidly within the tissue. For this reason, they could be used for cell labeling only in short-term studies.
The other fluorescent dyes, eg, Hoechst and DAPI, reveal a high affinity for double-stranded DNA. They are mostly used to stain the nuclei of fixed cells in immunofluorescent studies, but they also could be applied to track cells before transplantation.Citation160 However, despite the high labeling efficiency of dead cells after their fixation, DAPI and Hoechst are poor dyes for live cells due to inefficient penetration of the intact cell membrane. Moreover, since binding to DNA is noncovalent, the label can dissociate from DNA and it can be released from donor cells and taken up by the host cells, giving false-positive results.
All the abovementioned methods of staining are primarily based on immunohistological evaluation, which provides only “snapshot” evidence rather than a comprehensive study of transplanted cells over time. Such limited techniques partially reflect the conflicting observation of exogenous cells in the host. To address these limitations, the most reliable methods for assessing transplanted cells in vivo were determined. Among these methods, different reporter genes that are introduced into cells in vectors or integrated into the DNA of transplanted cells are highly accurate tools for following cell graft fate. Stable expression of fluorescent proteins in cells can be obtained through transfection and enables the long-term detection of genetically engineered cells in vitro and in vivo.
Reporter genes
Fluorescent proteins
GFP, first isolated from the jellyfish Aequorea victoria, is a widely used fluorescent reporter in various in vivo studies. The emission of light occurs after absorption of radiation in the ultraviolet spectrum. GFP-transgenic animals expressing this fluorescent protein in all tissues have been extensively maintained, and the isolated cells were applied in a suitable host.Citation161–Citation163 However, the level of GFP expression in transgenic animals is highly variable among animals and even among the cells within the same animal.Citation164 Harting et alCitation165 reported that only 50% of MSCs isolated from GFP-transgenic rodents expressed GFP. Due to insufficient GFP expression and the complexity and cost of development of transgenic animals, vector-mediating GFP transfection in vitro is still the preferred technique for GFP labeling.Citation166–Citation168 The generation of various colors of blue/yellow-shifted GFP variants, or the mutation-based development of red fluorescent proteins from anemones and tropical corals, have enlarged the reporter strategies available to track cells. The transfected GFP is densely localized and tightly packed in the nucleus, making the fluorescent signal in a small area easy to detect in the deep tissues after transplantation of GFP+ cells. However, the GFP gene is also problematic because many mammalian tissues are endogenously fluorescent. Another limitation of GFP usage is the oxygen accessibility that is necessary for proper fluorophore formation.Citation169 This type of fluorescent dye may also interfere with DNA replication or transcription, and thus, may affect cell function. While fluorescence can potentially also be visualized in vivo, the amount of fluorescent signal is very low due to light absorption by the host tissues. In addition, because of the additional autofluorescence, this method is used for postmortem identification of transplanted cells. Even in that case, the signal from GFP must be enhanced by the application of specific antibodies.
β-galactosidase
β-galactosidase is a hydrolase enzyme encoded by the LacZ gene of Escherichia coli, and has been applied to convert a colorless substrate, eg, Blue-gal or X-gal, into a blue reaction product. Various cells transfected with the LacZ gene or isolated from LacZ-transgenic rodents have been visualized histochemically after their infusion into the host.Citation126,Citation170,Citation171 However, the β-gal gene as a label is problematic because many mammalian cells demonstrate endogenous β-gal activity. While there are reports about the difference between bacterial and mammalian β-gal activity, it has been challenging for LacZ gene users to overcome the recipient tissue background problem.
Alkaline phosphatase
Alkaline phosphatase (AP) is a hydrolytic enzyme (EC 3.1.3.1) that catalyzes the nonspecific transestrification of phosphoryl ester optimally in alkaline environment. In in vitro research, E. coli AP gene (phoA) is commonly used as a reporter gene fused with gene of interest. Its product is naturally secreted to culture medium and can be easily detected by colorimetric and luminescent methods.Citation172 Moreover, it may work as sensor of subcellular localization of particular proteins.Citation173 The research with murine AP injected into the tail vein of mouse shows that this enzyme causes no immunogenic responses, which is a huge advantage in in vivo studies.Citation174 Currently secreted AP (SEAP) originating from human placenta is broadly used. The removal of some of amino acids from the carboxy end of this protein inhibits the secretion process, and AP protein is accumulated inside the cell. Furthermore, modified AP is stable at high temperature contrary to the endogenous expressing enzyme which is produced naturally by some cells. Due to this feature, the researchers can dispose of nonspecific background signal from endogenous AP.Citation175 SEAP fused with continuously expressing protein was successfully used to identify postmortem the transplanted neural cells in central nervous system by immunohistochemistry and fluorescence-activated cell sorting. Moreover, this technique enables assessment of percentage of cells which survive in host body after transplantation ().Citation176
Table 2 Summary of existing techniques for postmortem identification of transplanted cells
Conclusion
Cell therapy is a rapidly growing field of medicine. Cell imaging dramatically increases our understanding of the therapeutic effects. There are a variety of options to visualize transplanted cells, with a major division into premortem (in vivo) and postmortem (ex vivo) techniques (). Noninvasive, premortem techniques that permit evaluation of the efficiency of transplantation and the migration of transplanted cells are essential for animal model studies and human clinical therapeutic approaches. Postmortem techniques allow to see the transplanted cells within the context of surrounding tissue in detail due to the possibility of imaging at high magnification. Driven by the urgent need, there has been continuous progress in cell labeling approaches, which we have presented in our review. This progress includes novel nucleotide analogs for postmortem stem cell identification, such as EdU; novel MRI reporter genes, such as MagA; novel MR contrast techniques, such as CEST; as well as entirely novel methods such as optoacoustic imaging. The breadth of options allows for fitting the cell labeling and detection methods to the specific needs of particular applications on the preclinical and clinical level, which will allow for a more comprehensive application of regenerative medicine.
Figure 5 The summary of pre- and postmortem labeling and identification techniques described in detail in text.
Note: Reproduced with permission from © I-Hsun Wu 2014.
Abbreviations: PET, positron emission tomography; BLI, bioluminescent imaging; MRI, magnetic resonance imaging; HSV1-TK, herpes simplex virus type-1 thymidine kinase; LRP, lysine-rich protein; MR, magnetic resonance; EdU, 5-ethynyl-2-deoxyuridine; FISH, fluorescence in situ hybridization; GFP, green fluorescent protein; DAPI, 4′6-diamidino-2-phenylindole; BrdU, 5-bromo-2′-deoxyuridine.
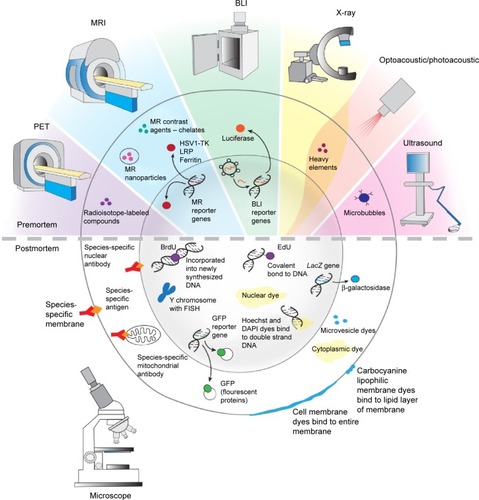
Acknowledgments
The study was supported by a National Centre for Research and Development grant number 101 in ERA-NET NEURON project: “MEMS-IRBI”, MSCRE-0178-00, R21 NS08154, R01 NS045062, R01 NS076573, and NMSS RG 4994-A-3. We would like to thank Mary McAllister for editorial assistance and I-Hsun Wu for her contribution to .
Disclosure
The authors report no conflicts of interest in this work.
References
- BhattacharyyaSDixitMMetallic radionuclides in the development of diagnostic and therapeutic radiopharmaceuticalsDalton Trans201140236112612821541393
- ZeglisMBHoughtonJLEvansMJViola-VillegasNLewisJSUnderscoring the influence of inorganic chemistry on nuclear imaging with radiometalsInorg Chem20145341880189924313747
- MathiasenABKastrupJNon-invasive in-vivo imaging of stem cells after transplantation in cardiovascular tissueTheranostics20133856157223946822
- KimMHWooaSKLeeKCLongitudinal monitoring adipose- derived stem cell survival by PET imaging hexadecyl-4-124I-iodobenzoate in rat myocardial infarction modelBiochem Biophys Res Commun20154561131925446095
- JanowskiMBulteJWWalczakPPersonalized nanomedicine advancements for stem cell trackingAdv Drug Deliv Rev201264131488150722820528
- BonnemaSJFastSHegedüsLThe role of radioiodine therapy in benign nodular goitreBest Pract Res Clin Endocrinol Metab201428461963125047210
- KhabbalJKerkeläEMitkariBDifferential clearance of rat and human bone marrow-derived mesenchymal stem cells from the brain after intra-arterial infusion in ratsCell Transplant201524581982824593908
- Barbosa da FonsecaLMBattistellaVde FreitasGREarly tissue distribution of bone marrow mononuclear cells after intra-arterial delivery in a patient with chronic strokeCirculation2009120653954119667245
- TarantalAFLeeCCKukisDLCherrySRRadiolabeling human peripheral blood stem cells for positron emission tomography (PET) imaging in young rhesus monkeysPLoS One2013810e7714824098579
- GaedickeaSBraunbFPrasadaSNoninvasive positron emission tomography and fluorescence imaging of CD133+ tumor stem cellsProc Natl Acad Sci U S A20141116E692E70124469819
- van der LelyAJde HerderWWKrenningEPKwekkeboomDJOctreoscan radioreceptor imagingEndocrine200320330731112721512
- TjuvajevJGStockhammerGDesaiRImaging the expression of transfected genes in vivoCancer Res19955524612661328521403
- QinCLanXHeJAn in vitro and in vivo evaluation of a reporter gene/probe system hERL/(18)F-FESPLoS One201384e6191123593502
- McCrackenMNGschwengEHNair-GillELong-term in vivo monitoring of mouse and human hematopoietic stem cell engraftment with a human positron emission tomography reporter geneProc Natl Acad Sci U S A201311051857186223319634
- GschwengEHMcCrackenMNKaufmanMLHSV-sr39TK positron emission tomography and suicide gene elimination of human hematopoietic stem cells and their progeny in humanized miceCancer Res201474185173518325038231
- VarmaNRBartonKNJanicBMonitoring adenoviral based gene delivery in rat glioma by molecular imagingWorld J Clin Oncol2013449110124926429
- GuoRZhangMXiYTheranostic studies of human sodium iodide symporter imaging and therapy using 188Re: a human glioma study in micePLoS One201497e10201125000403
- KnoopKSchwenkNDolpPStromal targeting of sodium iodide symporter using mesenchymal stem cells allows enhanced imaging and therapy of hepatocellular carcinomaHum Gene Ther201324330631623402366
- Hwang doWJangSJKimYHReal-time in vivo monitoring of viable stem cells implanted on biocompatible scaffoldsEur J Nucl Med Mol Imaging200835101887189818437378
- QuachCHJungKHPaikJYParkJWLeeEJLeeKHQuantification of early adipose-derived stem cell survival: comparison between sodium iodide symporter and enhanced green fluorescence protein imagingNucl Med Biol20123981251126022998839
- PantinJMHoytRFArasOOptimization of intrabone delivery of hematopoietic progenitor cells in a swine model using cell radiolabeling with [89]zirconiumAm J Transplant201515360661725656824
- LewisCMGravesASHernandezR52Mn production for PET/MRI tracking of human stem cells expressing divalent metal transporter 1 (DMT1)Theranostics20155322723925553111
- BarnettBPArepallyAStuberMArifinDRKraitchmanDLBulteJWSynthesis of magnetic resonance-, X-ray- and ultrasound-visible alg-inate microcapsules for immunoisolation and noninvasive imaging of cellular therapeuticsNat Protoc2011681142115121799484
- KedziorekDASolaiyappanMWalczakPUsing C-arm x-ray imaging to guide local reporter probe delivery for tracking stem cell engraftmentTheranostics201331191692624396502
- MohandasGOskolkovNMcMahonMTWalczakPJanowskiMPorous tantalum and tantalum oxide nanoparticles for regenerative medicineActa Neurobiol Exp (Wars)201474218819624993628
- CuiWTavriSBenchimolMJNeural progenitor cells labeling with microbubble contrast agent for ultrasound imaging in vivoBiomaterials201334214926493523578557
- TongJDingJShenXMesenchymal stem cell transplantation enhancement in myocardial infarction rat model under ultrasound combined with nitric oxide microbubblesPLoS One2013811e8018624244646
- KokhuisTJSkachkovINaaijkensBAIntravital microscopy of localized stem cell delivery using microbubbles and acoustic radiation forceBiotechnol Bioeng2015112122022725088405
- NejadnikHHenningTDDoTMR imaging features of gadofluorine-labeled matrix-associated stem cell implants in cartilage defectsPLoS One2012712e4997123251354
- BrekkeCWilliamsSCPriceJThorsenFModoMCellular multiparametric MRI of neural stem cell therapy in a rat glioma modelNeuroimage200737376978217613248
- CaoLLiBYiPThe interplay of T1- and T2-relaxation on T1-weighted MRI of hMSCs induced by Gd-DOTA-peptidesBiomaterials201435134168417424560458
- ShenYShaoYHeHGadolinium(3+)-doped mesoporous silica nanoparticles as a potential magnetic resonance tracer for monitoring the migration of stem cells in vivoInt J Nanomedicine2013811912723319863
- WangYXuCOwHCommercial nanoparticles for stem cell labeling and trackingTheranostics20133854456023946821
- IshibashiHHiraoKYamaguchiJInhibition of chloride outward transport by gadolinium in cultured rat spinal cord neuronsNeurotoxicology200930115515919007810
- AokiITakahashiYChuangKHCell labeling for magnetic resonance imaging with the T1 agent manganese chlorideNMR Biomed2006191505916411253
- YamadaMGurneyPTChungJManganese-guided cellular MRI of human embryonic stem cell and human bone marrow stromal cell viabilityMagn Reson Med20096241047105419526508
- ShinJAnisurRMKoMKImGHLeeJHLeeISHollow manganese oxide nanoparticles as multifunctional agents for magnetic resonance imaging and drug deliveryAngew Chem Int Ed Engl200948232132419040234
- KimTMominEChoiJMesoporous silica-coated hollow manganese oxide nanoparticles as positive T1 contrast agents for labeling and MRI tracking of adipose-derived mesenchymal stem cellsJ Am Chem Soc201113392955296121314118
- ChungJDashRKeeKTheranostic effect of serial manganese-enhanced magnetic resonance imaging of human embryonic stem cell derived teratomaMagn Reson Med201268259559922190225
- BulteJWKraitchmanDLIron oxide MR contrast agents for molecular and cellular imagingNMR Biomed200417748449915526347
- RieglerJLiewAHynesSOSuperparamagnetic iron oxide nano-particle targeting of MSCs in vascular injuryBiomaterials20133481987199423237516
- AndreasKGeorgievaRLadwigMHighly efficient magnetic stem cell labeling with citrate-coated superparamagnetic iron oxide nanoparticles for MRI trackingBiomaterials201233184515452522445482
- ChenXWZhuDJJuYLZhouSFTherapeutic effect of transplanting magnetically labeled bone marrow stromal stem cells in a liver injury rat model with 70%-hepatectomyMed Sci Monit20121810BR375BR38223018343
- AlbukhatySNaderi-ManeshHTiraihiTIn vitro labeling of neural stem cells with poly-L-lysine coated super paramagnetic nanoparticles for green fluorescent protein transfectionIran Biomed J2013172717623567848
- BakhruSHAltiokEHighleyCEnhanced cellular uptake and long-term retention of chitosan-modified iron-oxide nanoparticles for MRI-based cell trackingInt J Nanomedicine201274613462322942643
- KhuranaANejadnikHChapelinFFerumoxytol: a new, clinically applicable label for stem-cell tracking in arthritic joints with MRINanomedicine (Lond)20138121969198323534832
- GutovaMFrankJAD’ApuzzoMMagnetic resonance imaging tracking of ferumoxytol-labeled human neural stem cells: studies leading to clinical useStem Cells Transl Med201321076677524014682
- QinJLiKPengCMRI of iron oxide nanoparticle-labeled ADSCs in a model of hindlimb ischemiaBiomaterials201334214914492523535037
- AddicottBWillmanMRodriguezJMesenchymal stem cell labeling and in vitro MR characterization at 1.5 T of new SPIO contrast agent: Molday ION Rhodamine-B™Contrast Media Mol Imaging20116171820690161
- MengYZhangFBlairTMRI of auto-transplantation of bone marrow-derived stem-progenitor cells for potential repair of injured arteriesPLoS One201272e3113722363566
- JanowskiMWalczakPKropiwnickiTLong-term MRI cell tracking after intraventricular delivery in a patient with global cerebral ischemia and prospects for magnetic navigation of stem cells within the CSFPLoS One201492e9763124919061
- RooseDLerouxFDe VochtNMultimodal imaging of micron-sized iron oxide particles following in vitro and in vivo uptake by stem cells: down to the nanometer scaleContrast Media Mol Imaging20149640040824753446
- TarulliEChaudhuriJDGretkaVHoylesAMorsheadCMStaniszGJEffectiveness of micron-sized superparamagnetic iron oxide particles as markers for detection of migration of bone marrow-derived mesenchymal stromal cells in a stroke modelJ Magn Reson Imaging20133761409141823712844
- SchäferRKehlbachRMüllerMLabeling of human mesenchymal stromal cells with superparamagnetic iron oxide leads to a decrease in migration capacity and colony formation abilityCytotherapy2009111687819191056
- BulteJWIn vivo MRI cell tracking: clinical studiesAJR Am J Roentgenol2009193231432519620426
- AhrensETBulteJWTracking immune cells in vivo using magnetic resonance imagingNat Rev Immunol2013131075576324013185
- Ruiz-CabelloJBarnettBPBottomleyPABulteJWFluorine (19F) MRS and MRI in biomedicineNMR Biomed201124211412920842758
- RibotEJGaudetJMChenYGilbertKMFosterPJIn vivo MR detection of fluorine-labeled human MSC using the bSSFP sequenceInt J Nanomedicine201491731173924748787
- BengtssonNEBrownGScottEWWalterGAlacZ as a genetic reporter for real-time MRIMagn Reson Med201063374575320146234
- CampanMLionettiVAquaroGDFerritin as a reporter gene for in vivo tracking of stem cells by 1.5-T cardiac MRI in a rat model of myocardial infarctionAm J Physiol Heart Circ Physiol20113006H2238H225021335465
- IordanovaBAhrensETIn vivo magnetic resonance imaging of ferritin-based reporter visualizes native neuroblast migrationNeuroimage20125921004101221939774
- DeansAEWadghiriYZBernasLMYuXRuttBKTurnbullDHCellular MRI contrast via coexpression of transferrin receptor and ferritinMagn Reson Med2006561515916724301
- GoldhawkDELemaireCMcCrearyCRMagnetic resonance imaging of cells overexpressing MagA, an endogenous contrast agent for live cell imagingMol Imaging20098312913919723470
- ChungJKeeKBarralJKIn vivo molecular MRI of cell survival and teratoma formation following embryonic stem cell transplantation into the injured murine myocardiumMagn Reson Med20116651374138121604295
- DuynJHKoretskyAPNovel frontiers in ultra-structural and molecular MRI of the brainCurr Opin Neurol201124438639321734576
- AlfkeHStöpplerHNockenFIn vitro MR imaging of regulated gene expressionRadiology2003228248849212801999
- ZurkiyaOChanAWHuXMagA is sufficient for producing magnetic nanoparticles in mammalian cells, making it an MRI reporterMagn Reson Med20085961225123118506784
- GenoveGDeMarcoUXuHGoinsWFAhrensETA new transgene reporter for in vivo magnetic resonance imagingNat Med200511445045415778721
- CohenBDafniHMeirGHarmelinANeemanMFerritin as an endogenous MRI reporter for noninvasive imaging of gene expression in C6 glioma tumorsNeoplasia20057210911715802016
- LouieAYHüberMMAhrensETIn vivo visualization of gene expression using magnetic resonance imagingNat Biotechnol200018332132510700150
- KodibagkarVDYuJLiuLHetheringtonHPMasonRPImaging β-galactosidase activity using 19F chemical shift imaging of LacZ gene-reporter molecule 2-fluoro-4-nitrophenol-beta-D-galactopyranosideMagn Reson Imaging200624795996216916713
- WardKMAletrasAHBalabanRSA new class of contrast agents for MRI based on proton chemical exchange dependent saturation transfer (CEST)J Magn Reson20001431798710698648
- van ZijlPCYadavNNChemical exchange saturation transfer (CEST): what is in a name and what isn’t?Magn Reson Med201165492794821337419
- SherryADWoodsMChemical exchange saturation transfer contrast agents for magnetic resonance imagingAnnu Rev Biomed Eng20081039141118647117
- McMahonMTGiladAADeLisoMABermanSMBulteJWvan ZijlPCNew “multicolor” polypeptide diamagnetic chemical exchange saturation transfer (DIACEST) contrast agents for MRIMagn Reson Med200860480381218816830
- LiuGQinQChanKWNon-invasive temperature mapping using temperature-responsive water saturation shift referencing (T-WASSR) MRINMR Biomed201427332033124395616
- WuYSoesbeTCKieferGEZhaoPSherryADA responsive europium(III) chelate that provides a direct readout of pH by MRIJ Am Chem Soc201013240140021400320853833
- ChanKWLiuGSongXMRI-detectable pH nanosensors incorporated into hydrogels for in vivo sensing of transplanted-cell viabilityNat Mater201312326827523353626
- YooBPagelMDA PARACEST MRI contrast agent to detect enzyme activityJ Am Chem Soc200612843140321403317061878
- LiuGLiangYBar-ShirAMonitoring enzyme activity using a diamagnetic chemical exchange saturation transfer magnetic resonance imaging contrast agentJ Am Chem Soc201113341163261632921919523
- HingoraniDVRandtkeEAPagelMDA catalyCEST MRI contrast agent that detects the enzyme-catalyzed creation of a covalent bondJ Am Chem Soc2013135176396639823601132
- TrokowskiRRenJKálmánFKSherryADSelective sensing of zinc ions with a PARACEST contrast agentAngew Chem Int Ed Engl200544426920692316206314
- HarisMCaiKSinghAHariharanHReddyRIn vivo mapping of brain myo-inositolNeuroimage20115432079208520951217
- van ZijlPCJonesCKRenJMalloyCRSherryADMRI detection of glycogen in vivo by using chemical exchange saturation transfer imaging (glycoCEST)Proc Natl Acad Sci U S A2007104114359436417360529
- ChanKWMcMahonMTKatoYNatural D-glucose as a biodegradable MRI contrast agent for detecting cancerMagn Reson Med20126861764177323074027
- Walker-SamuelSRamasawmyRTorrealdeaFIn vivo imaging of glucose uptake and metabolism in tumorsNat Med20131981067107223832090
- LingWRegatteRRNavonGJerschowAAssessment of glycosaminoglycan concentration in vivo by chemical exchange-dependent saturation transfer (gagCEST)Proc Natl Acad Sci U S A200810572266227018268341
- GiladAAMcMahonMTWalczakPArtificial reporter gene providing MRI contrast based on proton exchangeNat Biotechnol200725221721917259977
- AiranRDBar-ShirALiuGMRI biosensor for protein kinase A encoded by a single synthetic geneMagn Reson Med20126861919192323023588
- ChoiJKimKKimTMultimodal imaging of sustained drug release from 3-D poly(propylene fumarate) (PPF) scaffoldsJ Control Release2011156223924521763735
- JaminYEykynTRPoonESpringerCJRobinsonSPDetection of the prodrug-activating enzyme carboxypeptidase G2 activity with chemical exchange saturation transfer magnetic resonanceMol Imaging Biol201416215215723955100
- Bar-ShirALiuGLiangYTransforming thymidine into a magnetic resonance imaging probe for monitoring gene expressionJ Am Chem Soc201313541617162423289583
- Bar-ShirALiuGGreenbergMMBulteJWGiladAASynthesis of a probe for monitoring HSV1-tk reporter gene expression using chemical exchange saturation transfer MRINat Protoc20138122380239124177294
- AhnBParashuramaNPatelMNoninvasive reporter gene imaging of human Oct4 (Pluripotency) dynamics during the differentiation of embryonic stem cells in living subjectsMol Imaging Biol201416686587624845530
- LiangYÅgrenLLyczekAWalczakPBulteJWNeural progenitor cell survival in mouse brain can be improved by co-transplantation of helper cells expressing bFGF under doxycycline controlExp Neurol2013247737923570903
- LiangYWalczakPBulteJWComparison of red-shifted firefly luciferase Ppy RE9 and conventional Luc2 as bioluminescence imaging reporter genes for in vivo imaging of stem cellsJ Biomed Opt201217101600422352654
- LiangYWalczakPBulteJWThe survival of engrafted neural stem cells within hyaluronic acid hydrogelsBiomaterials201334225521552923623429
- BermanSCGalpoththawelaCGiladAABulteJWWalczakPLong-term MR cell tracking of neural stem cells grafted in immunocompetent versus immunodeficient mice reveals distinct differences in contrast between live and dead cellsMagn Reson Med201165256457420928883
- GorelikMJanowskiMGalpoththawelaCNoninvasive monitoring of immunosuppressive drug efficacy to prevent rejection of intracerebral glial precursor allograftsCell Transplant201221102149215722508097
- MurphyCTMoloneyGHallLJUse of bioluminescence imaging to track neutrophil migration and its inhibition in experimental colitisClin Exp Immunol2010162118819620718784
- HastingsJWChemistries and colors of bioluminescent reactions: a reviewGene199617315118707056
- LengLWangYHeNMolecular imaging for assessment of mesenchymal stem cells mediated breast cancer therapyBiomaterials201435195162517024685267
- RiceBWCableMDNelsonMBIn vivo imaging of light-emitting probesJ Biomed Opt20016443244011728202
- WeisslederRA clearer vision for in vivo imagingNat Biotechnol200119431631711283581
- EvansMSChauretteJPAdamsSTJrA synthetic luciferin improves bioluminescence imaging in live miceNat Methods201411439339524509630
- HuangRViderJSerganovaIBlasbergRGATP binding cassette transporters modulate both coelenterazine- and D-luciferinbased bioluminescence imagingMol Imaging201110321522621496450
- NtziachristosVRipollJWangLVWeisslederRLooking and listening to light: the evolution of whole-body photonic imagingNat Biotechnol200523331332015765087
- LewandrowskiGKMageeCNMounayarMTannousBAAzziJSimultaneous in vivo monitoring of regulatory and effector T lymphocytes using secreted gaussia luciferase, firefly luciferase, and secreted alkaline phosphataseBadrCEBioluminescent Imaging: Methods and Protocols, Methods in Molecular BiologyNew York, NYSpringer2014211227
- JanowskiMEngelsCGorelikMSurvival of neural progenitors allografted into the CNS of immunocompetent recipients is highly dependent on transplantation siteCell Transplant201423225326223294627
- YaoJWangLVPhotoacoustic microscopyLaser Photon Rev201375758778
- Deán-BenXLRazanskyDPortable spherical array probe for volumetric real-time optoacoustic imaging at centimeter-scale depthsOpt Express20132123280622807124514320
- TserevelakisGJSolimanDOmarMNtziachristosVHybrid multiphoton and optoacoustic microscopeOpt Lett20143971819182224365806
- KneippMTurnerJHambauerSFunctional real-time optoa-coustic imaging of middle cerebral artery occlusion in micePLoS One201494e9611824776997
- iThera MedicalMSOT principle Available from: http://www.ithera-medical.com/technology/msot-principle.htmlAccessed January 1, 2015
- TaruttisARosenthalAKacprowiczMBurtonNCNtziachristosVMultiscale multispectral optoacoustic tomography by a stationary wavelet transform prior to unmixingIEEE Trans Med Imaging20143351194120224770922
- WangKSchoonoverRWSuROraevskyAAnastasioMADiscrete imaging models for three-dimensional optoacoustic tomography using radially symmetric expansion functionsIEEE Trans Med Imaging20143351180119324770921
- KusySContagCHReporter gene technologies for imaging cell fates in hematopoiesisMethods Mol Biol2014110912224473775
- ChengKChengZNear infrared receptor-targeted nanoprobes for early diagnosis of cancersCurr Med Chem201219284767478522873665
- DeliolanisNCAleAMorscherSDeep-tissue reporter-gene imaging with fluorescence and optoacoustic tomography: a performance overviewMol Imaging Biol201416565266024609633
- NamSYRiclesLMSuggsLJEmelianovSYIn vivo ultrasound and photoacoustic monitoring of mesenchymal stem cells labeled with gold nanotracersPLoS One201275e3726722615959
- LiopoAVConjusteauAChumakovaOVErmilovSASuROraevskyAAHighly purified biocompatible gold nanorods for contrasted optoacoustic imaging of small animal modelsNanosci Nanotechnol Lett20124768168623050035
- VonnemannJBeziereNBöttcherCPolyglycerolsulfate func-tionalized gold nanorods as optoacoustic signal nanoamplifiers for in vivo bioimaging of rheumatoid arthritisTheranostics20144662964124723984
- RiclesLMNamSYSokolovKEmelianovSYSuggsLJFunction of mesenchymal stem cells following loading of gold nanotracersInt J Nanomedicine2011640741621499430
- ZhangYSWangYWangLLabeling human mesenchymal stem cells with gold nanocages for in vitro and in vivo tracking by two-photon microscopy and photoacoustic microscopyTheranostics20133853254323946820
- FitzpatrickKMRaschkeJEmborgMECell-based therapies for Parkinson’s disease: past, present, and futureAntioxid Redox Signal20091192189220819485712
- FuocoCSalvatoriMLBiondoAInjectable polyethylene glycol-fibrinogen hydrogel adjuvant improves survival and differentiation of transplanted mesoangioblasts in acute and chronic skeletal-muscle degenerationSkelet Muscle2012212423181356
- HattiangadyBShettyAKNeural stem cell grafting counteracts hippocampal injury-mediated impairments in mood, memory, and neurogenesisStem Cells Transl Med20121969670823197876
- CoyneTMMarcusAJWoodburyDBlackIBMarrow stromal cells transplanted to the adult brain are rejected by an inflammatory response and transfer donor labels to host neurons and gliaStem Cells200624112483249216873764
- LinGHuangYCShindelAWLabeling and tracking of mesenchymal stromal cells with EdUCytotherapy200911786487319903099
- LinCSXinZCDaiJLueTFCommonly used mesenchymal stem cell markers and tracking labels: limitations and challengesHistol Histopathol20132891109111623588700
- NingHAlbersenMLinGLueTFLinCSEffects of EdU labeling on mesenchymal stem cellsCytotherapy2013151576323260086
- LinGNingHBanieLBone marrow cells stained by azide-conjugated Alexa fluors in the absence of an alkyne labelStem Cells Dev201221132552255922380729
- WalczakPChenNEveDHudsonJLong-term cultured human umbilical cord neural-like cells transplanted into the striatum of NOD SCID miceBrain Res Bull2007741–315516317683802
- LiPZhangRSunHPKH26 can transfer to host cells in vitro and vivoStem Cells Dev201322234034422913652
- HovakimyanMMüllerJWreeAOrtinauSRolfsASchmittOSurvival of transplanted human neural stem cell line (ReNcell VM) into the rat brain with and without immunosuppressionAnn Anat2012194542943522683000
- BaeSHKongTHLeeHSLong-lasting paracrine effects of human cord blood cells on damaged neocortex in an animal model of cerebral palsyCell Transplant201221112497251522524897
- ZhangCSaatmanKERoyoNCDelayed transplantation of human neurons following brain injury in rats: a long-term graft survival and behavior studyJ Neurotrauma200522121456147416379583
- CrainBJTranSDMezeyETransplanted human bone marrow cells generate new brain cellsJ Neurol Sci20052331–212112315949500
- BonillaCZuritaMOteroLAguayoCVaqueroJDelayed intralesional transplantation of bone marrow stromal cells increases endogenous neurogenesis and promotes functional recovery after severe traumatic brain injuryBrain Inj200923976076919637001
- MezeyEKeySVogelsangGSzalayovaILangeGDCrainBTransplanted bone marrow generates new neurons in human brainsProc Natl Acad Sci U S A200310031364136912538864
- AmariglioNHirshbergAScheithauerBWDonor-derived brain tumor following neural stem cell transplantation in an ataxia telangiectasia patientPLoS Med200962e100002919226183
- TranSDRedmanRSBarrettAJMicrochimerism in salivary glands after blood- and marrow-derived stem cell transplantationBiol Blood Marrow Transplant201117342943320940057
- VergheseEJohnsonCBertramJFRicardoSDDeaneJAThe fate of bone marrow-derived cells carrying a polycystic kidney disease mutation in the genetically normal kidneyBMC Nephrol2012139122931547
- ScheibeFLadhoffJHuckJImmune effects of mesenchymal stromal cells in experimental strokeJ Cereb Blood Flow Metab20123281578158822549620
- PolzerHVolkmerESallerMMLong-term detection of fluorescently labeled human mesenchymal stem cell in vitro and in vivo by semi-automated microscopyTissue Eng Part C Methods201218215616521951128
- PanHLanJLuoXGaoJXieXGuoHBiologic properties of gadolinium diethylenetriaminepentaacetic acid-labeled and PKH26-labeled human umbilical cord mesenchymal stromal cellsCytotherapy2014161748323867163
- ProgatzkyFDallmanMJLo CelsoCFrom seeing to believing: labelling strategies for in vivo cell-tracking experimentsInterface Focus2013332013000123853708
- FierabracciADel FattoreALucianoRMuracaMTetiAMuracaMRecent advances in mesenchymal stem cell immunomodulation. The role of microvesiclesCell Transplant201524213314924268069
- AdamsGBAlleyIRChungUIHaematopoietic stem cells depend on Galpha(s)-mediated signalling to engraft bone marrowNature2009459724310310719322176
- XuLMahairakiVKoliatsosVEHost induction by transplanted neural stem cells in the spinal cord: further evidence for an adult spinal cord neurogenic nicheRegen Med20127678579723164079
- DarkazalliALevensonCWTracking stem cell migration and survival in brain injury: current approaches and future prospectsHistol Histopathol201227101255126122936444
- FerrariAHannoucheDOudinaKIn vivo tracking of bone marrow fibroblasts with fluorescent carbocyanine dyeJ Biomed Mater Res200156336136711372053
- LassaillyFGriessingerEBonnetD“Microenvironmental contaminations” induced by fluorescent lipophilic dyes used for noninvasive in vitro and in vivo cell trackingBlood2010115265347535420215639
- PawelczykEJordanEKBalakumaranAIn vivo transfer of intracellular labels from locally implanted bone marrow stromal cells to resident tissue macrophagesPLoS One200948e671219696933
- SchormannWHammersenFJBrulportMTracking of human cells in miceHistochem Cell Biol2008130232933818425526
- MarkiewiczISypeckaJDomanska-JanikKWyszomirskiTLukomskaBCellular environment directs differentiation of human umbilical cord blood-derived neural stem cells in vitroJ Histochem Cytochem201159328930121378283
- JablonskaAJanowskiMLukomskaBDifferent methods of immunosuppresion do not prolong the survival of human cord blood-derived neural stem cells transplanted into focal brain-injured immunocompetent ratsActa Neurobiol Exp (Wars)20137318810123595286
- CellotSKroslJChagraouiJMelocheSHumphriesRKSauvageauGSustained in vitro trigger of self-renewal divisions in Hoxb4hiPbx1(10) hematopoietic stem cellsExp Hematol200735580281617577929
- KatoKCuiSKuickRIdentification of stem cell transcriptional programs normally expressed in embryonic and neural stem cells in alloreactive CD8+ T cells mediating graft-versus-host diseaseBiol Blood Marrow Transplant201016675177120116439
- CastanheiraPTorquettiLTMagalhãsDRNehemyMBGoesAMDAPI diffusion after intravitreal injection of mesenchymal stem cells in the injured retina of ratsCell Transplant200918442343119622229
- LiuNDeguchiKYamashitaTLiuWIkedaYAbeKIntracerebral transplantation of bone marrow stromal cells ameliorates tissue plasminogen activator-induced brain damage after cerebral ischemia in mice detected by in vivo and ex vivo optical imagingJ Neurosci Res201290112086209322791305
- MurakamiTKobayashiEGFP-transgenic animals for in vivo imaging: rats, rabbits, and pigsMethods Mol Biol201287217718922700411
- SinghVJainiRTorricelliAATuohyVKWilsonSEA method to generate enhanced GFP+ chimeric mice to study the role of bone marrow-derived cells in the eyeExp Eye Res201311636637024140502
- SwensonESPriceJGBrazeltonTKrauseDSLimitations of green fluorescent protein as a cell lineage markerStem Cells200725102593260017615263
- HartingMTJimenezFCoxCSJrIsolation of mesenchymal stem cells (MSCs) from green fluorescent protein positive (GFP+) transgenic rodents: the grass is not always green(er)Stem Cells Dev200918112713518518666
- De VochtNPraetJReekmansKTackling the physiological barriers for successful mesenchymal stem cell transplantation into the central nervous systemStem Cell Res Ther20134410123998480
- CzernikMFidanzaASardiMDifferentiation potential and GFP labeling of sheep bone marrow-derived mesenchymal stem cellsJ Cell Biochem2013114113414322886939
- ObokataHSasaiYNiwaHBidirectional developmental potential in reprogrammed cells with acquired pluripotencyNature2014505748567668024476891
- HeimRPrasherDCTsienRYWavelength mutations and posttrans-lational autoxidation of green fluorescent proteinProc Natl Acad Sci U S A1994912612501125047809066
- KoppHGHooperATShmelkovSVRafiiSβ-galactosidase staining on bone marrow. The osteoclast pitfallHistol Histopathol200722997197617523074
- KomatsuKHonmouOSuzukiJHoukinKHamadaHKocsisJDTherapeutic time window of mesenchymal stem cells derived from bone marrow after cerebral ischemiaBrain Res20101334849220382136
- LewisRELiaoGYoungKDouglasCKontoyiannisDPMacrophage reporter cell assay for screening immunopharmacological activity of cell wall-active antifungalsAntimicrob Agents Chemother20145831738174324395226
- ManoilCMekalanosJJBeckwithJAlkaline phosphatase fusions: sensors of subcellular locationJ Bacteriol199017225155182404939
- MaelandsmoGMRossPJPavlivMUse of a murine secreted alkaline phosphatase as a non-immunogenic reporter gene in miceJ Gene Med20057330731515515146
- SchenbornEGroskreutzDReporter gene vectors and assaysMol Biotechnol1999131294410934520
- MujtabaTHanSSFischerISandgrenEPRaoMSStable expression of the alkaline phosphatase marker gene by neural cells in culture and after transplantation into the CNS using cells derived from a transgenic ratExp Neurol20021741485711869033