Abstract
Purpose
Immune checkpoint inhibitors (ICIs)-related myocarditis is now one of the most critical immune-related adverse effects (irAEs) in tumor immunotherapy, which has raised great concern in cardio-oncology. The pathogenesis involved in cardiac injury remains elusive. Crocin, the main component of saffron, has shown distinct functions in cardioprotective and anti-inflammation properties. We therefore aimed to investigate the potential effect of crocin on the protection of ICIs-related myocarditis and its underlying molecular mechanism.
Methods
We immunized the BALB/c mice with murine cardiac troponin I (cTnI) peptide and additionally gave anti-mouse programmed death 1 (PD-1) to induce the mouse model of ICIs-related myocarditis. Mice were treated with crocin at different dosages. In vitro, HL-1 cells were pre-incubated with crocin at different concentrations and then stimulated with lipopolysaccharide (LPS). Myocardial contractile functions, myocardial inflammation and fibrosis, and myocardial injury were assessed. The expressions of pyroptosis-related proteins and nuclear factor-κB (NF-κB) pathway were evaluated.
Results
Crocin treatment could partially reverse the ICIs-related myocarditis in terms of improving heart function, ameliorating inflammation and fibrosis in the myocardium, and alleviating myocardial injury. Mechanistically, ICIs administration significantly activated pyrin domain-containing protein 3 (NLRP3) inflammasome in cardiomyocytes. Crocin treatments significantly downregulated the expression of NLRP3, cleaved gasdermin D (GSDMD), cleaved caspase1, interleukin-1β (IL-1β), and IL-18. Besides, crocin inhibited the activation of NF-κB pathway, which performed as reducing the phosphorylation of p-NF-kappa-B inhibitor-α (p-IκBα), degradation of IκBα, phosphorylation of p65 and p65 DNA binding activity both in vivo and in vitro.
Conclusion
By reversing the pyroptosis in cardiomyocytes, crocin treatment in a mouse model exerted great potential to aid in the prevention of ICIs-related myocarditis from a novel target.
Graphical Abstract
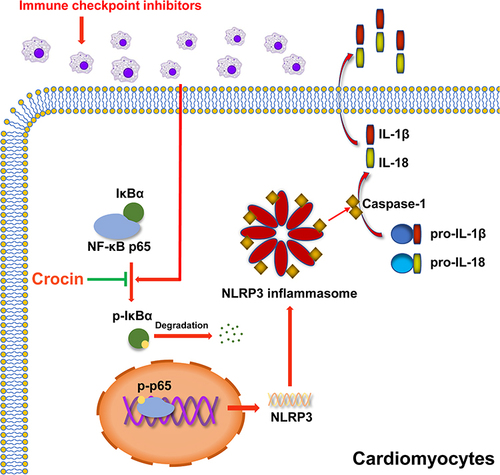
Introduction
In the past decade, the rise and development of immune checkpoint inhibitors (ICIs) have brought great clinical advances in cancer immunotherapy.Citation1,Citation2 ICIs target different immune checkpoints including the programmed death 1 (PD-1) such as Nivolumab and Pembrolizumab, programmed death ligand 1 (PD-L1) like Atezolizumab, Avelumab, and Durvalumab, and cytotoxic T-lymphocyte-associated antigen 4 (CTLA-4) inhibitors like Tremelimumab and Ipilimumab.Citation3 Since immune checkpoints also play a critical role in autoimmune tolerance, the activation of the immune system by ICIs may cause a wide range of autoimmune responses, which is called immune-related adverse effects (irAEs), including colitis, hepatitis, pneumonitis, thyroiditis, myositis, hypophysitis, and dermatitis.Citation4–6 These irAEs are largely reversible and can typically be controlled with the administration of glucocorticoid therapy.Citation5–7 However, severe cardiovascular effects, especially myocarditis and fatal heart failure caused by ICIs, have largely been underestimated in the past few years, which has raised great concern in cardio-oncology.Citation8
In 2018, a multicenter clinical study showed that the incidence of ICIs-related myocarditis was 1.14%.Citation9 In addition, the onset of ICIs-related myocarditis could be as early as 2 weeks after ICIs initiated,Citation10 the median time from symptom onset to death was only 32 days, and the incidence of fatality even reaches 46%.Citation11 The combination ICIs therapy such as a CTLA-4 inhibitor combined with a PD-1 inhibitor significantly increased the incidence and fatality of ICIs-related myocarditis compared to the ICIs monotherapy.Citation11 Currently, the treatment strategies for ICIs-related myocarditis are mainly for preventing further cardiotoxicity, immunosuppression to alleviate inflammatory changes, and supportive therapy.Citation12 However, no targeted drugs for the prevention and treatment of ICIs-related myocarditis have been developed and used.Citation13
The pyrin domain-containing protein 3 (NLRP3) inflammasome as a sensor for pathogen-derived danger signals in the innate immune system mediates caspase-1 activation, which cleaves gasdermin D (GSDMD) and promotes the secretion of proinflammatory cytokines interleukin-1β (IL-1β)/IL-18, leading to the lytic, pro-inflammatory form of cell death, pyroptosis.Citation14–17 Recently, many studies have shown that pyroptosis was involved in several non-inflammatory diseases, such as cryopyrin-associated periodic syndromes (CAPS), Alzheimer’s disease, diabetes, gout, autoinflammatory diseases, and atherosclerosis,Citation18,Citation19 which raised the possibility that anti-inflammasomes therapy may broaden its applications in diverse disease. In addition, NLRP3 has been shown to be activated in response to PD-1 blockade of CD8+ T cells in tumor cells and primary cardiomyocytes.Citation20,Citation21 Although the mechanism of ICIs-related myocarditis was still unclear, it might be a promising therapeutic target to inhibit pyroptosis and help alleviate the ICIs-related myocarditis.
Crocin is a major apo-carotenoid of saffron,Citation22 which has been shown great potential in anti-oxidant, anti-hypertensive, anti-depressant, cardioprotective, and nephron-protective properties, as well as the marked anti-inflammation properties.Citation23–26 Crocin was indicated not only to combat reactive oxygen species (ROS) production and inhibit pro-inflammatory cytokines secretion but also to suppress inflammation via regulating mainly nuclear factor-κB (NF-κB) pathway or phosphoinositide 3-kinase (PI3K)/Akt pathway in many animal models.Citation23,Citation27 However, the role of crocin in the protection of ICIs-related myocarditis was still unknown.
In this study, we investigated the protective effects and mechanisms of crocin against ICIs-related myocarditis in a mouse model. Moreover, we highlighted the effects of crocin in inhibiting the NLRP3 mediated pyroptosis via NF-κB pathway in vivo and in vitro. Our research provided a targeted therapeutic option for the treatment of ICIs-related myocarditis.
Materials and Methods
Materials
Crocin was a gift from Reyoung Pharmaceutical Co., Ltd. (Shanghai, China). InVivoMab Anti-mouse PD-1 was purchased from Bioxcell Co., Ltd. (West Lebanon, USA). Murine cardiac troponin I (TnI) peptide HARVDKVDEERYDVEAKVTKNITEIADLTQKIYDLRGKFKRPTLRRVRIS was synthesized by Sangon Biotech Co., Ltd. (Shanghai, China) according to the literature before.Citation28
Animals
Male 6-week-old BALB/c mice weighing 20–25 g were purchased from Vital River Laboratories (Shanghai, China) and housed in a standard rising condition of controlled humidity and a 12-h day/night cycle at 22°C. Animal studies were informed in compliance with the “Guide for the Care and Use of Laboratory Animals published by the US NIH (NIH publication No. 85-23, revised 2011)” and approved by the Animal Experiment Ethics Committee of Zhongshan Hospital of Fudan University (2019-109).
ICIs-Related Myocarditis Model and Animal Treatment
Except for the control group, all mice were immunized subcutaneously on day 0 and day 7, respectively, with a solution of 250ug murine cardiac TnI peptide (Sangon Biotech, China) diluted in complete Freund’s adjuvant (Sigma, USA) as described. From day 7 onwards, mice were given an intraperitoneal injection of anti-mouse PD-1 every 2 days at a dose of 5 mg/kg for 5 times to induce ICIs-related myocarditis.
BALB/c mice were randomly assigned to four groups (n = 10 in each group): (1) control group (CON, vehicle-treated group); (2) ICIs-related myocarditis group (ICIs, model group); (3) ICIs-related myocarditis + low-crocin (Crocin-L, crocin i.p. 20mg/kg/d); (4) ICIs-related myocarditis + high-crocin (Crocin-H, crocin i.p. 50mg/kg/d). Crocin was given from day 8 for 14 consecutive days. On day 21, echocardiography was implemented, and heart tissues were obtained from mice euthanized using deep isoflurane (5%) anesthesia, rinsed in ice-cold phosphate buffer saline, and snap-frozen in liquid nitrogen. The experimental design paradigm is shown in
Figure 1 Effect of crocin on ICIs-related myocarditis in myocardial contractile function. (A) BALB/c mice were randomly assigned to four groups (n=10 in each group): (1) control group (CON, vehicle-treated group); (2) ICIs-related myocarditis group (ICIs, model group); (3) ICIs-related myocarditis + low-crocin (Crocin-L, crocin i.p. 20mg/kg/d); (4) ICIs-related myocarditis + high-crocin (Crocin-H, crocin i.p. 50mg/kg/d). Except for control group, all mice were immunized subcutaneously on day 0 and day 7, respectively with murine cardiac TnI peptide. From day 7 onwards, mice were given anti-mouse PD-1 every 2 days for 5 times. Crocin was given from day 8 for 14 consecutive days. On day 21 since the first immunization, mice were killed after the implementation of echocardiography, and the heart tissues were obtained. (B) Representative images of thoracic echocardiography. (C and D) Quantifications of ejection fraction (EF) and fraction shortening (FS). (E–H) Quantifications of systolic left ventricular anterior wall (LVAW; s), diastolic left ventricular anterior wall (LVAW; d), and systolic left ventricular posterior wall (LVPW; s) and diastolic left ventricular posterior wall (LVPW; d). The data were expressed as mean ± SD. *p < 0.05 and ***p< 0.001.
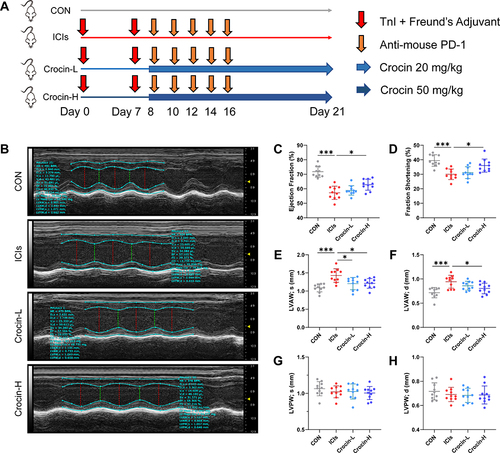
Echocardiography
Transthoracic echocardiography was performed using a 30-MHz high-frequency scan probe (Vevo 2100, VisualSonics, Canada) on day 21 in all animals. Mice were anesthetized and maintained in 2–3% isoflurane and 2 L/min 100% oxygen during the procedure. Left ventricular ejection fraction (LVEF), fractional shortening (FS), left ventricular anterior wall (LVAW) and left ventricular posterior wall (LVPW) were measured as previously described. All the measurements were performed by an investigator who was blinded to the experimental groups and the data was evaluated using Vevo Analysis software.
Histological Analysis
The heart tissue was fixed with 4% paraformaldehyde and embedded in paraffin. The paraffin-embedded hearts were then sectioned and stained with hematoxylin–eosin (HE), Masson trichrome staining, and Sirius Red staining reagent according to the manufacturer’s instructions (Servicebio Biotech, China). The images were observed and captured with a light microscope (LM, BX51, Olympus, Japan) and processed with Image J software.
Serum Markers of Myocardial Injury
The components of serum markers including the creatine kinase (CK), creatine kinase-MB (CK-MB) and lactate dehydrogenase-1 (LDH-1) were determined using commercial assay kits (Nanjing Jiancheng BioTech, China) according to the manufacturer’s instructions. The serum levels of cTnI and cTnT were quantified using commercial assays kits (Elabscience Biotechnology, China) according to the manufacturer’s instructions.
Enzyme-Linked Immunosorbent Assay (ELISA)
IL-1β and IL-18 levels in mouse serum and cell culture supernates were quantified using ELISA kits (R&D Systems, USA) according to the manufacturer’s instructions.
Cell Treatment
HL-1 mouse cardiomyocytes were purchased from Type Culture Collection of the Chinese Academy of Sciences (Shanghai, China) and cultured in Dulbecco’s modified Eagle’s medium (DMEM) (Hyclone, USA) with 10% fetal bovine serum (FBS) (Gibco, USA) and 1% penicillin–streptomycin solution (P/S) (Hyclone, USA) at 37°C in a humidified incubator containing 95% air and 5% CO2. Cells were seeded on a 6-well plate or a 96-well plate 24 hours before experiments. For crocin treatment, cells were pre-incubated with crocin at different concentrations (0, 5, 10, 20, 50μM) for 30 minutes and then stimulated with lipopolysaccharide (LPS) (Sigma, USA) at 1μg/mL for 24 hours to evaluate the protective effect of crocin in the NLRP3 mediated pyroptosis and its mechanism.
Cell Viability
HL-1 cells were seeded at a concentration of 5000 cells/well in a 96-well plate. Different treatments were implemented as described above. The cell viabilities of each group were detected using the Cell Counting Kit-8 (CCK-8, Beyotime Biotechnology, China) for measuring the absorbance values at a wavelength of 450 nm 1 hour after adding the working solution.
Western Immunoblot
Weighed heart tissue and HL-1 cells were homogenized in an ice-cold radioimmunoprecipitation assay (RIPA) lysis buffer with cocktail protease inhibitor (Beyotime Biotechnology, China) and then centrifuged at 12,000 rpm for 15 minutes at 4°C. The supernatant protein concentration was measured by the bicinchoninic acid method (Beyotime Biotechnology, China). Then, 20μg protein was size-fractionated by sodium dodecyl sulfate-polyacrylamide gel electrophoresis (SDS-PAGE) and transferred onto immobilon polyvinylidene difluoride membranes (Bio-Rad, USA). Membranes were cut into different parts according to the molecular weight of each protein and blocked with 5% nonfat milk (BD Biosciences, USA) for 2 hours. Each membrane was incubated with primary antibody at 4°C overnight: anti-NLRP3 (1:1000, Abcam, UK), anti-pro Caspase-1 + p10 + p12 (p10 and p12 are two kinds of cleaved Caspase-1) (1:1000, Abcam, UK), anti-Gasdermin D (1:1000, Cell Signaling Technology, USA), anti-cleaved Gasdermin D (1:1000, Cell Signaling Technology, USA), anti-NF-κB p65 (1:1000, Cell Signaling Technology, USA), anti-p-p65 (1:1000, Cell Signaling Technology, USA), anti-p-IκBα (1:1000, Cell Signaling Technology, USA), anti-IκBα (1:1000, Cell Signaling Technology, USA), anti-IL-1β (1:1000, Cell Signaling Technology, USA), anti-IL-18 (1:1000, ABclonal, China), anti-GAPDH (1:5000, Proteintech, USA). The membranes were thoroughly washed for the second day and incubated for 1 hour in the dark with secondary antibodies, DylightTM800 4XPEG-conjugated goat anti-rabbit IgG (H + L) or goat anti-mouse IgG (H + L) (1:10,000, Cell Signaling Technology, USA). The proteins were visualized and analyzed using the Odyssey Infrared Imaging System.
Immunofluorescent Staining
Slides of heart sections were deparaffinized, rehydrated, and boiled in Tris-EDTA solution (Beyotime Biotechnology, China) at 95°C for 20 min for antigen retrieval. In addition, the HL-1 cells were plated on slides, given different treatments as before, and fixed with 4% paraformaldehyde. Then, all slides were blocked with 5% goat serum and incubated with primary antibody at 4°C overnight: anti-NLRP3 (1:50, Abcam, UK), anti-cardiac Troponin T (cTnT, 1:200, Abcam, UK), anti-NF-κB p65 (1:100, Cell Signaling Technology, USA). The slides were rewarmed at 37°C for 1 hour, washed thoroughly and incubated with anti-rabbit IgG (1:1000, Alexa Fluro®594 Conjugate, Cell Signaling Technology) and anti-mouse IgG (1:1000, Alexa Fluro®488 Conjugate, Cell Signaling Technology) at 37°C for 1 hour. Nuclei were counterstained with DAPI. Finally, the stained sections were observed with a fluorescence microscope (Nikon, Japan, objective lens magnification of ×40; eyepiece magnification of ×10), and the fluorescence intensity of NLRP3 or nuclei NF-κB p65 was processed with Image J software.
Transcription Activity of NF-κB P65
Nuclear extracts from hearts and HL-1 cells were obtained by a nuclear extraction kit (Beyotime Biotechnology, China) according to the manufacturer’s protocol. The DNA-binding activity of NF-κB p65 in nuclear extracts was analyzed by using TransAM™ NF-κB p65 Colorimetric kits (Active Motif, USA) in duplicate and detected at a wavelength of 450 nm by a microplate spectrofluorometer (Bio-Tek, USA) as described.
Statistical Analysis
Continuous variables were presented as mean ± SD with at least three independent experiments. The two-tailed t-test was used to analyze the differences between the 2 groups. And, for comparisons among multiple groups, a one-way ANOVA followed by a Bonferroni post hoc test was used. P values <0.05 were considered to be statistically significant. Statistical analyses were performed using SPSS 22.0 software package.
Results
Effect of Crocin on ICIs-Related Myocarditis in Myocardial Contractile Function
The therapeutic potential of crocin in ICIs-related myocarditis was investigated in a mouse model as described above. On day 21, the left ventricular function and the thickness of the anterior and posterior walls of the left ventricle were assessed by echocardiography (). The results showed that the left ventricular ejection fraction and fraction shortening were significantly reduced in the ICIs group, compared to the control group. Administration of crocin in the high-dose group rather than crocin in the low-dose group significantly improved the left ventricular function ( and ). The thickness of the anterior walls of the left ventricle but not the posterior walls was significantly higher in the ICIs group than in the control group, which was reduced in both the Crocin-L group and Crocin-H group ().
Effect of Crocin on ICIs-Related Myocarditis in Myocardial Inflammation and Fibrosis
HE staining showed the inflammatory manifestations caused by ICIs (). In the ICIs group, the myocardial tissues were induced to a variable extent of inflammatory infiltration, ranging from 25% to 40%, compared to the control group, which received complete Freund’s adjuvant injections without TnI peptide and anti-mouse PD-1. The inflammatory infiltration was significantly reduced in both Crocin-L group and Crocin-H group (). Consistently, myocardial fibrosis and collagen deposition detected by Masson trichrome and Sirius Red staining were shown in the ICIs group, whereas the fibrotic scar formation was significantly reduced by crocin in a dose-dependent manner ( and ).
Figure 2 Effect of crocin on ICIs-related myocarditis in myocardial inflammation, fibrosis, and myocardial injury. Representative images of HE staining (A), Masson staining (B) and Sirius Red staining (C) of inflammatory manifestations, myocardial fibrosis and collagen deposition in heart sections. Scale bar = 100 μM. (D–F) Quantifications of the inflammatory area, fibrosis area and collagen volume fraction. (G–K) Serum levels of creatine kinase (CK), creatine kinase-MB (CK-MB), lactate dehydrogenase-1 (LDH-1), cardiac troponin T (cTnT) and cardiac troponin I (cTnI). The data were expressed as mean ± SD. *p < 0.05, **p < 0.01 and ***p< 0.001.
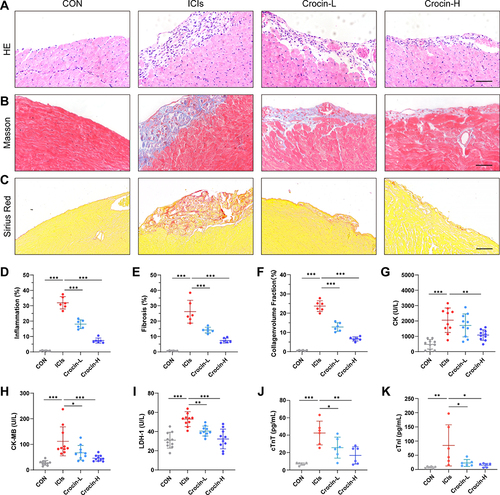
Effect of Crocin on ICIs-Related Myocarditis in Myocardial Injury
Further, the serum levels of creatine kinase (CK), creatine kinase-MB (CK-MB), and lactate dehydrogenase-1 (LDH-1) were detected to evaluate the myocardial injury. As are shown in , the induction of ICIs-related myocarditis resulted in a substantial rise in CK, CK-MB, and LDH-1. Administration with crocin of 20 mg/kg/d partially reversed the level of CK-MB and LDH-1, while crocin in 50 mg/kg/d significantly reduced the level of CK, CK-MB, and LDH-1. Besides, serum cTnT and cTnI were also quantified to evaluate the cardiac injury. Consistently, crocin treatments significantly reversed high levels of cTnT and cTnI caused by ICIs ( and ).
Effect of Crocin on ICIs-Related Myocarditis in NLRP3 Mediated Pyroptosis
In order to verify the role of NLRP3 mediated pyroptosis in ICIs-related myocarditis and identify the potential effect of crocin in reversing the pyroptosis, the heart tissue of the mice was extracted. Western blot showed that ICIs significantly upregulated the expression of NLRP3, cleaved Caspase 1, cleaved GSDMD, IL-1β and IL-18, which were significantly reversed in both crocin treated groups (). Meanwhile, the double immunofluorescent staining showed that red-stained NLRP3 positive signals were more intense in the ICIs group, which were located in the green-stained cTnT positive cardiomyocytes. This phenomenon was significantly ameliorated in the Crocin-L group and Crocin-H group ( and ).
Figure 3 Effect of crocin on ICIs-related myocarditis in NLRP3 mediated pyroptosis. (A–F) Representative images and quantitative analysis of Western blotting analysis of pyroptosis-related proteins (NLRP3, GSDMD, Cleaved GSDMD, Caspase 1, Cleaved Caspase1, interleukin-1β (IL-1β) and IL-18. (G) Representative images of double-stained immunofluorescence of NLRP3 (red) and cardiac troponin T (cTnT) (green) in heart sections. The nuclei were stained with DAPI (blue). Scale bar = 100 μM. (H) Quantification of NLRP3 fluorescence intensity. (I and J) Serum levels of IL-1β, IL-18. The data were expressed as mean ± SD. *p < 0.05, **p < 0.01 and ***p< 0.001.
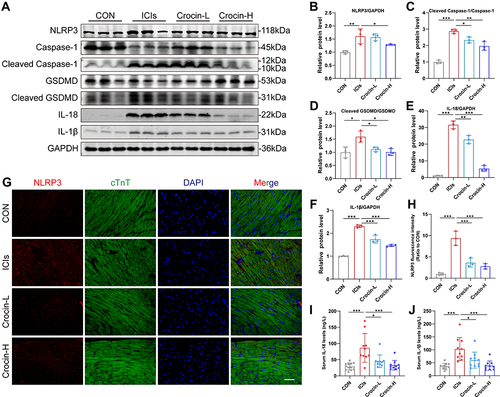
Moreover, the serum levels of IL-1β and IL-18 were significantly elevated in ICIs groups, which were processed as a result of caspase 1 activation. Treatment with crocin at both dosages significantly inhibited the productions of these cytokines consistently ( and ).
Effect of Crocin on LPS-Induced Pyroptosis in vitro
To further investigate the role of crocin in alleviating the pyroptosis in cardiomyocytes, HL-1 cell lines were used, and LPS was used to induce pyroptosis in vitro.Citation29,Citation30 The cell viabilities were detected by the CCK-8 kit. The results showed that LPS induced a significant decrease in cell viability, which was reversed by the pretreatment of crocin at the dose of 10μM, 20μM, and 50μM (). Western Blot showed that LPS induced a significant increase in the expression of NLRP3, cleaved Caspase 1, cleaved GSDMD, IL-1β and IL-18, compared to the control group. Pretreatment of crocin significantly reversed the effect by a dose-dependent reduction in NLRP3, cleaved Caspase 1, cleaved GSDMD, IL-1β and IL-18 expression (). Consistently, the levels of IL-1β and IL-18 in cell culture supernates were elevated by the stimulation of LPS, which were inhibited by the crocin pretreatments ( and ). Meanwhile, the immunofluorescent staining showed similar results that LPS induced stronger red-stained NLRP3 signals, which were significantly alleviated by crocin pretreatments ( and ).
Figure 4 Effect of crocin on LPS-induced pyroptosis in vitro. Lipopolysaccharide (LPS) was used to induce pyroptosis in vitro. HL-1 cells were pre-incubated with crocin at different concentrations (0, 5, 10, 20, 50μM) for 30 minutes and then stimulated with LPS at 1μg/mL for 24 hours to evaluate the protective effect of crocin in the NLRP3 mediated pyroptosis. (A) Cell viability was detected by CCK-8 cell viability assay. (B–G) Representative images and quantitative analysis of Western blotting analysis of pyroptosis-related proteins (NLRP3, GSDMD, Cleaved GSDMD, Caspase 1, Cleaved Caspase1, interleukin-1β (IL-1β) and IL-18). (H and I) Cell culture supernatant levels of IL-1β and IL-18. (J) Representative images of immunofluorescence of NLRP3 (red) in HL-1 cells. The nuclei were stained with DAPI (blue). Scale bar = 100 μM. (K) Quantification of NLRP3 fluorescence intensity. The data were expressed as mean ± SD. *p < 0.05, **p < 0.01 and ***p< 0.001.
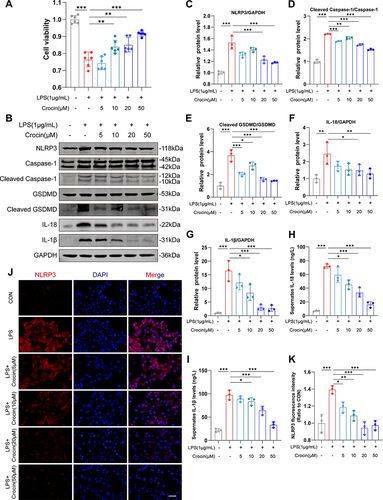
Effect of Crocin on NF-κB Pathway in vivo and in vitro
The activation of NF-κB pathway has been reported participating in the process of pyroptosis. Therefore, the role of crocin in regulating the NF-κB pathway in ICIs-related cardiomyocytes’ pyroptosis was accessed. As are shown in , the NF-κB pathway was activated in the ICIs groups, demonstrated by an increased level of p-p65 and p-IκBα, a decreased level of IκBα expression, as well as the upregulation of DNA binding activity of p65. The treatment of crocin at both dosages significantly inhibited the activation of NF-κB pathway. In agreement with the findings in vivo, the pretreatment of crocin significantly inhibited LPS-induced activation of NF-κB pathway in HL-1 cell lines, as characterized by reducing the phosphorylation of p65 and IκBα, the degradation of IκBα, and the level of p65 DNA binding activity (). Moreover, LPS stimulation significantly enhanced NF-κB p65 nuclear transfer performed as the red stained p65 positive in the nuclear, which was significantly alleviated by the pretreatments of crocin ( and ).
Figure 5 Effect of crocin on NFκB pathway in vivo and in vitro. (A–D) Representative images and quantitative analysis of Western blotting analysis of NFκB pathway (NFκB p65, p-p65, p-IκBα and IκBα) in mice myocardium after the administration of immunization, anti-PD-1, and crocin. (E) p65 DNA binding activity in heart nuclear extracts was measured. (F–I) Representative images and quantitative analysis of Western blotting analysis of NFκB pathway (NFκB p65, p-p65, p-IκBα and IκBα) in HL-1cells pretreated with crocin at different concentrations before LPS stimulation. (J) p65 DNA binding activity in HL-1 nuclear extracts was measured. (K) Representative images of immunofluorescence of NFκB p65 nuclear transfer (red) in HL-1 cells. The nuclei were stained with DAPI (blue). Scale bar = 50 μM. (L) Quantification of nuclei NFκB p65 fluorescence intensity. The data were expressed as mean ± SD. *p < 0.05, **p < 0.01 and ***p< 0.001.
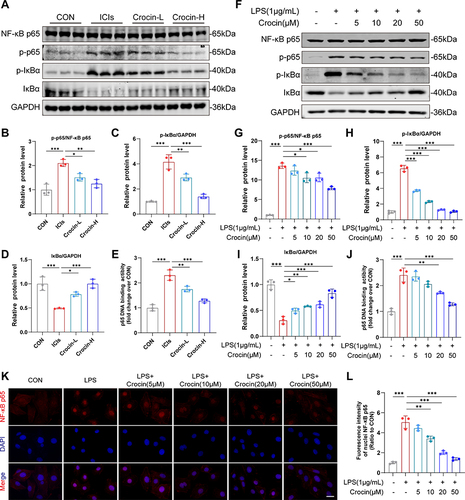
Discussion
The present study evaluated the phenotype and underlying mechanism of ICIs-related myocarditis in a mouse model. Crocin supplementation may alleviate ICIs-related myocarditis in terms of improving heart function, ameliorating inflammation, and inhibiting pyroptosis via NF-κB pathway. In addition, we verified the protective effect of crocin against NLRP3 mediated pyroptosis in vitro. Collectively, we supposed that crocin would be a potential therapeutic agent in preventing ICIs-related myocarditis by inhibiting the pyroptosis in cardiomyocytes.
In view of the fact that ICIs are widely used in the treatment of tumors, the occurrence of irAEs has gradually attracted attention. The American Society of Clinical Oncology (ASCO) developed the guideline for the management of irAEs, which separated myocarditis into 4 grades of severity, with grade 1 being the mildest. Concerned about the high mortality of ICIs-related myocarditis, it is recommended to hold the ICIs even for grade 1 toxicity and high-dose corticosteroids should be administered rapidly for all grades.Citation31,Citation32 As the indications of ICIs continue to expand, further research is needed for the identification of ICIs-related myocarditis, especially in its mechanism and treatments.
The mechanism of ICIs-related myocarditis was mostly reported to be related to autoimmune disorders caused by excessive T cell activation.Citation33–35 Prior studies demonstrated that fatal myocarditis mediated by T cells and myeloid cells was shown in PD-1 deficient MRL mice, which had an autoimmune tendency, while CTLA-4 deficient mice also showed similar early lethal lymphocytes proliferative disease.Citation34,Citation36 Cardiac troponin I was considered to be the specific cardiac antigens, leading to the development of autoimmune cardiotoxicity.Citation28 Therefore, we immunized mice with cTnI, and additionally gave anti-mouse PD-1 to simulate the clinical ICIs-related myocarditis. Here we found the impaired myocardial contractile function, the inflammatory manifestations, the myocardial fibrosis, and the increased level of myocardial enzyme profile in the model group, which provided a new mouse model for mechanism exploration and drug screening in ICIs-related myocarditis.
A recent study showed that anti-PD1 therapy disrupted immune homeostasis and induced dysregulated metabolism, exhibiting profound detrimental effects on cardiac integrity.Citation37 In addition, some scholars believed that common antigens in tissues could be recognized by T cells, and the increased levels of interferon-γ (IFN-γ), granzyme B, and tumor necrosis factor-α (TNF-α) produced by activated T cells could cause heart damage.Citation8 However, the direct association between ICIs-induced immune reactions and cardiac injury is still unknown. The previous study showed that PD-L1-dependent activation of the NLRP3 inflammasome in tumor tissues directly linked to CD8+ T cell activity by the recruitment of granulocytic myeloid-derived suppressor cells (PMN-MDSCs) to the tumor bed in response to the anti-PD1 therapy.Citation20 Besides, the AC16 human cardiomyocytes co-cultured with human peripheral blood mononuclear cells (hPBMCs) treated with ipilimumab manifested the overexpression of NLRP3/MyD88 and cytokines, which was likely a result of the lymphocytic infiltration of lymphocytes.Citation21 Similarly, the present study indicated that NLRP3 inflammasome was significantly activated in cardiomyocytes of the entire heart tissue by the ICIs treatment, not necessarily in the inflammation area. Therefore, we hypothesized that the excessive activation of T cells response to ICIs, upregulating the autoimmune activity of the cardiomyocytes, which leads to myocardial pyroptosis widely.
Immunosuppressive therapy is preferred as a treatment option for ICIs-related myocarditis, but it can counteract the anti-cancer effects as well.Citation13 Other treatments such as the blockade of TNF-α and the blockade of Janus kinase (JAK) signals have also been reported to limit changes in cardiac immunity.Citation37,Citation38 Our study found that crocin, as a derivative from traditional Chinese herbs, was able to alleviate the phenotype of ICIs-related myocarditis in mice via inhibiting the NLRP3 mediated pyroptosis in cardiomyocytes. Crocin is now widely used as a cardioprotective agent. It has been suggested to attenuate isoprenaline-induced myocardial fibrosis by targeting toll-like receptor 4 (TLR4)/NF-κB signaling.Citation27 In addition, crocin alleviated the LPS-induced toxicity in H9c2 cells via reducing the levels of inflammatory factors.Citation39 In line with the previous findings, our results showed the protective effect of crocin in inhibiting the activation of the NLRP3 inflammasome both in vivo and in vitro.
Mechanically, the traditional NF-κB pathway was widely reported as the first signal of the activation of NLRP3 inflammasome.Citation40–42 IκBα, a member of the NF-κB inhibitor family, was degraded by ubiquitin-dependent proteasomes, allowing p65 phosphorylation and transferred to the nucleus,Citation43 which upregulated the expression of NLRP3. In addition, NF-κB pathway exerted multiple therapeutic targets since its regulatory network ranged from cancers to inflammatory and immune disorders.Citation44–46 We found that crocin effectively reversed the activation of the NF-κB pathway both in vivo and in vitro, suggesting that NF-κB signaling was involved in the regulation of crocin in pyroptosis. Further, how crocin inhibited the activation of the NF-κB pathway might appear more complicated. Physiologically, NF-κB pathway was required for adaptive changes in gene expression and tissue homeostasis, which was responsive to oxidative stress, DNA damage, immune activation and growth regulatory signals.Citation43 Interestingly, crocin was reported to physically bind to various cellular proteins, such as structural proteins, membrane transporters, and enzymes, which are involved in adenosine triphosphate (ATP) synthesis, redox homeostasis, and signal transduction.Citation47 Substantial evidence showed that crocin reduced ROS generation and provided a potential explanation.Citation48,Citation49 A recent study suggested that toll-like receptor (TLR) 4 signaling might be a target for crocin to inhibit the activation of the NF-κB pathway, which still requires more validation.Citation27 Considering this, our findings indicated the potential effect of crocin on inhibiting NLRP3 mediated pyroptosis via regulating the NF-κB pathway in ICIs-related myocarditis.
Limitation
However, there were still some limitations in the current study. First, the mechanism of how the excessive T cells induced the activation of NLRP3 inflammasome needs further verification. Secondly, the co-culture of ICIs-treated PBMCs and cardiomyocytes was lacking, although we used LPS to stimulate the pyroptosis of cardiomyocytes. Finally, we initially evaluated the potential use of crocin in ameliorating the ICIs-related myocarditis, and its role in cancer and other irAEs still needs further exploration.
Conclusion
In summary, our study provided evidence that NLRP3 inflammasome was strongly activated in cardiomyocytes, which played a role in the disrupted immune homeostasis of the heart in ICIs therapy. Crocin treatment could partially ameliorate ICIs-related myocarditis and contributed to the suppression of pyroptosis by regulating NF-κB pathway. Our findings may provide a novel therapeutic agent to treat ICIs-induced cardiac dysfunction.
Disclosure
The authors report no conflicts of interest in this work.
Acknowledgments
This study was supported by the National Natural Science Foundation of China (No. 82170359, 81771840), Clinical Research Fund of Zhongshan Hospital (2020ZSLC21), and Smart medical treatment project of Zhongshan Hospital (2020ZHZS16).
References
- Bagchi S, Yuan R, Engleman EG. Immune checkpoint inhibitors for the treatment of cancer: clinical impact and mechanisms of response and resistance. Annu Rev Pathol. 2021;16:223–249. doi:10.1146/annurev-pathol-042020-042741
- Singh S, Hassan D, Aldawsari HM, Molugulu N, Shukla R, Kesharwani P. Immune checkpoint inhibitors: a promising anticancer therapy. Drug Discov Today. 2020;25(1):223–229. doi:10.1016/j.drudis.2019.11.003
- Ribas A, Wolchok JD. Cancer immunotherapy using checkpoint blockade. Science. 2018;359(6382):1350–1355. doi:10.1126/science.aar4060
- Wang DY, Salem JE, Cohen JV, et al. Fatal toxic effects associated with immune checkpoint inhibitors. JAMA Oncol. 2018;4(12):1721–1728. doi:10.1001/jamaoncol.2018.3923
- Johnson DB, Chandra S, Sosman JA. Immune checkpoint inhibitor toxicity in 2018. JAMA. 2018;320(16):1702–1703. doi:10.1001/jama.2018.13995
- Postow MA, Sidlow R, Hellmann MD. Immune-related adverse events associated with immune checkpoint blockade. N Engl J Med. 2018;378(2):158–168. doi:10.1056/NEJMra1703481
- Friedman CF, Proverbs-Singh TA, Postow MA. Treatment of the immune-related adverse effects of immune checkpoint inhibitors: a review. JAMA Oncol. 2016;2(10):1346–1353. doi:10.1001/jamaoncol.2016.1051
- Varricchi G, Galdiero MR, Marone G, et al. Cardiotoxicity of immune checkpoint inhibitors. ESMO Open. 2017;2(4):e000247. doi:10.1136/esmoopen-2017-000247
- Mahmood SS, Fradley MG, Cohen JV, et al. Myocarditis in patients treated with immune checkpoint inhibitors. J Am Coll Cardiol. 2018;71(16):1755–1764. doi:10.1016/j.jacc.2018.02.037
- Chen DY, Huang WK, Chien-Chia Wu V, et al. Cardiovascular toxicity of immune checkpoint inhibitors in cancer patients: a review when cardiology meets immuno-oncology. J Formos Med Assoc 2020;119(10):1461–1475. doi:10.1016/j.jfma.2019.07.025
- Johnson DB, Balko JM, Compton ML, et al. Fulminant myocarditis with combination immune checkpoint blockade. N Engl J Med. 2016;375(18):1749–1755. doi:10.1056/NEJMoa1609214
- Hu JR, Florido R, Lipson EJ, et al. Cardiovascular toxicities associated with immune checkpoint inhibitors. Cardiovasc Res. 2019;115(5):854–868. doi:10.1093/cvr/cvz026
- Salem JE, Allenbach Y, Vozy A, et al. Abatacept for severe immune checkpoint inhibitor-associated myocarditis. N Engl J Med. 2019;380(24):2377–2379. doi:10.1056/NEJMc1901677
- Lamkanfi M, Dixit VM. Mechanisms and functions of inflammasomes. Cell. 2014;157(5):1013–1022. doi:10.1016/j.cell.2014.04.007
- Liu X, Zhang Z, Ruan J, et al. Inflammasome-activated gasdermin D causes pyroptosis by forming membrane pores. Nature. 2016;535(7610):153–158. doi:10.1038/nature18629
- Martinon F, Burns K, Tschopp J. The inflammasome: a molecular platform triggering activation of inflammatory caspases and processing of proIL-beta. Mol Cell. 2002;10(2):417–426. doi:10.1016/S1097-2765(02)00599-3
- Shi J, Zhao Y, Wang K, et al. Cleavage of GSDMD by inflammatory caspases determines pyroptotic cell death. Nature. 2015;526(7575):660–665. doi:10.1038/nature15514
- Guo H, Callaway JB, Ting JPY. Inflammasomes: mechanism of action, role in disease, and therapeutics. Nat Med. 2015;21(7):677–687. doi:10.1038/nm.3893
- Menu P, Vince JE. The NLRP3 inflammasome in health and disease: the good, the bad and the ugly. Clin Exp Immunol. 2011;166(1):1–15. doi:10.1111/j.1365-2249.2011.04440.x
- Theivanthiran B, Evans KS, DeVito NC, et al. A tumor-intrinsic PD-L1/NLRP3 inflammasome signaling pathway drives resistance to anti-PD-1 immunotherapy. J Clin Invest. 2020;130(5):2570–2586. doi:10.1172/JCI133055
- Quagliariello V, De Laurentiis M, Cocco S, et al. NLRP3 as putative marker of ipilimumab-induced cardiotoxicity in the presence of hyperglycemia in estrogen-responsive and triple-negative breast cancer cells. Int J Mol Sci. 2020;21(20):7802. doi:10.3390/ijms21207802
- Bukhari SI, Manzoor M, Dhar MK. A comprehensive review of the pharmacological potential of Crocus sativus and its bioactive apocarotenoids. Biomed Pharmacother. 2018;98:733–745. doi:10.1016/j.biopha.2017.12.090
- Hashemzaei M, Mamoulakis C, Tsarouhas K, et al. Crocin: a fighter against inflammation and pain. Food Chem Toxicol. 2020;143:111521.
- Hashemzaei M, Rezaee R, Nabatzehi M, et al. Anti-hypertensive effect of crocin and hesperidin combination in high-fat diet treated rats. Exp Ther Med. 2020;19(6):3840–3844. doi:10.3892/etm.2020.8650
- Korani S, Korani M, Sathyapalan T, Sahebkar A. Therapeutic effects of Crocin in autoimmune diseases: a review. Bio Factors Oxf Engl. 2019;45(6):835–843. doi:10.1002/biof.1557
- Hatziagapiou K, Kakouri E, Lambrou GI, Bethanis K, Tarantilis PA. Antioxidant properties of Crocus sativus L. and its constituents and relevance to neurodegenerative diseases; focus on Alzheimer’s and Parkinson’s disease. Curr Neuropharmacol. 2019;17(4):377–402. doi:10.2174/1570159X16666180321095705
- Jin W, Zhang Y, Xue Y, et al. Crocin attenuates isoprenaline-induced myocardial fibrosis by targeting TLR4/NF-κB signaling: connecting oxidative stress, inflammation, and apoptosis. Naunyn Schmiedebergs Arch Pharmacol. 2020;393(1):13–23. doi:10.1007/s00210-019-01704-4
- Bockstahler M, Fischer A, Goetzke CC, et al. Heart-specific immune responses in an animal model of autoimmune-related myocarditis mitigated by an immunoproteasome inhibitor and genetic ablation. Circulation. 2020;141(23):1885–1902. doi:10.1161/CIRCULATIONAHA.119.043171
- Qiu Y, Ma Y, Jiang M, et al. Melatonin alleviates LPS-induced pyroptotic cell death in human stem cell-derived cardiomyocytes by activating autophagy. Stem Cells Int. 2021;2021:8120403. doi:10.1155/2021/8120403
- Qiu Z, He Y, Ming H, Lei S, Leng Y, Xia ZY. Lipopolysaccharide (LPS) aggravates high glucose- and hypoxia/reoxygenation-induced injury through activating ROS-dependent NLRP3 inflammasome-mediated pyroptosis in H9C2 cardiomyocytes. J Diabetes Res. 2019;2019:8151836. doi:10.1155/2019/8151836
- Brahmer JR, Lacchetti C, Schneider BJ, et al. Management of immune-related adverse events in patients treated with immune checkpoint inhibitor therapy: American society of clinical oncology clinical practice guideline. J Clin Oncol. 2018;36(17):1714–1768. doi:10.1200/JCO.2017.77.6385
- Palaskas N, Lopez‐Mattei J, Durand JB, Iliescu C, Deswal A. Immune checkpoint inhibitor myocarditis: pathophysiological characteristics, diagnosis, and treatment. J Am Heart Assoc. 2020;9(2):e013757.
- Ji C, Roy MD, Golas J, et al. Myocarditis in cynomolgus monkeys following treatment with immune checkpoint inhibitors. Clin Cancer Res. 2019;25(15):4735–4748. doi:10.1158/1078-0432.CCR-18-4083
- Wang J, Okazaki IM, Yoshida T, et al. PD-1 deficiency results in the development of fatal myocarditis in MRL mice. Int Immunol. 2010;22(6):443–452. doi:10.1093/intimm/dxq026
- Grabie N, Gotsman I, DaCosta R, et al. Endothelial programmed death-1 ligand 1 (PD-L1) regulates CD8+ T-cell mediated injury in the heart. Circulation. 2007;116(18):2062–2071. doi:10.1161/CIRCULATIONAHA.107.709360
- Waterhouse P, Penninger JM, Timms E, et al. Lymphoproliferative disorders with early lethality in mice deficient in ctla-4. Science. 1995;270(5238):985–988. doi:10.1126/science.270.5238.985
- Michel L, Helfrich I, Hendgen-Cotta UB, et al. Targeting early stages of cardiotoxicity from anti-PD1 immune checkpoint inhibitor therapy. Eur Heart J. 2021;43(4):ehab430.
- Liu X, Zhang X, Ye L, Yuan H. Protective mechanisms of berberine against experimental autoimmune myocarditis in a rat model. Biomed Pharmacother. 2016;79:222–230. doi:10.1016/j.biopha.2016.02.015
- Baradaran Rahim V, Khammar MT, Rakhshandeh H, Samzadeh-Kermani A, Hosseini A, Askari VR. Crocin protects cardiomyocytes against LPS-induced inflammation. Pharmacol Rep. 2019;71(6):1228–1234. doi:10.1016/j.pharep.2019.07.007
- Zhao W, Ma L, Cai C, Gong X. Caffeine Inhibits NLRP3 inflammasome activation by suppressing MAPK/NF-κB and A2aR signaling in LPS-induced THP-1 macrophages. Int J Biol Sci. 2019;15(8):1571–1581. doi:10.7150/ijbs.34211
- Boaru SG, Borkham-Kamphorst E, Van de Leur E, Lehnen E, Liedtke C, Weiskirchen R. NLRP3 inflammasome expression is driven by NF-κB in cultured hepatocytes. Biochem Biophys Res Commun. 2015;458(3):700–706. doi:10.1016/j.bbrc.2015.02.029
- Oeckinghaus A, Hayden MS, Ghosh S. Crosstalk in NF-κB signaling pathways. Nat Immunol. 2011;12(8):695–708. doi:10.1038/ni.2065
- Mitchell S, Vargas J, Hoffmann A. Signaling via the NFκB system. Wiley Interdiscip Rev Syst Biol Med. 2016;8(3):227–241. doi:10.1002/wsbm.1331
- Baud V, Karin M. Is NF-kappaB a good target for cancer therapy? Hopes and pitfalls. Nat Rev Drug Discov. 2009;8(1):33–40. doi:10.1038/nrd2781
- Cheong R, Hoffmann A, Levchenko A. Understanding NF-kappaB signaling via mathematical modeling. Mol Syst Biol. 2008;4:192. doi:10.1038/msb.2008.30
- Kearns JD, Hoffmann A. Integrating computational and biochemical studies to explore mechanisms in NF-κB Signaling. J Biol Chem. 2009;284(9):5439–5443. doi:10.1074/jbc.R800008200
- Hosseinzadeh H, Mehri S, Heshmati A, Ramezani M, Sahebkar A, Abnous K. Proteomic screening of molecular targets of crocin. DARU J Pharm Sci. 2014;22(1):5. doi:10.1186/2008-2231-22-5
- Ochiai T, Ohno S, Soeda S, Tanaka H, Shoyama Y, Shimeno H. Crocin prevents the death of rat pheochromyctoma (PC-12) cells by its antioxidant effects stronger than those of alpha-tocopherol. Neurosci Lett. 2004;362(1):61–64. doi:10.1016/j.neulet.2004.02.067
- Liang Y, Zheng B, Li J, et al. Crocin ameliorates arsenic trioxide‑induced cardiotoxicity via Keap1-Nrf2/HO-1 pathway: reducing oxidative stress, inflammation, and apoptosis. Biomed Pharmacother. 2020;131:110713. doi:10.1016/j.biopha.2020.110713