Abstract
Background
With the rapid growth of the elderly population, the incidence of osteoarthritis (OA) increases annually, which has attracted extensive attention in public health. The roles of dietary intake in controlling joint disorders are perhaps one of the most frequently posed questions by OA patients, while the information about the interaction between dietary intake and OA based on scientific research is limited. α-Chaconine is the richest glycoalkaloid in eggplants such as potatoes. Previous evidence suggests that α-Chaconine is a toxic compound to nervous and digestive systems with potentially severe and fatal consequences for humans and farm animals, but its effect on OA development remains obscure.
Objective
To determine whether α-Chaconine deteriorates OA progression through sensory innervation and chondrocyte pyroptosis via regulating nuclear factor-κB (NF-κB) signaling, providing evidence for a possible linkage between α-Chaconine and OA progression.
Methods
We established a mouse OA model by destabilization of medial meniscus (DMM) surgery and then intra-articular injection of 20 or 100 μM α-Chaconine into the OA mice for 8 and 12 weeks. The severity of OA progression was evaluated by histological staining and radiographic analyses. The expressions of matrix metabolic indicators, Col2, Mmp3, and Mmp13, as well as pyroptosis-related proteins, Nlrp3, Caspase-1, Gsdmd, IL-1β, IL-18, were determined by immunohistochemistry. And the changes in sensory nerve ingrowth and activity of NF-κB signaling were determined by immunofluorescence.
Results
We found that α-Chaconine could exacerbate mouse OA progression, resulting in subchondral sclerosis, osteophyte formation, and higher OARSI scores. Specifically, α-Chaconine could augment cartilage matrix degradation and induce chondrocyte pyroptosis and nerve ingrowth. Mechanistical analysis revealed that α-Chaconine stimulated NF-κB signaling by promoting I-κB α phosphorylation and p65 nuclear translocation.
Conclusion
Collectively, our findings raise the possibility that α-Chaconine intake can boost chondrocyte pyroptosis and nerve ingrowth to potentiate OA progression by activating NF-κB signaling.
Graphical Abstract
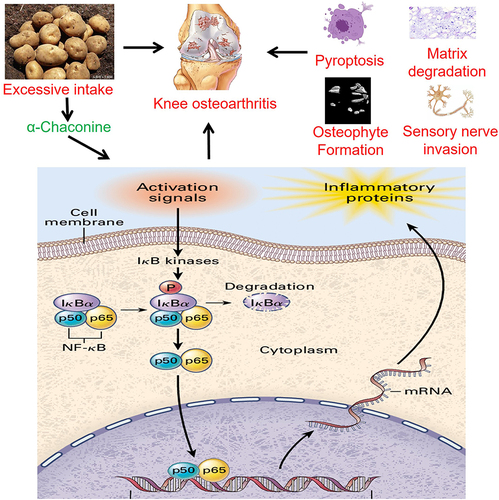
Introduction
Osteoarthritis (OA) is a major public health issue worldwide affecting 250 million people.Citation1,Citation2 Due to aging of the population, the prevalence of OA is continually increasing, leading to a great burden on health care system.Citation3 Dramatic increase in the prevalence of OA in developed nations over the past half-century provides important clues as to which contributors are responsible for OA development, with obesity, metabolic syndrome, dietary changes, dysbiotic gut microbiome, and physical inactivity as the main conspicuous candidates,Citation4,Citation5 strongly implicating those vicious contributors of OA may be derived from dietary intake. Questions regarding the role of dietary intake in controlling OA are perhaps one of the most frequently posed ones by OA patients, while the information about the interaction between dietary intake and OA based on scientific research remains limited.Citation6
Glycoalkaloids are present in many plants of the Solanaceae family (also known as nightshade plants), particularly in potatoes, and are considered part of the eggplant’s defense system against insects and pests.Citation7 α-Chaconine and α-solanine account for 95% of total glycoalkaloids, and the content of α-Chaconine in potato is generally slightly higher than that of α-solanine (the ratio of α-chaconine to α-solanine ranges from 1.2 to 2.6).Citation8 Increasing evidence suggests that glycoalkaloids have a variety of adverse as well as beneficial effects in cells, animals, and humans, which poses potential food safety concerns.Citation9 A significant positive correlation has been documented between serum glycoalkaloid levels and normal dietary intake of potatoes by the individual concerned.Citation7 And the accumulation of glycoalkaloids has been reported to be associated with OA-related swelling, pain and stiffness, whereas many OA patients do report symptom relief after the removal of potatoes from the diet.Citation10–12 Moreover, high levels (3–6 mg/kg body weight) intake of glycoalkaloids can lead to acute intoxication, concomitant with vomiting, diarrhea, internal haemorrhage and abdominal pain followed by fever, weak pulse, in severe cases coma and death.Citation13,Citation14 Based on the above results, we hypothesize that α-Chaconine may be responsible for the aggravation of OA progression in patients with potato intake.
Persistent pain becomes a dominant clinical symptom for OA patients and a major driver of medical consultation and health service use.Citation2 OA is a whole-joint disease, involving all structural changes of joint tissues, including articular cartilage, subchondral bone, synovial tissue, meniscus, ligaments and infrapatellar fat pad, etc.Citation15–17 An increasing body of evidence suggests that various pathogenesis associated with OA could worsen in combination, including cartilage degradation, subchondral bone sclerosis, nerve innervation, meniscal degeneration, synovial inflammation, inflammation and fibrosis of the infrapatellar fat pad.Citation18–22 As OA disease advances, ongoing inflammatory stimulation and progressive damage to local tissues may affect the afferent processing of nociceptive signals from joints and surrounding tissues, resulting in pain sensitivity.Citation23–25
Pyroptosis is a type of proinflammatory programmed cell death triggered by inflammation involving the activation of nod-like receptor protein-3 (NLRP3)/Caspase-1, which cleave precursors of IL-1β and IL-18 to promote their maturation and secretion.Citation26 Recent studies have shown that Nlrp3-mediated inflammasome contributes to chondrocyte pyroptosis and OA development through activation of nuclear factor-κB (NF-κB) signaling.Citation27 Besides, increasing evidence suggests that aberrant subchondral bone remodeling such as sensory nerve innervation contributes directly or indirectly to cartilage destruction, pain, and OA progression.Citation20,Citation28,Citation29 By using biochemical and genetic approaches, Zhu et al reported that, in the context of OA progression, osteoclasts secrete axonal guidance molecules, Netrin-1, to induce calcitonin gene–related peptide-positive (Cgrp+) sensory nerve axonal growth in subchondral bone; and specific ablation of Netrin-1 in osteoclasts reduces Cgrp+ sensory neurons in subchondral bone and alleviates OA pain behavior.Citation18
NF-κB family consists of five members: c-Rel, RelB, p52/p100, p50/p105, and RelA (p65).Citation30 Generally, p65 heterodimer is sequestered in cytoplasm in an inactive state complexed with inhibitor-κB (I-κBα). Once stimulated by inflammatory mediators or mechanical symbols, I-κB kinase phosphorylates IκBα at Ser32 and Ser36 followed by proteasome-mediated degradation. It is widely acknowledged that NF-κB signaling has been well affirmatory as the major calibrator of inflammatory responses in OA.Citation30,Citation31 Activation of NF-κB signaling leads to upregulation of matrix metalloproteinases (Mmps) and decrease of Collagen 2 (Col2), eventually contributing to extracellular matrix degradation and triggering chondrocyte pyroptosis during OA onset and progression.Citation32 Meanwhile, NF-κB signaling has been documented to regulate osteoclast formation, differentiation, and functions in subchondral bone as well as Netrin-1 expression.Citation33 Beyond that, gene-profiling experiments performed in glycoalkaloid-treated intestinal epithelial cell-line Caco-2 suggest that NF-κB signaling is significantly enriched by multiple targets induced by glycoalkaloid administration.Citation34 Accordingly, it is reasonable to speculate that NF-κB signaling may mediate the roles of α-Chaconie on OA pathogenesis.
The present study aimed to explore the potential relationship between α-Chaconine treatment and OA development, based on destabilization of medial meniscus (DMM) surgery-induced OA model, thereby providing deep insights into the effects of α-Chaconine intake on human and animal health.
Materials and Methods
Chemicals and Reagents
α-Chaconine powder (99.67% of purity) was purchased from ChromaDex (Irvine, CA, USA). A voucher specimen (ASB-00003371-005) has been deposited at the First Affiliated Hospital of Zhejiang Chinese Medical University. Primary antibodies against collagen 2 (Col2), matrix metallopeptidase 3 (Mmp3), matrix metallopeptidase 13 (Mmp13), Nlrp3, Caspase-1, Gasdermin D (Gsdmd), IL-1β, IL-18, and phospho-i-κB (p-i-κBα) were purchased from Ruiying Biological Co. (Jiangsu, China). Primary antibodies against p65 and phospho-p65 (p-p65) were purchased from Cell Signaling Technology (Beverly, MA, USA). Gsdmd, Cgrp, and Netrin-1 were purchased from Abcam (Cambridge, MA, USA). Fluorescent secondary antibodies were purchased from Sungene Biotech Co. (Tianjin, China). Unless otherwise mentioned, all chemicals were purchased from Sigma-Aldrich (St. Louis, MO, USA).
Animals and DMM-Induced OA Model
Eight-week-old male C57BL/6J mice (18–22 g) were provided by the animal experiment center of Zhejiang Chinese Medical University (Grade SPF, SCXK (Shanghai): 2017–0005). All mice were raised within a specific pathogen-free animal care facility and housed in a room at 23 ± 2℃ with a 12 h-12 h light/dark cycle, and mice had ad libitum access to water and lab chow. All mouse procedures were approved by the Ethics Committee on Animal Experimentation of Zhejiang Chinese Medical University (ZSLL-2018-012).
Experimental Design of Animal Studies
All mice were allowed to acclimatize for 1-week before surgery. Forty mice were randomly and equally divided into four groups (n = 10 in each group): Sham group (Sham), OA model group (Vehicle), low-dose α-Chaconine group (OA mice treated with low-dose α-Chaconine), and high-dose α-Chaconine group (OA mice treated with high-dose α-Chaconine). The experimental mice were subjected to DMM surgery-induced OA model as we previously described.Citation35 Mice in the low- and high-dose α-Chaconine groups were intra-articularly injected with 6 μL of α-Chaconine (20 and 100 μM, respectively) per right knee joint twice a week, starting from day 10 post-DMM surgery, while mice in the Sham group and vehicle group received the same volume of saline. The microinjection was performed with a 10 μL microinjector (Hamilton Company, Reno, NV, USA) and 33#-gauge needles (Hamilton Company). All mice were anesthetized with 1% pentobarbital sodium and sacrificed at 8- and 12-week post-DMM surgery, and all knee joints were collected for further analyses ().
Micro Computed Tomography (Micro-CT) Analysis
Before histologic processing, the knee joints were scanned using high-resolution micro-CT equipment (Skyscan1176, Bruker micro-CT N.V., Kontich, Belgium) at a voltage of 50 kV with a current of 500 μA and a resolution of 9 μm per pixel. Image reconstruction and quantitative morphologic analysis were achieved by using NRecon v1.6 and CTAn v1.15, respectively. Three-dimensional visualization images were reconstructed using 3D-creator software, CTVol v2.2. Coronal images of tibial subchondral bone were chosen to evaluate three-dimensional histomorphometric analyses. Bone volume/total volume (BV/TV), trabecular thickness (Tb.Th), and trabecular number (Tb.N, 1/mm) on subchondral bone medial compartment were calculated using X-Tek computational algorithms from 50 consecutive images.
Histology Staining
After fixation in 4% paraformaldehyde for 48 h, the knee joints were decalcified with 14% EDTA solution for 21 days, dehydrated by alcohol, embedded in paraffin, and sectioned at 4 μm. The sections were stained with Safranin O/Fast green. Cartilage destruction in the medial tibial plateau of the joint was scored by two blinded pathologists using the OARSI system, as previously described.Citation36 Osteophyte formation was evaluated semi-quantitatively using osteophyte formation scores, including osteophyte volume and osteophyte maturity.Citation37
Immunohistochemistry (IHC) and Immunofluorescence (IF) Analyses
IHC analysis was performed on 4 μm-thick sections using SP Link Detection Kits (ZSGB-BIO, Beijing, China), according to the manufacturer’s instructions. Briefly, sections were blocked with PBS containing 10% normal goat serum for 30 min, and subsequently incubated with primary antibodies, Col2 (diluted 1:1000), Mmp3 (diluted 1:100), Mmp13 (diluted 1:100), Nlrp3 (diluted 1:100), Caspase-1 (diluted 1:100), Gsdmd (diluted 1:100), IL-1β (diluted 1:100), IL-18 (diluted 1:100) at 4 ℃ overnight, respectively. The polymer-HRP labeled secondary antibody was added for 30 min the following day. The immunohistochemical reaction was visualized by incubation with 0.05% diaminobenzidine (DAB). Positive staining appeared in brown. Images were captured with a microscope (Carl Zeiss, Göttingen, Germany). For IF assay, sections were incubated with primary antibodies, including Cgrp (diluted 1:200), Netrin-1 (diluted 1:200), p-i-kbα (diluted 1:200), p65 (diluted 1:200) at 4℃ overnight, then treated with fluorescent-conjugated secondary-antibody (Sungene Biotech, Tianjin, China) for 30 min in the dark. After DAPI counterstaining, the images were captured with a fluorescence microscope (Carl Zeiss, Göttingen, Germany). The number of positive cells was quantified in a blinded manner with Image-Pro Plus 6.0 software (Media Cybernetics, Silver Spring, MD, USA) as we previously described.Citation38
Statistical Analysis
All numerical data were presented as mean ± SD. One-way analysis of variance (ANOVA) and post-hoc Tukey’s test were performed using GraphPad Prism 8 software (GraphPad Software Inc., La Jolla, CA). A P value of less than 0.05 was statistically significant.
Results
α-Chaconine Deteriorates OA Progression in DMM Surgery-Induced OA Mice
OA is an age-related degenerative joint disease characterized by progressive articular cartilage destruction, osteophyte formation, and subchondral bone sclerosis et al.Citation39 To investigate the effects of α-Chaconine on OA progression, mice that underwent DMM surgery were intra-articularly injected with two optimized concentrations of α-Chaconine (20 and 100 μM, concentrations used in the previous in vitro and in vivo studies of α-ChaconineCitation40–43) (). The morphological changes of knee joints were evaluated by Safranin O/Fast green staining, and we found that articular cartilage erosion was obviously observed in OA mice, and high-dose α-Chaconine could exacerbate the articular cartilage damage (). The mouse OA progression was further assessed by the OARSI score system, and we found that high-dose α-Chaconine treatment aggravated increased OARSI scores in DMM surgery-treated mice (). Moreover, the radiographic changes of knee joints were examined using micro-CT analysis. We found that high-dose α-Chaconine could significantly increase osteophyte maturity but have no effect on osteophyte volume (). Interestingly and intriguingly, compared with the OA mice, low-dose α-Chaconine could reduce increased BV/TV and Tb.Th in subchondral bone 8- and 12-week post-surgery, while high-dose α-Chaconine further enhanced the above indexes ( and ). In addition, high-dose α-Chaconine increased Tb.N in subchondral bone 8-week post-surgery, whereas low-dose α-Chaconine reduced Tb.N 12-week post-surgery (). Our findings suggest that only high-dose α-Chaconine may aggravate OA progression in DMM surgery-induced murine OA model.
Figure 2 α-Chaconine (α-Cha) deteriorates OA progression. (A) Safranin O/Fast green staining of cartilage. (B) OARSI scores for cartilage structure damage in (A). (C-C’) Representative three-dimensional images of knee joints. Three-dimensional reconstructions of knee joints in (C) and osteophytes (C’) were used to visualize calcification of meniscus and osteophyte formation 8- and 12-week after DMM surgery. Yellow triangles indicated osteophytes. (D–H) Statistical results of bone morphological parameters in (C). Data were expressed as the mean ± SD. *P < 0.05, **P < 0.01 vs Sham group, #P < 0.05, ##P < 0.01 vs Vehicle group.
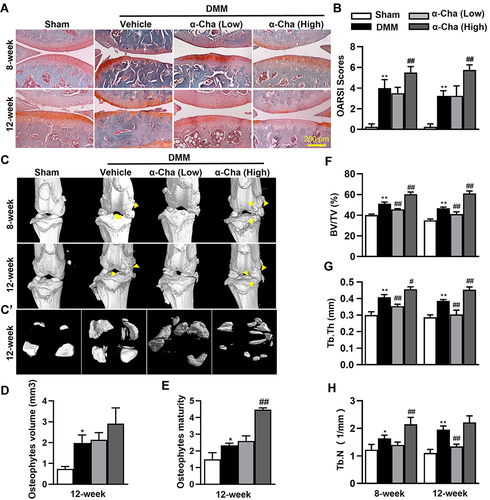
α-Chaconine Accelerates Articular Cartilage Matrix Degradation in OA Mice
Stable matrix content is crucial for maintaining the physiological function of knee joint.Citation44 To explore the effects of α-Chaconine on matrix metabolism of OA mice, we determined Col2, Mmp13, and Mmp3 expressions in articular cartilage by IHC analysis. The IHC results showed that, compared with Sham mice, lower Col2 and higher Mmp3 and Mmp13 expressions were observed in OA mice; and high-dose α-Chaconine could further exacerbate these alterations. Unexpectedly, low-dose α-Chaconine may partially attenuate Mmp13 expression in OA mice (). The above results suggest that high-dose α-Chaconine could accelerate matrix degradation in articular cartilage of OA mice.
Figure 3 α-Chaconine accelerates articular cartilage matrix degradation. (A–C) Immunohistochemistry results of Col2, Mmp3, and Mmp13 proteins. (D–F) The ratios of immunoreactive positive cells of Col2, Mmp3, and Mmp13 in (A–C). Data were expressed as the mean ± SD. **P < 0.01 vs Sham group, #P < 0.05, ##P < 0.01 vs Vehicle group.
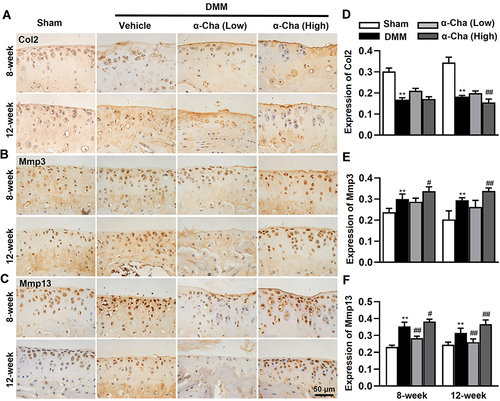
α-Chaconine Promotes Articular Chondrocytes Pyroptosis in OA Mice
Given the widely accepted role of IL-1β in OA pathology and the maturation of IL-1β by inflammasomes, the molecular mechanism for nod-like receptor protein-3 (NLRP3) inflammasome-mediated pyroptosis during OA progression has gained extensive attention.Citation35,Citation38 To identify whether α-Chaconine affects articular chondrocyte pyroptosis, we analyzed the expressions of key pyroptosis-related proteins, Nlrp3, Caspase-1, Gsdmd, IL-1β, and IL-18, in articular cartilage by IHC staining. Consistent with our previous findings,Citation35,Citation38 the expression levels of Nlrp3, Caspase-1, Gsdmd, IL-1β, and IL-18 were significantly increased in OA mice, and high-does α-Chaconine treatment could further accelerate their increases. Unexpectedly, low-does α-Chaconine treatment could downregulate these increases to a similar level to the Sham group, except Gsdmd (). The above findings indicate that α-Chaconine exposure aggravates chondrocyte pyroptosis-mediated inflammation.
Figure 4 α-Chaconine promotes chondrocyte pyroptosis. (A–E) Immunohistochemistry staining results of Nlrp3, Caspase-1, Gsdmd, IL-1β, and IL-18 proteins in articular cartilage 8- and 12-week post-DMM surgery. (F–J) The ratios of immunoreactive positive cells of Nlrp3, Caspase-1, Gsdmd, IL-1β, and IL-18 in (A–E). Data were expressed as the mean ± SD. **P < 0.01 vs Sham group, #P < 0.05, ##P < 0.01 vs Vehicle group.
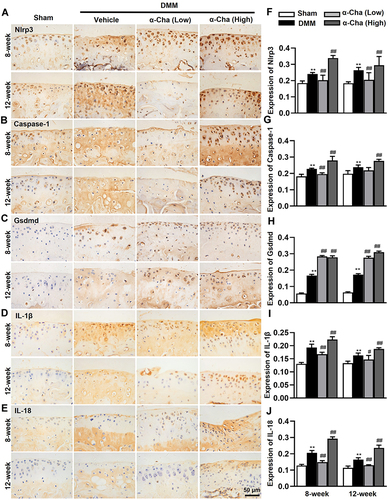
α-Chaconine Facilitates Nerve Ingrowth in OA Mice
A study carried out in the mouse OA model has demonstrated that secretion of Netrin-1 by osteoclasts is strongly associated with the recruitment of Cgrp+ sensory nerve innervation in subchondral bone and hypersensitivity to pain.Citation18 To evaluate whether α-Chaconine regulates sensory nerve ingrowth in subchondral bone, we examined expression changes in its specific marker, Cgrp, as well as Netrin-1 using IF analysis. Consistent with previous results,Citation20,Citation45 DMM surgery resulted in a significantly higher number of Cgrp+ neurons in cartilage and subchondral bone of OA mice ( and ). Notably, α-Chaconine administration substantially elevated Cgrp+ nerve cell ratio in subchondral bone of OA mice in a dose-dependent manner (). Similarly, an enhanced expression of Netrin-1 was found in cartilage and subchondral bone of DMM surgery-treated mice, and α-Chaconine-treated mice had a higher expression of Netrin-1 than OA mice ( and ). Based on the above results, we speculated that α-Chaconine could stimulate the sensitivity of OA-related pain, which may partly be due to the elevated secretion of Netrin-1 and its subsequent promotion of Cgrp+ sensory neuron recruitment.
Figure 5 α-Chaconine recruits sensory nerve ingrowth in subchondral bone. (A and B) Immunofluorescence staining of Cgrp and Netrin-1 in subchondral bone 8- and 12-Week post-surgery. (C and D) Quantification of Cgrp-positive cells and positive staining of Netrin-1 in cartilage. (E and F) Quantification of Cgrp-positive cells and positive staining of Netrin-1 in subchondral bone. Red arrows indicated Cgrp-positive neurons and positive expression of Netrin-1. Data were expressed as the mean ± SD. **P < 0.01 vs Sham group, #P < 0.05, ##P < 0.01 vs Vehicle group.
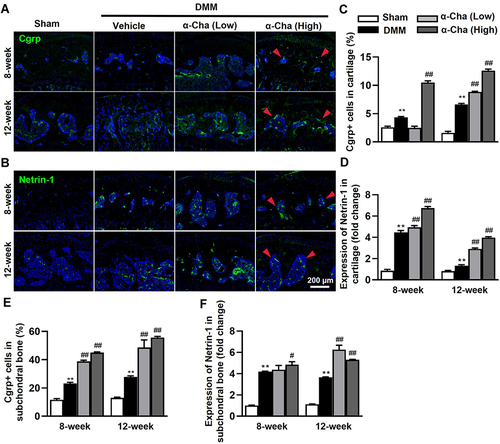
α-Chaconine Further Activates NF-κB Signaling in OA Mice
Subsequently, to clarify the molecular mechanism by which α-Chaconine-induced chondrocyte pyroptosis and sensory innervation, we examined NF-κB signaling activity by analyzing the expression of p65 as well as the phosphorylation status of I-κBα, p-i-κBα. As shown in and , we found that OA mice displayed a significant increase in nuclear translocation of p65 and p-i-κBα levels in articular cartilage and subchondral bone throughout OA modeling, while it was noteworthy that α-Chaconine dose-dependently augmented p-i-κBα and p65 levels (). Taken together, these results suggest that α-Chaconine might strengthen chondrocyte pyroptosis and sensory nerve ingrowth to exacerbate OA development by activating NF-κB activity ().
Figure 6 α-Chaconine activates NF-κB signaling pathway of knee joint. (A and B) Immunofluorescence staining of p65 and p-i-κBα both in articular cartilage and subchondral bone 8 and 12-week post-surgery. (C and D) Quantification of p65 and p-i-κBα positive cells in cartilage. (E and F) Quantification of p65 and p-i-κBα positive cells in subchondral bone. Red arrows indicate the positive expression of p65 and p-i-κBα. Data are expressed as the mean ± SD. **P < 0.01 vs Sham group, ##P < 0.01 vs Vehicle group.
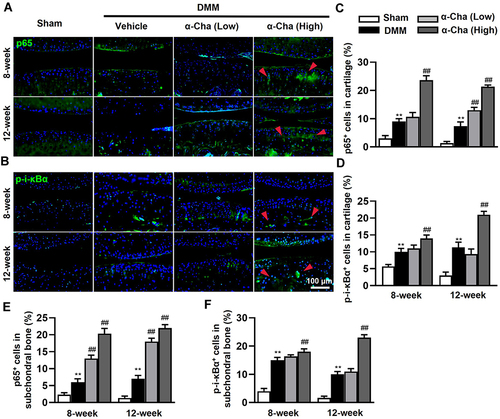
Discussion
Overwhelming evidence from studies in animal models and clinical patients confirms that high-level glycoalkaloids may have toxic effects on the health of humans and animals.Citation7,Citation9,Citation13,Citation14 Unfortunately, no data are available on the association between glycoalkaloid exposure and OA progression. In the current study, we aimed to investigate the effects of α-Chaconine on the progression of mouse OA model induced by DMM surgery. We found that α-Chaconine could impair articular cartilage and contribute to matrix degradation, osteophyte formation, chondrocyte pyroptosis, as well as sensory nerve ingrowth. Subsequent mechanistic analysis revealed that α-Chaconine could significantly activate NF-κB signaling activity, which acts as an upstream activator of chondrocyte dysfunction and pyroptosis, and sensory nerve innervation in subchondral bone. To the best of our knowledge, our findings provide the first in vivo evidence supporting excessive α-Chaconine exposure aggravates OA development.
As one of the most common degenerative diseases encountered clinically, OA is the primary cause of mobility impairment in the old due to pain and is also an extensive cause of disability worldwide.Citation46 As the primary symptom of this disease, joint pain is arising from any part dysfunction of a joint, such as inflammatory-mediated damage to articular chondrocytes, bones, ligaments, tendons, and the progressive degradation of chondrocyte extracellular matrix.Citation47 It has been well established that the increased expressions of matrix-degrading enzymes, such as Mmp3 and Mmp13, are the reason contributing to the symptoms and progression of OA.Citation48 Moreover, failure to correctly maintain cartilage homeostasis leads to softer, less elastic, and pitted cartilage, concomitant with the formation of osteophytes, or bone spurs.Citation49 In our research, α-Chaconine promoted osteophyte formation and exacerbated cartilage degradation with higher Mmp3 and Mmp13 but lower Col2, indicating that α-Chaconine is detrimental to OA progression.
Pyroptosis is morphologically and mechanistically distinct from other forms of cell death, characterized by Nlrp3- and Caspase-1-mediated inflammasome, triggering the release of pyrogenic cytokines IL-1β and IL-18.Citation50,Citation51 This process can induce cell rupture and inflammatory response, facilitating the pathogenesis of many chronic inflammatory diseases.Citation52–54 Substantial evidence from our and others’ studies suggests that chondrocyte pyroptosis contributes to the pathology of OA, leading to cartilage degeneration, formation of osteochondral fissures and osteophytes, and increase in nociceptive input in joint tissue.Citation35,Citation38,Citation55 In agreement with these studies, we found that α-Chaconine remarkably increased the expressions of IL-1β and IL-18 proteins via activating pyroptosis, suggesting α-Chaconine could cause a state of hyperalgesia and OA pain.
Knee pain not only needs outbreaks of inflammatory factors induced by pyroptosis but also needs the participation of nociceptive neurons in the transmission of pain.Citation20,Citation27 A previous clinical study has reported that aberrant remodeling of subchondral bone results in sensory nerve innervation, thereby aggravating pain in OA patients.Citation56 Further mechanism analysis demonstrated that netrin-1 secreted by osteoclasts during aberrant subchondral bone remodeling could induce Cgrp+ sensory innervation and exaggerate the sensation of pain;Citation18 while reducing Netrin-1 secretion could lower Cgrp+ sensory neurons in the subchondral bone and significantly relieve OA-related pain.Citation18–20 Consistently, the present study identified that α-Chaconine could significantly intensify the expression of Netrin-1 and the ratio of Cgrp+ neurons in OA mice. Based on the above results, we speculate that α-Chaconine may contribute to the expansion of nociceptive input by eliciting chondrocytes pyroptosis and sensory innervation. To verify whether α-Chaconine aggravates the development of human OA disease and the corresponding pain sensitivity, a clinical trial (eg, NCT00080171 registered in ClinicalTrials.gov) needs to be adopted to identify the correlation between serum α-Chaconine levels with pain sensitivity and OA progression in clinical OA patients.
It has been well established that NF-κB signaling is involved in OA pathophysiology through various effects, including upregulation of inflammatory and catabolism-related proteins.Citation57 Our recent findings on degenerative joint diseases have shown that aberrant activation of NF-κB pathway leads to chondrocyte pyroptosis and disruption of matrix metabolic homeostasis.Citation35,Citation38,Citation58–60 Emerging evidence suggests that the activity of NF-κB signaling is strongly correlated with the expression of pain-related neuropeptides such as Netrin-1, Cgrp, contributing to neuropathic pain.Citation33,Citation61 All these findings suggest that NF-κB signaling cascade is a known regulator of pyroptosis and nerve ingrowth. Consistently, in our study presented here, α-Chaconine activated NF-κB signaling both in articular chondrocytes and subchondral bone marrow, suggesting that α-Chaconine may induce chondrocyte pyroptosis and sensory innervation by activation of NF-κB signaling.
Potatoes serve as major, inexpensive low-fat food sources providing energy (starch), high-quality protein, fiber, and vitamins, which are consumed massively worldwide.Citation62 They do, however, also produce glycoalkaloids that at appropriate levels may be toxic to bacteria, fungi, viruses, and insects.Citation63 In high amounts, these compounds can also produce toxic effects in humans. Acute toxicity experiments have identified that glycoalkaloids could cause rapid damage to plasma membrane, leading to the lethal disorder of metabolism,Citation64 which mainly results from acetylcholinesterase inhibition and cell membrane disruption that affect the digestive and other organs.Citation40,Citation65 Toxicities induced in other species include hepatotoxicity in mice,Citation66 increased hepatic ornithine decarboxylase activity in rats,Citation67 craniofacial malformations in hamsters,Citation68 and anatomical developmental toxicities in frog embryos.Citation69 Likewise, our consequences also confirmed that α-Chaconine treatment is detrimental to OA progression by strengthening matrix metabolic degradation, potentiating chondrocyte pyroptosis and nerve ingrowth. Overall, our data indicated that long-time exposure to α-Chaconine may be harmful to OA development.
Besides, several limitations were noted in the present study. 1) Different from normal intragastric ingestion of α-Chaconine, in this study, we locally injected α-Chaconine into the joint cavity to focus on the direct pathogenic roles of α-Chaconine on OA joint tissues. Different administration routes of α-Chaconine (eg, gavage, intravenous injection, and intraperitoneal injection) are needed to further validate the findings in this study. In addition, it needs to be clarified whether α-Chaconine has a long-term cumulative toxic effect on knee function and phenotypic characteristics of normal mice without receiving OA modeling. 2) As α-Chaconine is more toxic than α-solanine, the present study focused on the deleterious effects of α-Chaconine on OA progress. However, except for α-Chaconine, is there anything else in potatoes (eg, α-solanine, which is concomitantly present in the potato and may have a similar or lower toxicity and teratogenicity) alone or together with α-Chaconine synergistically exacerbate OA progression remains uncertain, further work will be required to address this point. 3) To further confirm the effects of α-Chaconine on OA pain, it is necessary to comprehensively evaluate the joint function and behavior phenotypes of OA mice using motor coordination analysis, mechanical analysis, and thermal hyperalgesia analyses in the future. In addition, we focused only on the pathological changes of cartilage and subchondral bone, without evaluating other parts of the joint tissue (eg, meniscus and infrapatellar fat) involved in OA and OA pain. The implementation of all these aspects will unprecedentedly enhance our understanding of the multiple and overlapping aspects of glycoalkaloids in diet, which will benefit consumers of potatoes.
Conclusions
In summary, we found that high-dose α-Chaconine contributes to cartilage degradation, bone structure destruction, chondrocyte pyroptosis, and aberrant nerve ingrowth via activation of NF-κB signaling, thereby exacerbating OA progression. Our results provide new evidence and insights into the potential deleterious roles of α-Chaconine or eggplant high intake on OA development.
Author Contributions
All authors contributed to data analysis, drafting or revising the article, have agreed on the journal to which the article will be submitted, gave final approval of the version to be published, and agree to be accountable for all aspects of the work. Zhiguo Zhang: Conceptualization, Methodology, Investigation, Visualization, Writing-original draft, Writing-review & editing. Fangda Fu: Methodology, Investigation, Investigation, Formal analysis, Writing-review & editing. Yishan Bian: Methodology, Investigation, Writing-review & editing. Huihao Zhang: Methodology, Investigation, Writing-review & editing. Sai Yao: Methodology, Investigation, Writing-review & editing. Chengcong Zhou: Methodology, Investigation, Visualization, Writing-review & editing. Yuying Ge: Methodology, Investigation, Writing-review & editing. Yuying Chen: Investigation, Writing-review & editing. Huan Luo: Investigation, Writing-review & editing. Weifeng Ji: Conceptualization, Investigation, Writing-review & editing. Kun Tian: Conceptualization, Investigation, Writing-review & editing. Ming Yue: Conceptualization, Investigation, Writing-review & editing. Weibin Du: Conceptualization, Investigation, Writing-review & editing. Hongting Jin: Conceptualization, Investigation, Writing-review & editing. Peijian Tong: Conceptualization, Writing-review & editing. Chengliang Wu: Conceptualization, Methodology, Formal analysis, Writing-original draft, Writing-review & editing. Hongfeng Ruan: Conceptualization, Methodology, Formal analysis, Writing-original draft, Writing-review & editing.
Disclosure
The authors report no conflicts of interest in this work.
Acknowledgments
This work was financially supported by National Natural Science Foundation of China (No.: 82174140, 82174401, 82104164, 81973870, 81904053), China Postdoctoral Science Foundation (No.: 2018M632154), Natural Science Foundation of Zhejiang Province (No.: LY22H270003, and LQ19H080001), Joint Funds of the Zhejiang Provincial Natural Science Foundation of China (No. LBY22H270008), Traditional Chinese Medical Administration of Zhejiang Province (No.: 2022ZX005, 2022ZB119, 2021ZB090), Zhejiang medical and health science and technology project (No.: 2023RC194, 2021KY222), Research Project of Zhejiang Chinese Medical University (No.: 2021JKZDZC02, 2021JKZKTS036A, 2021JKJNTZ022B, 2019ZG25, 2018ZR06), National Undergraduate Innovation and Entrepreneurship Training Program (202210344006, 20221034402, 202210344037, 202210344064, 202110344005, 202110344025, S202110344007, 202010344004), General Research Project of Zhejiang Provincial Education Department ”Special Project for the Reform of Cultivation Mode of Professional Degree Graduate Students in Higher Education Institutions” (No.: Y202145932), Postgraduate Science Research Fund of Zhejiang Chinese Medical University (No.: 2021YKJ02, 2020YKJ07), The Science and Technology Innovation Program for College Students (new talent program) of Zhejiang Province (No.: 2022R410A001, 2022R410A009, 2022R410A018).
References
- Nelson AE. Osteoarthritis year in review 2017: clinical. Osteoarthritis Cartilage. 2018;26(3):319–325. doi:10.1016/j.joca.2017.11.014
- Hunter DJ, Bierma-Zeinstra S. Osteoarthritis. Lancet. 2019;393(10182):1745–1759. doi:10.1016/S0140-6736(19)30417-9
- Charlier E, Deroyer C, Ciregia F, et al. Chondrocyte dedifferentiation and osteoarthritis (OA). Biochem Pharmacol. 2019;165:49–65. doi:10.1016/j.bcp.2019.02.036
- Berenbaum F, Wallace IJ, Lieberman DE, Felson DT. Modern-day environmental factors in the pathogenesis of osteoarthritis. Nat Rev Rheumatol. 2018;14(11):674–681. doi:10.1038/s41584-018-0073-x
- Sánchez Romero EA, Meléndez Oliva E, Alonso Pérez JL, et al. Relationship between the gut microbiome and osteoarthritis pain: review of the literature. Nutrients. 2021;13(3):716. doi:10.3390/nu13030716
- McAlindon T, Felson DT. Nutrition: risk factors for osteoarthritis. Ann Rheum Dis. 1997;56(7):397–400. doi:10.1136/ard.56.7.397
- Schrenk D, Bignami M, Bodin L, et al. Risk assessment of glycoalkaloids in feed and food, in particular in potatoes and potato-derived products. EFSA. 2020;18(8):e06222. doi:10.2903/j.efsa.2020.6222
- Yang SA, Paek SH, Kozukue N, Lee KR, Kim JA. Alpha-chaconine, a potato glycoalkaloid, induces apoptosis of HT-29 human colon cancer cells through caspase-3 activation and inhibition of ERK 1/2 phosphorylation. Food Chem Toxicol. 2006;44(6):839–846. doi:10.1016/j.fct.2005.11.007
- Friedman M. Potato glycoalkaloids and metabolites: roles in the plant and in the diet. J Agric Food Chem. 2006;54(23):8655–8681. doi:10.1021/jf061471t
- Liu Q, Hebert JR, Shivappa N, et al. Inflammatory potential of diet and risk of incident knee osteoarthritis: a prospective cohort study. Arthritis Res Ther. 2020;22(1):209. doi:10.1186/s13075-020-02302-z
- Iablokov V, Sydora BC, Foshaug R, et al. Naturally occurring glycoalkaloids in potatoes aggravate intestinal inflammation in two mouse models of inflammatory bowel disease. Dig Dis Sci. 2010;55(11):3078–3085. doi:10.1007/s10620-010-1158-9
- Best vegetables for arthritis; 2022. Available from: https://www.arthritis.org/health-wellness/healthy-living/nutrition/healthy-eating/best-vegetables-for-arthritis#:~:text=Eggplants%2C%20peppers%2C%20tomatoes%20and%20potatoes%20are%20all%20members,some%20people%20claim%20aggravates%20arthritis%20pain%20and%20inflammation. Accessed August 31, 2022.
- Langkilde S, Mandimika T, Schrøder M, et al. A 28-day repeat dose toxicity study of steroidal glycoalkaloids, alpha-solanine and alpha-chaconine in the Syrian Golden hamster. Food Chem Toxicol. 2009;47(6):1099–1108. doi:10.1016/j.fct.2009.01.045
- Friedman M, Henika PR, Mackey BE. Effect of feeding solanidine, solasodine and tomatidine to non-pregnant and pregnant mice. Food Chem Toxicol. 2003;41(1):61–71. doi:10.1016/S0278-6915(02)00205-3
- Stoppiello LA, Mapp PI, Wilson D, Hill R, Scammell BE, Walsh DA. Structural associations of symptomatic knee osteoarthritis. Arthritis Rheumatol. 2014;66(11):3018–3027. doi:10.1002/art.38778
- Zeng N, Yan ZP, Chen XY, Ni GX. Infrapatellar fat pad and knee osteoarthritis. Aging Dis. 2020;11(5):1317–1328. doi:10.14336/AD.2019.1116
- Kwon H, Brown WE, Lee CA, et al. Surgical and tissue engineering strategies for articular cartilage and meniscus repair. Nat Rev Rheumatol. 2019;15(9):550–570. doi:10.1038/s41584-019-0255-1
- Zhu S, Zhu J, Zhen G, et al. Subchondral bone osteoclasts induce sensory innervation and osteoarthritis pain. J Clin Invest. 2019;129(3):1076–1093. doi:10.1172/JCI121561
- Fang C, Guo JW, Wang YJ, et al. Diterbutyl phthalate attenuates osteoarthritis in ACLT mice via suppressing ERK/c-fos/NFATc1 pathway, and subsequently inhibiting subchondral osteoclast fusion. Acta Pharmacol Sin. 2022;43(5):1299–1310. doi:10.1038/s41401-021-00747-9
- Jiang W, Jin Y, Zhang S, et al. PGE2 activates EP4 in subchondral bone osteoclasts to regulate osteoarthritis. Bone Res. 2022;10(1):27. doi:10.1038/s41413-022-00201-4
- Lee KI, Choi S, Matsuzaki T, et al. FOXO1 and FOXO3 transcription factors have unique functions in meniscus development and homeostasis during aging and osteoarthritis. Proc Natl Acad Sci U S A. 2020;117(6):3135–3143. doi:10.1073/pnas.1918673117
- Greene MA, Loeser RF. Aging-related inflammation in osteoarthritis. Osteoarthritis Cartilage. 2015;23(11):1966–1971. doi:10.1016/j.joca.2015.01.008
- Jiang W, Wang H, Li YS, Luo W. Role of vasoactive intestinal peptide in osteoarthritis. J Biomed Sci. 2016;23(1):63. doi:10.1186/s12929-016-0280-1
- Belluzzi E, Macchi V, Fontanella CG, et al. Infrapatellar fat pad gene expression and protein production in patients with and without osteoarthritis. Int J Mol Sci. 2020;21(17):6016. doi:10.3390/ijms21176016
- Ozeki N, Koga H, Sekiya I. Degenerative meniscus in knee osteoarthritis: from pathology to treatment. Life. 2022;12(4):603. doi:10.3390/life12040603
- Gram AM, Booty LM, Bryant CE; Chopping GSDMD. caspase-8 has joined the team of pyroptosis-mediating caspases. EMBO J. 2019;38(10):e102065. doi:10.15252/embj.2019102065
- An S, Hu H, Li Y, Hu Y. Pyroptosis plays a role in osteoarthritis. Aging Dis. 2020;11(5):1146–1157. doi:10.14336/AD.2019.1127
- Lin C, Liu L, Zeng C, et al. Activation of mTORC1 in subchondral bone preosteoblasts promotes osteoarthritis by stimulating bone sclerosis and secretion of CXCL12. Bone Res. 2019;7:5. doi:10.1038/s41413-018-0041-8
- Hu Y, Chen X, Wang S, Jing Y, Su J. Subchondral bone microenvironment in osteoarthritis and pain. Bone Res. 2021;9(1):20. doi:10.1038/s41413-021-00147-z
- Jimi E, Fei H, Nakatomi C. NF-κB signaling regulates physiological and pathological chondrogenesis. Int J Mol Sci. 2019;20(24):6275. doi:10.3390/ijms20246275
- Choi MC, Jo J, Park J, Kang HK, Park Y. NF-κB signaling pathways in osteoarthritic cartilage destruction. Cells. 2019;8(7):734. doi:10.3390/cells8070734
- Lepetsos P, Papavassiliou KA, Papavassiliou AG. Redox and NF-κB signaling in osteoarthritis. Free Radic Biol Med. 2019;132:90–100. doi:10.1016/j.freeradbiomed.2018.09.025
- Paradisi A, Maisse C, Bernet A, et al. NF-kappaB regulates netrin-1 expression and affects the conditional tumor suppressive activity of the netrin-1 receptors. Gastroenterology. 2008;135(4):1248–1257. doi:10.1053/j.gastro.2008.06.080
- Mandimika T, Baykus H, Vissers Y, et al. Differential gene expression in intestinal epithelial cells induced by single and mixtures of potato glycoalkaloids. J Agric Food Chem. 2007;55(24):10055–10066. doi:10.1021/jf0724320
- Hu J, Zhou J, Wu J, et al. Loganin ameliorates cartilage degeneration and osteoarthritis development in an osteoarthritis mouse model through inhibition of NF-κB activity and pyroptosis in chondrocytes. J Ethnopharmacol. 2020;247:112261. doi:10.1016/j.jep.2019.112261
- Glasson SS, Blanchet TJ, Morris EA. The surgical destabilization of the medial meniscus (DMM) model of osteoarthritis in the 129/SvEv mouse. Osteoarthritis Cartilage. 2007;15(9):1061–1069. doi:10.1016/j.joca.2007.03.006
- Lim NH, Wen C, Vincent TL. Molecular and structural imaging in surgically induced murine osteoarthritis. Osteoarthritis Cartilage. 2020;28(7):874–884. doi:10.1016/j.joca.2020.03.016
- Yu H, Yao S, Zhou C, et al. Morroniside attenuates apoptosis and pyroptosis of chondrocytes and ameliorates osteoarthritic development by inhibiting NF-κB signaling. J Ethnopharmacol. 2021;266:113447. doi:10.1016/j.jep.2020.113447
- Timmins KA, Leech RD, Batt ME, Edwards KL. Running and knee osteoarthritis: a systematic review and meta-analysis. Am J Sports Med. 2017;45(6):1447–1457. doi:10.1177/0363546516657531
- McGehee DS, Krasowski MD, Fung DL, Wilson B, Gronert GA, Moss J. Cholinesterase inhibition by potato glycoalkaloids slows mivacurium metabolism. Anesthesiology. 2000;93(2):510–519. doi:10.1097/00000542-200008000-00031
- Alozie SO, Sharma RP, Salunkhe DK. Inhibition of rat cholinesterase isoenzymes in vitro and in vivo by the potato alkaloid, α-Chaconine1,2. J Food Biochem. 1978;2:3. doi:10.1111/j.1745-4514.1978.tb00621.x
- Hellenäs KE, Nyman A, Slanina P, Lööf L, Gabrielsson J. Determination of potato glycoalkaloids and their aglycone in blood serum by high-performance liquid chromatography. Application to pharmacokinetic studies in humans. J Chromatogr. 1992;573(1):69–78. doi:10.1016/0378-4347(92)80476-7
- Mandimika T, Baykus H, Poortman J, Garza C, Kuiper H, Peijnenburg A. Induction of the cholesterol biosynthesis pathway in differentiated Caco-2 cells by the potato glycoalkaloid alpha-chaconine. Food Chem Toxicol. 2007;45(10):1918–1927. doi:10.1016/j.fct.2007.04.009
- Guilak F, Nims RJ, Dicks A, Wu CL, Meulenbelt I. Osteoarthritis as a disease of the cartilage pericellular matrix. Matrix Biol. 2018;71:40–50. doi:10.1016/j.matbio.2018.05.008
- Schou WS, Ashina S, Amin FM, Goadsby PJ, Ashina M. Calcitonin gene-related peptide and pain: a systematic review. J Headache Pain. 2017;18(1):34. doi:10.1186/s10194-017-0741-2
- Burr DB, Gallant MA. Bone remodelling in osteoarthritis. Nat Rev Rheumatol. 2012;8(11):665–673. doi:10.1038/nrrheum.2012.130
- Chen Z, Ge Y, Zhou L, et al. Pain relief and cartilage repair by nanofat against osteoarthritis: preclinical and clinical evidence. Stem Cell Res Ther. 2021;12(1):477. doi:10.1186/s13287-021-02538-9
- McAllister MJ, Chemaly M, Eakin AJ, Gibson DS, McGilligan VE. NLRP3 as a potentially novel biomarker for the management of osteoarthritis. Osteoarthritis Cartilage. 2018;26(5):612–619. doi:10.1016/j.joca.2018.02.901
- Sharma AR, Jagga S, Lee SS, Nam JS. Interplay between cartilage and subchondral bone contributing to pathogenesis of osteoarthritis. Int J Mol Sci. 2013;14(10):19805–19830. doi:10.3390/ijms141019805
- Matsuoka T, Yoshimatsu G, Sakata N, et al. Inhibition of NLRP3 inflammasome by MCC950 improves the metabolic outcome of islet transplantation by suppressing IL-1β and islet cellular death. Sci Rep. 2020;10(1):17920. doi:10.1038/s41598-020-74786-3
- Guo R, Wang H, Cui N. Autophagy regulation on pyroptosis: mechanism and medical implication in sepsis. Mediators Inflamm. 2021;2021:9925059. doi:10.1155/2021/9925059
- Vande Walle L, Lamkanfi M. Pyroptosis. Curr Biol. 2016;26(13):R568–R572. doi:10.1016/j.cub.2016.02.019
- Sharma BR, Kanneganti TD. NLRP3 inflammasome in cancer and metabolic diseases. Nat Immunol. 2021;22(5):550–559. doi:10.1038/s41590-021-00886-5
- Liu Z, Gan L, Xu Y, et al. Melatonin alleviates inflammasome-induced pyroptosis through inhibiting NF-κB/GSDMD signal in mice adipose tissue. J Pineal Res. 2017;63:1. doi:10.1111/jpi.12414
- Yan Z, Qi W, Zhan J, et al. Activating Nrf2 signalling alleviates osteoarthritis development by inhibiting inflammasome activation. J Cell Mol Med. 2020;24(22):13046–13057. doi:10.1111/jcmm.15905
- Mapp PI, Walsh DA. Mechanisms and targets of angiogenesis and nerve growth in osteoarthritis. Nat Rev Rheumatol. 2012;8(7):390–398. doi:10.1038/nrrheum.2012.80
- Martel-Pelletier J, Barr AJ, Cicuttini FM, et al. Osteoarthritis. Nat Rev Dis Primers. 2016;2:16072. doi:10.1038/nrdp.2016.72
- Li Z, Huang Z, Zhang H, et al. P2X7 receptor induces pyroptotic inflammation and cartilage degradation in osteoarthritis via NF-κB/NLRP3 crosstalk. Oxid Med Cell Longev. 2021;2021:8868361. doi:10.1155/2021/8868361
- Chang SH, Mori D, Kobayashi H, et al. Excessive mechanical loading promotes osteoarthritis through the gremlin-1-NF-κB pathway. Nat Commun. 2019;10(1):1442. doi:10.1038/s41467-019-09491-5
- Chen Z, Lin CX, Song B, et al. Spermidine activates RIP1 deubiquitination to inhibit TNF-α-induced NF-κB/p65 signaling pathway in osteoarthritis. Cell Death Dis. 2020;11(7):503. doi:10.1038/s41419-020-2710-y
- Bowles RD, Mata BA, Bell RD, et al. In vivo luminescence imaging of NF-κB activity and serum cytokine levels predict pain sensitivities in a rodent model of osteoarthritis. Arthritis Rheumatol. 2014;66(3):637–646. doi:10.1002/art.38279
- Muraki I, Rimm EB, Willett WC, Manson JE, Hu FB, Sun Q. Potato consumption and risk of type 2 diabetes: results from three prospective cohort studies. Diabetes Care. 2016;39(3):376–384. doi:10.2337/dc15-0547
- Baur S, Frank O, Hausladen H, et al. Biosynthesis of α-solanine and α-chaconine in potato leaves (Solanum tuberosum L.) – a (13)CO(2) study. Food Chem. 2021;365:130461. doi:10.1016/j.foodchem.2021.130461
- Yamashoji S, Matsuda T. Synergistic cytotoxicity induced by α-solanine and α-chaconine. Food Chem. 2013;141(2):669–674. doi:10.1016/j.foodchem.2013.03.104
- Baker D, Keeler R, Gaffield W. Lesions of potato sprout and extracted potato sprout alkaloid toxicity in Syrian hamsters. J Toxicol Clin Toxicol. 1987;25(3):199–208. doi:10.3109/15563658708992624
- Friedman M, Henika PR, Mackey BE. Feeding of potato, tomato and eggplant alkaloids affects food consumption and body and liver weights in mice. J Nutr. 1996;126(4):989–999. doi:10.1093/jn/126.4.989
- Caldwell KA, Grosjean OK, Henika PR, Friedman M. Hepatic ornithine decarboxylase induction by potato glycoalkaloids in rats. Food Chem Toxicol. 1991;29(8):531–535. doi:10.1016/0278-6915(91)90045-9
- Gaffield W, Keeler RF. Implication of C-5, C-6 unsaturation as a key structural factor in steroidal alkaloid-induced mammalian teratogenesis. Experientia. 1993;49(10):922–924. doi:10.1007/BF01952611
- Friedman M, Rayburn JR, Bantle JA. Developmental toxicology of potato alkaloids in the frog embryo teratogenesis assay—Xenopus (FETAX). Food Chem Toxicol. 1991;29(8):537–547. doi:10.1016/0278-6915(91)90046-A