Abstract
Introduction
Atherosclerosis (AS) is the underlying cause of cardiovascular events. Endothelial cell mitochondrial damage and pyroptosis are important factors contributing to AS. Changes in internal mitochondrial conformation and increase in reactive oxygen species (ROS) lead to the disruption of mitochondrial energy metabolism, activation of the NLRP3 inflammasome and pyroptosis, which in turn affect atherogenesis by impairing endothelial function. AMPK is a core player in the regulation of cellular metabolism, not only by regulating mitochondrial homeostasis but also by regulating cellular inflammatory responses. Sodium tanshinone IIA sulfonate (STS), a water-soluble derivative of tanshinone IIA, has significant antioxidant and anti-inflammatory effects, and roles in cardiovascular protection.
Purpose
In this study, we investigated whether STS plays a protective role in AS by regulating endothelial cell mitochondrial function and pyroptosis through an AMPK-dependent mitochondrial pathway.
Methods and Results
Male ApoE−/− mice and HUVECs were used for the experiments. We found that STS treatment largely abrogated the upregulation of key proteins in aortic vessel wall plaques and typical pyroptosis signaling in ApoE−/− mice fed a western diet, consequently enhancing pAMPK expression, plaque stabilization, and anti-inflammatory responses. Consistently, STS pretreatment inhibited cholesterol crystallization (CC) -induced cell pyroptosis and activated pAMPK expression. In vitro, using HUVECs, we further found that STS treatment ameliorated mitochondrial ROS caused by CC, as evidenced by the finding that STS inhibited mitochondrial damage caused by CC. The improvement of endothelial cell mitochondrial function by STS is blocked by dorsomorphin (AMPK inhibitor). Consistently, the blockade of endothelial cell pyroptosis by STS is disrupted by dorsomorphin.
Conclusion
Our results suggest that STS enhances maintenance of mitochondrial homeostasis and inhibits mitochondrial ROS overproduction via AMPK, thereby improving endothelial cell pyroptosis during AS.
Introduction
Atherosclerosis (AS) is a commonly recognized metabolic and chronic inflammatory disease. And AS is accompanied by endothelial dysfunction, lipid deposition, and vascular wall thickening.Citation1 AS is a significant cardiovascular burden and a leading cause of death worldwide, and is recognized as the basis of cardiovascular disease (CVD).Citation2,Citation3 ASCVD results in approximately 2.4 million deaths annually in China.Citation4–6 Vascular endothelial cells constitute the inner wall of arterial vessels, and the morphological integrity and balance of their function significantly contribute to vascular health.Citation7 Endothelial dysfunction, structural alterations, and chronic inflammation of the vascular wall are known to be critical steps in AS development, as well as in the instability of advanced plaques.Citation8,Citation9 However, the underlying mechanisms and appropriate means to clinically manage the disease require further investigation.
Endothelial cell dysfunction is characterized by proinflammatory cytokines, elevated ROS, disrupted vascular tone, and various types of programmed cell death (PCD), including apoptosis, autophagy, pyroptosis, and necroptosis.Citation10 Pyroptosis is a newly discovered form of programmed inflammatory cell death, which is suggested to contribute to vascular endothelial cell dysfunction and the pathogenesis of AS.Citation11,Citation12 Pyroptosis can be driven by the NOD-like receptor family pyrin domain-containing 3 (NLRP3) inflammasome, which is composed of NLRP3, the adaptor apoptosis-associated speck-like protein containing a CARD (ASC), and pro-caspase1.Citation13,Citation14 Once activated, NLRP3 recruits and cleaves pro-caspase1 into its active forms, which process pro-inflammatory cytokines such as IL-1β and IL-18 and HMGB1.Citation15 Active forms of caspase-1 also cleave gasdermin D (GSDMD), separating the N-terminal pore-forming structural domain (PFD) of GSDMD from the C-terminal deterrent structural domain (RD) and the subsequent oligomerization of PFD transferred into the cell membrane. And the oligomer of GSDMD leads to massive pore formation, resulting in cell death, leakage of the cellular contents, including proinflammatory substances, and increased secondary inflammation.Citation16 Oxidized low density lipoprotein (ox-LDL) and cholesterol crystals (CC) are the two main factors promoting atherosclerosis. In addition, ox-LDL and cholesterol crystals represent the central activators of the NLRP3 inflammasome and endothelial cell pyroptosis through excessive ROS production.Citation17–19 Moreover, some risk factors for AS, such as hyperlipidemia, smoking, obesity, diabetes, and hypertension, can result in EC dysfunction and even death by triggering inflammasome assembly.Citation20 Therefore, endothelial cell pyroptosis may play a prominent role in AS-interrelated inflammation, and targeted regulation of the endothelial NLRP3 inflammasome in atherosclerotic lesions may represent a novel direction for treating AS.Citation21
Recent studies have suggested that some risk factors for AS (eg, ox-LDL and cholesterol crystals) mediate endothelial cell dysfunction primarily via mitochondrial damage.Citation22 Mitochondrial dysfunction can lead to ROS production, mitochondrial DNA (mtDNA) damage, ATP reduction, and membrane potential decline. Mitochondria are the main source of ROS production and mtROS has also been shown to drive NLRP3 inflammasome activation.Citation23,Citation24 Thus, maintenance of mitochondrial homeostasis is necessary to sustain endothelial cell survival and modulate the inflammation in AS.Citation25 AMP-activated protein kinase (AMPK) is a highly conserved serine/threonine protein kinase and a key molecule in the regulation of bioenergy metabolism.Citation26 Importantly, activated AMPK monitors mitochondrial function and cellular energy status in AS.Citation27 The up-regulation of the AMPK pathway has been proven to be conducive to the preservation of mitochondrial function in metabolic diseases under the overactivation of the NLRP3 inflammasome.Citation28 Thus, the AMPK/PGC-1α pathway shows potential as a novel therapeutic target for AS.
Tanshinone IIA, a diterpene quinone that originates from the roots of the traditional Chinese herb Salvia miltiorrhiza, is used as a treatment for CVD and columnar ectopy.Citation29 Sodium tanshinone IIA sulfonate (STS) is a water-soluble derivative of tanshinone IIA,Citation30 and sodium tanshinone IIA sulfonate injection has been approved by the Chinese State Food and Drug Administration (CFDA) for the treatment of cardiovascular diseases.Citation31,Citation32 Recently, several studies have explored other potential therapeutic effects of tanshinone IIA and its derivatives in various diseases, including central nervous system disorders,Citation33 diabetes,Citation34 cancer,Citation35 depressionCitation36 and liver diseases.Citation37,Citation38 Tanshinone IIA and its derivatives also displays a potent beneficial effect in AS by improving endothelial function via multiple processes, including by stabilizing vulnerable AS plaques via the TLR4/MyD88/NF-κB signaling pathway,Citation39 improving mitochondrial function,Citation40 alleviating oxidative stress,Citation41 inhibiting cell deathCitation42,Citation43 and reducing the inflammatory response.Citation41 Nonetheless, whether STS impacts NLRP3 inflammasome activation and subsequent pyroptosis in endothelial cells, and what the underlying mechanisms could be, remain unclear. Considering the NLRP3 inflammasome and pyroptosis are important in endothelial dysfunction and AS, these factors warrant investigation. Therefore, we examined the protective effects of STS, particularly on NLRP3 inflammasome activation and pyroptosis, and explored the potential mechanism by which these occur. In the present study, we found that STS inhibits NLRP3 inflammasome activation and subsequent pyroptosis, and may be mediated by the AMPK/mitochondria axis in HUVECs treated with cholesterol crystals.
Materials and Methods
Materials
Dorsomorphin (Compound C, S7840), and Sodium tanshinone IIA sulfonate (STS, purity 98.61%, S3766) were obtained from Selleck (Shanghai, China). LPS (Escherichia coli O11:B4) and cholesterol were purchased from Sigma (St Louis, USA). And cholesterol crystals as were prepared as previously described.Citation44,Citation45
Animals and Establishment of as Model
Male ApoE−/− mice (18–22 g, 6 weeks of age) were provided by GemPharmatech Co., Ltd (Nanjing, China). The animals were acclimated for 7 days with a 12 h light-dark cycle with water and food ad libitum. Animal care and experiments were performed according to the Guide of Chinese Regulation for the Use and Care of Laboratory Animals. The experiments were approved by the Medical Code and Ethics Committee of Zhejiang Chinese Medical University (approval number: 20200720-06). ApoE−/− mice were fed with a western diet [containing 0.5% (wt/wt) cholesterol, fat 42% kcal, carbohydrate 42.7% kcal, protein 15.3% kcal, #TP26304, Trophi Feed High-tech Co., Ltd., Nantong, China] for 12 weeks to establish the AS model. Then the ApoE−/− mice were with a western diet were randomly assigned to three groups as follows: (1) the western diet (WD) AS model group, in which the mice were administrated intragastrically saline every day and continually fed a western diet for 4 weeks; (2) the low STS (AS+LT) group, in which the mice were administrated intragastrically 10 mg/kg STS every day and continually fed a western diet for 4 weeks; and (3) the high STS (AS+HT)group, in which the mice were administrated intragastrically 20 mg/kg STS every day and continually fed a western diet for 4 weeks. Each group has four mice. And in normal control group, four male ApoE−/− mice were fed with a normal control diet [containing fat 12.5% kcal, carbohydrate 68.1% kcal, protein 19.4% kcal, #TP26352, Trophi Feed High-tech Co., Ltd., Nantong, China] for 16 weeks.
Analysis of Atherosclerotic Lesions
Mouse aortas were opened longitudinally from the ascending aorta to the abdominal aorta, and fixed in 4% paraformaldehyde for 36 h. Hematoxylin and eosin (HE) staining assay was used to detect atherosclerotic lesions, and the Oil Red O (Solarbio, China) staining kit was used to evaluate lipid accumulation. Masson staining was used to evaluate the content of collagen fibers in atherosclerotic plaques. Immunofluorescence staining was performed to stain the location of endothelial cells in atherosclerotic lesions using CD31 antibody (1:100, Abcam, USA), and to detect the expression of GSDMD (1:50, Santa Cruz, USA) and the co-localization of endothelial NLRP3 (1:100, AdipoGen, USA) and ASC (1:100, AdipoGen, USA) by visualizing using a fluorescence microscope (Olympus, Japan). Immunohistochemistry staining was performed to stain the level of IL-1β and IL-18 in atherosclerotic lesions using IL-1β antibody (1:100, Proteintech, China) and IL-18 antibody (1:100, Proteintech, China) by visualizing using a Nano Zoomer (Hamamatsu, Japan). Quantitative analysis of lesions was performed using Fiji software (NIH, USA).
Cell Culture
HUVECs (Lonza, USA) cultured in Endothelial cell medium (ECM, Sciencell, USA) at 37°C with 5% CO2 and 95% air.
Cell Death Assay
Cell death was evaluated using a PI assay. For assay of cell death, HUVECs after treatment were detected using a Calcein-AM/PI Double Staining Kit (Dojindo Kumamoto, Japan).
Cellular Mitochondrial ROS (mtROS) and Mitochondrial Membrane Potential (MMP) Assay
After incubating with Mito-Tracker Green (100 nM, Thermo Fisher Scientific, USA) at 37°C for 30 min, HUVECs were incubated with MitoSox Red (5 μM, Thermo Fisher Scientific, USA) in phosphate-buffered saline (PBS) at 37°C for 30 min. The cells were then washed thrice with warm PBS. The MMP was determined with TMRE (100nM, MCE, USA). And the level of mtROS and MMP were assayed using the ImageXpress® Micro Confocal High-Content Imaging System (Molecular Devices, USA) as described before.Citation46,Citation47
Western Blot Analysis
Total protein was extracted from HUVECs using Cell lysis buffer for Western and IP [20mM Tris(pH7.5), 150mM NaCl, 1% Triton X-100, Beyotime, China]. The concentrations of protein were determined by a BCA protein assay kit (Vazime, China). 20 μg proteins were separated by BeyoGel™ Plus Precast PAGE Gel (Beyotime, China) and transferred onto PVDF membranes (Millipore, USA). After blocking with 5% dry milk, the membrane was incubated with primary antibodies against NLRP3 (1:1000, AdipoGen, USA), ASC (1:1000, AdipoGen, USA), Caspase-1 (1:1000, Abcam, UK), GSDMD (1:500, Santa, USA), and IL-1β (1:500, Santa Cruz, USA), AMPKα (1:1000, Cell Signaling Technology, USA), and Phospho-AMPKα (Thr172) (1:1000, Cell Signaling Technology, USA), β-actin (1:2000, Proteintech, China) overnight at 4°C. Following incubation, the membrane was washed thrice with TBST (0.05% Tween 20). After incubation with the corresponding secondary antibody [goat anti-rabbit (1:5000, BOSTER Biological Technology co. ltd, China) and goat anti-mouse (1:5000, BOSTER Biological Technology co. ltd, China)], the membrane was exposed to an enhanced chemiluminescence kit (Vazime, China), and observed using a Clinx ChemiScope 3500 (Clinx Science instrument Co. Ltd, China).
Measurement of TG, TC, LDL-C, and HDL-C Contents
Levels of TC, TG, LDL-C and HDL-C in serum were measured using commercial kits (Nanjing Jiancheng Bioengineering Institute, China)
Statistical Analysis
GraphPad Prism 8.0 software was used for statistical analysis. One-way ANOVA was used to analyze differences among groups. All data in this study are presented as the mean ± SD. P-values < 0.05 were considered statistically significant.
Results
Effects of STS on Aortic Lesions, Lipid Homeostasis, and Inflammation in WD-Induced ApoE−/− Mice
To confirm the protective effect of STS postconditioning on AS, we used HE and Masson staining in histological sections of the ascending aorta of the ApoE−/− mice. And Oil red O staining was performed to show aortic lesions. The serum levels of low-density lipoprotein cholesterol (LDL-C), high density lipoprotein cholesterol (HDL-C), triglyceride (TG), and total cholesterol (TC) were also determined to investigate the effect of STS on lipid profiles. Our results showed that after treatment with STS, the atherosclerotic plaque decreased significantly compared to the WD group as shown by Oil red O staining (). H&E staining indicated that the aortas from the WD group exhibited an increased intimal lesion area containing a necrotic core and Masson staining showed unstable plaque with cholesterol crystal and healed plaque rupture in WD group (). However, STS treatment improved this condition (). The results showed that STS significantly decreased the levels of LDL-C, TG, and TC, especially in the high-dose group (). However, the HDL-C levels were not changed in the WD group ().
Figure 1 Sodium tanshinone IIA sulfonate alleviates atherosclerosis in ApoE−/− mice. (A) Body Weight, (B) plasma total cholesterol (TC) level, (C) plasma triglyceride (TG) level, (D) Plasma low-density lipoprotein cholesterol (LDL-c) level, and (E) plasma high density liptein cholesterol (HDL-c) level. (F) H&E staining of the aortic arches of the ApoE−/− mice. (G) Masson staining of the aortic arches. (H) Oil red O staining of the aortic arches. Data are represented as mean ± SD of three independent replicates; **P < 0.01 vs NCD group; †P < 0.05, ††P < 0.01 vs AS model group.
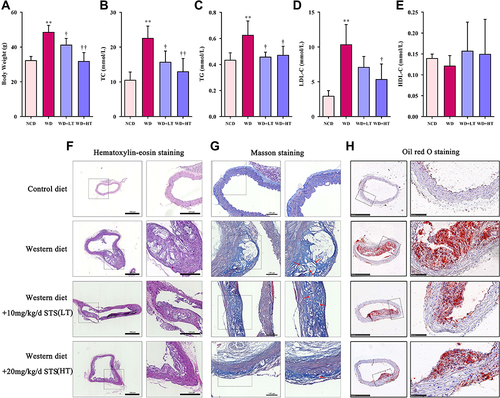
STS Inhibited Pyroptosis in the Aortic Intima of WD-Induced ApoE−/− Mice
Compared to the NCD group, immunofluorescent triple staining of the aortic sinus of NLRP3, ASC, and CD31 (endothelial cell marker) revealed that the content of endothelial NLRP3-ASC colocalization was significantly increased in the ascending aorta of WD group, while STS significantly suppressed this increase (). Our results further showed that the expression of GSDMD in the ascending aorta of WD-fed ApoE−/− mice was significantly increased compared to that of the NCD group, while STS significantly suppressed the enhanced expression of GSDMD (). Furthermore, the positive area of IL-1β and IL-18 was increased in plaques of mice fed with Western diet, but decreased in plaques of mice administrated with STS (). Our findings suggested that STS suppressed the pyroptosis of endothelial cells in the aortic intima of WD-fed ApoE−/− mice.
Figure 2 Sodium tanshinone IIA sulfonate alleviates the pyroptosis in atherosclerotic plaques. (A) NLRP3 and ASC in endothelial cells (marked by CD31) the aortic arches were detected by immunofluorescence. (B) GSDMD level in sections of the aortic arches was detected by immunofluorescence. (C) Immunohistochemistry analysis of IL-1β expression. (D) Immunohistochemistry analysis of IL-18 expression. Data are represented as mean ± SD of three independent replicates; *P < 0.05, **P < 0.01 vs NCD group; †P < 0.05, ††P < 0.01 vs AS model group; Scale bar = 50 μm.
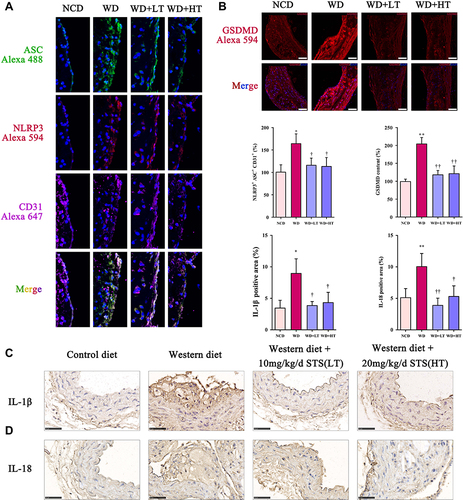
STS Inhibited NLRP3 Inflammasome and Pyroptosis in HUVECs
We used 500 μM cholesterol crystals to induce an inflammatory response in HUVECs. Using the PI assay, we demonstrated that treatment with STS dose-dependently decreased the cell death with stimulus of cholesterol crystals (). To evaluate the effects of STS on the NLRP3 inflammasome and pyroptosis, we measured the level of NLRP3, ASC, activated caspase-1, IL-1β, and GSDMD. STS treatment decreased the levels of NLRP3, ASC, cleaved caspase-1, cleaved IL-1β, and cleaved GSDMD in HUVECs compared to the cholesterol crystals group (). We then investigated the specific function of STS in NLRP3 inflammasome assembly. First, NLRP3 specks were detected by immunofluorescence, and our results showed that treatment with STS decreased the number of cholesterol crystals-induced NLRP3 specks (). These results suggest that STS regulates the formation of the NLRP3 inflammation to suppress pyroptotic cell death in HUVECs.
Figure 3 Sodium tanshinone IIA sulfonate alleviates cholesterol crystals-induced pyroptotic damage in HUVECs. HUVECs were incubated with 500 μM cholesterol crystals (CC) for 16 h. 10, 20, 40 μM Sodium tanshinone IIA sulfonate (STS) was added 1 h before CC treatment. (A) Cell death was determined using PI staining. (B) NLRP3, ASC, caspase-1, GSDMD and IL-1β protein levels were determined by Western blot. (C) NLRP3 specks were detected by immunofluorescence. Data are represented as mean ± SD of three independent replicates; **P < 0.01 vs VEH group; §P < 0.05, §§P < 0.01 vs cholesterol crystals group; Scale bar = 10 μm.
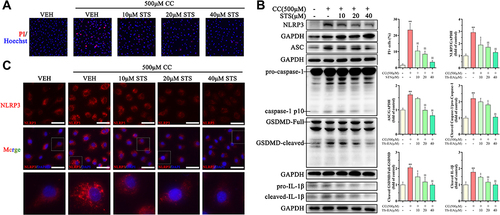
STS Improved Mitochondrial Function in HUVECs
Mitochondrial dysfunction is one of the upstream signaling events for NLRP3 activation.Citation24 Cholesterol crystals-induced mitochondrial damage and mtROS over-production were detected in HUVECs. Treatment with STS inhibited the cholesterol crystals-induced decrease in mitochondrial membrane potential (MMP) and elevated mtROS (). These results indicate that STS suppressed damage to mitochondria and mtROS.
Figure 4 Sodium tanshinone IIA sulfonate alleviates cholesterol crystals-induced mitochondrial damage in HUVECs. HUVECs were incubated with 500 μM cholesterol crystals (CC) for 16 h. 10, 20, 40 μM Sodium tanshinone IIA sulfonate (STS) was added 1 h before CC treatment. (A) Representative images of TMRE staining in HUVECs that were stained with TMRE (red, to label MMP) and Hoechst 33342 (blue, to label nuclear). (B) HUVECs were stained with Mito-SOX probe (red, to label mtROS), Mito-tracker Green (green, to label mitochondrial) and Hoechst 33342 (blue, to label nuclear) and images were detected under Confocal High-Content Imaging System. Data are represented as mean ± SD of three independent replicates;**P < 0.01 vs VEH group; §P < 0.05, §§P < 0.01 vs cholesterol crystals group; Scale bar = 10 μm.
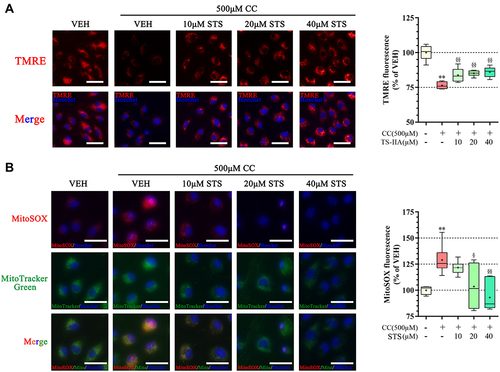
STS Inhibited Mitochondrial Dysfunction, the NLRP3 Inflammasome, and Pyroptosis in HUVECs by Activating the AMPK Pathway
AMPK is an essential regulator bridging energy metabolism, mitochondria and inflammation.Citation48,Citation49 We found that STS treatment increased AMPK phosphorylation in HUVECs, which was also confirmed in mice aortic sinus (). Subsequently, dorsomorphin, an AMPK inhibitor,Citation50 was used to confirm whether STS could suppress mtROS over-production through increasing AMPKThr172 phosphorylation. Our results showed that the inhibitory effects of STS on cell death () and mtROS () were blocked by treatment with dorsomorphin. With regard to mitochondrial homeostasis, our results showed that the beneficial effects of STS on mitochondrial membrane potential (MMP) () was disrupted by dorsomorphin. With regard to NLRP3 activation, we found that the effects of STS on inhibiting cholesterol crystals-induced activation of NLRP3 and pyroptosis were blocked by dorsomorphin (). Dorsomorphin also increased the specks of NLRP3 foci in the cell membrane (). These results indicated that STS could increase AMPK phosphorylation to suppress mtROS production, resulting in inhibition of NLRP3 inflammasome activation. These results suggest that STS can increase AMPK phosphorylation to increase mitochondrial biogenesis, thereby restoring mitochondrial function to inhibit mtROS production and thus inhibit NLRP3 activation induced-pyroptosis.
Figure 5 Sodium tanshinone IIA sulfonate activates AMPK. HUVECs were incubated with 500 μM cholesterol crystals (CC) for 16 h. 10, 20, 40 μM Sodium tanshinone IIA sulfonate (STS) was added 1 h before CC treatment. (A) p-AMPK level in sections of the aortic arches was detected by immunofluorescence. (B) p-AMPKThr172, AMPK protein levels were determined by Western blot. Data are represented as mean ± SD of three independent replicates; **P < 0.01 vs VEH group; §§P < 0.01 vs cholesterol crystals group; ††P < 0.01 vs STS treatment group.
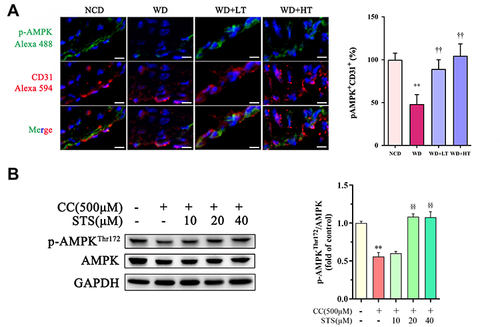
Figure 6 Inhibition of AMPK signaling eliminates the protective effect of Sodium tanshinone IIA sulfonate on cholesterol crystals-induced endothelial pyroptosis. HUVECs were incubated with 500 μM cholesterol crystals (CC) for 16 h and 40 μM Sodium tanshinone IIA sulfonate (STS) was added 1 h before CC treatment. 10 μM Dorsomorphin was added 2 h before STS treatment. (A) Cell death was determined using PI staining. (B) NLRP3, ASC, caspase-1, GSDMD and IL-1β protein levels were determined by Western blot. (C) NLRP3 specks were detected by immunofluorescence. Data are represented as mean ± SD of three independent replicates; **P < 0.01 vs VEH group; §P < 0.05, §§P < 0.01 vs cholesterol crystals group; †P < 0.05, ††P < 0.01 vs STS treatment group; Scale bar = 10 μm.
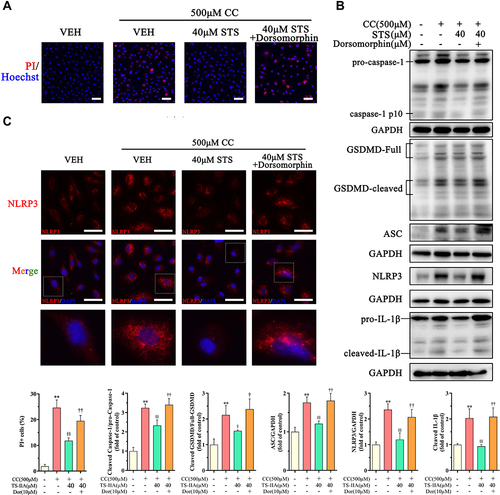
Figure 7 Inhibition of AMPK signaling eliminates the protective effect of Sodium tanshinone IIA sulfonate on cholesterol crystals-induced mitochondrial damage. HUVECs were incubated with 500 μM cholesterol crystals (CC) for 16 h and 40 μM Sodium tanshinone IIA sulfonate (STS) was added 1 h before CC treatment. 10 μM Dorsomorphin was added 2 h before STS treatment. (A) Representative images of TMRE staining in HUVECs that were stained with TMRE (red, to label MMP) and Hoechst 33342 (blue, to label nuclear). (B) HUVECs were stained with Mito-SOX probe (red, to label mtROS), Mito-tracker Green (green, to label mitochondrial) and Hoechst 33342 (blue, to label nuclear) and images were detected under Confocal High-Content Imaging System. Data are represented as mean ± SD of three independent replicates; **P < 0.01 vs VEH group; §§P < 0.01 vs cholesterol crystals group; ††P < 0.01 vs STS treatment group; Scale bar = 10 μm.
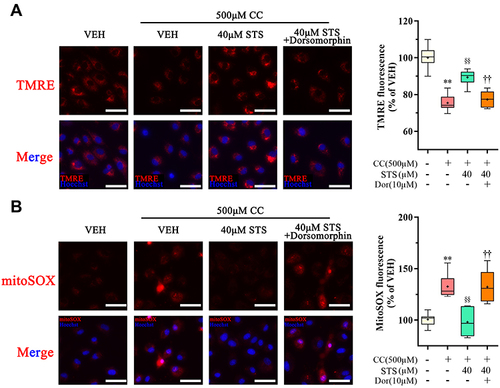
Discussion
STS is a water-soluble derivative of tanshinone IIA, which is present in the roots of the traditional Chinese herb Salvia miltiorrhiza. In recent years, studies have shown beneficial effects of STS in CVD, including antioxidant, antiplatelet aggregating, and anti-inflammatory effects.Citation51 Herein, we demonstrated that STS attenuated the formation of atherosclerotic lesions and inhibited endothelial pyroptosis in the aortic intima of WD-fed ApoE−/− mice. In addition, STS also inhibited the expression of LDL-C in serum, but the effect of HDL-C by STS is not significant. However, some studies have shown that other HDL-related biomarkers, such as HDL function or HDL particle number, may have more clinical significance than the relationship between HDL-C levels and cardiovascular disease.Citation52–54 Moreover, in vitro, STS inhibited mitochondrial dysfunction, NLRP3 inflammasome formation, and pyroptosis in cholesterol crystals-stimulated HUVECs. We demonstrated that STS may play a role in inflammation by regulating pyroptosis in endothelial cells to prevent and treat AS. Importantly, our results showed that might prevent pyroptosis in vascular endothelial cells via up-regulation of AMPK related signaling pathway.
In this study, we observed that STS attenuated the formation of atherosclerotic lesions in WD-fed ApoE−/− mice. The vascular endothelium plays a physiological role in maintaining vascular homeostasis and its dysfunction is one of the major causes of CVDs.Citation7 Some studies and our previous study, have demonstrated that STS exerts anti-inflammatory effects in CVDs.Citation41 Moreover, inflammasome-induced pyroptosis has recently been shown to be an important cause of endothelial damage.Citation55 In line with this, several studies have confirmed that precise regulation of NLRP3 can effectively inhibit vascular endothelial injury in AS.Citation20 The NLRP3 inflammasome is composed of NLRP3, ASC, and pro-caspase1.Citation56 Caspase-1 is activated by the NLRP3 polyprotein complex and subsequently cleaves inflammatory factors and GSDMD, allowing the GSDMD domain to penetrate the cell membrane, induce pyroptotic cell death, and promote the release of inflammatory factors.Citation57 In this study, the formation of the NLRP3, ASC, and GSDMD were remarkably inhibited by STS in the aortic endothelial cells of WD-fed ApoE−/− mice. And STS administration also attenuated IL-1β and IL-18 expression in the aorta of mice. Combined with the above results, the beneficial effects of STS in the prevention and reversal of AS may depend on the suppression of the activated NLRP3 inflammasome signaling pathway.
It is well-known that cholesterol crystals induce endothelial cell pyroptosis and endothelial dysfunction and cholesterol crystals markedly increase endothelial ROS production, thereby inducing NLRP3 inflammasome activation and endothelial cell pyroptosis.Citation58,Citation59 We observed that STS treatment of HUVECs alleviated cholesterol crystals-induced pyroptosis by decreasing the number of PI-positive cells, the enhanced expression of the NLRP3 inflammasome, mature caspase-1, and cleaved-GSDMD, and the level of activated-IL-1β. These results suggest that STS plays an anti-AS role by inhibiting endothelial cell pyroptosis.
Mitochondrial damage is thought to be one of the upstream signaling events for endothelial dysfunction, NLRP3 inflammasome activation, and pyroptosis, and excessive products such as mtROS and mtDNA released after mitochondrial damage. And these products can cause NLRP3 activation.Citation60 We found that cholesterol crystals treatments resulted in an increase in mtROS production, as well as changes in mitochondrial membrane potential, suggesting mitochondrial dysfunction. STS can exhibit a dose-effect restoration of mitochondrial membrane potential, and reduced mtROS production. Our work shows that STS is beneficial for regulating mitochondrial function.
AMPK, as an energy receptor, plays a central role in maintaining mitochondrial homeostasis, and controlling inflammatory stress and oxidative stress.Citation61 AMPK is considered a potential target for metabolism-related CVD, including AS, coronary heart disease, and diabetic cardiomyopathy.Citation62 AMPK inhibits inflammatory signaling and NLRP3 activation.Citation48 In this study, we found that WD caused decreased pAMPK expression in the aortic vasculature, and cholesterol crystals decreased the expression of pAMPK in endothelial cells in vitro. STS increased AMPK expression in WD mice aortic vessels, as well as in cholesterol crystals-treated HUVECs. Moreover, dorsomorphin, an AMPK inhibitor, inhibited the activation of AMPK in endothelial cells by STS and also suppressed the inhibitory effect of STS on endothelial cell pyroptosis. AMPK not only inhibits inflammation but also promotes oxidative phosphorylation and mitochondrial homeostasis.Citation61 The addition of dorsomorphin greatly disrupted the restoration of STS for mitochondrial membrane potential, and also further increased the production of mtROS. ROS generation was the first intermediate found to be common to various stimuli-induced NLRP3 inflammasome activation. And mitochondria are the major source of intracellular ROS. The over-production of mtROS is responsible for the activation of the NLRP3 inflammasome.Citation56 Overall, the regulation of endothelial pyroptosis by STS is AMPK-dependent, and the regulation of mtROS plays a central role in this process.
In summary, STS ameliorates endothelial pyroptotic cell death and AS in an NLRP3-dependent manner. STS increases the phosphorylation of AMPK, where it improves mitochondrial function and blocks mtROS production, leading to suppression of NLRP3 inflammasome activation and pyroptosis in endothelial cells. Our results suggest that STS may be used for preventing AS.
Data Sharing Statement
All of the data used in the study are available from the corresponding author.
Ethics Statement
The animal study was reviewed and approved by the Animal Experimental Ethics Committee of Zhejiang Chinese Medical University (approval number: IACUC-20200720-06).
Author Contributions
DZ Lu and J Zhu conceived and designed the study. J Zhu, H Chen, and JN Guo performed major experiments, data analysis, and drafted the manuscript. C Zha provided technological support. All authors made a significant contribution to the work reported, whether that is in the conception, study design, execution, acquisition of data, analysis and interpretation, or in all these areas; took part in drafting, revising or critically reviewing the article; gave final approval of the version to be published; have agreed on the journal to which the article has been submitted; and agreed to be accountable for all aspects of the work. These authors have contributed equally to this work and share first authorship: Ji Zhu and Hang Chen.
Disclosure
The authors report no conflicts of interest in relation to this work and declare that the research was conducted in the absence of any commercial or financial relationships that could be construed as a potential conflict of interest.
Acknowledgments
We appreciated the technical support from the Public Platform of Medical Research Center, Academy of Chinese Medical Science, Zhejiang Chinese Medical University.
Additional information
Funding
References
- Ross R, Epstein FH. Atherosclerosis — an inflammatory disease. N Engl J Med. 1999;340(2):115–126. doi:10.1056/NEJM199901143400207
- Bansilal S, Castellano JM, Fuster V. Global burden of CVD: focus on secondary prevention of cardiovascular disease. Int J Cardiol. 2015;201:S1–S7. doi:10.1016/S0167-5273(15)31026-3
- Herrington W, Lacey B, Sherliker P, Armitage J, Lewington S. Epidemiology of atherosclerosis and the potential to reduce the global burden of atherothrombotic disease. Circ Res. 2016;118(4):535–546. doi:10.1161/CIRCRESAHA.115.307611
- Yan R, Li W, Yin L, et al. Cardiovascular diseases and risk‐factor burden in urban and rural communities in high‐, middle‐, and low‐income regions of china: a large community‐based epidemiological study. J Am Heart Assoc. 2017;6(2). doi:10.1161/JAHA.116.004445
- Xi B, Liu F, Hao Y, Dong H, Mi J. The growing burden of cardiovascular diseases in China. Int J Cardiol. 2014;174(3):736–737. doi:10.1016/j.ijcard.2014.04.098
- Yang G, Wang Y, Zeng Y, et al. Rapid health transition in China, 1990–2010: findings from the Global Burden of Disease Study 2010. Lancet. 2013;381(9882):1987–2015. doi:10.1016/S0140-6736(13)61097-1
- Gimbrone MA, García-Cardeña G. Endothelial cell dysfunction and the pathobiology of atherosclerosis. Circ Res. 2016;118(4):620–636. doi:10.1161/CIRCRESAHA.115.306301
- Ali L, Schnitzler JG, Kroon J. Metabolism. Curr Opin Lipidol. 2018;29(6):474–480. doi:10.1097/MOL.0000000000000550
- Brown RA, Shantsila E, Varma C, Lip GYH. Current understanding of atherogenesis. Am J Med. 2017;130(3):268–282. doi:10.1016/j.amjmed.2016.10.022
- He B, Nie Q, Wang F, et al. Role of pyroptosis in atherosclerosis and its therapeutic implications. J Cell Physiol. 2021;236(10):7159–7175. doi:10.1002/jcp.30366
- Jia C, Chen H, Zhang J, et al. Role of pyroptosis in cardiovascular diseases. Int Immunopharmacol. 2019;67:311–318. doi:10.1016/j.intimp.2018.12.028
- Zhaolin Z, Guohua L, Shiyuan W, Zuo W. Role of pyroptosis in cardiovascular disease. Cell Prolif. 2019;52(2):e12563. doi:10.1111/cpr.12563
- Shi J, Gao W, Shao F. Pyroptosis: gasdermin-mediated programmed necrotic cell death. Trends Biochem Sci. 2017;42(4):245–254. doi:10.1016/j.tibs.2016.10.004
- Xue Y, Enosi Tuipulotu D, Tan WH, Kay C, Man SM. Emerging activators and regulators of inflammasomes and pyroptosis. Trends Immunol. 2019;40(11):1035–1052. doi:10.1016/j.it.2019.09.005
- Vande Walle L, Lamkanfi M. Pyroptosis. Curr Biol. 2016;26(13):R568–R572. doi:10.1016/j.cub.2016.02.019
- Hoseini Z, Sepahvand F, Rashidi B, Sahebkar A, Masoudifar A, Mirzaei H. NLRP3 inflammasome: its regulation and involvement in atherosclerosis. J Cell Physiol. 2018;233(3):2116–2132. doi:10.1002/jcp.25930
- Bai B, Yang Y, Wang Q, et al. NLRP3 inflammasome in endothelial dysfunction. Cell Death Dis. 2020;11(9):776. doi:10.1038/s41419-020-02985-x
- Duewell P, Kono H, Rayner KJ, et al. NLRP3 inflammasomes are required for atherogenesis and activated by cholesterol crystals. Nature. 2010;464(7293):1357–1361. doi:10.1038/nature08938
- Christ A, Günther P, Lauterbach MAR, et al. Western diet triggers NLRP3-dependent innate immune reprogramming. Cell. 2018;172(1–2):162–175.e14. doi:10.1016/j.cell.2017.12.013
- He X, Fan X, Bai B, Lu N, Zhang S, Zhang L. Pyroptosis is a critical immune-inflammatory response involved in atherosclerosis. Pharmacol Res. 2021;165:105447. doi:10.1016/j.phrs.2021.105447
- Xu YJ, Zheng L, Hu YW, Wang Q. Pyroptosis and its relationship to atherosclerosis. Clin Chim Acta. 2018;476:28–37. doi:10.1016/j.cca.2017.11.005
- He Y, Hara H, Núñez G. Mechanism and regulation of NLRP3 inflammasome activation. Trends Biochem Sci. 2016;41(12):1012–1021. doi:10.1016/j.tibs.2016.09.002
- Jin H, Ko YS, Park SW, Kim HJ. P2Y2R activation by ATP induces oxLDL-mediated inflammasome activation through modulation of mitochondrial damage in human endothelial cells. Free Radic Biol Med. 2019;136:109–117. doi:10.1016/j.freeradbiomed.2019.04.004
- Zhou R, Yazdi AS, Menu P, Tschopp J. A role for mitochondria in NLRP3 inflammasome activation. Nature. 2011;469(7329):221–225. doi:10.1038/nature09663
- Liu Q, Zhang D, Hu D, Zhou X, Zhou Y. The role of mitochondria in NLRP3 inflammasome activation. Mol Immunol. 2018;103:115–124. doi:10.1016/j.molimm.2018.09.010
- Herzig S, Shaw RJ. AMPK: guardian of metabolism and mitochondrial homeostasis. Nat Rev Mol Cell Biol. 2018;19(2):121–135. doi:10.1038/nrm.2017.95
- Gao F, Chen J, Zhu H. A potential strategy for treating atherosclerosis: improving endothelial function via AMP-activated protein kinase. Sci China Life Sci. 2018;61(9):1024–1029. doi:10.1007/s11427-017-9285-1
- Tian L, Cao W, Yue R, et al. Pretreatment with Tilianin improves mitochondrial energy metabolism and oxidative stress in rats with myocardial ischemia/reperfusion injury via AMPK/SIRT1/PGC-1 alpha signaling pathway. J Pharmacol Sci. 2019;139(4):352–360. doi:10.1016/j.jphs.2019.02.008
- Su X, Yao Z, Li S, Sun H. Synergism of Chinese herbal medicine: illustrated by Danshen compound. Evid Based Complement Altern Med. 2016;2016:1–10. doi:10.1155/2016/7279361
- Wu TW, Zeng LH, Fung KP, et al. Effect of sodium tanshinone IIA sulfonate in the rabbit myocardium and on human cardiomyocytes and vascular endothelial cells. Biochem Pharmacol. 1993;46(12):2327–2332. doi:10.1016/0006-2952(93)90624-6
- Xu H, Ji Chen K. Integrating traditional medicine with biomedicine towards a patient-centered healthcare system. Chin J Integr Med. 2011;17(2):83–84. doi:10.1007/s11655-011-0641-2
- Shang Q, Xu H, Huang L. Tanshinone IIA: a promising natural cardioprotective agent. Evid Based Complement Altern Med. 2012;2012:1–7. doi:10.1155/2012/716459
- Zhang D, Lu X, He S, et al. Sodium tanshinone IIA sulfonate protects against Aβ‐induced cell toxicity through regulating Aβ process. J Cell Mol Med. 2020;24(6):3328–3335. doi:10.1111/jcmm.15006
- Chen L, He W, Peng B, et al. Sodium Tanshinone IIA sulfonate improves post-ischemic angiogenesis in hyperglycemia. Biochem Biophys Res Commun. 2019;520(3):580–585. doi:10.1016/j.bbrc.2019.09.106
- Zhou X, Pan Y, Wang Y, et al. Tanshinones induce tumor cell apoptosis via directly targeting FHIT. Sci Rep. 2021;11(1):12217. doi:10.1038/s41598-021-91708-z
- Cheng Y, An Q, Wang J, Wang Y, Dong J, Yin J. RasGRF1 participates in the protective effect of tanshinone IIA on depressive like behaviors of a chronic unpredictable mild stress induced mouse model. Gene. 2020;754:144817. doi:10.1016/j.gene.2020.144817
- Yang N, Chen H, Gao Y, et al. Tanshinone IIA exerts therapeutic effects by acting on endogenous stem cells in rats with liver cirrhosis. Biomed Pharmacother. 2020;132:110815. doi:10.1016/j.biopha.2020.110815
- Yang G, Jia L, Wu J, et al. Effect of tanshinone IIA on oxidative stress and apoptosis in a rat model of fatty liver. Exp Ther Med. 2017. doi:10.3892/etm.2017.5162
- Wang N, Zhang X, Ma Z, et al. Combination of tanshinone IIA and astragaloside IV attenuate atherosclerotic plaque vulnerability in ApoE(-/-) mice by activating PI3K/AKT signaling and suppressing TRL4/NF-κB signaling. Biomed Pharmacother. 2020;123:109729. doi:10.1016/j.biopha.2019.109729
- Zhu H, Chen Z, Ma Z, et al. Tanshinone IIA protects endothelial cells from H 2 O 2 -induced injuries via PXR activation. Biomol Ther. 2017;25(6):599–608. doi:10.4062/biomolther.2016.179
- Zhu J, Xu Y, Ren G, et al. Tanshinone IIA Sodium sulfonate regulates antioxidant system, inflammation, and endothelial dysfunction in atherosclerosis by downregulation of CLIC1. Eur J Pharmacol. 2017;815:427–436. doi:10.1016/j.ejphar.2017.09.047
- Qun Jia L, Lin Yang G, Ren L, et al. Tanshinone IIA reduces apoptosis induced by hydrogen peroxide in the human endothelium-derived EA.hy926 cells. J Ethnopharmacol. 2012;143(1):100–108. doi:10.1016/j.jep.2012.06.007
- He L, Liu YY, Wang K, et al. Tanshinone IIA protects human coronary artery endothelial cells from ferroptosis by activating the NRF2 pathway. Biochem Biophys Res Commun. 2021;575:1–7. doi:10.1016/j.bbrc.2021.08.067
- Chen HW, Yen CC, Kuo LL, et al. Benzyl isothiocyanate ameliorates high-fat/cholesterol/cholic acid diet-induced nonalcoholic steatohepatitis through inhibiting cholesterol crystal-activated NLRP3 inflammasome in Kupffer cells. Toxicol Appl Pharmacol. 2020;393:114941. doi:10.1016/j.taap.2020.114941
- Rajamäki K, Lappalainen J, Öörni K, et al. Cholesterol crystals activate the NLRP3 inflammasome in human macrophages: a novel link between cholesterol metabolism and inflammation. PLoS One. 2010;5(7):e11765. doi:10.1371/journal.pone.0011765
- Ma X, Lu D, Liu Y, et al. Multiplexed quantitative evaluation on mitochondrial toxicity of tris (2,3-dibromopropyl) phosphate in hepatocyte. Ecotoxicol Environ Saf. 2021;221:112425. doi:10.1016/j.ecoenv.2021.112425
- Le Y, Shen H, Yang Z, Lu D, Wang C. Comprehensive analysis of organophosphorus flame retardant-induced mitochondrial abnormalities: potential role in lipid accumulation. Environ Pollut. 2021;274:116541. doi:10.1016/j.envpol.2021.116541
- Liu X, Xu Y, Cheng S, et al. Geniposide combined with notoginsenoside R1 attenuates inflammation and apoptosis in atherosclerosis via the AMPK/mTOR/Nrf2 signaling pathway. Front Pharmacol. 2021;12. doi:10.3389/fphar.2021.687394
- Xie T, Wang C, Jin Y, et al. CoenzymeQ10-induced activation of AMPK-YAP-OPA1 pathway alleviates atherosclerosis by improving mitochondrial function, inhibiting oxidative stress and promoting energy metabolism. Front Pharmacol. 2020;11. doi:10.3389/fphar.2020.01034
- Zhou G, Myers R, Li Y, et al. Role of AMP-activated protein kinase in mechanism of metformin action. J Clin Invest. 2001;108(8):1167–1174. doi:10.1172/JCI13505
- Ming Li Z, Wen Xu S, Qing Liu P. Salvia miltiorrhizaBurge (Danshen): a golden herbal medicine in cardiovascular therapeutics. Acta Pharmacol Sin. 2018;39(5):802–824. doi:10.1038/aps.2017.193
- Amaya-Montoya M, Pinzón-Cortés JA, Silva-Bermúdez LS, et al. ApoE and apoC-III-defined HDL subtypes: a descriptive study of their lecithin cholesterol acyl transferase and cholesteryl ester transfer protein content and activity. Lipids Health Dis. 2020;19(1):106. doi:10.1186/s12944-020-01291-x
- Li YH, Tseng WK, Yin WH, et al. Prognostic effect of high-density lipoprotein cholesterol level in patients with atherosclerotic cardiovascular disease under statin treatment. Sci Rep. 2020;10(1):21835. doi:10.1038/s41598-020-78828-8
- Khera AV, Demler OV, Adelman SJ, et al. Cholesterol efflux capacity, high-density lipoprotein particle number, and incident cardiovascular events. Circulation. 2017;135(25):2494–2504. doi:10.1161/CIRCULATIONAHA.116.025678
- Qian Z, Zhao Y, Wan C, et al. Pyroptosis in the initiation and progression of atherosclerosis. Front Pharmacol. 2021;12. doi:10.3389/fphar.2021.652963
- Swanson KV, Deng M, Ting JPY. The NLRP3 inflammasome: molecular activation and regulation to therapeutics. Nat Rev Immunol. 2019;19(8):477–489. doi:10.1038/s41577-019-0165-0
- Zhao Y, Shi J, Shao F. Inflammatory caspases: activation and cleavage of gasdermin-D in vitro and during pyroptosis. Methods Mol Biol. 2018;131–148. doi:10.1007/978-1-4939-7519-8_9
- Yang M, Lv H, Liu Q, et al. Colchicine alleviates cholesterol crystal-induced endothelial cell pyroptosis through activating AMPK/SIRT1 pathway. Oxid Med Cell Longev. 2020;2020:1–18. doi:10.1155/2020/9173530
- Abdul-Muneer PM, Alikunju S, Mishra V, et al. Activation of NLRP3 inflammasome by cholesterol crystals in alcohol consumption induces atherosclerotic lesions. Brain Behav Immun. 2017;62:291–305. doi:10.1016/j.bbi.2017.02.014
- Bock FJ, Tait SWG. Mitochondria as multifaceted regulators of cell death. Nat Rev Mol Cell Biol. 2020;21(2):85–100. doi:10.1038/s41580-019-0173-8
- Qi D, Young LH. AMPK: energy sensor and survival mechanism in the ischemic heart. Trends Endocrinol Metab. 2015;26(8):422–429. doi:10.1016/j.tem.2015.05.010
- Wu S, Zou MH. AMPK, mitochondrial function, and cardiovascular disease. Int J Mol Sci. 2020;21(14):4987. doi:10.3390/ijms21144987