Abstract
Sepsis is a disorder of the immune response to infection or infectious factors with high morbidity and mortality in clinical settings. The lactylation of lysine residues, fueled by lactate, plays a pivotal role in its pathophysiology. In conducting a literature review on sepsis-related research, we employed a systematic approach to ensure comprehensiveness and accuracy. Initially, we conducted an extensive literature search through the PubMed database, utilizing a range of keywords including “sepsis”, “lactate”, “lactylation”, and “epigenetic modification”. The aim was to capture the most recent research related to the pathophysiological mechanisms of sepsis, metabolic disorders, and the role of lactylation. The results of the literature review revealed a close link between sepsis and metabolic dysfunction, particularly the pivotal role of lactylation in regulating immune responses and inflammatory processes. Lactate, not only an energy metabolic byproduct produced during glycolysis, affects the activity of various proteins, including those involved in immune regulation and cell signaling, through lactylation. In the context of sepsis, changes in the levels of lactylation may be closely associated with the severity and prognosis of the disease. In summary, lactylation, as an emerging type of epigenetic modification, provides a new perspective for the diagnosis and treatment of sepsis. Future research needs to further elucidate the exact mechanisms of lactylation in sepsis and explore its potential as a therapeutic target.
Plain Language Summary
The annual incidence and mortality rates associated with sepsis are on the rise, and to date, no medications or therapies have been proven effective in clinical practice. Glycolysis plays a pivotal role in regulating lactylation, a process derived from lactate generated by cellular glucose metabolism. In the context of sepsis, elevated lactate levels are indicative of a poor prognosis. It is imperative to delve into the mechanisms underlying lactylation alterations during sepsis to enhance our comprehension of its complex pathophysiology and to pinpoint innovative therapeutic targets for the condition.
Introduction
The Third International Consensus Task Force defines sepsis as a “life-threatening organ dysfunction caused by a dysregulated host response to infection”.Citation1 Sepsis is a leading cause of morbidity and the leading cause of death in clinically critical patients. In 2017, an analysis of the Global Burden of Disease Survey reported approximately 48.9 million cases of sepsis worldwide, with11.0 million deaths.Citation2 Because of the complicated pathophysiological basis of sepsis, there is still no a viable treatment to increase patient survival.Citation3 The Surviving Sepsis Campaign guidelines’ recommendations for the clinical management of sepsis are mostly all-encompassing and include the use of antibiotics to treat the underlying infection, fluid resuscitation, etc.Citation4,Citation5 Sepsis involves an initial inflammatory response followed by immune suppression, leading to increased risk of secondary infections and potential multi-organ failure, which are major contributors to its high mortality rate.Citation6 Effective management of this immune dysregulation is critical for improving patient survival.
Epigenetic alterations are intimately linked with the progression of sepsis, spanning from host-pathogen interactions to the acute inflammatory phase and subsequent immune suppression.Citation7 With advancements in genomics, epigenetics has shown promise in the diagnosis and prognosis of sepsis.Citation8 DNA methylation and post-translational protein modifications are two of the most prevalent types of epigenetic changes.Citation9,Citation10 Notably, lactylation has emerged as a novel form of post-translational protein modification. This process involves the accumulation of lactate from cellular metabolism altering lysine residues on histones. Specifically, lactate produced through aerobic glycolysis is implicated in modulating inflammation and immune suppression in sepsis.Citation11–14
Clinically, capillary lactate (CL) and serum lactate (SL) serve as prognostic indicators for mortality within 48 hours and 7 days, respectively.Citation15 These markers enable emergency physicians to rapidly and accurately identify sepsis patients at a higher risk of death, facilitating appropriate prognostic assessments and aiding in the management and diagnosis of sepsis. Furthermore, lactyl-histones have been recognized as potential biomarkers for sepsis diagnosis and outcome prediction.Citation16 Building on this foundation, we propose that lactate and its modifications as a product of glycolysis may be key factors in regulating immune function in sepsis patients.
The Pathophysiological Mechanism of Sepsis
Sepsis has a complicated pathophysiological process and pathogenesis that includes an imbalanced inflammatory response, endothelial dysfunction, mitochondrial damage, abnormal coagulation, and other pathophysiological processes that ultimately result in multiple organ failures in the body.Citation17 The early phase of sepsis is typified by a hyper-inflammatory state, complete with a robust cytokine storm. Toll-like receptors (TLRs), a class of pattern recognition receptors, release a barrage of pro-inflammatory cytokines, chemokines, interferons, and reactive oxygen species in response to pathogen-associated molecular patterns (PAMPs) and damage-associated molecular patterns (DAMPs), thereby instigating acute inflammation.Citation18 Hotchkiss et al have posited that even patients who survive the initial inflammatory storm may continue to experience persistent immunosuppression in the later stages of sepsis, predisposing them to secondary infections and increasing their risk of mortality.Citation19 Sepsis-induced immunosuppression (closely related to innate and adaptive immunity) is considered one of the major causes of death in patients with sepsis.Citation6 During the course of sepsis, inflammatory and immunosuppressive processes may occur in tandem or sequentially. The immune system’s swift elimination of pathogens can reestablish immune homeostasis in the early stages of the systemic inflammatory response. However, a failure to promptly clear pathogens can result in an imbalanced immune response, an overproduction of anti-inflammatory mediators, and the development of a compensatory anti-inflammatory response syndrome.Citation20 This leads to the suppression of immune function and a heightened vulnerability to infections.Citation1 The immunosuppressive phase is marked by a decrease in CD4 lymphocytes, diminished B-lymphocyte function, reduced expression of HLA-DR on monocytes, and a dominance of anti-inflammatory Th2 and Treg responses over pro-inflammatory Th1 and T17 responses, as well as impaired neutrophil phagocytosis.Citation20 Consequently, sepsis should not be simplistically categorized as either a pro-inflammatory or an anti-inflammatory condition but rather as a dynamic interplay between inflammation and immunosuppression.
Sepsis and Metabolic Disorders
The cause of sepsis organ dysfunction is not the direct damage caused by pathogens and endotoxin, but the serious immune and metabolic dysfunction caused by host damage when facing infection.Citation21 Therefore, metabolism-associated molecular patterns are thought to be strongly associated with metabolic disorders in sepsis. Systemic and organ-specific metabolic changes that disrupt normal metabolic homeostasis characterize sepsis. Sepsis leads to hypermetabolism, increased energy expenditure, and the development of insulin resistance, which is clinically characterized by hyperglycemia, hyper-lactylation, and increased proteolytic effects.Citation22 Mitochondria are bioenergetic organelles and sepsis-induced mitochondrial damage or dysfunction is a major cause of cellular metabolic disorders, inadequate energy supply, and oxidative stress, which leads to organ cell and immune cell apoptosis and ultimately to immune disorders and multi-organ dysfunction, resulting in increased mortality in patients with sepsis.Citation23
During various stages of sepsis, immune cells are governed by distinct cellular metabolic pathways. Macrophages, as crucial innate immune cells, play a pivotal role in the immune response. The metabolic traits of macrophages vary with their activation state and the phase of metabolism they are in, which has different implications for their immunomodulatory functions.Citation24 Under normal physiological conditions, oxidative phosphorylation of glucose is the predominant metabolic pathway for macrophages. M1 macrophages enhance glucose uptake and aerobic glycolysis, whereas M2 macrophages increase fatty acid uptake and improve the efficiency of oxidative phosphorylation.Citation25 The inflammatory response engages multiple cell types, including pro-inflammatory cells like M1 macrophages, Th1, and Th17 lymphocytes, which produce lactate through glycolysis to propagate inflammation. Regulatory T cells and M2 macrophages contribute to immunomodulation and anti-inflammatory actions via fatty acid oxidation in the tricarboxylic acid cycle.Citation16,Citation26 Glycolysis is essential for immune cell activation but also contributes to immunosuppression in sepsis, being upregulated in hyper-inflammatory cells and downregulated in immunosuppressive cells.Citation27
With the development of sepsis, the early stage of high inflammation transits to the late stage of low inflammatory immunosuppression. At present, there is no specific stage of treatment for sepsis.Citation4,Citation15 While clinical trials have historically concentrated on the inflammatory aspect of sepsis with limited success, recent findings suggest that metabolic dysregulation is a significant factor in sepsis pathogenesis, offering new hope for treatment via metabolic intervention. Sepsis triggers a robust metabolic response, characterized by the release of high-energy metabolites such as lactate and free fatty acids.Citation28 Metabolic reprogramming and the strategic targeting of lactate, a key metabolite in this process, may present a promising therapeutic avenue for sepsis management.
Lactate and Sepsis
Glycolysis and Lactate
Glycolysis is the process of decomposition of glucose into pyruvate and lactate, the main metabolic pathway of sepsis. Lactate, a glycolytic product, is generated through both aerobic and anaerobic glycolysis and serves as a vital energy source and a precursor for glucose synthesis.Citation29 In the presence of oxygen, glycolysis converts glucose into pyruvate and ATP; the pyruvate then enters mitochondria, facilitated by pyruvate dehydrogenase, to form acetylCoA. This compound subsequently joins the TCA cycle and oxidative phosphorylation for further energy production.Citation30–32 Conversely, under anaerobic conditions, pyruvate is reduced to lactate with the help of NADH.Citation33 L-lactic dehydrogenase plays a crucial role in lactate production, catalyzing the interconversion of pyruvate and lactate in both hypoxic and aerobic states.Citation34,Citation35
An increasing body of research indicates that lactate participates in the regulation of numerous biological and pathological processes. Hypoxia, inflammation, viral infections, and the tumor microenvironment are known to stimulate lactate production.Citation36 Recent theories regarding lactate’s role include the lactate shuttle hypothesis, lactate homeostasis, and the microenvironmental interactions of lactate in various diseases.Citation37 Lactate also engages in complex interactions with its environment; it activates the G-protein-coupled receptor 81through monocarboxylate transporters or shuttles across different organs, cells, and cell types to generate ATP.Citation38 It fuels cell growth and development, and as a signaling molecule, it influences the functional alterations of intracellular proteins.Citation36,Citation38,Citation39 These multifaceted roles of lactate underscore its importance in both physiological and pathological contexts, particularly in conditions such as sepsis.
Role of Lactate in Sepsis
A retrospective analysis of septic/non-septic patients revealed higher serum lactate levels in septic patients.Citation40 Serum lactate levels greater than 2 mmol/L are identified as septic shock.Citation41 The interaction between blood pressure and lactate was evaluated and found to be nonsignificant (p = 0.67 for the interaction term).Citation42 In a state of septic shock, anaerobic glycolysis caused by tissue hypoxia produces large amounts of lactate, which increases the lactate concentration and even leads to lactylation.Citation43 Serum lactate levels are an important biomarker for sepsis and correlates positively with morbidity and mortality in sepsis or septic shock. Also, in animal models, lactate ≥1.64 mmol/L can act as a potential biomarker to identify sepsis models with cecal ligation and puncture in rats.Citation44 In sepsis clinical treatment, lactate levels and clearance rates are key clinical indicators linked to patient prognosis.Citation45 Elevated lactate reflects tissue hypoperfusion and metabolic disruption. Lactate clearance rate is a measure of hemodynamic improvement and tissue perfusion recovery, with optimized fluid resuscitation and hemodynamics potentially reducing mortality.Citation17
Studies have shown that there metabolic changes occur between the lactate produced by glycolysis and septic endothelial cells. Lactate can lead to vascular permeability and organ dysfunction. Inhibition of lactate production in the body or the elimination of the lactate receptor GPR81 may reduce vascular permeability and multiple organ damage and improve survival in sepsis.Citation46 Interestingly, studies related to immune metabolism have shown that activation of immune cells by aerobic glycolysis and the production of lactate may play an immunosuppressive role in the local environment of innate immunity and inflammation.Citation47 The lipopolysaccharide-induced sepsis tolerance model is considered being a mechanism of immunosuppression, and it was found that lactate levels first increased and then decreased 24 to 48 hours after induction of sepsis.Citation48,Citation49 In another study, inhibition of glycolysis via the PI3K/Akt-HIF-1α pathway mediated the downregulation of lactate dehydrogenase resulting in immunosuppression of neutrophils during sepsis and providing a therapeutic target for improving immune function in sepsis.Citation34,Citation50
Lactate is closely related to glycolysis, which involves a variety of enzymes and complexes that play a role in the regulation of sepsis (). The pyruvate dehydrogenase complex is a multienzyme complex of a glycolytic metabolic pathway.Citation51 Protective mechanisms of PDHC in sepsis include regulation of lactate balance, release of inflammatory mediators, remodeling of the TCA cycle, and enhancement of lipid and energy metabolism.Citation52 Activation of PDHC with dichloroacetate has been shown to mitigate sepsis-induced endothelial dysfunction by inhibiting lactate formation.Citation51 By knocking down pyruvate kinase isoenzymes M2, hypoxia-inducible factor 1α and glycolysis-related genes.Citation53–55 Shikonin, a PKM2 inhibitor, lowered serum lactate levels and high mobility group protein 1 release in septic mice.Citation44,Citation56 Shikonin administration has also been shown to decrease HMGB1 release, lactate generation, and septic mouse mortality in Adenosine 5‘-monophosphate -activated protein kinase -deficient mice.Citation57,Citation58 A discovery by Ding et al showed that Lycium barbarum polysaccharide plays a role in inhibiting LPS-induced inflammation by enhancing ubiquitination and downregulation of PKM2 by altering the glycolytic pathway and M1 differentiation in macrophages.Citation53 Lactate also exerts anti-inflammatory effects in macrophages, reducing the production of IL-6 and TNF-α and curbing NF-κB and YAP activation.Citation59 Moreover, 2-deoxy-D-glucose, an aerobic glycolysis inhibitor, alleviates sepsis-induced acute kidney injury by promoting autophagy via the lactate/SIRT3/AMPK pathway.Citation60–62
Table 1 Role of Lactate and Aerobic Glycolysis in Sepsis
Research indicates that modulating lactate production through glycolytic pathways holds promise for sepsis treatment.Citation64 Lactate’s role extends to histone lactylation, influencing cancer, inflammation, and pulmonary fibrosis, and impacting immune regulation and homeostatic maintenance.Citation65 Lactate acts as a double-edged sword and can play different roles depending on the metabolic state, cell type, and pathological process. On the one hand, activated immune cells rely on the use of lactate to support their function. On the other hand, lactate that accumulates in the tissue microenvironment acts as a signaling molecule that restricts f immune cell function. Recently, researchers have proposed a new line of research for lactate, namely the lactylation of lysine residues mediated by lactate as a substrate.Citation66
Lactylation
Discovery of Lactylation
The notion of lactylation, introduced by Professor Zhao from the University of Chicago in 2019, distinguishes between histone and non-histone lactylation. Research has predominantly concentrated on the latter, with fewer studies exploring lactylation in non-histone proteins. It is recognized that lactate, a byproduct of glycolysis, can lactylate lysine residues on histones through lactyl-coenzyme A, with L-lactate specifically promoting this modificationCitation39,Citation67 (). However, lactylation in non-histones was initially identified only in plant pathogenic fungi, and its occurrence and downstream function in other organisms are still unclear.Citation68 Recently, Wan et al identified novel lactylation sites on both histones and non-histone proteins, utilizing fragment ions as a diagnostic marker for lactylation and employing proteomic data. Their findings provide preliminary evidence for lactylation on human non-histone proteins, such as the metabolic enzyme aldolase A, which has significant regulatory implications.Citation69 Lactylation is regulated by enzymes and non-enzymes. Enzymatic regulation mainly influences inflammatory homeostasis via the inflammatory pathway; non-enzymatic regulation influences the covalently modified glycolytic pathway, inhibits enzyme activity and reduces metabolites of glycolysis.Citation65 The study also found that glycolytic inhibitors not only inhibit the production of lactate, but also reduce lactylation. Mitochondrial inhibitors or hypoxia conditions increase production of lactate and promote lactylation, which is involved in the homeostatic regulation of M1 macrophages infected by bacteria, inflammation, cancer, and other diseases.Citation70 So far, the discovery of lactylation has given a new direction to the study of metabolite lactate in tumors, immunity and other fields.
Figure 1 The role of lactylation in sepsis. Lactate shuttles through organs, cells, and cells through monocarboxylate transporterCitation32 and G protein-coupled receptor 81.Citation71 Under the conditions of aerobic or anoxic and bacterial stimulation, the lactate produced by glycolysis catalyzes the production of lactyl-CoA, and the lactate is transferred to histone through a writerCitation38 or an eraserCitation72 and participates in the lactylation of lysine residues. PI3K-regulated B-cell adapterCitation12 regulates FOXO1 and GSK3β. Reducing histone lactylation affects the expression of damage repair genes, and affects the transformation of inflammatory macrophages to repair macrophages. Lactylation of histone H3K18 promotes the expression of damage repair gene Arg1 and polarization to M2-type macrophages.
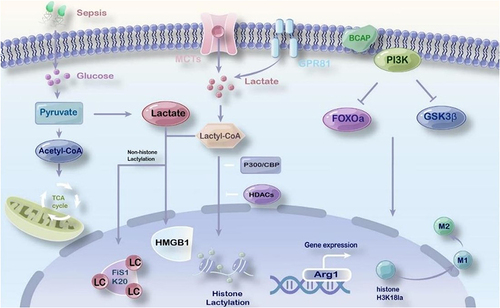
Histone Lactylation Sites
In 2019, Zhang et al identified 26 histone lactylation sites in human HeLa cells and 18 in mouse macrophages using mass spectrometry, revealing the importance of this epigenetic modification in gene regulation.Citation63 Subsequent studies found histone lactylation sites in gastric cancer cells, HEK 293 cells, and the fungus Botrytis cinerea, underscoring its prevalence and potential roles in diverse biological contexts.Citation68,Citation73–75 H3K18la, a specific lactylation site, has been linked to the advancement of sepsis, colon cancer, and ocular melanoma by influencing the expression of genes like METTL3 and YTHDF2, which are involved in methylation and mRNA decay, respectively.Citation76–78
Non-Histone Lactylation Sites
However, due to the limitations of current methods for analyzing lactylated proteins, the substrate range of lactylated proteins, especially the substrate range of non-histones, cannot be determined. Sun et al utilized an innovative approach with an alkynyl-functionalized bioorthogonal chemical reporter, YnLac, to conduct proteomic analysis in mammalian cells. This method allowed for the identification of two previously unknown histone lactylation sites, H2AK4 and H2AK9, and the discovery of four novel lactylation sites on non-histone proteins: HMGN1, NPM1, PDCD4, and PHF3.Citation79 This work underscores the potential of innovative bioorthogonal chemistry tools in expanding our understanding of lactylation’s role in cellular processes. Recently, Yang et al reported 9275 lactylation sites in hepatocellular carcinoma, of which 9256 were found on non-histones. And they showed that lactylation at K28 on the non-histone AK2 can lead to energy disturbances and cell deterioration in HCC.Citation80,Citation81 In summary, lactylation is an emerging post-translational modification with wide-ranging effects on both histone and non-histone proteins. The identification of lactylation sites on non-histone proteins in various cancers and the functional implications of these modifications are crucial for understanding cell metabolism and function, and they may offer new targets for therapeutic intervention.
“Writer” and “Eraser”
Protein PTMs, including lactylation, play a vital role in epigenetic regulation, with specific enzymes acting as “writers” and “erasers” to control cellular functions.Citation82,Citation83 Zhang et al found that p300 can promote lactylation, while HDAC1-3 can remove it, along with other modifications.Citation16,Citation55,Citation73,Citation84 Moreno Yruela et al demonstrated the roles of HDACs and SIRT1-3 in delactylation, with varying efficiency in removing different lysine marks.Citation71,Citation85,Citation86 Sun et al identified Sirtuin1 as a potential delactylase.Citation87 And Dong et al discovered YiaC and CobB as regulators of lactylation in prokaryotes.Citation88 These studies collectively advance our understanding of the enzymatic regulation of lactylation.
Signaling Pathways Related to Lactylation
Lactate signaling and lactylation are implicated in numerous pathways and processes, linking to diseases such as cancer, inflammation, and immune-related disorders ().Citation64 Under hypoxia, lactate accumulation can stabilize NDRG3, influencing Raf-ERK signaling for cell growth and angiogenesis.Citation89 Chen et al reported that hypoxia-induced mROS inhibited HIF-1α hydroxylation and triggered glycolytic conversion in hypoxic pulmonary artery smooth muscle cells via the upregulated HIF-1α/PDK1&2/p-PDH-E1α axis, thereby promoting lactate accumulation and histone lactylation.Citation31 Second, lactate has been shown to be associated with tumor progression. Lactate regulates cellular metabolic processes in non-small cell lung cancer by downregulating glycolytic enzymes and upregulating mRNA levels of TCA cycle enzymes, increasing histone lactylation, and mediating gene expression.Citation26 Von Hippel-Lindau, which is associated with metabolic reprogramming, has been reported to stimulate histone lactylation through activation of platelet-derived growth factor receptor β (PDGFRβ) and promote clear cell carcinoma (ccRCC) progression.Citation89,Citation90 Tumor progression of hepatocellular carcinoma stem cells was found to be inhibited by inhibition of histone H3K9la and H3K56la modification site.Citation80 However, Yang et al found that lactylation at non-histone K28 promotes the proliferation and metastasis of HCC cells by inhibiting the function of adenylate kinase 2.Citation81 In addition, Yu et al found that lactylation of histone H3K18 recognized m6A modification and promoted the expression of YTH N6-methyladenosine RNA binding protein 2, thereby accelerating tumorigenesis in ocular melanoma.Citation78 Similarly, Xiong et al reported that l METTL3-mediated RNA m6A modification induced by lactylation promoted immunosuppression of the tumor immunological microenvironment.Citation2,Citation77 Gu et al found that the tumor metabolite lactate promotes tumorigenesis by regulating MOESIN lactylation and enhancing transforming growth factor-β (TGF-β) signaling in Treg cells.Citation91 In inflammation, lactate promotes histone lactylation and pro-fibrotic gene expression, while in Alzheimer’s disease, it influences microglial function and cognitive abilities.Citation92,Citation93 These findings highlight lactate’s role in epigenetic regulation and suggest that targeting lactate-related signaling could offer novel therapeutic strategies for various diseases.
Table 2 Lactylation-Related Signaling Pathways
Lactylation and Sepsis
Inflammation and Infection
Recent data suggest metabolic dysregulation occurs in sepsis and targeting these pathways could be a therapeutic target for sepsis.Citation28 During sepsis, the host and pathogen trigger a robust anti-infection response. This leads to reduced tissue perfusion, edema induction, decreased oxygen transport between cells, increased in oxygen consumption by immune cells in inflammatory tissues and cell hypoxia. Additionally, lactate produced by aerobic glycolysis in hypoxia conditions or because of bacterial stimulation has been found to influence the activation of macrophages and the expression of specific genes, relying on certain signaling pathways.Citation94 Furthermore, B-cell adaptor deletion regulated by Phosphoinositide 3-kinase has been linked to defective aerobic glycolysis and reduced lactate production, affecting gene expression and the transformation of inflammatory macrophages into reparative ones.Citation71,Citation95 Histone lactylation has been observed in both healthy individuals and critically ill patients. H3K18 has been identified as a potential biomarker for diagnosing and predicting the severity of septic shock, as it mediates inflammatory cytokine expression, promotes Arg1 overexpression, and stimulates the anti-inflammatory function of macrophages in sepsis.Citation96 Ma et al conducted a study using a mouse model of peritonitis infection to evaluate the impact of methylxanthoylmethane on methicillin-resistant Staphylococcus aureus infection.Citation76 Their findings suggest that methylxanthoylmethane promotes Arg1 expression via H3K18 lactylation to control macrophage polarization toward M2, offering potential therapeutic benefits for drug-resistant infections and sepsis.Citation71,Citation76
Organ Dysfunction
From the perspective of pathogenesis, sepsis is associated with inflammatory disorder, disseminated intravascular coagulation, and organ dysfunction. Clinically, lactate levels and high mobility group protein-1 are frequently used as biomarkers and are associated with sepsis sepsis severity and mortality.Citation76 Yang et al found that lactate contributes to increased vascular permeability and worsens organ dysfunction in CLP sepsis. Vascular permeability and organ damage could be mitigated by inhibiting lactate production and depleting of the lactate receptor GPR81 in vivo.Citation97 Additionally, the research team also found that the extracellular lactate uptake by MCT promoted the lactylation/acetylation of HMGB1 through p300/CBP-dependent pathway and GPR81. The modified HMGB1 is translocated from the nucleus to the cytoplasm, released into the circulatory system, resulting in endothelial barrier dysfunction and promoting sepsis development.Citation38,Citation46 Therefore, inhibiting lactylated HMGB1 and exosomal release may be a therapeutic target for sepsis. The results presented in highlight the significant role of lactylation in the occurrence and development of sepsis, expanding our understanding of lactylation’s biological function. Seng et al’s study reveals a novel mechanism between lactate and organ damage in sepsis, indicating that septic kidney injury will be lessened by decreased levels of non-histone mitochondrial fission protein 1 lactylation.Citation72,Citation98
Immune Response
An innovative potential treatment for reducing inflammation involves regulating cell metabolism, leading to the repolarization of inflammatory immune cells in an anti-inflammatory manner. Strict regulation of the metabolic pathway in immune cells is crucial to maintaining immunological balance and proper functional specialization.Citation98 Innate immune cells play a role in modulating the immune response during sepsis. For instance, dysfunction in macrophages and dendritic cells can impair the body’s ability to respond to initial infections and weaken the defense against secondary hospital-acquired infections.Citation99 Immune tolerance to infections is a characteristic of the immunosuppressive stage of sepsis, during which dendritic cell transition to a tolerant phenotype and promote the development of regulatory T cells to suppress the immune system.Citation100 Recent studies have shown that exogenous lactate can induce a shift in metabolism and induce innate immune cells to assume a tolerant phenotype.Citation101,Citation102 Myeloid-derived suppressor cells are immunosuppressive immune cells that play a role in suppressing the immune system during sepsis by restricting T cell activity and promoting Treg development.Citation103,Citation104 The development of MDSC and immunosuppression is triggered by an increase in glycolysis and lactate concentration. In order to regulate cell activity and maintain homeostasis, T cells monitor the concentration of extracellular lactate and initiate intracellular signal transduction.Citation105 Zhang et al discovered that LPS-activated M1 macrophages increased lactate concentration through glycolysis and promoted histone lactylation, leading to increased expression of Arg1 and other genes related to wound healing, indicating a phenotypic shift to immunosuppressive M2 macrophages.Citation34
Discussion
Sepsis is a severe systemic inflammatory syndrome usually caused by infection and characterized by metabolic reprogramming of immune cells, in particular by abnormal activation of glycolytic pathways under aerobic conditions. This metabolic reprogramming leads to increased glucose consumption, increased lactate secretion and increased inflammation levels, which in turn affects the patient’s prognosis. Lactate, a metabolic byproduct of cellular glycolysis, has attracted considerable attention due to its role in the Warburg effect within the tumor microenvironment. Research has increasingly implicated lactate in the development of sepsis, where it correlates with the severity of mortality and prognosis. Although the pathophysiological mechanisms of sepsis are not fully elucidated, evidence points to epigenetic processes that modulate the inflammatory response in sepsis, with lactate acting as a substrate for histone lactylation and influencing immune cell function. The identification of lactylation as a novel epigenetic modification has emerged as a critical area of sepsis research. Glycolysis inhibitors such as DCA (dichloroacetic acid), 2-DG and oxamate reduce lactate production by inhibiting key enzymes of the glycolytic pathway.Citation60 By reducing the rate of glycolysis, these inhibitors reduce intracellular lactate levels, which can mitigate the inflammatory response and tissue damage caused by sepsis. Lactylation is an epigenetic modification that involves the lactylation of lysine residues on proteins. The extent and pattern of lactylation can change during sepsis and influence the function of immune cells and the regulation of inflammatory responses. The extent of lactylation can be regulated by influencing lactylation modifying enzymes such as p300/CBP, HDACs and sirtuins (deacetylases).Citation12,Citation85 By regulating the activity of these enzymes, the balance of lactylations can be influenced, which in turn can improve the immune response and inflammatory state in sepsis patients. Further research is needed to clarify the upstream and downstream regulatory pathways of these lactylation enzymes. Employing proteomics in tandem with genomics and metabolomics to monitor proteomic alterations and the differential expression of identified proteins in sepsis may offer a comprehensive view of the pathophysiological mechanisms at play. For instance, interventions that reduce the lactylation of HMGB1 and Fisi1 have shown promise in ameliorating organ dysfunction and acute kidney injury in sepsis. Current research has predominantly focused on core histones such as H3, H4, H2A, and H2B, with a relative paucity of studies on non-histone proteins. Further investigation into additional histone lactylation sites and non-histone lactylation sites are essential for advancing our understanding in this area.
Conclusion
In summary, targeting glycolysis inhibition, modulating lactylation enzymes and and discovering new histone lactylation sites and non-histone lactylation sites presents a promising therapeutic strategy to improve the prognosis of sepsis patients. These approaches have the potential to offer new perspectives and methods for the treatment of sepsis by regulating the metabolic state and inflammatory response of immune cells. However, further research and validation are required to ensure the safety and efficacy of these treatment strategies in clinical applications. In the future, these strategies may become an integral part of sepsis treatment, providing patients with a broader range of therapeutic options.
Author Contributions
All authors made a significant contribution to the work reported, whether that is in the conception, study design, execution, analysis and interpretation, or in all these areas; took part in drafting, revising or critically reviewing the article; gave final approval of the version to be published; have agreed on the journal to which the article has been submitted; and agree to be accountable for all aspects of the work.
Disclosure
The authors declare that the research was conducted in the absence of any commercial or financial relationships that could be construed as a potential conflict of interest.
Additional information
Funding
References
- Singer M, Deutschman CS, Seymour CW, et al. The Third International Consensus Definitions for Sepsis and Septic Shock (Sepsis-3). JAMA. 2016;315(8):801–810. doi:10.1001/jama.2016.0287
- Rudd KE, Johnson SC, Agesa KM, et al. Global, regional, and national sepsis incidence and mortality, 1990–2017: analysis for the Global Burden of Disease Study. Lancet. 2020;395(10219):200–211. doi:10.1016/S0140-6736(19)32989-7
- Guarino M, Perna B, Cesaro AE, et al. 2023 Update on Sepsis and Septic Shock in Adult Patients: management in the Emergency Department. J Clin Med. 2023;12(9):3188. doi:10.3390/jcm12093188
- Evans L, Rhodes A, Alhazzani W, et al. Surviving sepsis campaign: international guidelines for management of sepsis and septic shock 2021. Intensive Care Med. 2021;47(11):1181–1247. doi:10.1007/s00134-021-06506-y
- Perner A, Gordon AC, De Backer D, et al. Sepsis: frontiers in diagnosis, resuscitation and antibiotic therapy. Intensive Care Med. 2016;42(12):1958–1969. doi:10.1007/s00134-016-4577-z
- Liu D, Huang S-Y, Sun J-H, et al. Sepsis-induced immunosuppression: mechanisms, diagnosis and current treatment options. Mil Med Res. 2022;9(1):56. doi:10.1186/s40779-022-00422-y
- Binnie A, Tsang JLY, Hu P, et al. Epigenetics of Sepsis. Crit Care Med. 2020;48(5):745–756. doi:10.1097/CCM.0000000000004247
- Hornbeck PV, Zhang B, Murray B, et al. PhosphoSitePlus, 2014: mutations, PTMs and recalibrations. Nucleic Acids Res. 2015;43(Database issue):D512–20. doi:10.1093/nar/gku1267
- Chan JC, Maze I. Nothing Is Yet Set in (Hi)stone: novel Post-Translational Modifications Regulating Chromatin Function. Trends Biochem Sci. 2020;45(10):829–844. doi:10.1016/j.tibs.2020.05.009
- Cao X. Self-regulation and cross-regulation of pattern-recognition receptor signalling in health and disease. Nat Rev Immunol. 2016;16(1):35–50. doi:10.1038/nri.2015.8
- Tannahill GM, Curtis AM, Adamik J, et al. Succinate is an inflammatory signal that induces IL-1beta through HIF-1alpha. Nature. 2013;496(7444):238–242. doi:10.1038/nature11986
- Ciarlo E, Savva A, Roger T. Epigenetics in sepsis: targeting histone deacetylases. Int J Antimicrob Agents. 2013;42:S8–12. doi:10.1016/j.ijantimicag.2013.04.004
- Levy B. Lactate and shock state: the metabolic view. Curr Opin Crit Care. 2006;12(4):315–321. doi:10.1097/01.ccx.0000235208.77450.15
- Ganeshan K, Chawla A. Metabolic regulation of immune responses. Annu Rev Immunol. 2014;32(1):609–634. doi:10.1146/annurev-immunol-032713-120236
- Guarino M, Perna B, Cesaro AE, et al. Comparison between Capillary and Serum Lactate Levels in Predicting Short-Term Mortality of Septic Patients at the Emergency Department. Int J Mol Sci. 2023;24(11):9121. doi:10.3390/ijms24119121
- Bo F, Shengdong L, Zongshuai W, et al. Global analysis of lysine 2-hydroxyisobutyrylation in wheat root. Sci Rep. 2021;11(1):6327. doi:10.1038/s41598-021-85879-y
- Huang M, Cai S, Su J. The Pathogenesis of Sepsis and Potential Therapeutic Targets. Int J Mol Sci. 2019;20(21):5376. doi:10.3390/ijms20215376
- Kumar V. Toll-like receptors in sepsis-associated cytokine storm and their endogenous negative regulators as future immunomodulatory targets. Int Immunopharmacol. 2020;89(Pt B):107087. doi:10.1016/j.intimp.2020.107087
- Hotchkiss RS, Monneret G, Payen D. Sepsis-induced immunosuppression: from cellular dysfunctions to immunotherapy. Nat Rev Immunol. 2013;13(12):862–874. doi:10.1038/nri3552
- Giamarellos-Bourboulis EJ. What is the pathophysiology of the septic host upon admission? Int J Antimicrob Agents. 2010;36(Suppl 2):S2–5. doi:10.1016/j.ijantimicag.2010.11.003
- Zhu XX, Zhang -W-W, Wu C-H, et al. The Novel Role of Metabolism-Associated Molecular Patterns in Sepsis. Front Cell Infect Microbiol. 2022;12:915099. doi:10.3389/fcimb.2022.915099
- Trager K, DeBacker D, Radermacher P. Metabolic alterations in sepsis and vasoactive drug-related metabolic effects. Curr Opin Crit Care. 2003;9(4):271–278. doi:10.1097/00075198-200308000-00004
- Preau S, Vodovar D, Jung B, et al. Energetic dysfunction in sepsis: a narrative review. Ann Intensive Care. 2021;11(1):104. doi:10.1186/s13613-021-00893-7
- Gordon S, Taylor PR. Monocyte and macrophage heterogeneity. Nat Rev Immunol. 2005;5(12):953–964. doi:10.1038/nri1733
- Kumar V. Targeting macrophage immunometabolism: dawn in the darkness of sepsis. Int Immunopharmacol. 2018;58:173–185. doi:10.1016/j.intimp.2018.03.005
- Jiang J, Huang D, Jiang Y, et al. Lactate Modulates Cellular Metabolism Through Histone Lactylation-Mediated Gene Expression in Non-Small Cell Lung Cancer. Front Oncol. 2021;11:647559. doi:10.3389/fonc.2021.647559
- Arts RJ, Gresnigt MS, Joosten LAB, et al. Cellular metabolism of myeloid cells in sepsis. J Leukoc Biol. 2017;101(1):151–164. doi:10.1189/jlb.4MR0216-066R
- Vandewalle J, Libert C. Sepsis: a failing starvation response. Trends Endocrinol Metab. 2022;33(4):292–304. doi:10.1016/j.tem.2022.01.006
- Fothergill-Gilmore LA, Michels PA. Evolution of glycolysis. Prog Biophys Mol Biol. 1993;59(2):105–235. doi:10.1016/0079-6107(93)90001-z
- Cheng YM, Peng Z, Chen H-Y, et al. Posttranslational lysine 2-hydroxyisobutyrylation of human sperm tail proteins affects motility. Hum Reprod. 2020;35(3):494–503. doi:10.1093/humrep/dez296
- Chen J, et al. Histone lactylation driven by mROS-mediated glycolytic shift promotes hypoxic pulmonary hypertension. J Mol Cell Biol. 2022.
- Pan RY, He L, Zhang J, et al. Positive feedback regulation of microglial glucose metabolism by histone H4 lysine 12 lactylation in Alzheimer’s disease. Cell Metab. 2022;34(4):634–648 e6. doi:10.1016/j.cmet.2022.02.013
- Luengo A, Li Z, Gui DY, et al. Increased demand for NAD(+) relative to ATP drives aerobic glycolysis. Mol Cell. 2021;81(4):691–707 e6. doi:10.1016/j.molcel.2020.12.012
- Li W, Tanikawa T, Kryczek I, et al. Aerobic Glycolysis Controls Myeloid-Derived Suppressor Cells and Tumor Immunity via a Specific CEBPB Isoform in Triple-Negative Breast Cancer. Cell Metab. 2018;28(1):87–103 e6. doi:10.1016/j.cmet.2018.04.022
- Kvacskay P, Yao N, Schnotz J-H, et al. Increase of aerobic glycolysis mediated by activated T helper cells drives synovial fibroblasts towards an inflammatory phenotype: new targets for therapy? Arthritis Res Ther. 2021;23(1):56. doi:10.1186/s13075-021-02437-7
- Luo Y, Li L, Chen X, et al. Effects of lactate in immunosuppression and inflammation: progress and prospects. Int Rev Immunol. 2022;41(1):19–29. doi:10.1080/08830185.2021.1974856
- Brooks GA. The Science and Translation of Lactate Shuttle Theory. Cell Metab. 2018;27(4):757–785. doi:10.1016/j.cmet.2018.03.008
- Khatib-Massalha E, Bhattacharya S, Massalha H, et al. Lactate released by inflammatory bone marrow neutrophils induces their mobilization via endothelial GPR81 signaling. Nat Commun. 2020;11(1). doi:10.1038/s41467-020-17402-2
- Liberti MV, Locasale JW. The Warburg Effect: how Does it Benefit Cancer Cells? Trends Biochem Sci. 2016;41(3):211–218. doi:10.1016/j.tibs.2015.12.001
- Suo S, Luo L, Song Y, et al. Early Diagnosis and Prediction of Death Risk in Patients with Sepsis by Combined Detection of Serum PCT, BNP, Lactic Acid, and Apache II Score. Contrast Media Mol Imaging. 2022;2022:8522842. doi:10.1155/2022/8522842
- Schneider L, Chalmers D, O’Beirn S, et al. Premorbid beta blockade in sepsis is associated with a lower risk of a lactate concentration above the lactate threshold, a retrospective cohort study. Sci Rep. 2022;12(1):20843. doi:10.1038/s41598-022-25253-8
- Howell MD, Donnino M, Clardy P, et al. Occult hypoperfusion and mortality in patients with suspected infection. Intensive Care Med. 2007;33(11):1892–1899. doi:10.1007/s00134-007-0680-5
- Suetrong B, Walley KR. Lactic Acidosis in Sepsis: it’s Not All Anaerobic: implications for Diagnosis and Management. Chest. 2016;149(1):252–261. doi:10.1378/chest.15-1703
- An R, Zhao L, Xi C, et al. Melatonin attenuates sepsis-induced cardiac dysfunction via a PI3K/Akt-dependent mechanism. Basic Res Cardiol. 2016;111(1). doi:10.1007/s00395-015-0526-1
- Baysan M, Baroni GD, van Boekel AM, et al. The Added Value of Lactate and Lactate Clearance in Prediction of In-Hospital Mortality in Critically Ill Patients With Sepsis. Critic Care Explorat. 2020;2(3):e0087. doi:10.1097/CCE.0000000000000087
- Yang K, Fan M, Wang X, et al. Lactate induces vascular permeability via disruption of VE-cadherin in endothelial cells during sepsis. Sci Adv. 2022;8(17):eabm8965. doi:10.1126/sciadv.abm8965
- Nolt B, Tu F, Wang X, et al. Lactate and Immunosuppression in Sepsis. Shock. 2018;49(2):120–125. doi:10.1097/SHK.0000000000000958
- Chen L, Xu J, Deng M, et al. Telmisartan mitigates lipopolysaccharide (LPS)-induced production of mucin 5AC (MUC5AC) through increasing suppressor of cytokine signaling 1 (SOCS1). Bioengineered. 2021;12(1):3912–3923. doi:10.1080/21655979.2021.1943605
- Lee MJ, et al. Serial Change of Endotoxin Tolerance in a Polymicrobial Sepsis Model. Int J Mol Sci. 2022;23(12).
- Pan T, Sun S, Chen Y, et al. Immune effects of PI3K/Akt/HIF-1alpha-regulated glycolysis in polymorphonuclear neutrophils during sepsis. Crit Care. 2022;26(1):29. doi:10.1186/s13054-022-03893-6
- Mao L, Sun M, Chen Z, et al. The Pyruvate Dehydrogenase Complex Mitigates LPS-Induced Endothelial Barrier Dysfunction by Metabolic Regulation. Shock. 2022;57(6):308–317. doi:10.1097/SHK.0000000000001931
- Zeng Z, Huang Q, Mao L, et al. The Pyruvate Dehydrogenase Complex in Sepsis: metabolic Regulation and Targeted Therapy. Front Nutr. 2021;8:783164. doi:10.3389/fnut.2021.783164
- Ding H, Wang -J-J, Zhang X-Y, et al. Lycium barbarum Polysaccharide Antagonizes LPS-Induced Inflammation by Altering the Glycolysis and Differentiation of Macrophages by Triggering the Degradation of PKM2. Biol Pharm Bull. 2021;44(3):379–388. doi:10.1248/bpb.b20-00752
- Luo Y, Yang Z, Yu Y, et al. HIF1alpha lactylation enhances KIAA1199 transcription to promote angiogenesis and vasculogenic mimicry in prostate cancer. Int J Biol Macromol. 2022;222:2225–2243. doi:10.1016/j.ijbiomac.2022.10.014
- Cai WQ, Xu D, Zeng C, et al. Modulating Lysine Crotonylation in Cardiomyocytes Improves Myocardial Outcomes. Circulation Res. 2022;131(5):456–472. doi:10.1161/CIRCRESAHA.122.321054
- Yang L, Xie M, Yang M, et al. PKM2 regulates the Warburg effect and promotes HMGB1 release in sepsis. Nat Commun. 2014;5(1):4436. doi:10.1038/ncomms5436
- Leite-Avalca MCG, Staats FT, Verona D, et al. Cannabinoid CB1 Receptor Antagonist Rimonabant Decreases Levels of Markers of Organ Dysfunction and Alters Vascular Reactivity in Aortic Vessels in Late Sepsis in Rats. Inflammation. 2019;42(2):618–627. doi:10.1007/s10753-018-0919-z
- Huang J, Liu K, Zhu S, et al. AMPK regulates immunometabolism in sepsis. Brain Behav Immun. 2018;72:89–100. doi:10.1016/j.bbi.2017.11.003
- Yang YC, et al. Endothelial Protective Effect of Ulinastatin on Early Rats with High-Voltage Burns through Nf-Kb Signalling Pathway. Acta Med Mediterr. 2020;36(5):3005–3009.
- Zheng ZB, Ma H, Zhang X, et al. Enhanced Glycolytic Metabolism Contributes to Cardiac Dysfunction in Polymicrobial Sepsis. J Infect Dis. 2017;215(9):1396–1406. doi:10.1093/infdis/jix138
- Dai X, Lv X, Thompson EW, et al. Histone lactylation: epigenetic mark of glycolytic switch. Trends Genet. 2022;38(2):124–127. doi:10.1016/j.tig.2021.09.009
- Tan C, Gu J, Li T, et al. Inhibition of aerobic glycolysis alleviates sepsis‑induced acute kidney injury by promoting lactate/Sirtuin 3/AMPK‑regulated autophagy. Int J Mol Med. 2021;47(3). doi:10.3892/ijmm.2021.4852
- Yang K, Xu J, Fan M, et al. Lactate Suppresses Macrophage Pro-Inflammatory Response to LPS Stimulation by Inhibition of YAP and NF-kappaB Activation via GPR81-Mediated Signaling. Front Immunol. 2020;11:587913. doi:10.3389/fimmu.2020.587913
- Chen A-N, Luo Y, Yang Y-H, et al. Lactylation, a Novel Metabolic Reprogramming Code: current Status and Prospects. Front Immunol. 2021;12:688910. doi:10.3389/fimmu.2021.688910
- Xie Y, Hu H, Liu M, et al. The role and mechanism of histone lactylation in health and diseases. Front Genet. 2022;13:949252. doi:10.3389/fgene.2022.949252
- Ye L, Jiang Y, Zhang M. Crosstalk between glucose metabolism, lactate production and immune response modulation. Cytokine Growth Factor Rev. 2022;68:81–92. doi:10.1016/j.cytogfr.2022.11.001
- Xin Q, Wang H, Li Q, et al. Lactylation: a Passing Fad or the Future of Posttranslational Modification. Inflammation. 2022;45(4):1419–1429. doi:10.1007/s10753-022-01637-w
- Gao M, Zhang N, Liang W. Systematic Analysis of Lysine Lactylation in the Plant Fungal Pathogen Botrytis cinerea. Front Microbiol. 2020;11:594743. doi:10.3389/fmicb.2020.594743
- Wan N, Wang N, Yu S, et al. Cyclic immonium ion of lactyllysine reveals widespread lactylation in the human proteome. Nat Methods. 2022;19(7):854–864. doi:10.1038/s41592-022-01523-1
- Li X, Yang Y, Zhang B, et al. Lactate metabolism in human health and disease. Signal Transduct Target Ther. 2022;7(1):305. doi:10.1038/s41392-022-01151-3
- Hayes C, Donohoe CL, Davern M, et al. The oncogenic and clinical implications of lactate induced immunosuppression in the tumour microenvironment. Cancer Lett. 2021;500:75–86. doi:10.1016/j.canlet.2020.12.021
- Yang K, Fan M, Wang X, et al. Lactate promotes macrophage HMGB1 lactylation, acetylation, and exosomal release in polymicrobial sepsis. Cell Death Differ. 2022;29(1):133–146. doi:10.1038/s41418-021-00841-9
- Zhang D, Tang Z, Huang H, et al. Metabolic regulation of gene expression by histone lactylation. Nature. 2019;574(7779):575–580. doi:10.1038/s41586-019-1678-1
- Yang D, Yin J, Shan L, et al. Identification of lysine-lactylated substrates in gastric cancer cells. iScience. 2022;25(7):104630. doi:10.1016/j.isci.2022.104630
- Gaffney DO, Jennings EQ, Anderson CC, et al. Non-enzymatic Lysine Lactoylation of Glycolytic Enzymes. Cell Chem Biol. 2020;27(2):206–213 e6. doi:10.1016/j.chembiol.2019.11.005
- Ma W, Ao S, Zhou J, et al. Methylsulfonylmethane protects against lethal dose MRSA-induced sepsis through promoting M2 macrophage polarization. Mol Immunol. 2022;146:69–77. doi:10.1016/j.molimm.2022.04.001
- Xiong J, He J, Zhu J, et al. Lactylation-driven METTL3-mediated RNA m(6)A modification promotes immunosuppression of tumor-infiltrating myeloid cells. Mol Cell. 2022;82(9):1660–1677 e10. doi:10.1016/j.molcel.2022.02.033
- Yu J, Chai P, Xie M, et al. Histone lactylation drives oncogenesis by facilitating m(6)A reader protein YTHDF2 expression in ocular melanoma. Genome Biol. 2021;22(1):85. doi:10.1186/s13059-021-02308-z
- Sun Y, Chen Y, Peng T. A bioorthogonal chemical reporter for the detection and identification of protein lactylation. Chem Sci. 2022;13(20):6019–6027. doi:10.1039/D2SC00918H
- Pan L, Feng F, Wu J, et al. Demethylzeylasteral targets lactate by inhibiting histone lactylation to suppress the tumorigenicity of liver cancer stem cells. Pharmacol Res. 2022;181:106270. doi:10.1016/j.phrs.2022.106270
- Yang Z, et al. Lactylome analysis suggests lactylation-dependent mechanisms of metabolic adaptation in hepatocellular carcinoma. Nat Metab. 2023.
- Hyun K, Jeon J, Park K, et al. Writing, erasing and reading histone lysine methylations. Exp Mol Med. 2017;49(4):e324. doi:10.1038/emm.2017.11
- Hou J, Wen X, Long P, et al. The role of post-translational modifications in driving abnormal cardiovascular complications at high altitude. Front Cardiovasc Med. 2022;9:886300. doi:10.3389/fcvm.2022.886300
- Huang H, Luo Z, Qi S, et al. Landscape of the regulatory elements for lysine 2-hydroxyisobutyrylation pathway. Cell Res. 2018;28(1):111–125. doi:10.1038/cr.2017.149
- Moreno-Yruela C, Zhang D, Wei W, et al. Class I histone deacetylases (HDAC1-3) are histone lysine delactylases. Sci Adv. 2022;8(3):eabi6696. doi:10.1126/sciadv.abi6696
- Moreno-Yruela C, Bæk M, Monda F, et al. Chiral Posttranslational Modification to Lysine epsilon-Amino Groups. Acc Chem Res. 2022;55(10):1456–1466. doi:10.1021/acs.accounts.2c00115
- Sun Y, Chen Y, Xu Y, et al. Genetic encoding of ε- N - l -lactyllysine for detecting delactylase activity in living cells. Chem Commun (Camb). 2022;58(61):8544–8547. doi:10.1039/D2CC02643K
- Dong H, Zhang J, Zhang H, et al. YiaC and CobB regulate lysine lactylation in Escherichia coli. Nat Commun. 2022;13(1):6628. doi:10.1038/s41467-022-34399-y
- Lee DC, Sohn H, Park Z-Y, et al. A lactate-induced response to hypoxia. Cell. 2015;161(3):595–609. doi:10.1016/j.cell.2015.03.011
- Yang J, Luo L, Zhao C, et al. A Positive Feedback Loop between Inactive VHL-Triggered Histone Lactylation and PDGFRbeta Signaling Drives Clear Cell Renal Cell Carcinoma Progression. Int J Biol Sci. 2022;18(8):3470–3483. doi:10.7150/ijbs.73398
- Gu J, Zhou J, Chen Q, et al. Tumor metabolite lactate promotes tumorigenesis by modulating MOESIN lactylation and enhancing TGF-beta signaling in regulatory T cells. Cell Rep. 2022;39(12):110986. doi:10.1016/j.celrep.2022.110986
- Cui H, Xie N, Banerjee S, et al. Lung Myofibroblasts Promote Macrophage Profibrotic Activity through Lactate-induced Histone Lactylation. Am J Respir Cell Mol Biol. 2021;64(1):115–125. doi:10.1165/rcmb.2020-0360OC
- Agatemor C, Middleton SAD, Toledo D. How pervasive are post-translational and -transcriptional modifications? Trends Cell Biol. 2022;32(6):475–478. doi:10.1016/j.tcb.2021.11.002
- Dichtl S, Lindenthal L, Zeitler L, et al. Lactate and IL6 define separable paths of inflammatory metabolic adaptation. Sci Adv. 2021;7(26). doi:10.1126/sciadv.abg3505
- Irizarry-Caro RA, McDaniel MM, Overcast GR, et al. TLR signaling adapter BCAP regulates inflammatory to reparatory macrophage transition by promoting histone lactylation. Proc Natl Acad Sci U S A. 2020;117(48):30628–30638. doi:10.1073/pnas.2009778117
- Chu X, Di C, Chang P, et al. Lactylated Histone H3K18 as a Potential Biomarker for the Diagnosis and Predicting the Severity of Septic Shock. Front Immunol. 2021;12:786666. doi:10.3389/fimmu.2021.786666
- Faix JD. Biomarkers of sepsis. Crit Rev Clin Lab Sci. 2013;50(1):23–36. doi:10.3109/10408363.2013.764490
- An S, Yao Y, Hu H, et al. PDHA1 hyperacetylation-mediated lactate overproduction promotes sepsis-induced acute kidney injury via Fis1 lactylation. Cell Death Dis. 2023;14(7). doi:10.1038/s41419-023-05952-4
- Michaels M, Madsen KL. Immunometabolism and microbial metabolites at the gut barrier: lessons for therapeutic intervention in Inflammatory Bowel Disease. Mucosal Immunol. 2023;16(1):72–85. doi:10.1016/j.mucimm.2022.11.001
- Capan M, Hoover S, Ivy JS, et al. Not all organ dysfunctions are created equal - Prevalence and mortality in sepsis. J Crit Care. 2018;48:257–262. doi:10.1016/j.jcrc.2018.08.021
- Pena OM, Hancock DG, Lyle NH, et al. An Endotoxin Tolerance Signature Predicts Sepsis and Organ Dysfunction at Initial Clinical Presentation. EBioMedicine. 2014;1(1):64–71. doi:10.1016/j.ebiom.2014.10.003
- Fan X, Liu Z, Jin H, et al. Alterations of dendritic cells in sepsis: featured role in immunoparalysis. Biomed Res Int. 2015;2015:903720. doi:10.1155/2015/903720
- Marin E, Bouchet-Delbos L, Renoult O, et al. Human Tolerogenic Dendritic Cells Regulate Immune Responses through Lactate Synthesis. Cell Metab. 2019;30(6):1075–1090 e8. doi:10.1016/j.cmet.2019.11.011
- Sim WJ, Ahl PJ, Connolly JE. Metabolism Is Central to Tolerogenic Dendritic Cell Function. Mediators Inflamm. 2016;2016:2636701. doi:10.1155/2016/2636701
- Schrijver IT, Theroude C, Roger T. Myeloid-Derived Suppressor Cells in Sepsis. Front Immunol. 2019;10:327. doi:10.3389/fimmu.2019.00327