Abstract
Background
G protein-gated inwardly rectifying potassium (GIRK) channels are involved in the regulation of neuronal excitability. Four GIRK subunits (GIRK1-4) are expressed in rat dorsal root ganglia (DRGs). Recently, we have characterized the expression of GIRK1 and −2, and both are downregulated in rat DRGs and spinal cord after a complete sciatic nerve transection (axotomy). Here, we aimed to study the neurochemical characteristics of GIRK3, and its regulation in rat DRGs and spinal cord induced by nerve injury.
Methods
A sciatic nerve axotomy was performed to study the influences of injury on GIRK3 expression in DRGs and spinal cord. A dorsal root rhizotomy and a sciatic nerve crush were employed to study the axonal transport of GIRK3 protein, respectively. Immunohistochemistry analysis was employed for investigating the neurochemical characteristics of GIRK3.
Results
In control DRGs, ~18% of neuron profiles (NPs) were GIRK3-positive (+), and ~41%, ~48% and ~45% of GIRK3+ NPs were CGRP+, IB4+ and NF200+, respectively. GIRK3-like immunoreactivity was observed in glabrous skin of hind paws and axons originating from DRG neurons. Fourteen days after axotomy, more than one-third of DRG NPs were GIRK3+, and among these ~51% and ~56% coexpressed galanin and neuropeptide Y, respectively. In control animals, a small group of interneurons found in the dorsal horn was GIRK3+. In addition, GIRK3+ processes could be observed in superficial laminae of spinal dorsal horn. After nerve injury, the intensity of GIRK3-like immunoreactivity in the superficial layers was increased. Evidence based on rhizotomy and sciatic nerve crush indicated both anterograde and retrograde transport of GIRK3.
Conclusion
Our study demonstrates that GIRK3 is expressed in sensory neurons and spinal cord. GIRK3 has both anterograde and retrograde axonal transport. GIRK3 expression can be regulated by peripheral nerve injury.
Introduction
Inhibitory (Gi/o) G protein-coupled receptor signaling pathways regulate membrane excitability in many cell types, including neurons, endocrine cells and cardiac myocytes.Citation1 G protein-gated inwardly rectifying potassium (GIRK) channels are common downstream effectors for PTX-sensitive Gi/o-dependent signaling pathways in the nervous system.Citation2 The mechanisms underlying activation of GIRK channels have been explored and evidence shows that GIRK channels are activated by Gβγ subunits after the ligand binding with the corresponding G protein-coupled receptors.Citation3,Citation4 A well-established example is opioid-induced analgesia which involves GIRK channels acting as downstream effectors.Citation5–Citation8 In addition, GIRK channels are known to mediate the inhibitory effects of many other neurotransmitters and drugs of abuse.Citation9,Citation10 GIRK channel subunits have been detected in dorsal root ganglion (DRG) neurons of rats and humans,Citation11–Citation14 and mice.Citation15,Citation16 Opiates are known to be highly effective in acute pain and acute inflammatory pain,Citation17–Citation21 but are less effective in neuropathic pain patients or animal models. After peripheral nerve injury, the GIRK1 and −2 proteins are significantly downregulated in DRGs and spinal dorsal horn.Citation22 This suggests a possible loss of opioid-induced inhibition.
There are four GIRK subunits (GIRK1-4) in mammals, of which GIRK1 and −2 have splice variants, but not GIRK3 or GIRK4.Citation23 Functional GIRK channels are composed of homotetramers or heterotetramers. GIRK3 cannot form functional homotetramersCitation24 but rather assembles with other GIRK subunits to form functional channels. In addition, GIRK3 is suggested to regulate GIRK signaling differently from other GIRK subunits based on the findings that in GIRK2- and/or 1-knockout (KO) mice, a strong reduction of GIRK current is detected in the brain,Citation25 however, GIRK3-KO mice exhibit similar currents induced by agonist but less severe sedatives induced withdrawal as compared to wild-type (WT) mice.Citation26 In GIRK2-KO mice, massive behavioral abnormalities have been found, whereas GIRK3-KO mice are no different from WT mice in behavioral tests.Citation1,Citation27,Citation28 Moreover, a lysosomal targeting sequence in GIRK3 can promote the degeneration of GIRK channels.Citation29 All evidence above points out the significance of GIRK3 for GIRK channel function, which is different from other GIRK subunits.
Recently, the expression of GIRK1 and GIRK2 has been studied in rat lumbar (L) DRGs and spinal cord, and both subunits are downregulated by peripheral axotomy.Citation22 In this study, the effects of nerve injury on GIRK3 expression were investigated in DRGs and spinal cord, and the axonal transport of GIRK3 was also analyzed.
Materials and Methods
Animals
Adult male Sprague–Dawley rats (200–250 g, B&K Universal, Stockholm, Sweden) were used in this study. The rats were housed 2 or 3 per cage and allowed to access to food and water ad libitum. All experiments were performed according to the Ethical Guidelines of the International Association for the Study of Pain and were approved by the local research ethics committee (Stockholm’s Norra Djurförsöksetiska Nämnd (N150/11)).
Surgical Procedures
The surgery for a unilateral complete sciatic nerve transection (axotomy) was made according to a previous study.Citation30 The rats subjected to axotomy were allowed to survive for 14 days. Dorsal root rhizotomy and sciatic nerve crush were performed according to previous procedures.Citation31,Citation32 Briefly, both skin and muscles were incised to expose vertebral laminae under deep anesthesia, followed by transection of L2-5 dorsal roots, and finally muscles and skin were sutured. The rats subjected to rhizotomy were allowed to survive for 14 days. The rats subjected to sciatic nerve crush were sacrificed 10 hrs postsurgery.
Immunohistochemistry
All rats were deeply anesthetized with sodium pentobarbital (50 mg/kg, i.p.). The anaesthetic animals were transcardially perfused with 50 mL warm saline (0.9%; 37°C), followed by 50 mL 4% paraformaldehyde, and finally by 250 mL of the same, but ice-cold fixative. The contra- and ipsilateral DRGs (L4-5), as well as corresponding spinal cord at injured levels, were dissected out and post-fixed in the fixative for 90 min at 4°C. Subsequently, all specimens were stored in 10% sucrose at 4°C for 2 days. Tissues were embedded in OCT compound (Tissue Tek, Sakura, Leiden, Netherland), and then sectioned in a cryostat (Microm, Heidelberg, Germany) at 12 μm (DRGs) or 14 μm (hind paw skin and sciatic nerve), and 20 μm (spinal cord) thickness. Immunoreactivities were visualized by tyramide signal amplification system (TSA Plus; NEN Life Science Products, Boston, MA) as described previously.Citation33 To perform colocalization study in DRG sections, adjacent ones (5 μm) were used for staining with different primary antibodies. In spinal cord, sciatic nerve or skin of hind paws, TSA Plus staining was performed, followed by indirect immunohistochemistry,Citation34 visualized by lissamine rhodamine sulfonylchloride-conjugated donkey anti-rabbit or mouse antibodies (1:100; Jackson Immuno Research, West Grove, PA, USA). Information for primary antibodies used in this study was provided in .
Table 1 Primary Antibody List
The specificity of anti-GIRK3 polyclonal antibody was confirmed by immunohistochemistry using brain samples from GIRK3-KO mice.Citation35 To detect IB4-binding neurons, the sections were incubated with isolectin B4 (IB4) (Griffonia simplicifolia I, 2.5 g/mL; Vector Laboratories, Burlingame, CA), followed by incubation with a goat anti-GSA I antiserum (1:2,000; Vector Laboratories).
Western Blot
On day 14 following axotomy, the contra- and ipsilateral DRGs (L4-5) were respectively collected, and then immediately snap frozen on dry ice. The tissues were roughly broken with knife and placed in lysis buffer containing protease inhibitor (P8340; Sigma), followed by sonication. The lysates were centrifuged for 30 min under 10,000×g at 4 °C, and the supernatants were used for Western blot analysis according to our previous procedures.Citation22 Briefly, 20 μg of protein was loaded on 10% SDS-PAGE gel and transferred to polyvinylidene fluoride membrane (Millipore, Hemel, Hempstead, UK). The anti-GIRK3 polyclonal antibody used in this study is known to recognize a protein of ~45 kD protein in WT cerebellum, but absent in either GIRK3-KO or GIRK2/3-KO mice.Citation35 The β-actin was used as a loading control.
Image Analysis and Quantification
The sections were analysed in a confocal scanning microscope (Bio-Rad, Hemel, Hempstead, UK) equipped with ×10 (0.5 NA), ×20 (0.75 NA) and ×63 oil (1.40 NA) objectives. In some experiments, a LSM 700 confocal microscope (Zeiss, Oberkochen, Germany) was used as described in previous studies.Citation33,Citation36 The quantification was performed following the same set as in a previous report.Citation22
Statistical Analysis
The statistical results were expressed as mean ± SEM. Percentages of GIRK3 immunoreactive (-IR) neuron profiles (NPs) were evaluated by unpaired Student’s t-test between contra- and ipsilateral DRGs. The band intensity in Western blot and fluorescence levels for GIRK3-like immunoreactivity (LI) in proximal and distal sciatic nerve was performed by densitometry with Image J software.
Results
Expression of GIRK3 in Control DRGs
In this study, the immunofluorescent signal of GIRK3 was observed in rat DRGs but was undetectable when the anti-GIRK3 antibody was pre-incubated with the homologous antigen in an absorption test ( vs. ). High power immunofluorescence micrographs showed that the GIRK3-LI was largely accumulated in a punctate pattern in the cytoplasm surrounding the nucleus ().
Figure 1 GIRK3 co-localizes with neuronal markers in control DRGs. (A, B) Arrows indicate the neurons expressing GIRK3 (A) and CGRP (B). (C) High magnification immunofluorescence micrograph shows punctate distribution of GIRK3-LI in the cytoplasm surrounding the nucleus. The dashed line indicates cellular border. The asterisk indicates nucleus. (D) GIRK3+ signal is absent after pre-incubation of the antibodies with 10−6 M immunogen in the absorption test. (E–M) GIRK3-LI (E, H, K) co-localizes with CGRP (F), IB4 (I) and NF200 (L) indicated by the arrows, respectively. (G), (J) and (M) are merged images. (N) Pie-graphs show the proportion of co-localization of GIRK3 with each marker in control DRGs. The labeled number indicates counted NPs. Scale bars indicate 100 μm (A, B), 50 μm (E–M) and 20 μm (C, D).
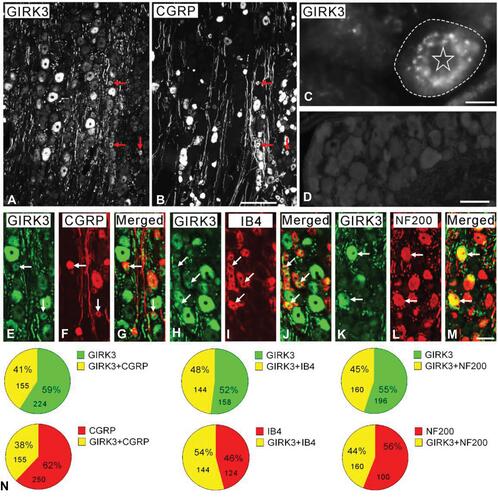
Based on previously described classification principle, DRG neurons were grouped into three subpopulations using neuronal markers calcitonin gene-related peptide (CGRP), IB4 and neurofilament 200 (NF200).Citation37 CGRP, IB4 and NF200 were used to label small peptidergic, small non-peptidergic, and medium-sized and large myelinated neurons, respectively. GIRK3-LI was detected in peptidergic and non-peptidergic NPs with a broad size spectrum, including small, medium-sized and large ones (, and –). Quantification results showed ~41%, ~48% and ~45% of GIRK3-IR NPs expressed CGRP, IB4 and NF200, respectively. Conversely, ~38%, 54% and ~44% CGRP-IR, IB4-IR and NF200-IR NPs expressed GIRK3, respectively ().
GIRK3 in Hind Paws and Sciatic Nerve
In rat glabrous skin of hind paws, GIRK3-LI was seen within the dermis layer (–). GIRK3-LI co-localized with CGRP and PGP9.5 in nerve terminals (–, –). GIRK3-LI was intensively expressed in the nerve bundles, as well as epineurium in the dermal layer of the hind paw skin (–). In addition, GIRK3-LI was also observed in non-neuronal cells (–). GIRK3 was expressed in intact sciatic nerve and co-localized with CGRP, IB4 and PGP9.5, respectively ().
Figure 2 Characteristics of GIRK3 expression in skin of hind paw. (A–C) A large amount of GIRK3-LI (A) is seen in upper dermis, some co-localize with CGRP+ fibers (B). (A1, B1, C1) High magnification of immunofluorescence micrographs shows the co-localized fibers of GIRK3-LI (A1; arrowheads) and CGRP-LI (B1; arrowheads). (A2, B2, C2) Some GIRK3+ (A2; arrowheads), but CGRP− (B2; arrowheads) fibers are seen in the upper dermis. (D–F) GIRK3+ fibers (D; arrowheads) are also PGP9.5+ (E; arrowheads) in upper dermis. (G–I) GIRK3-LI (G) co-localizes with PGP9.5-LI (H) largely in nerve bundles (white arrowheads) and epineurium (yellow arrowheads) in lower dermis (I). Scale bars indicate 100 μm (A–C, G–I) and 20 μm (A1–C2, D–F).
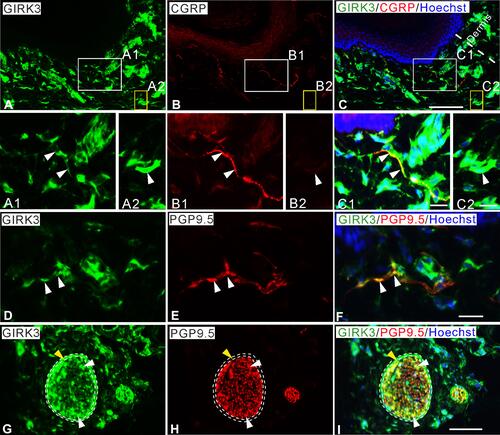
Figure 3 Expression of GIRK3-LI in sciatic nerve. (A–C) Double-staining shows a few GIRK3+ fibers (A) are CGRP+ (B) in sciatic nerve. (D–F) Some GIRK3+ fibers (D) are IB4+ (E). (G–I) GIRK3-LI (G) in sciatic nerve shows co-localization with an axonal marker PGP9.5 (H). Arrowheads indicate the co-localization of GIRK3-LI with each marker in sciatic nerve. (C), (F), and (I) are merged images. Scale bar indicates 20 μm (A–I).
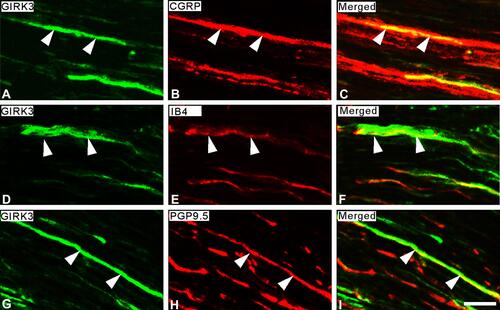
Upregulation of GIRK3 in Axotomized DRGs
On day 14 following axotomy, a significant upregulation in the percentage of GIRK3-IR NPs was observed in injured DRGs (ipsi vs. contra; 38.5 ± 0.9% vs. 17.1 ± 0.8%; ***p < 0.001, n = 5) (–). The relative levels of GIRK3 were also confirmed to be upregulated in the ipsilateral as compared to contralateral DRGs (from two rats, the same side of DRGs was pooled) by Western blot (). Of note, only one strong band with predicted molecular weight was observed in the intact membrane for Western blot, further confirming the antibody specificity ().
Figure 4 GIRK3 is upregulated in DRGs 14 days after axotomy. (A, B) Immunofluorescence micrographs show GIRK3-LI in contra- and ipsilateral DRGs, respectively. (C) Quantification analysis shows percentage of GIRK3+ NPs is significantly higher in ipsilateral compared to contralateral DRGs after axotomy (n = 5 per group; ***p < 0.001). (D) Western blot shows total protein of GIRK3 in DRGs is upregulated after axotomy. (E–G) GIRK3 (E) co-localizes with NPY (F) shown by arrows in ipsilateral DRGs. (H–J) GIRK3 (H) co-localizes with Gal (I) shown by arrows in ipsilateral DRGs. (G) and (J) are merged images. (K) Pie-graphs show the proportion of DRG neurons exhibiting co-localization of GIRK3 with NPY and Gal in ipsilateral DRGs, respectively. The labeled numbers indicate counted NPs. Arrows indicate co-localized neurons in (E–J). Scale bars indicate 40 μm (A, B) and 50 μm (E–J).
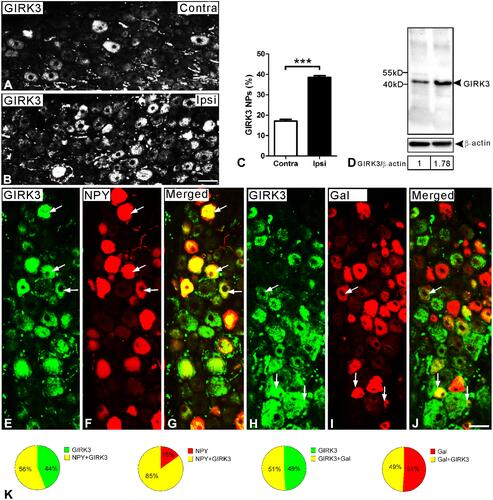
The 29-amino acid neuropeptide (30 in humans) galanin (Gal) and the 36-amino acid neuropeptide Y (NPY) are often used as indicators of nerve injury-associated outcomes. Under normal condition, NPY is usually undetectable in rat DRGs but its mRNA and protein levels are dramatically increased, predominantly in medium-sized and large NPs, after peripheral nerve injury.Citation38–Citation41 Gal is expressed in a small portion (<5%) of DRG NPs, but is strongly upregulated (up to 50%) in injured DRGs, with peak levels on 7–14 days after axotomy, at both mRNA and protein levels.Citation42,Citation43 In the present study, ~56% and ~51% of GIRK3-positive (+) NPs co-expressed NPY and Gal 14 days after axotomy, respectively (–). Conversely, ~85% of NPY+ and ~49% of Gal+ NPs were GIRK3+, respectively (–).
Peripheral Nerve Injury Induced an Upregulation of GIRK3 in Spinal Dorsal Horn
GIRK3-LI was mainly observed in the superficial layers of dorsal horn (, and ). The GIRK3-LI was absent in the absorption test (). CGRP+ fibers were found in lamina I and II with some extension to the deeper laminae (). GIRK3-IR interneurons were found in deeper laminae, but not superficial layers (, , and ). These interneurons were CGRP-negative (–). An increase of GIRK3+ processes was detected in the ipsilateral as compared with the contralateral dorsal horn on day 14 after axotomy ().
Figure 5 Expression and regulation of GIRK3 in spinal cord. GIRK3-LI is present in control dorsal horn (A), and is absent after pre-incubation of the antibody with 10−6 M immunogen in the absorption test (B). (C, D) GIRK3-LI (D) is largely localized in the superficial laminae but much weaker than CGRP-LI (C), and also present in a few interneurons in deeper laminae (E, G, H). Representative micrographs show GIRK3+ (G and H; arrows) but CGRP− (F; arrows) interneurons in deeper laminae (GIRK3, green; CGRP, red). (I) Fourteen days after axotomy, a dramatic upregulation of GIRK3-LI (red arrows) is seen on the ipsilateral (ipsi) as compared to contralateral (contra) spinal dorsal horn. Scale bars indicate 500 μm (I), 100 μm (A–D), 50 μm (F–H) and 25 μm (E).
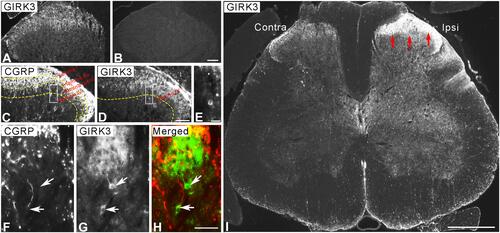
Axonal Transport of GIRK3
Spinal dorsal rhizotomy was performed unilaterally. After rhizotomy, CGRP-LI was largely reduced in the ipsilateral dorsal horn as compared with contralateral one ( vs ). Similar to that of CGRP, GIRK3+ signals were mostly eliminated in the ipsilateral dorsal horn after rhizotomy, indicating an anterograde transport of GIRK3 from peripheral sensory neurons to central spinal cord ( vs ). Sciatic nerve crush caused an accumulation of GIRK3 at the injured site where GIRK3-LI was significantly stronger in proximal than distal sites ( and ), thus indicating both anterograde and retrograde transport of GIRK3 in DRG neurons.
Figure 6 Axonal transport of GIRK3. (A–D) Fourteen days after unilateral dorsal rhizotomy, both CGRP- and GIRK3-LIs are largely decreased (red arrows) in ipsilateral dorsal horn compared to contralateral one. (E, F) Ten hours after sciatic nerve crush (red arrows indicate injured site), significant more GIRK3-LI is accumulated on the proximal side of the crush (green arrows) than the distal side (n = 3; **p < 0.01). Scale bars indicate 100 μm (A–D) and 50 μm (E).
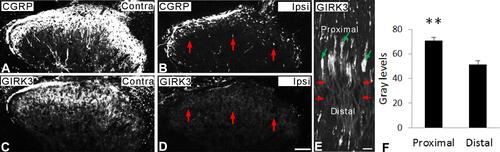
Discussion
We have recently investigated the expression and regulation of GIRK1 and −2 in rat DRGs and spinal cord following peripheral nerve injury.Citation22 In the present study, similar methods have been employed to analyze the expression of GIRK3 in rat DRGs and spinal cord. GIRK3 was expressed in ~17% of the DRG NPs and significantly increased after axotomy (to around 39%). This contrasts with the expression of both GIRK1 and −2, for which the corresponding proportions in controls were ~70% and <10%, respectively, and both were downregulated (to ~55% and <2%, respectively). GIRK3 was also detected in the spinal dorsal horn and also increased after axotomy.
Using RNA-seq, Flegel and colleagues investigated the expression of G protein-coupled receptors and ion channels in human sensory ganglia, including DRGs and trigeminal ganglia.Citation12 All GIRK channel subunits were found in human DRGs, with a highest expression level of GIRK1, lower expression levels of GIRK3 and GIRK4, and lowest expression level of GIRK2. Our previously published resultsCitation22 and results presented in this study reveal a similar expression pattern of GIRK1-3 in rats compared to humans.
We further detected GIRK3-LI in all three major DRG neuron subpopulations, which include neurons expressing CGRP (small peptidergic), IB4 (small non-peptidergic) or NF200 (medium-sized and large myelinated Aß), whereby each of the three was found in 40~50% of the GIRK3+ NPs. This means that there is a certain overlap, but the details still need to be sorted out. Moreover, and importantly, also GIRK1 coexpressed these three markers with the following percentages: ~17%, ~51% and ~39%.Citation22 This indicates a potential for interaction of GIRK3 with GIRK1 to form heterotetramer channels in the subpopulations of DRG neurons. In contrast, none of the GIRK2+ neurons expressed CGRP, but a high percentage IB4 (~73%) and a lower percentage NF200 (~32%).Citation22 Thus, our findings suggest a possible interaction between GIRK3 and GIRK2 in non-peptidergic and myelinated neurons. We found a large amount of GIRK3-LI surrounding the nucleus in the cytoplasm without clear membrane localization. This maybe because that GIRK3 lacks an endoplasmic reticulum (ER) export signalCitation29 and therefore it is retained in the ER after translation. In addition, GIRK1 but not GIRK2 or 4 also lacks an ER export signal. Thus, the GIRK1 is mostly not seen on the membrane.Citation22
GIRK3+ processes were seen in the superficial layers of spinal dorsal horn, especially in the lamina II. Interestingly, GIRK1+ and GIRK2+ processes were also found abundantly in the lamina II. However, only accountable GIRK3+ interneurons were seen in the deeper spinal dorsal horn laminae. Thus, most of GIRK3+ fibers in the dorsal horn presumably originate in DRGs. This is supported by our findings (i) sciatic nerve crush causes an accumulation of GIRK3 on proximal side indicating the existence of anterograde fast axonal transport; and, especially, (ii) GIRK3-LI was completely eliminated in the ipsilateral dorsal horn after dorsal root rhizotomy. Thus, the majority of GIRK3 is located in the presynaptic terminals of sensory input to the spinal cord, indicating a role in modulating spinal nociceptive processing at the presynaptic level. In contrast, much more GIRK1 and −2 LIs were detected in local dorsal horn neurons. Moreover, both GIRK1 and −2 are axonally transported,Citation22 and they are also present in afferent nerve endings. These two subunits may thus have both presynaptic actions as well as controlling signalling in postsynaptic dorsal horn interneurons.
It has been demonstrated that several types of potassium (K+) channel-components are downregulated by peripheral nerve injury in somatosensory system, including voltage-gated K+ channels, two-pore-domain K+ channels, calcium2+-activated K+ channels, ATP-sensitive K+ channels and inwardly-rectifying K+ channels.Citation2,Citation44,Citation45 The activation of these K+ channels can induce K+ outflow and cause the membrane potential of neurons more negative, which is more inhibited. Here, we reported an upregulation of GIRK3 in both DRGs and dorsal horn following peripheral nerve injury, which is in contrast to the downregulation of most of the other K+ channel-components as listed above. These data reflect a unique and complex role of GIRK3 in the sensory neurons. Considering a role of GIRK3 in reducing the number of other GIRK channels on the cell surface by targeting other subunits to lysosome for degradation,Citation29 the upregulation of GIRK3 may be a possible reason for the downregulation of GIRK1 and −2.
Gal and NPY are both strongly upregulated and have been shown to be involved in nociceptive processing, especially after peripheral nerve injury. Here, we observed that a high proportion of GIRK3+ neurons contained Gal and NPY-LIs, further supporting a role of GIRK3 in pain modulation.
Concluding Remarks
This work was an extension of our previous study on GIRK1 and −2. Our aim was to investigate the expression and regulation of GIRK3 in sensory neurons after nerve injury. Together with our previously published data, a summary of expression of GIRK channel subunits 1–3, in rat DRGs and spinal dorsal horn, is as follows:
Of the three channel subunits, GIRK1 has the highest level of protein.
In contrast to the downregulation of GIRK1 and −2, GIRK3-LI is upregulated after peripheral nerve injury.
Author Contributions
C.L., G.W.L. and T.J.S.S. performed experiments. C.L. and T.J.S.S. designed the experiments. C.L., G.W.L. and T.J.S.S. analyzed the data. J.M. and A.M. contributed reagents, materials, analysis tools and resources. C.L. and T.J.S.S. wrote the manuscript. J.M. and A.M. reviewed and revised the draft manuscript. All authors made substantial contributions to conception and design, acquisition of data, or analysis and interpretation of data; took part in drafting the article or revising it critically for important intellectual content; gave final approval of the version to be published; and agree to be accountable for all aspects of the work.
Acknowledgments
We thank Dr. Tomas Hökfelt (Karolinska Institutet) for providing the anti-GIRK3 antibody which was an original gift from Dr. Masahiko Watanabe (Hokkaido University). We thank Dr. Kaj Fried (Karolinska Institutet) for help with animal experiments. We thank Dr. Tomas Hökfelt for critical reading of the manuscript. This work was supported by the China Scholarship Council (CSC) award to Dr. Chuang Lyu (HIT, 2013), and The Research Council of Norway (811397) to A.M. and T.J.S.S.
Disclosure
The authors report no conflicts of interest in this work.
References
- Lujan R, Marron Fernandez de Velasco E, Aguado C, Wickman K. New insights into the therapeutic potential of Girk channels. Trends Neurosci. 2014;37(1):20–29. doi:10.1016/j.tins.2013.10.006
- Hibino H, Inanobe A, Furutani K, et al. Inwardly rectifying potassium channels: their structure, function, and physiological roles. Physiol Rev. 2010;90(1):291–366. doi:10.1152/physrev.00021.2009
- Huang CL, Slesinger PA, Casey PJ, Jan YN, Jan LY. Evidence that direct binding of G beta gamma to the GIRK1 G protein-gated inwardly rectifying K+ channel is important for channel activation. Neuron. 1995;15(5):1133–1143. doi:10.1016/0896-6273(95)90101-9
- Huang CL, Jan YN, Jan LY. Binding of the G protein betagamma subunit to multiple regions of G protein-gated inward-rectifying K+ channels. FEBS Lett. 1997;405(3):291–298. doi:10.1016/S0014-5793(97)00197-X
- Ikeda K, Kobayashi T, Kumanishi T, Niki H, Yano R. Involvement of G-protein-activated inwardly rectifying K (GIRK) channels in opioid-induced analgesia. Neurosci Res. 2000;38:113–116. doi:10.1016/S0168-0102(00)00144-9
- Minami M, Satoh M. Molecular biology of the opioid receptors: structures, functions and distributions. Neurosci Res. 1995;23(2):121–145. doi:10.1016/0168-0102(95)00933-K
- Patil N, Cox DR, Bhat D, et al. A potassium channel mutation in weaver mice implicates membrane excitability in granule cell differentiation. Nat Genet. 1995;11(2):126–129. doi:10.1038/ng1095-126
- Ikeda K, Kobayashi T, Kumanishi T, Niki H, Yano R. Involvement of G-protein-activated inwardly rectifying K (GIRK) channels in opioid-induced analgesia. Neurosci Res. 2000;38(1):113–116. doi:10.1016/S0168-0102(00)00144-9
- Luscher C, Jan LY, Stoffel M, Malenka RC, Nicoll RA. G protein-coupled inwardly rectifying K+ channels (GIRKs) mediate postsynaptic but not presynaptic transmitter actions in hippocampal neurons. Neuron. 1997;19(3):687–695. doi:10.1016/S0896-6273(00)80381-5
- Lomazzi M, Slesinger PA, Luscher C. Addictive drugs modulate GIRK-channel signaling by regulating RGS proteins. Trends Pharmacol Sci. 2008;29(11):544–549. doi:10.1016/j.tips.2008.07.011
- Nockemann D, Rouault M, Labuz D, et al. The K + channel GIRK2 is both necessary and sufficient for peripheral opioid-mediated analgesia. EMBO Mol Med. 2013;5(8):1263–1277. doi:10.1002/emmm.v5.8
- Flegel C, Schöbel N, Altmüller J, et al. RNA-Seq analysis of human trigeminal and dorsal root ganglia with a focus on chemoreceptors. PLoS One. 2015;10(6):e0128951. doi:10.1371/journal.pone.0128951
- Gao XF, Zhang HL, You ZD, Lu CL, He C. G protein-coupled inwardly rectifying potassium channels in dorsal root ganglion neurons. Acta Pharmacol Sin. 2007;28(2):185–190. doi:10.1111/j.1745-7254.2007.00478.x
- Zhang H, Dougherty PM. Enhanced excitability of primary sensory neurons and altered gene expression of neuronal ion channels in dorsal root ganglion in paclitaxel-induced peripheral neuropathy. Anesthesiology. 2014;120(6):1463–1475. doi:10.1097/ALN.0000000000000176
- Usoskin D, Furlan A, Islam S, et al. Unbiased classification of sensory neuron types by large-scale single-cell RNA sequencing. Nat Neurosci. 2015;18(1):145–153. doi:10.1038/nn.3881
- Uttam S, Wong C, Amorim IS, et al. Translational profiling of dorsal root ganglia and spinal cord in a mouse model of neuropathic pain. Neurobiol Pain. 2018;4:35–44. doi:10.1016/j.ynpai.2018.04.001
- Luger NM, Sabino MAC, Schwei MJ, et al. Efficacy of systemic morphine suggests a fundamental difference in the mechanisms that generate bone cancer vs inflammatory pain. Pain. 2002;99(3):397–406. doi:10.1016/S0304-3959(02)00102-1
- Gonzalez-Rodriguez S, Hidalgo A, Baamonde A, Menendez L. Involvement of Gi/o proteins and GIRK channels in the potentiation of morphine-induced spinal analgesia in acutely inflamed mice. Naunyn-Schmiedeberg’s Arch Pharmacol. 2010;381(1):59–71. doi:10.1007/s00210-009-0471-3
- Morrone LA, Scuteri D, Rombola L, Mizoguchi H, Bagetta G. Opioids resistance in chronic pain management. Curr Neuropharmacol. 2017;15(3):444–456. doi:10.2174/1570159X14666161101092822
- Bedini A, Spampinato SM. Innovative opioid peptides and biased agonism: novel avenues for more effective and safer analgesics to treat chronic pain. Curr Med Chem. 2017;25(32):3895–916.
- Basurto Ona X, Rigau Comas D, Urrutia G. Opioids for acute pancreatitis pain. Cochrane Database Syst Rev. 2013;7:CD009179.
- Lyu C, Mulder J, Barde S, et al. G protein-gated inwardly rectifying potassium channel subunits 1 and 2 are down-regulated in rat dorsal root ganglion neurons and spinal cord after peripheral axotomy. Mol Pain. 2015;11(1):44. doi:10.1186/s12990-015-0044-z
- Luscher C, Slesinger PA. Emerging roles for G protein-gated inwardly rectifying potassium (GIRK) channels in health and disease. Nat Rev Neurosci. 2010;11(5):301–315. doi:10.1038/nrn2834
- Jelacic TM, Sims SM, Clapham DE. Functional expression and characterization of G-protein-gated inwardly rectifying K+ channels containing GIRK3. J Membr Biol. 1999;169(2):123–129. doi:10.1007/s002329900524
- Koyrakh L, Luján R, Colón J, et al. Molecular and cellular diversity of neuronal G-protein-gated potassium channels. J Neurosci. 2005;25(49):11468–11478. doi:10.1523/JNEUROSCI.3484-05.2005
- Kozell LB, Walter NA, Milner LC, Wickman K, Buck KJ. Mapping a barbiturate withdrawal locus to a 0.44 Mb interval and analysis of a novel null mutant identify a role for Kcnj9 (GIRK3) in withdrawal from pentobarbital, zolpidem, and ethanol. J Neurosci. 2009;29(37):11662–11673. doi:10.1523/JNEUROSCI.1413-09.2009
- Pravetoni M, Wickman K. Behavioral characterization of mice lacking GIRK/Kir3 channel subunits. Genes Brain Behav. 2008;7(5):523–531. doi:10.1111/gbb.2008.7.issue-5
- Arora D, Haluk DM, Kourrich S, et al. Altered neurotransmission in the mesolimbic reward system of Girk mice. J Neurochem. 2010;114(5):1487–1497. doi:10.1111/j.1471-4159.2010.06864.x
- Ma D, Zerangue N, Raab-Graham K, et al. Diverse trafficking patterns due to multiple traffic motifs in G protein-activated inwardly rectifying potassium channels from brain and heart. Neuron. 2002;33(5):715–729. doi:10.1016/S0896-6273(02)00614-1
- Shi TJ, Tandrup T, Bergman E, et al. Effect of peripheral nerve injury on dorsal root ganglion neurons in the C57 BL/6J mouse: marked changes both in cell numbers and neuropeptide expression. Neuroscience. 2001;105(1):249–263. doi:10.1016/S0306-4522(01)00148-8
- Shi TJ, Liu S-XL, Hammarberg H, et al. Phospholipase C{beta}3 in mouse and human dorsal root ganglia and spinal cord is a possible target for treatment of neuropathic pain. Proc Natl Acad Sci U S A. 2008;105(50):20004–20008. doi:10.1073/pnas.0810899105
- Dahlstrom AB, Li JY. Fast and slow axonal transport-different methodological approaches give complementary information: contributions of the stop-flow/crush approach. Neurochem Res. 1994;19(11):1413–1419. doi:10.1007/BF00972470
- Shi T-JS, Xiang Q, Zhang M-D, et al. Secretagogin is expressed in sensory CGRP neurons and in spinal cord of mouse and complements other calcium-binding proteins, with a note on rat and human. Mol Pain. 2012;8:80. doi:10.1186/1744-8069-8-80
- Mulder J, Björling E, Jonasson K, et al. Tissue profiling of the mammalian central nervous system using human antibody-based proteomics. mol Cell Proteom. 2009;8(7):1612–1622. doi:10.1074/mcp.M800539-MCP200
- Aguado C, Colón J, Ciruela F, et al. Cell type-specific subunit composition of G protein-gated potassium channels in the cerebellum. J Neurochem. 2008;105(2):497–511. doi:10.1111/j.1471-4159.2007.05153.x
- Shi T-JS, Xiang Q, Zhang M-D, et al. Somatostatin and its 2A receptor in dorsal root ganglia and dorsal horn of mouse and human: expression, trafficking and possible role in pain. Mol Pain. 2014;10:12. doi:10.1186/1744-8069-10-12
- McMahon SB, Priestley JV. Nociceptor plasticity. In: Hunt SP, Koltzenburg M, editors. The Neurobiology of Pain. Oxford Univ Press; 2005:35–64.
- Gibson SJ, Polak JM, Allen JM, et al. The distribution and origin of a novel brain peptide, neuropeptide Y, in the spinal cord of several mammals. J Comp Neurol. 1984;227(1):78–91. doi:10.1002/(ISSN)1096-9861
- Wakisaka S, Kajander KC, Bennett GJ. Increased neuropeptide Y (NPY)-like immunoreactivity in rat sensory neurons following peripheral axotomy. Neurosci Lett. 1991;124(2):200–203. doi:10.1016/0304-3940(91)90093-9
- Corness J, Shi TJ, Xu ZQ, Brulet P, Hokfelt T. Influence of leukemia inhibitory factor on galanin/GMAP and neuropeptide Y expression in mouse primary sensory neurons after axotomy. Exp Brain Res. 1996;112(1):79–88. doi:10.1007/BF00227180
- Shi TJ, Cui JG, Meyerson BA, Linderoth B, Hokfelt T. Regulation of galanin and neuropeptide Y in dorsal root ganglia and dorsal horn in rat mononeuropathic models: possible relation to tactile hypersensitivity. Neuroscience. 1999;93(2):741–757. doi:10.1016/S0306-4522(99)00105-0
- Hokfelt T, Wiesenfeld-Hallin Z, Villar M, Melander T. Increase of galanin-like immunoreactivity in rat dorsal root ganglion cells after peripheral axotomy. Neurosci Lett. 1987;83(3):217–220. doi:10.1016/0304-3940(87)90088-7
- Lang R, Gundlach AL, Holmes FE, et al. Physiology, signaling, and pharmacology of galanin peptides and receptors: three decades of emerging diversity. Pharmacol Rev. 2015;67(1):118–175.
- Chen SR, Chen H, Yuan WX, Pan HL. Increased presynaptic and postsynaptic alpha2-adrenoceptor activity in the spinal dorsal horn in painful diabetic neuropathy. J Pharmacol Exp Ther. 2011;337(1):285–292. doi:10.1124/jpet.110.176586
- Du X, Gamper N. Potassium channels in peripheral pain pathways: expression, function and therapeutic potential. Curr Neuropharmacol. 2013;11(6):621–640. doi:10.2174/1570159X113119990042
- Orazzo C, Pieribone VA, Ceccatelli S, Terenius L, Hokfelt T. CGRP-like immunoreactivity in A11 dopamine neurons projecting to the spinal cord and a note on CGRP-CCK cross-reactivity. Brain Res. 1993;600(1):39–48. doi:10.1016/0006-8993(93)90399-8
- Perry MJ, Lawson SN, Robertson J. Neurofilament immunoreactivity in populations of rat primary afferent neurons: a quantitative study of phosphorylated and non-phosphorylated subunits. J Neurocytol. 1991;20(9):746–758. doi:10.1007/BF01187848
- Brumovsky P, Stanic D, Shuster S, et al. Neuropeptide Y2 receptor protein is present in peptidergic and nonpeptidergic primary sensory neurons of the mouse. J Comp Neurol. 2005;489(3):328–348. doi:10.1002/(ISSN)1096-9861