Abstract
Microcirculation plays a crucial role in physiological processes of tissue oxygenation and nutritional exchange. Measurement of microcirculation can be applied on many organs in various pathologies. In this paper we aim to review the technique of non-invasive methods for imaging of the microcirculation. Methods covered are: videomicroscopy techniques, laser Doppler perfusion imaging, and laser speckle contrast imaging. Videomicroscopy techniques, such as orthogonal polarization spectral imaging and sidestream dark-field imaging, provide a plentitude of information and offer direct visualization of the microcirculation but have the major drawback that they may give pressure artifacts. Both laser Doppler perfusion imaging and laser speckle contrast imaging allow non-contact measurements but have the disadvantage of their sensitivity to motion artifacts and that they are confined to relative measurement comparisons. Ideal would be a non-contact videomicroscopy method with fully automatic analysis software.
Introduction
Microcirculation is usually defined as small arteries (<150 μm in diameter), arterioles, capillaries, and venules.Citation1,Citation2 It plays a crucial role in physiological processes of tissue oxygenation and nutritional exchange. In addition, studies of microcirculation have shown its involvement in a number of pathological processes, which have increased the interest in studying microcirculation in recent years.
There are several ways to measure microcirculation. Both morphological and functional studies are possible. Morphological parameters measurable with imaging methods include vessel density, rate of perfused vessels and vessel diameter in addition to dynamic measurements of microcirculatory blood flow velocity and blood cell concentration.Citation3
Functional studies, especially targeting microcirculatory endothelial function, include pharmacological tests with vasoactive drugs and post-occlusive reactive hyperemia test.Citation1,Citation4 Post-occlusive hyperemia test is performed by measuring distal flow of microcirculation after a period of proximal vascular occlusion using a cuff with a pressure exceeding the systolic pressure. This is typically used to study forearm microcirculation. Further, microvascular evaluation can be performed with both single-point measurements and imaging methods, giving a full-field image of the microcirculation of a tissue surface. The use of imaging methods is preferable due to the spatial heterogeneity of perfusion seen in microvascular beds. This leads to a better reproducibility using imaging methods which measure larger areas as compared to methods with single-point measurements.Citation5,Citation6 In addition, imaging methods have also been shown to have good inter-day reproducibility.Citation5
One example of the use of microvascular assessment in pathophysiology is in the field of cardiovascular disease. Studies of patients with hypertension and normotensive patients with obesity have shown structural changes in small resistance arteries by an increased tunica media to vessel lumen diameter ratio.Citation7,Citation8 And as for hypertension, these structural changes have been correlated to a higher risk of developing stroke and myocardial infarction.Citation7 Using the post-occlusive reactive hyperemia test, a decrease in post-occlusive flow has been shown in patients with atherosclerosis.Citation9 Thus, as in this model disease, microcirculatory assessment could potentially be used to stage, monitor, and even predict pathological processes and be a useful tool in the assessment of diseases.
In this paper we aim to review the technique of noninvasive methods for imaging the microcirculation and give an overview of their clinical applications. Methods covered in this review include: videomicroscopy techniques, laser Doppler perfusion imaging (LDPI), and laser speckle contrast imaging (LSCI).
Videomicroscopy techniques
Technology
Videomicroscopy techniques can be used for direct visualization of microcirculation and involve orthogonal polarization spectral (OPS) imaging and its successor sidestream dark-field (SDF) imaging. Both techniques are based on the same underlying principles that were developed many years ago.Citation10 However, it was first in 1999 that Groner et al used OPS in a handheld device measuring microcirculation.Citation11
The underlying technique of OPS is described in detail by Groner et al.Citation11 Summarily, polarized light is illuminating the tissue to be examined. Only light that penetrates the tissue more deeply becomes depolarized and by sending the reflected light through a orthogonal polarizer this enables only depolarized light to be passed, thus excluding direct reflections. The collected light then forms an image of the illuminated area and can be recorded with a charge coupled device (CCD) video camera. By polarizing the light from the light source with a wavelength that is absorbed by hemoglobin (548 nm), red blood cells (RBC) will appear dark, thus forming an image of the microcirculation. This results in vessels only being visible if they contain RBC. In , the absorption spectrum of oxygenated hemoglobin is shown. OPS imaging has been validated against intra-vital fluorescence microscopy and conventional capillary microscopy.Citation12–Citation14
Figure 1 Absorption spectra in tissue of oxygenated hemoglobin (HbO2) and water.
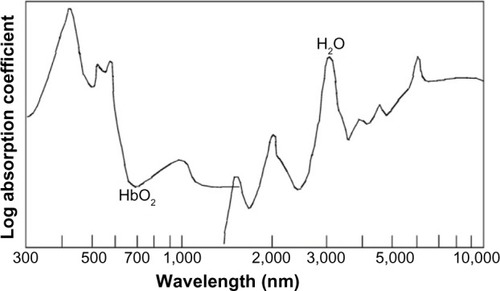
Driven by the fact that a relatively strong external light source is needed in OPS imaging and the limited visualization of the capillaries due to blurring, SDF imaging was developed.Citation15,Citation16 In SDF imaging, the light source is made up of concentrically placed light emitting diodes surrounding the optics (see ). The light emitting diodes provide pulsed green light in synchrony with the CCD frame rate, thus preventing smearing of moving objects. The whole microscope including optics and light source is housed in one handheld device. Like in OPS imaging, the light wavelength is chosen so that the RBC appear dark (530 nm). SDF imaging has been validated by comparison to OPS imaging.Citation16
Figure 2 Sidestream dark-field (SDF) imaging technique.
Abbreviation: LED, light emitting diodes.
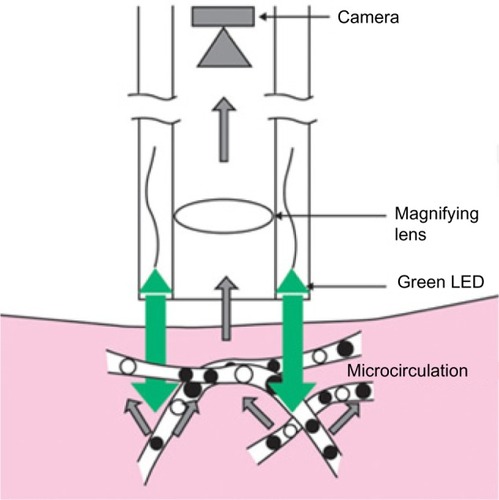
These methods allow direct visualization of the micro-circulation and vessel diameter, vessel density and red blood cell velocity can be measured. Microcirculatory analysis is made with semi-automatic analysis software. Yet, some manual intervention is still needed. According to Micro-Vision Medical web page, they are working on a new software package which requires less manual intervention.Citation17 Different analyses scores of microcirculation have been developed and De Backer et al propose the use of proportion of perfused vessels (PPV), perfused vessel density, microvascular flow index (MFI), and heterogeneity index.Citation3 PPV is calculated after manually identifying the amount of vessels that have continuous or intermittent flow. Perfused vessel density is calculated by multiplying vessel density by PPV. MFI score is based on flow characterization (absent, intermittent, sluggish or normal) in the four quadrants of the recorded images of the microcirculation. Finally, heterogeneity index can be calculated by measuring the MFI in three to five sites and then taking the difference between highest MFI minus the lowest MFI divided by the mean flow velocity.
Applications
The most studied area with these techniques is the sublingual mucosa in patients with sepsis where it has been shown that alterations in microvascular blood flow are frequent in patients with sepsis and more severe alterations have been associated with severe disease and a higher mortality rate.Citation18,Citation19 However, several other organs have been studied such as skin, gingiva, conjunctiva, brain, lungs, tongue, liver, gut, ileostomies or colostomies, and rectal mucosa.Citation13,Citation20–Citation30 In liver transplants, assessment of hepatic microcirculation is reported to be predictive of early graft postoperative function and in liver resections, hepatic microcirculation parameters have been used to distinguish histologically damaged livers.Citation27,Citation30 shows a typical SDF image of rat liver parenchyma.
Advantages and limitations
The greatest advantage with videomicroscopic techniques is their direct visualization of the microcirculation. Yet, there are limitations as well. These methods are sensitive to motion and pressure artifacts.Citation16,Citation27,Citation31 Motion can result in movement artifacts and pressure can disturb vessel blood flow. Analysis software has a built-in image stabilization that partly deals with movement artifacts and the use of a stabilization attachment has been suggested.Citation32,Citation33 Also, inability to measure high blood flow velocities has been reported.Citation32,Citation34 Moreover, when using these methods on solid organs, the organ capsule has to be thin.Citation31 Otherwise, the capsule has to be removed before measuring.Citation27 Also, the offline analysis is time consuming, for liver analysis approximately 5 minutes per measurement point.Citation27
LDPI
Technology
Studying retinal perfusion, Riva et al were the first to describe flow measurements in microvascular vessels using laser Doppler flowmetry.Citation35 The technique uses coherent laser light which undergoes a small shift in frequency due to the Doppler effect when striking moving particles such as RBC.Citation36 This only appears when light is reflected on moving objects and reflected light from static tissue surrounding the RBC returns with unchanged frequency. The average amount of change in frequency, called Doppler shift, in the reflected light is proportional to the product of average speed and concentration of RBC. The Doppler shift is the output of laser Doppler flowmetry.
Laser Doppler flowmetry is a single-point technique measuring an average of the microcirculation in about 1 mm3 of tissue. By combining several single measurements over a tissue surface one can create an image of microcirculation of the tissue. This technique is called LDPI.Citation37–Citation39 The standard LDPI apparatus uses a movable mirror that directs the laser beam on the different measurement points, scanning the tissue surface of an area up to 50×50 cm2.Citation40 illustrates a principal model of the LDPI technique.
Figure 4 Laser Doppler perfusion imaging technique.
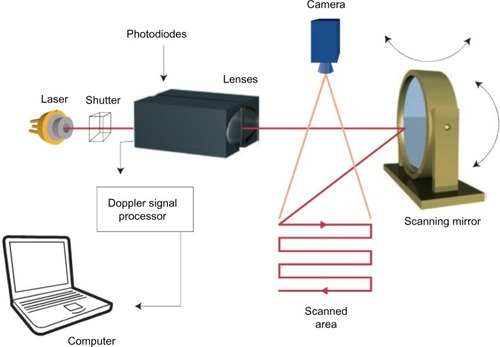
Using this approach of mapping with subsequent measuring points is both time consuming and produces large amounts of raw data requiring long processing times. Classically, this has impeded imaging of dynamic processes such as functional tests and increased the susceptibility to motion artifacts.Citation5,Citation36,Citation40 However, in recent years, the introductions of high-speed complementary metal-oxide semiconductor cameras in combination with new ways of mapping algorithms, have shortened measurement times for LDPI substantially.Citation41–Citation43 The experience of these high-speed detectors in clinical settings is still small as compared to the standard mapping techniques.
Measurement depth with LDPI is dependent on laser wavelength and tissue properties but ranges from 1 to 1.5 mm.Citation44 This is deeper than for LSCI (described in the LSCI section) and has to be accounted for when choosing a method for the specific tissue to be studied.
Applications
LDPI has been tested on a large range of organs, skin being the most frequent because of its easy accessibility. One dermatologic application is the use of LDPI for differentiation between malignant and benign skin lesions.Citation45 Further, several studies have been conducted studying different kinds of wounds, including ischemic ulcers, diabetic foot ulcers, and skin burn wounds.Citation46 Studying healing wounds, Gschwandtner et al, have shown increased perfusion in areas of granulation as a marker of angiogenesis, indicating that areas with low perfusion have diminished healing potential.Citation47
Equally, in evaluation of perfusion in skin burns, LDPI can be used to grade the severity of damage of the burnt tissue.Citation48 A high flux indicates superficial damage and low flux a deeper injury. This knowledge has been used to develop a prognostic tool for healing time and helping to decide which patients are in need of surgical treatment.Citation49 In general, prognostic information about healing potential may be used to assist in the choice of wound therapy.
In the pathogenesis of diabetic foot ulcers both microangiopathy and autonomous neuropathy contribute to development of ulcers. Besides studying the angiogenesis of the wound healing process, LDPI has been used to study the neural component of the pathogenesis. By using a functional test, using heating and cooling of fingers, the autonomous nerve function have shown to be impaired in the vessels of diabetic patients.Citation50
In addition, application on internal organs has been made in intra-operative settings. Hajivassiliou et al used LDPI on patients undergoing colectomies for inflammatory bowel disease and found inflammatory bowels to have an increased blood flow as compared to normal.Citation51 Studies implementing LDPI in neurosurgery have been carried out in animal models. In a model of cerebral ischemia, Liu et al used LDPI to monitor an increase in cerebral perfusion as a response to albumin infusion.Citation52
Advantages and limitations
On the contrary to videomicroscopy techniques, LDPI has the advantage of being a non-contact method, avoiding interaction with the studied tissue and thus avoiding the generation of pressure artifacts. Furthermore, the possibility of measuring large areas with a good spatial resolution gives LDPI a good reproducibility.Citation5,Citation53 However, measuring average Doppler shift, LDPI cannot evaluate absolute flow properties in eg, mL/s but rather measure relative comparisons of flux which are based on a normalized baseline value and presented as arbitrary flux units.Citation54
The LDPI apparatus requires calibration before measurement due to the presence of a biological zero signal. Even during complete flow occlusion, the LDPI output signal would not be zero due to noise in the Doppler signal, denoted as the biological zero. The noise has been suggested to consist of local redistribution of blood cells within the vessels and Brownian motions of molecules.Citation40 How to interpret and handle the biological zero is under debate and needs to be investigated further.Citation55,Citation56
In addition, the standard LDPI setting using a scanning technique requires measurement times of about a minute, which gives the method a low temporal resolution, unsuitable for functional tests. The new high-speed methods may provide a solution to the problem, but currently the clinical experience is low.
LSCI
Technology
Another laser based technique for microvascular imaging is LSCI. Laser light illuminating biological tissue gives rise to a light phenomenon called laser speckle.Citation57 Laser speckle arises from the irregularities in the structure of the tissue, which backscatter the laser light irregularly, creating dark and light interference patterns. These patterns can be detected by a CCD camera. The speckle pattern of tissue changes with movement within the tissue such as blood flow,Citation58 creating blurring of the image on the capturing camera. Speckle contrast is a quantification of the blurring at a given exposure time of the camera. Increased blood flow will increase image blurring thus lowering the contrast which is measured as the ratio of the standard deviation to the mean grayscale intensity in a square of pixels (eg, 5×5 pixels), thus representing the average velocity and concentration of moving particles in that square.Citation44 LDPI and LSCI are related methods but rely on different physical theories and have different ways of data sampling. LSCI does not use a scanning technique as LDPI but instead measures nearly instantly the whole tissue under study enabling fast measurements with a high sampling rate. As for LDPI, LSCI measures average velocity and data are presented in an arbitrary unit.
A correlation of perfusion results between the two methods at different perfusion rates has been made showing LSCI to have a non-linear relation to blood flow and to LDPI, especially at low blood flows.Citation59 The need for a new algorithm for comparison of the methods has been suggested.Citation60 However, LSCI measuring depth is more superficial at about 300 μm as compared to the measuring depth of 1–1.5 mm of LDPI, which has to be accounted for when choosing a measuring technique for specific organs.Citation40 On skin, for example, LSCI measures the more superficial slower flowing vessels while LDPI measures deeper, faster flowing vessels hence giving different results.
Applications
Briers and Fercher made the first clinical measurements with laser speckle photography on retinal perfusion in the 1980sCitation61 and later developments of the technique led to real-time perfusion imaging with LSCI.Citation62,Citation63 Having faster temporal resolution than standard LDPI setups, LSCI has proven a useful method in functional tests of skin microcirculation.Citation1 For instance, studies of burn injuries have correlated faster healing times with increased perfusion in the first week post-injury assessed with LSCI.Citation64 Further, LSCI has been of interest in the assessment of intraoperative cerebral microcirculation where local cerebral blood flow has been measured after revascularization surgery.Citation65 Bezemer et al have proposed LSCI for monitoring renal cortex ischemia/reperfusion injury.Citation6 LSCI has also proven useful in the assessment of hepatic microcirculation during liver resection surgery.Citation33,Citation66 A typical example of an LSCI measurement of human liver is shown in .
Advantages and limitations
An advantage of LSCI is its fast measurement time, nearly instantly measuring the microcirculation over a large area. This makes LSCI suitable for dynamic functional tests requiring high temporal resolution. Comparative studies of LDPI and LSCI have been made highlighting the advantages of faster measurement time with LSCI compared to LDPI.Citation40,Citation67 In addition, LSCI has good reproducibility.Citation5 Another advantage is that the method is non-contact, not interacting with the tissue under study.
However, LSCI is sensitive to movement artifacts.Citation1,Citation66 Techniques of handling these artifacts have been suggested by using an opaque reference area for subtraction of artifact signals.Citation68,Citation69
Relying on similar physical principles as LDPI, LSCI also suffers from a biological zero signal, but for LSCI it is even larger. Likewise, because the LSCI signal is based on the average velocity and concentration of RBC, values cannot be given in absolute numbers, but are limited to relative measurements.
Conclusion and future challenges
In this paper we have reviewed three different techniques for imaging the microcirculation and presented examples of several different clinical applications. When choosing a method for study of a specific organ and pathological process it is important to consider the different properties of the methods. To measure the dynamic processes of functional tests, imaging methods with high temporal resolution have to be used, thus methods with fast measuring times are required. Differences in measurement depth have to be considered as the differences in optical properties of tissues.
The non-contact methods do not affect the tissue with pressure. However, they are more susceptible to motion artifacts, which both LDPI and LSCI cannot automatically differentiate from the movements of RBC. Further, LDPI and LSCI measures relative flow properties and are in need of calibration due to a relatively large biological zero signal, which is not the case for videomicroscopy techniques. Videomicroscopy techniques also have the benefit of offering direct visualization of microcirculation. In , the major differences between the methods are presented.
Table 1 Overview of microcirculation imaging methods
Regardless of the choice of technique, a challenge lies in the interpretation of the measurement output and its relation to physiological and pathological processes. The methods offer several output variables and careful thought has to be given to which one is of importance. In an attempt to solve this problem, expert conferences have been carried out, trying to set standards for experimental settings and define output variables of each technique.Citation3,Citation54
Another challenge is to compare microcirculatory results between different methods. Several studies have been conducted, attempting to compare the results of different techniques.Citation33,Citation59,Citation60 These are important efforts for the understanding of the microvascular results and will make microcirculatory imaging more accessible for use in the clinical assessment of patients.
In the authors’ opinion, future improvement of imaging methods should focus on developing methods which allow measurement without interaction of the studied tissue, such as a non-contact method. In addition, measurements must be fast to decrease vulnerability to motion artifacts and making it possible to study dynamic processes. To simplify the interpretation of the output, data should be given in an absolute variable, for example flow in mL/s. Finally data analysis should be automatic.
Measurement of microcirculation can be applied to many organs in various pathologies. Above, examples of clinical fields of applications have been presented. The numerous skin applications described above are an example of how imaging techniques can be used to assist the clinician in staging, monitoring, and even predicting pathological processes and be a useful tool in the assessment of patients. Still, great potential lies in the implementation of microvascular imaging systems for intraoperative assessment and future studies may outline new fields of use.
Disclosure
The authors report no conflicts of interest in this work.
References
- RoustitMCracowskiJLAssessment of endothelial and neurovascular function in human skin microcirculationTrends Pharmacol Sci201334737338423791036
- RizzoniDAalkjaerCDe CiuceisCHow to assess microvascular structure in humansHigh Blood Press Cardiovasc Prev201118416917722283671
- De BackerDHollenbergSBoermaCHow to evaluate the microcirculation: report of a round table conferenceCrit Care2007115R10117845716
- CracowskiJLMinsonCTSalvat-MelisMHalliwillJRMethodological issues in the assessment of skin microvascular endothelial function in humansTrends Pharmacol Sci200627950350816876881
- RoustitMMilletCBlaiseSDufournetBCracowskiJLExcellent reproducibility of laser speckle contrast imaging to assess skin microvascular reactivityMicrovasc Res201080350551120542492
- BezemerRLegrandMKlijnEReal-time assessment of renal cortical microvascular perfusion heterogeneities using near-infrared laser speckle imagingOpt Express20101814150541506120639991
- De CiuceisCPorteriERizzoniDStructural alterations of subcutaneous small-resistance arteries may predict major cardiovascular events in patients with hypertensionAm J Hypertens200720884685217679031
- GrassiGSeravalleGScopellitiFStructural and functional alterations of subcutaneous small resistance arteries in severe human obesityObesity (Silver Spring)2010181929819521345
- RossiMMatteucciEPesceMPeripheral microvascular dysfunction as an independent predictor of atherosclerotic damage in type 1 diabetes patients: a preliminary studyClin Hemorheol Microcirc201354438139123089884
- ShermanHKlausnerSCookWAIncident dark-field illumination: a new method for microcirculatory studyAngiology19712252953035089888
- GronerWWinkelmanJWHarrisAGOrthogonal polarization spectral imaging: a new method for study of the microcirculationNat Med19995101209121210502828
- BiberthalerPLangerSLuchtingBKhandogaAMessmerKIn vivo assessment of colon microcirculation: comparison of the new OPS imaging technique with intravital microscopyEur J Med Res200161252553411772540
- MathuraKRVollebregtKCBoerKDe GraaffJCUbbinkDTInceCComparison of OPS imaging and conventional capillary microscopy to study the human microcirculationJ Appl Physiol (1985)2001911747811408415
- HarrisAGSinitsinaIMessmerKValidation of OPS imaging for microvascular measurements during isovolumic hemodilution and low hematocritsAm J Physiol Heart Circ Physiol20022824H1502H150911893588
- InceCSidestream dark field imaging: an improved technique to observe sublingual microcirculationCrit Care20059Suppl 172
- GoedhartPTKhalilzadaMBezemerRMerzaJInceCSidestream Dark Field (SDF) imaging: a novel stroboscopic LED ring-based imaging modality for clinical assessment of the microcirculationOpt Express20071523151011511419550794
- MicroVisionMedical [homepage on the Internet] Available from: http://www.microvisionmedical.com/Accessed June 1, 2013
- De BackerDCreteurJPreiserJCDuboisMJVincentJLMicrovascular blood flow is altered in patients with sepsisAm J Respir Crit Care Med200216619810412091178
- PaizeFSarginsonRMakwanaNChanges in the sublingual microcirculation and endothelial adhesion molecules during the course of severe meningococcal disease treated in the paediatric intensive care unitIntensive Care Med201238586387122398755
- LupiOSemenovitchITreuCBouskelaEOrthogonal polarization technique in the assessment of human skin microcirculationInt J Dermatol200847542543118412856
- LindeboomJAMathuraKRHarkisoenSvan den AkkerHPInceCEffect of smoking on the gingival capillary density: assessment of gingival capillary density with orthogonal polarization spectral imagingJ Clin Periodontol200532121208121216268996
- SchaserKDSettmacherUPuhlGNoninvasive analysis of conjunctival microcirculation during carotid artery surgery reveals microvascular evidence of collateral compensation and stenosis-dependent adaptationJ Vasc Surg200337478979712663979
- PenningsFAInceCBoumaGJContinuous real-time visualization of the human cerebral microcirculation during arteriovenous malformation surgery using orthogonal polarization spectral imagingNeurosurgery200659116717116823313
- den UilCABezemerRMirandaDRIntra-operative assessment of human pulmonary alveoli in vivo using Sidestream Dark Field imaging: a feasibility studyMed Sci Monit20091510MT137MT14119789519
- DubinAPozoMOFerraraGSystemic and microcirculatory responses to progressive hemorrhageIntensive Care Med200935355656419127356
- PuhlGSchaserKDVollmarBMengerMDSettmacherUNoninvasive in vivo analysis of the human hepatic microcirculation using orthogonal polorization spectral imagingTransplantation200375675676112660497
- NilssonJErikssonSBlindPJRisslerPSturessonCMicrocirculation changes during liver resection – a clinical studyMicrovasc Res201494475124840670
- BoermaECvan der VoortPHSpronkPEInceCRelationship between sublingual and intestinal microcirculatory perfusion in patients with abdominal sepsisCrit Care Med20073541055106017334238
- DondorpAMInceCCharunwatthanaPDirect in vivo assessment of microcirculatory dysfunction in severe falciparum malariaJ Infect Dis20081971798418171289
- PuhlGSchaserKDPustDInitial hepatic microcirculation correlates with early graft function in human orthotopic liver transplantationLiver Transpl200511555556315838880
- De BackerDOspina-TasconGSalgadoDFavoryRCreteurJVincentJLMonitoring the microcirculation in the critically ill patient: current methods and future approachesIntensive Care Med201036111813182520689916
- LindertJWernerJRedlinMKuppeHHabazettlHPriesAROPS imaging of human microcirculation: a short technical reportJ Vasc Res200239436837212187127
- SturessonCMilsteinDMPostICMaasAMvan GulikTMLaser speckle contrast imaging for assessment of liver microcirculationMicrovasc Res201387344023403398
- CernyVTurekZParizkovaROrthogonal polarization spectral imagingPhysiol Res200756214114716555953
- RivaCRossBBenedekGBLaser Doppler measurements of blood flow in capillary tubes and retinal arteriesInvest Ophthalmol197211119369444634958
- HumeauASteenbergenWNilssonHStrombergTLaser Doppler perfusion monitoring and imaging: novel approachesMed Biol Eng Comput200745542143517340155
- EssexTJByrnePOA laser Doppler scanner for imaging blood flow in skinJ Biomed Eng19911331891941870327
- NilssonGETenlandTObergPAEvaluation of a laser Doppler flowmeter for measurement of tissue blood flowIEEE Trans Biomed Eng198027105976046449469
- WardellKJakobssonANilssonGELaser Doppler perfusion imaging by dynamic light scatteringIEEE Trans Biomed Eng19934043093168375866
- O’DohertyJMcNamaraPClancyNTEnfieldJGLeahyMJComparison of instruments for investigation of microcirculatory blood flow and red blood cell concentrationJ Biomed Opt200914303402519566318
- SerovASteinacherBLasserTFull-field laser Doppler perfusion imaging and monitoring with an intelligent CMOS cameraOpt Express200513103681368919495275
- LeuteneggerMMartin-WilliamsEHarbiPReal-time full field laser Doppler imagingBiomed Opt Express2011261470147721698011
- DraijerMHondebrinkEvan LeeuwenTSteenbergenWTwente Optical Perfusion Camera: system overview and performance for video rate laser Doppler perfusion imagingOpt Express20091753211322519259158
- BriersJDLaser Doppler, speckle and related techniques for blood perfusion mapping and imagingPhysiol Meas2001224R35R6611761081
- StuckerMHorstmannINuchelCRochlingAHoffmannKAltmeyerPBlood flow compared in benign melanocytic naevi, malignant melanomas and basal cell carcinomasClin Exp Dermatol199924210711110233665
- KhanFNewtonDJLaser Doppler imaging in the investigation of lower limb woundsInt J Low Extrem Wounds200322748615866831
- GschwandtnerMEAmbrozyESchneiderBFaschingSWillfortAEhringerHLaser Doppler imaging and capillary microscopy in ischemic ulcersAtherosclerosis199914212252329920526
- NiaziZBEssexTJPapiniRScottDMcLeanNRBlackMJNew laser Doppler scanner, a valuable adjunct in burn depth assessmentBurns19931964854898292231
- PapeSABakerRDWilsonDBurn wound healing time assessed by laser Doppler imaging (LDI). Part 1: Derivation of a dedicated colour code for image interpretationBurns201238218719422115981
- FrecceroCSvenssonHBornmyrSWollmerPSundkvistGSympathetic and parasympathetic neuropathy are frequent in both type 1 and type 2 diabetic patientsDiabetes Care200427122936294115562210
- HajivassiliouCAGreerKFisherAFinlayIGNon-invasive measurement of colonic blood flow distribution using laser Doppler imagingBr J Surg199885152559462383
- LiuYBelayevLZhaoWBustoRBelayevAGinsbergMDNeuroprotective effect of treatment with human albumin in permanent focal cerebral ischemia: histopathology and cortical perfusion studiesEur J Pharmacol2001428219320111675036
- KlonizakisMManningGDonnellyRAssessment of lower limb microcirculation: exploring the reproducibility and clinical application of laser Doppler techniquesSkin Pharmacol Physiol201124313614321212723
- FullertonAStuckerMWilhelmKPGuidelines for visualization of cutaneous blood flow by laser Doppler perfusion imaging. A report from the Standardization Group of the European Society of Contact Dermatitis based upon the HIRELADO European community projectContact Dermatitis200246312914012000320
- ZhongJSeifalianAMSalerudGENilssonGEA mathematical analysis on the biological zero problem in laser Doppler flowmetryIEEE Trans Biomed Eng19984533543649509751
- Humeau-HeurtierAAbrahamPMaheGLinguistic analysis of laser speckle contrast images recorded at rest and during biological zero: comparison with laser Doppler flowmetry dataIEEE Trans Med Imaging Epub9162013
- RigdenJDGordonEIThe granularity of scattered optical maser lightProc IRE19625023672368
- SternMDIn vivo evaluation of microcirculation by coherent light scatteringNature1975254549556581113878
- TewGAKlonizakisMCrankHBriersJDHodgesGJComparison of laser speckle contrast imaging with laser Doppler for assessing microvascular functionMicrovasc Res201182332633221803051
- BinzoniTHumeau-HeurtierAAbrahamPMaheGBlood perfusion values of laser speckle contrast imaging and laser Doppler flowmetry: is a direct comparison possible?IEEE Trans Biomed Eng20136051259126523232361
- BriersJDFercherAFRetinal blood-flow visualization by means of laser speckle photographyInvest Ophthalmol Vis Sci19822222552597056639
- BriersJDWebsterSLaser speckle contrast analysis (LASCA): a nonscanning, full-field technique for monitoring capillary blood flowJ Biomed Opt19961217417923014683
- BriersJDRichardsGHeXWCapillary Blood Flow Monitoring Using Laser Speckle Contrast Analysis (LASCA)J Biomed Opt19994116417523015182
- LindahlFTesselaarESjobergFAssessing paediatric scald injuries using Laser Speckle Contrast ImagingBurns201339466266623092702
- HechtNWoitzikJDreierJPVajkoczyPIntraoperative monitoring of cerebral blood flow by laser speckle contrast analysisNeurosurg Focus2009274E1119795950
- ErikssonSNilssonJLindellGSturessonCLaser speckle contrast imaging for intraoperative assessment of liver microcirculation: a clinical pilot studyMed Devices (Auckl)20147425726125114599
- StewartCJFrankRForresterKRTulipJLindsayRBrayRCA comparison of two laser-based methods for determination of burn scar perfusion: laser Doppler versus laser speckle imagingBurns200531674475216129229
- MaheGAbrahamPLe FaucheurABruneauAHumeau-HeurtierADurandSCutaneous microvascular functional assessment during exercise: a novel approach using laser speckle contrast imagingPflugers Arch2013465445145823328863
- MaheGRousseauPDurandSBricqSLeftheriotisGAbrahamPLaser speckle contrast imaging accurately measures blood flow over moving skin surfacesMicrovasc Res201181218318821156183
- MoorInstuments [homepage on the Internet] Available from: http://gb.moor.co.uk/Accessed October 26, 2014