Abstract
Background
Differences in the thalamocortical system have been shown in patients with major depressive disorder (MDD). Given prior evidence of phenotypic heterogeneity by the age of onset in MDD, we examined whether differences in thalamocortical connectivity could identify biological subtypes of MDD defined by the age of illness onset.
Methods
A total of 94 subjects including 20 early-onset (EO) MDD (onset, 18 years), 34 adult-onset (AO) MDD, and 40 healthy controls (HCs) underwent resting-state functional MRI. Blood-oxygen-level-dependent time courses were extracted from six cortical regions of interest (ROIs) consisting of frontal, temporal, parietal, and occipital lobes and precentral and postcentral gyri. Each ROI’s time course was then correlated with each voxel in thalamus, while covarying out signal from every other ROI.
Results
The analysis of variance results showed significant main effects of group in frontal and temporal connectivity with thalamus. Group contrasts showed a right fronto-thalamic hypo-connectivity only in AO-MDD, but not in EO-MDD, when compared to HCs. However, direct comparison between EO-MDD and AO-MDD showed no differences. Furthermore, there was a right temporal–thalamic hyperconnectivity in both EO-MDD and AO-MDD patients relative to HCs. These results were not accounted for by sex, age, or illness burden.
Conclusion
The age of illness onset may be a source of heterogeneity in fronto-thalamic intrinsic connectivity in MDD.
Introduction
Major depressive disorder (MDD) is a prevalent and disabling mental disorder characterized by a variety of emotional and cognitive impairments that are associated with changes in brain function. MDD is a highly heterogeneous condition, with much variability in symptom type, illness severity, and course.Citation1 One source of heterogeneity that contributes to long-term morbidity is the age of onset of MDD. Preadult onset or early-onset (EO) MDD (under the age of 18 years) was found to be associated with greater illness severity, more depressive episodes, and increased suicidality as compared to patients with adult-onset (AO) MDD (onset age ≥18 years),Citation2 suggesting that the illness onset age could be representative of different clinical subtypes. Besides clinical features, findings from MRI studies have also shown to be distinct in EO-MDD compared with AO-MDD patients, further validating the clinical subtypes based on the onset age. Volumetric differences have been predominantly noted in genu of corpus callosum, subgenual cingulate cortex, and hippocampus and in cortical thickness of superior temporal cortex between EO-MDD and AO-MDD patients.Citation3–Citation5 Furthermore, fronto-striatal functional compensation during cognitive control was found to be less substantial in youth MDD patients compared with older individuals with MDD.Citation6
The thalamus is a critical brain region implicated in depression and a hub for multiple streams of sensory, perceptual, cognitive, and emotional processing.Citation7 The thalamo-cortical circuit has consistently been identified as the primary network associated with the underlying psychopathology of MDD.Citation8–Citation11 A number of studies have demonstrated substantial volumetric abnormalities in the cortico-striatal-pallidal-thalamic circuits, fronto-thalamic white matter (WM) connectivity, and resting-state functional connectivity of thalamocortical circuits in MDD.Citation12–Citation16 One lesion study has shown an association between thalamic damage and cognitive and emotional deficits, highlighting a potentially causal link between disturbed thalamic function and MDD psychopathology.Citation17
Previous work from our group has demonstrated abnormalities in thalamocortical connectivity in adult MDD patients.Citation18 Our earlier study showed hyperconnectivity between medial thalamus and temporal regions and between medial thalamus and somatosensory areas in MDD patients compared with healthy controls (HCs). Furthermore, there was also a positive correlation between thalamotemporal connectivity and the severity of symptoms. This study extended our previous work in examining whether there is heterogeneity in thalamocortical connectivity based on the age of onset subtypes given that the neurodevelopment of thalamus and that of cortex are closely linked and that we know that alterations in the developmental trajectories of limbic and striatal regions are associated with depression onset.Citation19,Citation20 Ultimately, this work could later be used to identify thalamocortical circuit abnormalities as imaging markers of clinical subtypes and therefore could contribute to a better understanding of the underlying neurological changes in these subtypes to work toward more effective treatment targets.
The aim of this study was to use resting-state functional MRI (fMRI) to compare thalamocortical connectivity between EO-MDD (<18 years) and AO-MDD (≥18 years) in a group of medication-free MDD adult patients. We hypothesized that there would be regionally specific differences in thalamocortical connectivity between EO-MDD and AO-MDD patients, which would also differ from a group of HCs. More specifically, in the light of the findings from a systematic review of fMRI studies that cognitive abnormalities were more pronounced in late onset or AO than in EO-MDD,Citation21 we predicted that the most prominent group differences would be seen in functional connectivity between thalamus, and frontal and temporal regions as these circuits have been implicated in cognitive control in MDD.Citation6
Method
Participants
In total, 54 patients diagnosed with MDD, according to the Structured Clinical Interview for the Diagnostic and Statistical Manual of Mental Disorders Axis-1 Disorders (SCID for DSM-IV),Citation22 were recruited. The Hamilton Rating Scale for Depression (HAM-D)Citation23 was administered to all the patients in order to determine the severity of depressive symptoms. The primary inclusion criteria were that the patients had to have received a score of ≥18 on the HAM-D and had an acute episode of MDD. The patients were also required to be free from psychotropic medication for at least 3–4 weeks prior to recruitment. Three patients were medication-naïve, and all others had previously been exposed to antidepressant medication. Exclusion criteria included the presence of other Axis I disorders, history of substance abuse within the last 6 months, any neurological or unstable medical disorders, severe suicidal thoughts, or treatment resistance to three trials of different antidepressant medications. The patients were divided into two subgroups according to their age of illness onset, with an EO group (onset before the age of 18 years) and AO group (onset at or after the age of 18 years), with 20 in the EO group and 34 in the AO group. The study psychiatrist (RR) determined the age of onset based on participants’ self-report on at what age they began to experience the constellation of symptoms that would fulfill the criteria for MDD.
A group of 40 HC participants were also recruited and screened for Axis I psychiatric disorders, current or past, using the SCID for DSM-IV. Exclusion criteria included a family history of Axis-I disorders, a neurological disorder, or substance abuse within the last 6 months. The study was approved by the Conjoint Health Research Ethics Board of the University of Calgary. All the participants gave written informed consent before taking part, and all research procedures in this study were performed in accordance with the Declaration of Helsinki.
MRI data acquisition
MRI data were acquired across two different scanners. Resting-state fMRI blood-oxygen-level-dependent (BOLD) images from 45 MDD and 18 HC participants were collected using a 3T General Electric MR scanner (Signa VHi; General Electric Healthcare, Waukesha, WI, USA) using an eight-channel, phased-array head coil. Five minutes of resting data were acquired using a single-shot gradient-recalled echo, echo planar imaging (EPI) sequence (150 volumes, repetition time [TR]/echo time [TE] =2,000/30 ms, 24×24 cm field of view, flip angle =65°, 30 slices of 4 mm thickness). In addition, a T1-weighted structural MRI (TR =9.2 ms, TE = minimum, flip angle =20°, 180 slices at a resolution of 1 mm) was acquired for anatomical registration of the functional data. Resting-state fMRI BOLD images of nine MDD and 22 HC participants were collected using 3T General Electric MR scanner (Discovery™ MR750; General Electric Healthcare, Waukesha, WI, USA). Of these participants, the MDD patients had the same scanning parameters and protocol as that from the other scanner. All HC participants scanned on the Discovery scanner had a total of 5 minutes of resting-state data, acquired with an EPI sequence (119 volumes, TR/TE =2,500/30 ms, 24×24 cm field of view, flip angle =77°, 40 slices of 3 mm thickness). A T1-weighted structural MRI (TR =8.2 ms, TE = minimum, flip angle =18°, at a resolution of 1.3 mm) was also acquired for anatomical registration. In all scans, the participants were asked to remain as still as possible in the MRI scanner with their eyes open and focused on a fixation cross at the center of the projection screen. They were asked to relax, not to think about anything in particular, and to stay awake.
Image preprocessing and thalamocortical connectivity analysis
Preprocessing of functional images included brain extraction, spatial smoothing with a Gaussian filter full-width-half-maximum of 5 mm, and slice timing correction with a Hanning Window Sinc Interpolation and a temporal high-pass filter of 100 seconds. Then, functional images were coregistered to individual subject’s anatomical images and then to a standard Montreal Neurological Institute (MNI) template. Each participant’s functional image was segmented into gray matter, WM, and cerebrospinal fluid (CSF) using manual tracing, of which the time series were later used as temporal covariates in the general linear model (GLM). The FMRIB Software LibraryCitation24 was used for processing all the images. Global brain signal was not regressed out due to reports of the thalamus highly correlating with this signal.Citation25
After preprocessing, six cortical regions of interest (ROIs) were defined using the Oxford Thalamic Connectivity Atlas,Citation26 following a similar approach used by Zhang et al.Citation25,Citation27 This consisted of six cortical regions shown to have specific anatomical connections with thalamic regions, ie, 1) frontal, 2) motor (including primary and premotor), 3) somatosensory, 4) temporal, 5) parietal, and 6) occipital cortices. illustrates these six ROIs. BOLD time courses were extracted from each cortical ROI and used as separate seeds in a seed-to-voxel functional connectivity analysis to reveal correlations in activity between each cortical seed and each voxel in the thalamus. GLMs were created for each cortical seed where activity in every other cortical ROI was covaried out to identify the unique contribution in the functional connectivity of that particular cortical ROI. Movement parameters, WM, and CSF time courses were also included in the GLMs as variables of no interest in the first-level analysis. As we used two different scanners to acquire the MRI data, we included scanner and age and sex in the GLMs as variables of no interest in the second-level analysis to control for their potential confounding effects. Group mean statistical maps of functional connectivity were then created for each cortical ROI, for each group (HC, EO-MDD, and AO-MDD), showing connectivity between each cortical ROI and each voxel in the thalamus. To have a more conservative and thus more robust test for group differences, an F-test (analysis of variance [ANOVA]) was performed with all three groups’ statistical maps to identify clusters that showed main group effects. Following this, post hoc analyses were performed in which two group contrasts were performed to reveal the direction of effects and the group that drove the effects. Only the clusters that emerged from the group contrasts that overlapped with clusters identified in the ANOVA result were considered to be significant.
Figure 1 Statistical maps of thalamocortical connectivity in EO-MDD and AO-MDD patients and HC: (A) six cortical ROIs used as seed regions in the seed-to-voxel analysis of thalamocortical connectivity; (B) the first three rows show group mean clusters from HCs and EO-MDD and AO-MDD patients. The fourth row displays significant clusters showing a main effect of group from ANOVA results; (C) significant clusters emerging from group contrasts (showing only clusters that overlap with group main effects from ANOVA results); (D) overlaid group mean clusters to demonstrate differences in the spatial distribution of thalamocortical connectivity regions in the thalamus across all the three groups.
Abbreviations: ANOVA, analysis of variance; AO, adult onset; EO, early onset; FWE, family-wise error; HC, healthy control; MDD, major depressive disorder; ROIs, regions of interest.
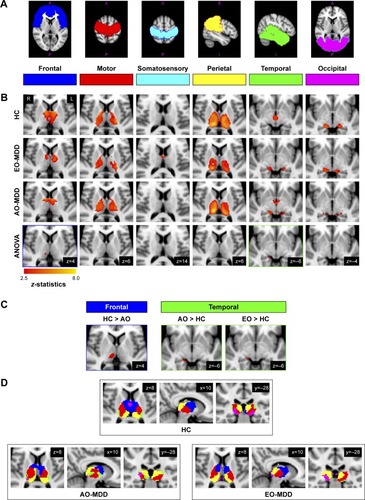
shows all statistical maps (group means, ANOVA main effects, and group contrasts), which were thresholded at a voxel-wise α level of P<0.005. Correction for multiple comparisons was done for group means and contrasts using small volume correction with the SPM WFU Pickatlas toolboxCitation28 using a corrected α level of P=0.001 family-wise error (cluster size >6 voxels), considering only voxels within the Harvard–Oxford thalamus atlas.Citation26
Results
shows the demographic and clinical characteristics of patients in each group. The age, sex, age of MDD onset, and the number of episodes significantly differed between groups.
Table 1 Demographic and clinical variables
Table 2 Cluster table showing thalamocortical group differences from group contrasts (only clusters that overlap with those showing the main effects of the group from ANOVA comparing HC, AO-MDD, and EO-MDD patients)
Thalamocortical connectivity in EO-MDD/AO-MDD groups
displays statistical maps for group means for HC, EO-MDD, and AO-MDD groups and main effects from the ANOVA results comparing the three groups. Significant main effects of groups emerged from the ANOVA between frontal (MNI coordinates for local maxima: x=10, y=−22, z=4; cluster size =4; z-score =2.69) and temporal (MNI coordinates for local maxima: x=18, y=−32, z=−8; cluster size =12; z-score =3.03) connectivity with thalamus. More specifically, the main effect of the group in thalamofrontal connectivity was localized in the right mediodorsal/midline thalamic nucleus, and the main effect of group in thalamotemporal connectivity was localized in the right posterior ventral part of the pulvinar. The fourth row of displays the results from the ANOVA and the regions of connectivity within the thalamus showing the main effects of the group.
Age of onset and thalamocortical connectivity
The post hoc analysis of group contrasts in regions that showed significant effects from the F-test showed a signifi-cantly decreased connectivity between right mediodorsal/midline thalamus and frontal cortices in the AO-MDD group, but not in the EO-MDD group, when compared to HCs. Second, both AO-MDD and EO-MDD groups exhibited an increased connectivity between right posterior ventral thalamus and temporal cortices. It is worth noting that the size of the cluster that showed a hyperconnectivity between temporal cortices and thalamus was larger in the AO-MDD group, when compared to the EO-MDD group. lists the significant clusters, and shows the statistical maps for significant illness onset effects. shows group mean clusters overlaid on one another for HC, EO-MDD, and AO-MDD groups, in order to illustrate the spatial distribution of thalamocortical connectivity patterns.
In order to control for illness burden, and as we see a significant difference in the number of depressive episodes between EO-MDD and AO-MDD groups, we performed an additional analysis using the number of episodes as a variable of no interest. After controlling for the number of episodes, we did not see any substantial change in the main ANOVA results or group contrasts. We also checked for correlations between age and z-scores of thalamofrontal and thalamo-temporal connectivity (r(53)=0.051, P=0.713; r(53)=0.009, P=0.948, respectively), in order to demonstrate minimal confounding effects of age on our results.
Discussion
In this study, we sought to investigate whether there are differences in the thalamocortical connectivity between EO-MDD and AO-MDD patients and how each group differed from HCs. Our main findings first showed reduced connectivity between frontal cortex and medial thalamus only in AO-MDD patients, but not in the EO-MDD group, when compared to HC. Second, both AO-MDD and EO-MDD groups showed a greater thalamotemporal connectivity between temporal cortex and posterior thalamus when compared to HCs. Another notable finding was that the effects were not accounted for by the current age, sex, or illness burden, suggesting that the effects may have been specific to the age of onset per se. Our results have shown both differential and shared abnormalities in thalamocortical connectivity in EO-MDD and AO-MDD. The differences in thalamofrontal connectivity may help to validate clinical subtypes based on the illness onset age.
Taking into account the direct relationship between dorsomedial thalamus–prefrontal abnormalities and cognitive impairment,Citation29 the observed decreased connectivity between frontal cortex and thalamus in AO-MDD is partly in agreement with the reported greater cognitive impairment in AO-MDD relative to youth MDD.Citation21 This thalamofrontal hypoconnectivity seems to be driven by the AO group as this finding was much weaker and emerged only at a lower threshold than in our previous study that investigated MDD adult patients as a whole group including both EO-MDD and AO-MDD patients.Citation18 It is noteworthy that this finding could not be accounted for higher age in AO-MDD than in EO-MDD or HC, and therefore, it is more likely related to the adult age of onset in MDD. However, it remains unclear why this abnormality was absent in EO-MDD. One possibility is that prolonged exposure to antidepressants in the context of longer duration of illness in EO-MDD than in AO-MDD might have normalized this abnormality in EO-MDD. Another possibility is that thalamic abnormalities may be rare in EO-MDD as the neurodevelopmental trajectory suggests nonlinear increases in thalamic volumes during childhood and adolescence and linear volumetric decreases in thalamus starting in the early adulthood continuing into the old age.Citation30 This might explain why the volumetric reductions were more pronounced in late-onset MDD than in EO-MDD.Citation12 In addition, the structural and neurochemical abnormalities in thalamus were reported in pediatric patients with obsessive–compulsive disorder but not in pediatric patients with MDD.Citation31,Citation32
The increased connectivity between thalamus and temporal cortex in both EO-MDD and AO-MDD groups may reflect impaired emotional memory adaptation and memory modulations through thalamo-amygdalaCitation33 and thalamohippocampal pathwaysCitation34 and impaired social cognition through thalamic connections with anterior temporal lobes.Citation35 This shared abnormality in thalamotemporal hyperconnec-tivity may also subserve the severity of illnessCitation18 and negative bias in MDD,Citation36,Citation37 which occur as a universal characteristic of depressive psychopathology, regardless of the age of onset.
Limitations
The main limitation of the study was the use of retrospective self-reports of age of onset of MDD, which we cannot guarantee were absolutely accurate. The recall bias might have caused overdiagnosis or false diagnosis of MDD, especially in the EO group. Hence, it is possible that the patients who had a less severe form of depression (subclinical depression) and high neuroticism might have been included in the EO-MDD group. As discussed in the previous section, we cannot rule out the possible effects of long-term antidepressant use on thalamocortical connectivity. However, all the participants had refrained from taking medication in 3–5 weeks leading up to the scanning; therefore, we can at least say that the results were not driven by acute drug effects. Furthermore, our results showed some overlap between cortical seed regions used in the ROI analysis, which may have accounted for the lack of significant clusters in connectivity between somatosensory cortex and thalamus. Future studies may seek to identify smaller subregions of thalamocortical connectivity to look for more localized abnormalities in MDD. There was a substantial difference in age between groups in our study, and although we tried to control for this by including age as a covariate of no interest, we still may have not fully accounted for all the potential confounding effects of age. We did use two scanners to collect the imaging data, but believe that the effects of scanner changes were minimal, especially as we used a similar scanning protocol across scanners, and we used scanner as a covariate of no interest in our analysis. Given these methodological limitations and small sample size, our findings should be considered preliminary until replicated in a large sample. Our research question would also benefit from future longitudinal studies that could have the potential for finding more causal links between changes in thalamocortical connectivity and the age of illness onset in depression. However, here, we presented a first step that could provide crucial clues for further investigation.
Conclusion
We first demonstrated that AO-MDD exhibited a specific thalamofrontal hypoconnectivity not seen in EO-MDD, which may be a marker for dissociating EO-MDD and AO-MDD subtypes. Second, we showed thalamotemporal hyperconnectivity in both EO-MDD and AO-MDD groups, which appears to be not related to the age of onset, but more generally related to the underlying psychopathology of MDD. Our study provides a further support to investigating the neural underpinnings of MDD subgroups based on the age of illness onset.
Acknowledgments
We acknowledge the contribution of Bradley Goodyear, Ismael Gaxiola, and Filomeno Cortese from the Seaman Family MR Research Center for imaging data acquisition, storage, and quality assurance. The authors wish to thank all the participants who volunteered their time for this study. This work was supported by an investigator initiated grant from Astra Zeneca awarded to Dr Ramasubbu. The funding agency (Astra Zeneca) had no role in the design of the study, collection, and analysis of data and in the decision to publish it. He also received an investigator initiated grant from Pfizer. He has served as a member of the advisory committee for Lundbeck and Janssen and received honorarium. Dr Mac-Queen has received honoraria from Pfizer, Janssen, Lilly, Allergen, and Lundbeck. The authors Dr Brown and Dr Clark are currently supported by fellowships from Alberta Innovates Health Solutions, and Dr Brown was supported by a fellowship from the Mathison Centre for Mental Health Research & Education for a part of the duration of data analysis and manuscript preparation.
Disclosure
This work was supported by investigator initiated grant by Astra Zeneca to Dr. Rajamannar Ramasubbu. The authors report no other conflicts of interest in this work.
References
- MerikangasKRWickiWAngstJHeterogeneity of depression. Classification of depressive subtypes by longitudinal courseBr J Psychiatry J Ment Sci1994164342348
- ZisookSRushAJAlbalaAFactors that differentiate early vs. later onset of major depression disorderPsychiatry Res2004129212714015590040
- KempAMacmasterFPJaworskaNAge of onset and corpus callosal morphology in major depressionJ Affect Disord2013150270370623769291
- JaworskaNMacmasterFPYangX-RInfluence of age of onset on limbic and paralimbic structures in depressionPsychiatry Clin Neuroscis20146812812820
- JaworskaNA preliminary study of the influence of age of onset and childhood trauma on cortical thickness in major depressive disorderBioMed Res Int2014
- RaoJAKasselMTWeldonALThe double burden of age and major depressive disorder on the cognitive control networkPsychol Aging201530247548526030776
- HaberSNThe primate basal ganglia: parallel and integrative networksJ Chem Neuroanat200326431733014729134
- DrevetsWCVideenTOPriceJLPreskornSHCarmichaelSTRaichleMEA functional anatomical study of unipolar depressionJ Neurosci1992129362836411527602
- PriceJLDrevetsWCNeurocircuitry of mood disordersNeuropsychopharmacology201035119221619693001
- ShepherdGMCorticostriatal connectivity and its role in diseaseNat Rev Neurosci201314427829123511908
- SwerdlowNRKoobGFDopamine, schizophrenia, mania, and depression: toward a unified hypothesis of cortico-striato-pallido-thalamic functionBehavioral and Brain Sciences19871002197208
- BoraEHarrisonBJDaveyCGYücelMPantelisCMeta-analysis of volumetric abnormalities in cortico-striatal-pallidal-thalamic circuits in major depressive disorderPsychol Med201242467168121910935
- WebbCAWeberMMundyEAKillgoreWDReduced gray matter volume in the anterior cingulate, orbitofrontal cortex and thalamus as a function of mild depressive symptoms: a voxel-based morphometric analysisPsychol Med201444132833284325066703
- GreiciusMDFloresBHMenonVResting-state functional connectivity in major depression: abnormally increased contributions from subgenual cingulate cortex and thalamusBiol Psychiatry200762542943717210143
- JiaZWangYHuangXImpaired frontothalamic circuitry in suicidal patients with depression revealed by diffusion tensor imaging at 3.0 TJ Psychiatry Neurosci201439317017724119793
- LuiSWuQQiuLResting-state functional connectivity in treatment-resistant depressionAm J Psychiatry2011168664264821362744
- LiebermannDOstendorfFKoppUASubjective cognitive-affective status following thalamic strokeJ Neurol2013260238639622854887
- BrownECClarkDLHasselSMacqueenGRamasubbuRThalamocortical connectivity in major depressive disorderJ Affect Disord201721712513128407555
- WhittleSLichterRDennisonMStructural brain development and depression onset during adolescence: a prospective longitudinal studyAm J Psychiatry2014171556457124577365
- JonesEGThe ThalamusCambridgeCambridge University Press2007
- KerestesRDaveyCGStephanouKWhittleSHarrisonBJFunctional brain imaging studies of youth depression: a systematic reviewNeuroimage Clin2014420923124455472
- FirstMGibbonMWilliamsJStructured Clinical Interview for DSM-IV-TR Axis I Disorders, Research Version, Patient Edition (SCID-I/P)New York, NYBiometric Research, New York State Psychiatric Institute2002
- HamiltonMDevelopment of a rating scale for primary depressive illnessBr J Soc Clin Psychol1967642782966080235
- SmithSMJenkinsonMWoolrichMWAdvances in functional and structural MR image analysis and implementation as FSLNeuroimage200423Suppl 1S208S21915501092
- ZhangDSnyderAZFoxMDSansburyMWShimonyJSRaichleMEIntrinsic functional relations between human cerebral cortex and thalamusJ Neurophysiol200810041740174818701759
- BehrensTEJohansen-BergHWoolrichMWNon-invasive mapping of connections between human thalamus and cortex using diffusion imagingNat Neurosci20036775075712808459
- ZhangDSnyderAZShimonyJSFoxMDRaichleMENoninvasive functional and structural connectivity mapping of the human thalamo-cortical systemCereb Cortex20102051187119419729393
- MaldjianJALaurientiPJKraftRABurdetteJHAn automated method for neuroanatomic and cytoarchitectonic atlas-based interrogation of fMRI data setsNeuroimage20031931233123912880848
- ParnaudeauSBolkanSSKellendonkCThe mediodorsal thalamus: an essential partner of prefrontal cortex for cognitionBiol Psychiatry201783864865629275841
- WalhovdKBFjellAMReinvangIEffects of age on volumes of cortex, white matter and subcortical structuresNeurobiol Aging20052691261127016005549
- KimJJGrey matter abnormalities in obsessive-compulsive disorder: statistical parametric mapping of segmented magnetic resonance imagesBr J Psychiatry J Ment Sci2001179330334
- SmithEARussellALorchEIncreased medial thalamic choline found in pediatric patients with obsessive-compulsive disorder versus major depression or healthy control subjects: a magnetic resonance spectroscopy studyBiol Psychiatry200354121399140514675804
- RomanskiLMLedouxJEEquipotentiality of thalamo-amygdala and thalamo-cortico-amygdala circuits in auditory fear conditioningJ Neurosci19921211450145091331362
- VertesRPHooverWBSzigeti-BuckKLeranthCNucleus reuniens of the midline thalamus: link between the medial prefrontal cortex and the hippocampusBrain Res Bull200771660160917292803
- OlsonIRMccoyDKlobusickyERossLASocial cognition and the anterior temporal lobes: a review and theoretical frameworkSoc Cogn Affect Neurosci20138212313323051902
- HaoLYangJWangYNeural correlates of causal attribution in negative events of depressed patients: evidence from an fMRI studyClin Neurophysiol201512671331133725487911
- SeidelEMSatterthwaiteTDEickhoffSBNeural correlates of depressive realism – an fMRI study on causal attribution in depressionJ Affect Disord2012138326827622377511