Abstract
The localized surface plasmon resonance (LSPR) property of gold nanoparticles (GNP) has been exploited in a variety of optical sensor configurations including solution-based bioassays, paper-based colorimetric detection, surface-confined nanoparticle film/array-based sensing, etc. Amongst these, gold nanostructured films are of great interest because of their high stability, good reproducibility, robustness, and cost-effectiveness. The inherent optical characteristics of GNP, are attributed to parameters like size and shape (eg, nanospheres, nanorods, nanostars), eg, LSPR spectral location sensitivity to the local environment, composition (eg, gold–silver or silica–gold nanoshells), sensing volume, mesospacing, and multiplexing. These properties allow sensor tunability, enabling enhanced sensitivity and better performance of these biosensors. Ultrasensitive biosensor designs were realized using gold nanostructured films fabricated by bottom-up as well as top-down approaches. In this review, we describe the past, present, and future trends in the development of GNP-LSPR-based sensors, concentrating on both design (fabrication) and application. In the process, we have discussed various combinations of GNP size and shape, substrate, and application domains.
Introduction
Over the last few decades, progress in transducer fabrication technology coupled with an increase in understanding of biomolecular interactions has led rapid strides in biosensor development. Such ultrasensitive biosensors have leveraged on the exquisite specificity, affinity, and versatility of biomolecular interactions. A variety of biosensor matrices has been incorporated on various types of physicochemical transducers. Among the different types of transducers, optical biosensors have been studied extensively because of their potential advantages over the other sensing modes.Citation1 Amongst these, sensors based on surface plasmon resonance (SPR) have evinced significant interest amongst researchers due to the fact that such detection systems neither require any fluorescent labels nor do they require light to pass through the analyte medium.Citation1 In addition, the overall detection process is accelerated with the potential to detect multiple targets in an unknown, complex biological sample.Citation2 As a result, a number of biochemical sensors have been explored on the basis of SPR properties of different metallic thin films.Citation2,Citation3
In parallel with advancements in SPR sensors, several researchers studied the plasmonic properties of gold colloids. The optical properties of gold colloids were empirically known for hundreds of years. Colloidal gold was used by ancient Romans and during the medieval era for tinting glass. It was also popular among alchemists for some of its medicinal values. In a breakthrough study in 1857, Faraday reported the formation of deep red solutions of colloidal gold by reduction of an aqueous solution of gold salt using phosphorus in carbon disulphide.Citation4 Five decades later, in 1908, the nature of optical extinction and scattering by spherical noble metal nanoparticles was theoretically modeled by Mie, in one of the most widely cited articles in this sphere of science.Citation5 According to this theory, the extinction spectrum of a spherical nanoparticle is expressed as the sum of a series of vector spherical harmonics of the electromagnetic fields of the incident wave, scattered wave, and the particle wave. Mie’s theory also accounts for the field retardation effects observed when the particle size becomes comparable to the wavelength of the photon. Other approximation theories such as discrete dipole approximationCitation6 and finite-difference time domainCitation7 have been proposed to study the optical properties of nonspherical metallic nanoparticles. After the theoretical explanation of optical properties, metallic nanoparticles (eg, gold, silver) attracted researchers of all domains to explore their applications.
It is now well accepted that the plasmonic properties of metallic nanoparticles (eg, gold, silver) arise from localized surface plasmons (LSP) on the atoms of colloid surfaces caused by interaction with photons ().Citation8 This phenomenon is typically present only when the particle size is smaller than the wavelength of the incident light. The resonance frequency of LSP, ie, LSPR, in metallic nanoparticles is intrinsically the same characteristic as manifested in SPR, and the resonance frequency is determined by the complex dielectric function of the metal and the dielectric constant of the surrounding medium.Citation9
Although both SPR and LSPR have been exploited equally for sensor development by measuring refractive index (RI) changes, nanoparticle-based LSPR sensors have a number of advantages over thin film-based SPR sensors, most of which will be apparent in the later sections of this article. However, it should be mentioned upfront that the instrumentation for such sensors is potentially easier than that for SPR-based sensors. Further, since the LSPR peak may be tuned or changed by changing size and shape of gold colloids, multiplexed sensing is possible using different gold colloids on a single sensor matrix. The configuration of these sensors also allows the detection of molecules of very low (<1 kD) molecular weight, which is uncommon with thin-film SPR sensors.Citation10 It has been seen that silver nanoparticles display sharper and more intense LSPR bands and thereby higher RI sensitivity than gold nanoparticles. However, the higher chemical stability of gold has resulted in a wider preference for this noble metal for biosensing applications.
The optical properties of GNP due to the LSP have been instrumental in the realization of several optical biosensor platforms. Two distinct classes of plasmonic biosensors have emerged. The first class of biosensors is based on localized field enhancement of electromagnetic signals interacting with LSP. This has been exploited in surface-enhanced Raman scattering,Citation11,Citation12 surface-enhanced fluorescence,Citation13 and thin metal film SPR-based biosensors.Citation14,Citation15 The other class is based on the RI sensitivity of LSP, which has been utilized in the development of LSPR biosensors.Citation9 With a suitable receptor immobilized on the surface of GNP, an LSPR sensor can detect target molecules even if the molecules do not have a spectral signature in the visible–NIR range.
Earlier, free gold colloid-based optical biosensors, ie, aggregation-based bioassays, were established for qualitative and quantitative determination of biomolecular interactions.Citation16,Citation17 By using this approach, researchers have been able to achieve attomolar to femtomolar sensitivity.Citation18 However, uncertainty with respect to nanoparticle concentration during the multiple washing steps (to remove unbound analytes) and low shelf stability of gold colloids have resulted in a limited number of applications evolving from this technique. It may be noted that a number of factors (solvent, stabilizer, surfactant, pH, etc) might affect the stability of the gold colloids. On the other hand, immobilized gold colloid-based sensing has shown immense potential to develop versatile sensors with enhanced performance, eg, stability, sensitivity, selectivity, reusability, and the ability to be multiplexed for complex analyte sensing. In the past few years, several excellent reviews have been published that discuss the experimental and theoretical aspects of nanometric particle optics as well as the use of metallic nanoparticles in sensing applications.Citation8,Citation19–Citation25 This review complements those by discussing the fabrication and application of LSPR-based biosensors, with emphasis on immobilization of gold colloids. We discuss the characteristics of gold colloids (size, shape, and composition) that influence biosensor performance. We also review some recent advances in the construction of gold colloid-based LSPR biosensors and their applications in immunosensing, nucleic acid detection, and quantification of toxins.
Synthesis strategies of gold colloid
Numerous routes have been suggested to synthesize gold colloids with tunable plasmonic properties. The most common approach is the reduction of a metal salt in solution to form noble metal colloidal suspension. Size control is generally achieved by varying the molar ratio of the metallic salt and reducing agent. Some important strategies for synthesis of different types of gold colloids are summarized in .
Table 1 Synthesis methods for different types of gold colloids
The last decade has witnessed some top-down techniques such as electron beam lithography,Citation46,Citation47 focused ion beam lithography,Citation48 nanosphere lithography (NSL),Citation49 nanoimprint lithography,Citation50,Citation51 and soft interference lithographyCitation52 developed to fabricate gold nanostructures on a substrate. These techniques have provided greater control on size, shape, and interparticle spacing of gold nanostructures and hence proved their potential for fabricating microarrays. However, some of these techniques are very expensive compared to the direct use of capped gold colloids.
The assembly of gold colloids on solid sensor substrates is generally carried out by chemical binding and exploiting the high affinity of gold toward amino, cyano, and thiol functional groups. However, other strategies such as electrostatic interactions between oppositely charged surfaces and metallic nanoparticles have also been used. A self assembled layer of GNP yields an optically transparent monolayer, where the assembly is stabilized by the opposite forces of strong attractive colloid surface interactions and repulsive colloid–colloid electrostatic interactions.Citation27,Citation53,Citation54
Characteristics of gold colloids and their influence on sensor performance
The LSPR properties of gold colloids are strongly dependent on their composition, size, shape, spatial arrangement, and RI of the dielectric medium around them. The environmental sensitivity of GNP was first demonstrated by Englebienne 1998, who fabricated a biosensor based on colorimetric detection.Citation55 Later Okamoto et alCitation56 showed that the absorbance of an immobilized monolayer of gold colloids was sensitive to RI of the surrounding solvent. In this section, we have focused on the characteristics of gold colloids, which have been exploited in development of biosensors by various groups of researchers.
Size and shape
It has been mentioned earlier that the size and shape of gold colloids can be controlled by slight modification of reaction protocol, reaction conditions, and molar concentrations of reactants.Citation39,Citation41 This has an impact on the spectral signature of the gold colloids in the UV–vis–NIR range and has led to the development of a variety of biosensors. A change in size and shape of gold colloids modulates the wavelength of maximum extinction (λmax) and the bandwidth. It has been observed that a red shift in the peak wavelength as well as an increase in bandwidth occurs with an increase in the particle size.Citation57 This is largely due to phase-retardation effects in larger nanoparticles and the contribution of scattering to the extinction coefficient. For larger nanoparticles, contribution of scattering to the extinction spectrum dominates that of absorption and results in an increase in the extinction intensity, a red shift in λmax, and an increase in the bandwidth. In addition, increased polydispersity in a collection of larger GNP manifests itself in a broadening of the extinction spectrum.Citation58
In some of the recent studies, researchers have optimized the size and shape of gold colloids to develop high performance LSPR sensors. For example, Nath and Chilkoti found that sensors fabricated with 39 nm diameter spherical gold nanoparticles (sGNP) exhibited maximum sensitivity to bulk RI change.Citation57 These researchers suggested that the fractional coverage of sensor surface increased as a function of the particle size, and for particles of 39 nm size, it was more than 1.5 times as compared to 12 nm particle size.
In the past few years, gold colloids of different shapes have been created, including spheres, rods, stars, crescents, decahedra, and bipyramids (). Some of these shapes have displayed much higher sensitivity toward the bulk RI changes than nanospheres. This can be attributed to the shape-related plasmonic properties that are tunable from UV–visible to NIR range. It has also been suggested that the sensitivity of the plasmon band to RI changes is solely a function of the wavelength of the plasmon band for particles of a given composition and that nanoparticles with a plasmon band at higher wavelengths are more sensitive to their local environment than those at shorter wavelengths.Citation59 In addition to greater RI sensitivity, higher LSPR wavelength avoids background absorption and scattering due to endogenous chromophores from biological mixture serum, blood, etc. This indicates that nanospheres may have the least sensitivity amongst nanoparticles of different shapes but constant volume.
Gold nanorods (GNR) exhibit stronger and tunable absorption (corresponding to the longitudinal plasmon oscillation) and the possibility of unidirectional plasmon propagation.Citation60,Citation61 GNR exhibit two distinct plasmon bands, one associated with the transverse (λ = 520 nm) mode and the other with the longitudinal mode (usually λ ≥ 600 nm). This property of GNR has motivated researchers to take interest in controlled assembly of GNR into functional architectures as well as for analyte-sensing applications. Marinakos and colleaguesCitation62 observed that bulk RI senstivity of GNR was more than four times that of GNP. Gold nanorods (aspect ratio [AR] ~2.25)-based LSPR chips showed wavelength sensitivity of 252 nm/RIU, while the sensitivity of LSPR chips fabricated from 39 nm diameter sGNP was 60 nm/RIU. On comparing different AR it was found that RI sensitivity increased as a function of AR and reached 366 nm/RIU sensitivity for GNR with mean AR = 5.2.Citation63 Jain and colleagues have also demonstrated experimentally and theoretically that the longitudinal plasmon wavelength was highly sensitive to change in the dielectric properties of the surroundings and that the sensitivity increases as the AR of the nanorods increases.Citation61
Recently, gold bipyramids (GBP) have been employed to develop LSPR sensor chips.Citation42 This sensor showed higher figure of merit (FOM), ie, sensitivity divided by the LSPR full width at half-maximum, values of 3.5–3.9 compared to GNR substrates. It was attributed to the monodispersity of GBP and sharp tips with a potential for strong field enhancement. Similar to GNR-based sensors, sensitivity of these GBP-based sensors increased with mean AR. GBP with AR of 2.5 exhibited 289 nm/RIU sensitivity, while AR of 3.2 resulted in 381 nm/RIU sensitivity.
Nehl et al synthesized star-shaped GNPs using a seed-mediated surfactant-directed method.Citation43 Spectroscopic measurements revealed that these nanoparticles exhibited multiple plasmon resonances resulting in polarization-dependent scattering with multiple spectral peaks, which correspond to the different tips on the star-shaped structure. The plasmon resonances were also found to be extremely sensitive to the local dielectric environment. The authors reported that individual gold nanostars displayed 1410 meV/RIU dielectric sensitivity, and the FOM for these particles was 10.7, which was higher than that previously observed for any other colloid.
Composition
Similar to the different anisotropic GNP, researchers have been able to produce gold colloids of different composition, eg, gold nanoshells (GNS). The plasmonic properties of GNS can be tuned systematically in the NIR region of the spectrum (700–1300 nm) by changing the relative dimensions of the core and shell layers. Thus, the LSPR peaks of these GNS are considerably red-shifted as compared to sGNP having the same dimensions. In addition, GNS exhibit high RI sensitivity compared to sGNP of similar size. For example, GNS (mean size = 50 nm; wall thickness = 4.5 nm) showed 408.8 nm/RIU sensitivity, which was ~7 times more when compared to sGNP with roughly the same dimensions.Citation40 The enhanced sensitivity is due to the thin walls of GNS and changes in the dielectric property of hollow nanostructures. It has also been suggested that the sensitivity of GNS (silica– gold) increases largely with overall nanoparticle size and to a lesser extent with core/shell ratio. For silica–gold nanoshells, 555.4 nm/RIU sensitivity has been observed, which is similar to or greater than the sensitivities achieved with other gold colloid types.Citation64
Sensing volume
An important consideration in the design of an LSPR sensor is the quantification of distance from the surface of the nanoparticles at which the particle can no longer detect a change in the RI. As all receptor–analyte binding events occur within a short, but variable, distance of the nanoparticle– solution interface, this distance must be commensurate with the dimension of the receptor–analyte pair to maximize sensitivity. The range of this effective analytical volume depends on the size and shape of gold colloids.
It is known that the electromagnetic field of nanoparticles decays with the distance from the surface and is dependent on the size and anisotropy of the nanoparticles. Okamoto et alCitation56 experimentally observed that the peak absorbance and the resonance wavelength are highly sensitive to variations in the thickness of additional layers up to ~10 nm, beyond which the response seems to saturate. Larger nanoparticles possess greater sensing volume compared to smaller nanoparticles although the change in sensing distance is not linearly related to the size of the GNP. It has been demonstrated that sGNP of 12 nm size could detect a RI change up to 24 nm away from the nanoparticle surface, whereas 39 nm-sized sGNP could detect RI change at a distance greater than 40 nm.Citation57 Similarly, GNR (74 nm in length and 33 nm in diameter, AR = 2.25)-based LSPR chips could detect a RI change to a distance of at least 40 nm with the most sensitivity in the first 10 nm of their surface.Citation62 Therefore, binding of a large antigen to an antibody that is immobilized on the nanoparticle can be detected using comparatively larger nanoparticles.
Mesospacing
Controlled lateral spacing between GNP on a sensor surface, ie, mesospacing, plays a vital role for precise control of the sensor performance. Interparticle spacing affects the sensitivity and the electric field distribution around the nanoparticles. For plasmonic wavelength shift-based assays, it has been proved experimentally and by computational modeling that greater average interparticle spacing results in higher wavelength sensitivity and larger sensing volume due to a wider spread of intensified electric field.Citation65 Nath and Chilkoti found that the extinction sensitivity of LSPR sensors (based on the extinction measurements at a single wavelength) increased linearly as a function of the surface density of the immobilized nanoparticles on the sensor substrates and approaches saturation.Citation57 It can be observed from both these studies that the sensitivity and sensing distance depend crucially on the surface density or surface coverage of the nanoparticles.
Multiplexing
Currently, microarrays and microchips are gaining importance in the present age of multiplexed sensing schemes. Gold nanostructure-based biosensing platforms have shown immense potential in the development of multiplexed biosensors due to their tunable plasmonic properties. Researchers have developed a multithroughput LSPR (MLSPR) chip by immobilizing five kinds of GNR (differing in AR) on silanized glass slides.Citation66 These sensor chips exhibited five plasmon peaks implying a possibility to detect five acceptor–ligand interactions simultaneously in the wavelength range from 530 to 940 nm (). Further, the authors successfully used MLSPR sensor to detect two different antibody–antigen interactions simultaneously.
Figure 2 Scheme of multithroughput localized surface plasmon resonance. Copyright © 2009, Elsevier. Reproduced with permission from Huang H, He C, Zeng Y, et al. A novel label-free multi throughput optical biosensor based on localized surface plasmon resonance. Biosens Bioelectron. 2009;24:2255–2259.
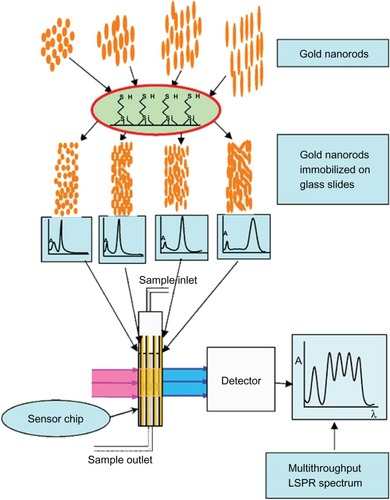
Recently, researchers fabricated hybrid sensor chips using three different types of functionalized metallic nanoparticles that exhibit LSPR peaks at different wavelengths.Citation67 The three types of metallic nanoparticles used in this study were silver nanoparticles, sGNP, and nanoshells (silver–gold) with their corresponding LSPR peaks at 427, 534, and 772 nm, respectively. These hybrid chips were shown to be able to detect nine types of volatile organic compounds with various functional groups, demonstrating their applicability toward detection of complex analytes with multiple molecules of interest.
Biosensor applications based on LSPR of gold colloids
Decades ago, significant amount of research in gold colloids and functionalization of such colloids for biosensing resulted in sensing systems using aggregation-based assays.Citation16,Citation17 These gold colloid assays were popular because of their low cost and ease of use. Over the past decade, the transduction and signal capture from LSPR-based biosensors have evolved through the research of several groups around the world. These nanobiosensors have proved their versatility in various applications such as clinical and environmental monitoring, biotechnology, and food safety. These sensors have been widely employed to monitor a broad range of analyte–surface binding interaction such as adsorption of small molecules, ligand–receptor interactions, antibody–antigen interactions, DNA and RNA hybridization, protein–DNA interaction, biomarkers, toxin detection, etc. Different types of transducer designs and their fabrication protocols have been proposed along with claims toward their potential advantages. Among these, most of the designs are in the form of chips, while others include fiber optic probes, lab-on-chip integrated with microfluidic, and cuttlebone-derived matrix. Applying the LSPR phenomenon of gold colloids in different sensor designs, researchers have demonstrated the detection of some medically relevant analytes in clinical samples at picomolar level. In this section, we have focused on the recent developments and the applications of LSPR biosensors. Although some research groups are still engaged in developing cost-effective in vitro diagnostic strips,Citation68,Citation69 we have excluded such types of qualitative bioassays from this review.
Immunosensor applications
Gold colloid-based LSPR sensors have been used for quantification of a variety of analytes using antigen-antibody interactions (). Chilkoti’s group fabricated nano-SPR chips by exploiting the LSPR properties of sGNP and GNR.Citation57,Citation62,Citation70 These chips have shown good sensitivity for immunobiosensor applications. After immobilizing biotin on the sGNP of diameter 39 nm, these nano-SPR chips were able to detect 0.83 nM streptavidin. When GNR (AR ~2.25) were used in nano-SPR chips, the detection limit of 94 pM was achieved, which was much lower than that of sGNP. The GNR-anchored nano-SPR chips exhibited LSPR peaks in the NIR region. These biotin-functionalized LSPR chips were employed for bioassays of streptavidin in serum sample. The minimum detection limit of 19 nM that was achieved was possible due to lack of interferences in the NIR region by the carrier fluid (viz, serum). Further, the same group proposed the use of a single GNR as a plasmonic transducer for the detection of biomolecular interactions.Citation89,Citation90 The lowest streptavidin concentration that was experimentally measured was 100 pM, which induced a mean resonant wavelength shift of 0.59 nm. Analytical modeling of the same proved that the predicted minimum detection limit is 18 streptavidin molecules for this sensor, which was in good agreement with the experimental results.Citation90 In another study, GNR of higher AR (~5.2) were used to fabricate immunosensor chips.Citation63 These chips displayed 25 ng/mL (4.2 × 10−10 M) limit of detection for streptavidin, which was lower than those with sGNP. Association equilibrium constant (K) value was obtained as 1.2 × 107 M−1 for biotin-streptavidin binding, which is equal to the typical binding constant. In a recent study, researchers have been able to achieve a linear equation between the concentration of antigen and the intensity of the longitudinal plasmonic band of GNR.Citation91 These LSPR chips detected 0.1 pM of anti-goat IgG using goat IgG as a probe.
Table 2 Immunosensors based on localized surface plasmon resonance of gold colloids
Gold nanorods are commonly synthesized by a seed-mediated, surfactant-directed method.Citation37,Citation38 The most commonly used surfactant is cetyl trimethylammonium bromide (CTAB), which acts as both the source of anisotropic growth and the stabilizer. Due to the presence of CTAB, further chemical manipulation of the gold colloids becomes more complicated than that of classic citrate-stabilized gold colloids. Hafner’s group suggested PEGylation of the nanorods to displace CTAB.Citation79,Citation92 These PEGylated GNR were found to be stable in solution. In addition, their bioconjugation is easier compared to CTAB-protected GNR, and processing of these nanorods on the sensor substrates leads to well-ordered films (). The PEGylated GNR (AR ~3.3)-anchored LSPR chips have been demonstrated for the detection of anti-rabbit IgG using rabbit IgG as the probe moiety.Citation79 The immunoreaction was monitored through shifts in the LSPR spectral extinction peak. The limit of detection of this sensor was observed to be 1 nM. The equilibrium constant, Keq, was found to be 2.0 × 109 M−1, which is the typical equilibrium constant for an antigen-antibody interaction.
Figure 3 Scanning electron microscopy of films of PEGylated gold nanorods. Copyright © 2008, American Chemical Society. Reproduced with permission from Mayer KM, Lee S, Liao H, et al. A label-free immunoassay based upon localized surface plasmon resonance of gold nanorods. ACS Nano. 2008;2:687–692.
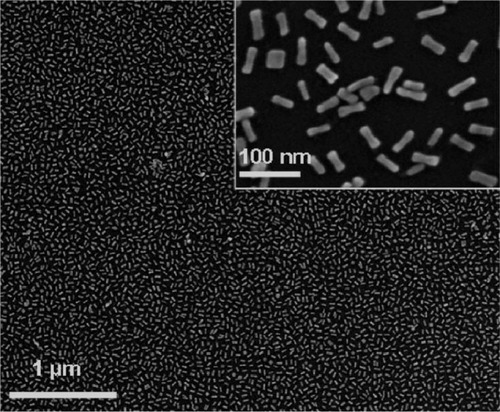
Similar to GNR, GNS exhibit LSPR peaks in the NIR range, depending upon their size and core to shell ratio. This is useful for chip-based bioassays of blood samples since most of the blood constituents display absorbance in the UV–visible range and not in the NIR region. GNS-based chips have been designed to quantify biotin-streptavidin interactions in diluted whole blood ().Citation80 Using LSPR peak wavelength at 730 nm, the sensors displayed a detection limit of 3 μg/mL with a wide dynamic range (3–50 (μg/mL).
Figure 4 Schematic of fabrication process of the gold nanoshell self-assembled monolayers and the analysis of streptavidin in diluted blood sample. Copyright © 2008, Elsevier. Reproduced with permission from Wang Y, Qian W, Tan Y, Ding S. A label-free biosensor based on gold nanoshell monolayers for monitoring biomolecular interactions in diluted whole blood. Biosens Bioelectron. 2008;23:1166–1170.
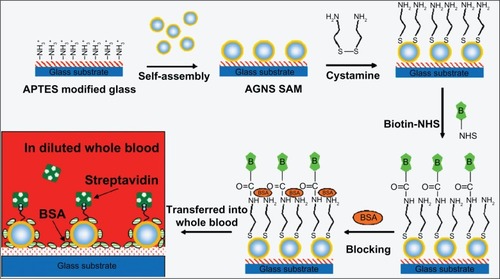
Recently, GBP have been employed to fabricate LSPR chips in order to exploit their high bulk RI sensitivity.Citation42 During real time kinetic studies, at 1 nM concentration, the extinction peak shifted at a rate of 4.0 × 10−5 nm/sec, and at 10 nM, the rate increased to 5.5 × 10−4 nm/sec. These bipyramid peak shift rates were larger than those using nanorods (2.1 × 10−5 and 2.1 × 10−4 nm/sec, respectively) by a factor of two. An immunoassay sensitivity constant was found to be KLSPR = 0.01 nm•μm2 for GBP.
Tamiya’s group fabricated core-shell nanostructured LSPR chips, using silica nanoparticle as the “core”, and the outer “shell” was constructed by depositing a gold layer ().Citation73,Citation76,Citation77,Citation93 After biofunctionalization, these LSPR chips have been used for the detection of a variety of analytes. By using anti-fibrinogen antibody-immobilized LSPR biochips, a 10 ng/mL detection limit of fibrinogen was achieved. These biochips have been adapted for multiarrays applications, for screening of bimolecular interactions.Citation93 Parallel biomolecular detection using immunoglobulins (IgA, IgD, IgG, IgM), C-reactive protein, and fibrinogen was demonstrated. These multianalyte sensor chips achieved a detection limit of 100 pg/mL. Later, this chip was modified to develop a casein immunosensor after immobilizing anti-casein antibody.Citation76 Under optimum conditions, the detection limit of the casein immunosensor was determined to be 10 ng/mL. Recently, these chips were further employed to detect specific reactions between the antibodies and the cell metabolite, IL-2.Citation77 In this case, the detection limit was 10 pg/mL. Further, the researchers demonstrated the time-course analysis of cell metabolism using the isolated cells from mouse thymus.
Figure 5 A) Schematic representation of core-shell (silica–gold) nanostructures on the glass substrate. Furthermore, the gold (Au) layer was formed on the nanoparticle layer using thermal evaporation. B) Optical characteristics of the gold-capped nanoparticle layer substrate. Copyright © 2008, Elsevier. Reproduced with permission from Endo T, Yamamura S, Kerman K, Tamiya E. Label-free cell-based assay using localized surface plasmon resonance biosensor. Anal Chim Acta. 2008;614:182–189.
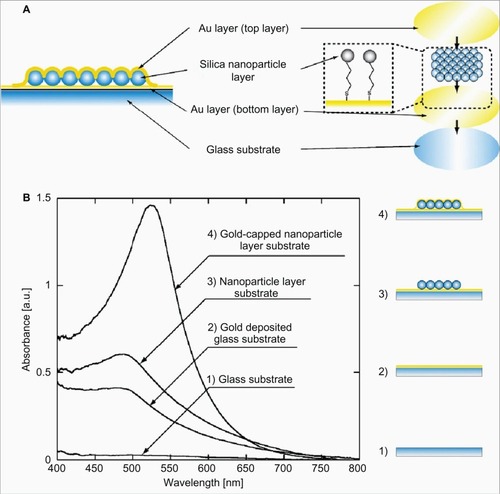
Advancing the applications of core-shell gold nanostructures, Tamiya’s group fabricated an innovative sensing nanostructure, which exhibited a phenomenon termed as interference localized surface plasmon resonance (iLSPR), and used it for configuring sensor chips.Citation78,Citation87,Citation94–Citation96 This iLSPR was based on plasmonic GNP with photonic thin-film multilayers of porous aluminium oxide and aluminium (Al) on a substrate. In this iLSPR design, the high refection Al substrate and the gold-nanoparticle layer caused multiple interactions of the incident light with the nanostructure similar to a Fabry–Perot resonator. These iLSPR chips have shown the capability of simultaneous monitoring of the changes in both the LSPR and the interferometric characteristics. Initially, these sensors were used for the detection of thrombin, using a sandwich-type assay. Two different aptamers were used, which bind to different sites of thrombin. The limit of detection was found to be 1 nM. More recently, Hiep et alCitation87 demonstrated specific interaction of biomolecules including biotin, avidin, and 5-fluorouracil (5-FU) and its antibody, anti-5-fluorouracil (anti-5-FU). This iLSPR biosensor was able to detect nanogram level of proteineous analytes.
The RI sensitivity of LSPR chips has been improved by three-dimensional GNP assemblies on the substrates.Citation88 Exploiting this innovative approach, recently three-dimensional LSPR chips have been fabricated by layer-by-layer deposition of GNP and mercaptosilanized multiwalled carbon nanotubes (MWCNT) on a glass substrate.Citation92 Mercaptosilanized MWCNT acted as a three-dimensional linker to assemble the GNP and also provided a large surface area and multiple binding sites. This sensor matrix allows high accessibility of target molecules due to the microporous nature of MWCNT. These three-dimensional chips showed 20 times higher sensitivity than the two-dimensional monolayer chips for streptavidin (0.5 nM) and anti-human serum albumin (3.33 nM).
Advances in the design of cost-effective, label-free, portable biosensors have led to the development of LSPR-based fiber optic sensors. Various geometric configurations have been suggested to enhance the sensitivity of the LSPR sensors. As a result, tipped,Citation97 straight,Citation88 tapered,Citation98 and U bentCitation85 fiber optic probes designs have emerged. Cheng and Chau fabricated a colloidal gold-modified fiber optic sensor and demonstrated its applicability as an RI sensor.Citation71 These biotin-functionalized LSPR fiber-optic probes achieved a detection limit of 98 pM for streptavidin, which is less than that achieved by the nano-SPR chip reported by Nath and Chilkoti.Citation57 In another approach, a tip-based miniaturized fiber optic biosensor has been demonstrated by coating the distal end of a 50-μm fiber core diameter with GNP.Citation97 Tip-based fiber optic LSPR sensor has been demonstrated for detecting biotin–avidin interactions for avidin concentrations of 20 μg/mL. Sai et alCitation85 reported a U shaped LSPR probe for immunosensing applications (). These LSPR probes reached the detection limit of 0.8 nM for anti-IgG on IgG-functionalized surface.
Figure 6 GNP-coated U bent fiber optic probe for immunosensor application. Copyright © 2009, Elsevier. Reproduced with permission from Sai VVR, Kundu T, Mukherji S. Novel U-bent fiber optic probe for localized surface plasmon resonance based biosensor. Biosens Bioelectron. 2009;24:2804–2809.
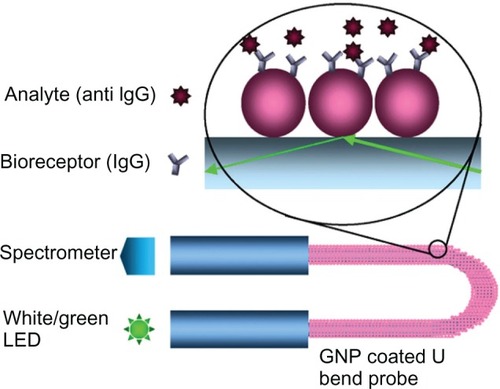
Monolayers of gold colloids are generally assembled using organosilanes as linkers. Silanization of sensor substrates is a time-consuming process and may require significant pretreatment as well as post-treatment. Recently, the use of polyelectrolyte (PE) multilayers has been suggested for assembling gold nanostructures on a sensor substrate.Citation88 PE films, eg, poly(diallyldimethyl ammoniumchloride), on gold nanostructures produce a weak negatively charged surface on which nonspecific electrostatic adsorption of streptavidin could be eliminated.Citation82 An LSPR sensor matrix having poly(diallyldimethyl ammoniumchloride)-coated GNP has been employed for immunosensing applications using biotin–streptavidin specific interaction. The detection limit of streptavidin in a microfluidic channel was found to be 100 ng/mL.Citation82 In another study, GNP immobilization on fiber optic sensor probes was achieved on a PE matrix composed of a trilayer of 3 different PEs [poly(diallyldimethylammonium chloride), poly(propyleneammonium chloride), and poly(styrene sulfonic acid sodium)] ().Citation88 This LSPR sensor matrix has shown good stability and reproducibility. Further, this fiber optic LSPR sensor showed promising potential for immunosensor applications. By modifying the sensor surface with rabbit IgG, the lowest detectable concentration was found to be 11.1 ng/mL of goat anti-rabbit IgG.
Figure 7 Trilayer of polyelectrolytes with immobilized sGNP on fiber optic probe. Copyright © 2010, MDPI. Reproduced with permission from Shao Y, Xu S, Zheng X, Wang Y, Xu W. Optical fiber LSPR biosensor prepared by gold nanoparticle assembly on polyelectrolyte multilayer. Sensors. 2010;10:3585–3596.
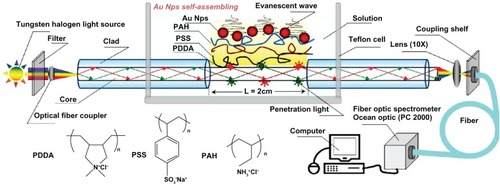
Gold colloids have been used in conjunction with a compact microfluidic platform, enabling a fusion of plasmonics with microfluidics, for which a new term “plasmofluidics” has been coined.Citation83 This leads to both surface plasmon-based “lab-on-a-chip” and fluid-based active plasmonics. These devices have demonstrated the capability of transporting a specific amount of biosamples into the sensing chambers to achieve sensitive and specific biosensing with decreased reaction time and less reagent consumption. Such devices, used as immunosensors, have demonstrated a detection limit of 270 ng/mL of antibiotin analyte.Citation84
Kitano’s group developed LSPR sensor chips for recep-tor–ligand interactions and quantification.Citation72,Citation74 The researchers demonstrated selective binding of concanavalin A (Con A) to the sugar residues of glycolipids immobilized on nanogold slides, with a nanomolar detection limit.Citation72 In another study, the same group studied the sensing capability of colloidal gold monolayer modified with polymers containing phenylboronic acid terminals for detection of glycoproteins.Citation74 These sensor chips showed a concentration-dependent binding of ovalbumin with a detection limit of 100 nM. Later, Guo et alCitation75 suggested that incorporation of an additional monolayer (eg, alkylthiol) between the receptor (ie, glycolipid) monolayer and the transducer (ie, gold colloids) might improve the sensor performance. Integration of a 1-dodecanethiol layer between the gold colloids and the glycolipids lowered the minimum detection concentration of Con A to 0.1 nM.
Nucleic acid sensor
Miniaturized nucleic acid sensors using microarrays and biochips have received a lot of attention lately, particularly in the field of genomics and proteomics. Such devices are based on detection of supramolecular interactions, ie, hybridizations, that occur between complementary oligonucleotides, one linked to a solid surface (probe DNA) and the other one to be analyzed (target DNA). The LSPR property of gold colloids has been exploited to develop nucleic acid biosensors ().
Table 3 Nucleic acid sensors based on localized surface plasmon resonance of gold colloids
Endo et al developed an LSPR-based DNA biosensor using a gold-capped nanoparticle layer immobilized with peptide nucleic acids.Citation99 Hybridization interactions with target oligonucleotides could be detected down to a limit of 0.677 pM of target DNA. Selective discrimination against a single base mismatch was also achieved. In a similar fashion, a multispot gold-capped nanoparticle array (MG-NPA) chip has been fabricated recently and used for point mutation detection.Citation102 Under optimal conditions, these MG-NPA chips achieved a picomolar limit of detection for target DNA. Also, selective discrimination against a single-base mismatch DNA sequence was achieved by using both homozygous and heterozygous DNA samples.
Cao et alCitation100 have developed an optical nanobiosensor using 24 nm sGNP and successfully performed real time DNA detection. Monoclonal antibodies, c-Myc, bound nanosensor showed a linear response for pCMV-Myc plasmid detection ranging from 6.2 to 20.0 ng/μL concentration (R2 = 0.99). The detection limit of the DNA plasmid was 2.4 ng/μL. The authors reported that the response concentration range and the detection limit were lower compared to the conventional methods, showing that this nanosensor could be applied to the detection of oncogenes.
Toxin biosensors
In the past few decades, a variety of pesticides has been used indiscriminately, which have posed serious environmental risks by affecting aquatic ecosystems and endangering human health as well.Citation32 In addition to pesticides and related toxins, heavy metals and their compounds, typically discharged as industrial effluents and contaminating surface and ground water, are another category of serious environmental and clinical concern. Ingestion of such heavy metals, even in minute quantities, through food and water can cause severe health problems, including anemia, birth defects, mental retardation, and behavioral disorders. Most commonly used techniques such as liquid and gas chromatography are time consuming, and a significant amount of pretreatment of the sample is generally required. A demand to rapidly detect these organophosphorous toxins in the environment as well as in human body fluids has been addressed by LSPR biosensors. Gold colloid-based LSPR sensors have also been successfully demonstrated to detect various toxic heavy metals and other toxins () as a first step toward remediation of such problems.
Table 4 Toxin sensors based on Localized surface plasmon resonance of gold colloids
A fiber optic biosensor for detecting paraoxon (an organophosphorous pesticide) has been developed using sGNP covalently coupled with acetylcholinesterase (AChE).Citation32 The sensor, based on inhibition of the enzyme, showed a linear response in the range of 1-100 ppb with 0.234 ppb limit of detection. Also, the biosensor retained 94% of its original activity after six cycles of inhibition with 500 ppb paraoxon followed with reactivation of AChE by 0.5 mM 2-pyridine-aldoxime methoiodide. The biosensor conserved its activity and produced reproducible results after storage in a dry state at 4°C for 60 days. Later, the same group designed a biofunctionalized fiber optic LSPR nanobiosensor for toxic heavy metal detection, eg, lead and cadmium.Citation103,Citation104 The researchers found that the absorbability of the monoclonal antibody (K55061M) anchored to the LSPR sensor increased to 12.2% upon changing the Pb(II)-EDTA level from 10 to 100 ppb with a detection limit of 0.27 ppb.Citation103 For cadmium detection, phytochelatins (PCs) peptides were chosen as probe moiety because PCs are capable of forming chelates with Cd(II) by thiolate coordination.Citation104 These PC-functionalized fiber optic LSPR sensors showed a sensitivity of 1.24 ppb−1 and a detection limit of 0.16 ppb.
An optical fiber-based LSPR sensor has been explored for the detection of staphylococcal enterotoxin B (SEB).Citation105 This LSPR biosensor achieved a lowest limit of detection of ~1.4 pM, which compares favorably with conventional immunological methods such as western immunoblot and enzyme-linked immunosorbent assay and competing biosensor technologies such as propagating SPR sensors, bidiffractive grating sensors, and fluorescence-based sensors. Recently, the use of triangular hybrid Au–Ag nanoparticles array was proposed for biosensing of SEB ().Citation106 Hybrid nanoparticles were constructed on a glass substrate employing NSL. Further, these arrays were demonstrated for SEB detection at nanogram per milliliter sensitivity using monoclonal anti-mouse SEB-anchored hybrid arrays.
Figure 8 Schematic of localized surface plasmon resonance sensor representing the detection of SEB on mouse anti-SEB functionalized triangular Ag–Au nanoparticles. Copyright © 2009, Elsevier. Reproduced with permission from Zhu S, Dua C, Fu Y. Localized surface plasmon resonance-based hybrid Au–Ag nanoparticles for detection of Staphylococcus aureus enterotoxin B. Opt Mater. 2009;31:1608–1613.
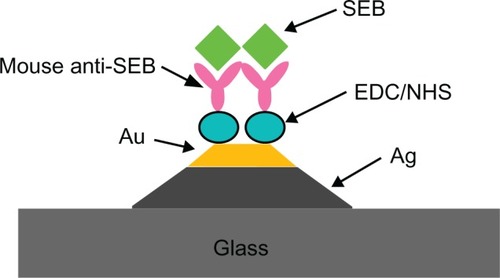
Miscellaneous biosensor applications
Lee et al fabricated a tapered optical fiber-based nanoprobe for the real-time RI measurement of a single live cell by exploiting the evanescent wave-based excitation of the localized plasmons of GNP.Citation98 By measuring the optical intensity scattered from the nanoparticles, the probe showed intensity sensitivity up to ~5400% per RIU. These fiber optic nanoprobes were tested to detect the RI of 3T3 fibroblast cell by inserting the probe into the cytoplasm. The RI measured by the localized plasmonic effect was 1.3506, which was consistent with the results of RI measurements obtained using holographic methods. Some small molecular weight molecules, eg, glucose, have been detected using LSPR of gold colloids ().
Table 5 Miscellaneous sensing applications based on localized surface plasmon resonance of gold colloids
Recently, an LSPR biosensor has been fabricated on a cuttlebone-derived matrix substrate (CDMS) using sGNP and was employed for glucose detection.Citation107 As CDMS is a three-dimensional chamber-like structure with naturally abundant amino groups, sGNP can be immobilized directly without surface modification steps. Also, the sGNP-immobilized CDMS framework resulted in effective optical signal transduction and improved the sensitivity of the detection system. The biosensor depends on the catalysis of glucose by glucose oxidase, which produces hydrogen peroxide. The peroxide produced reduces chloroauric acid. All the reactions happened in free medium and none of the ingredients were immobilized on the sGNP. The reduction of chloroauric acid caused deposition of gold on the immobilized sGNP of size 3–5 nm, thereby causing enlargement of the sGNP. This, as discussed earlier, predictably causes a shift in the LSPR peak. A reaction with glucose (5 × 10−5 M) for 20 minutes resulted in enlargement of GNP up to ~12 nm due to reduction of the gold salt by H2O2. The detection range of this biosensor was from 5 × 10−6 to 5 × 10−5 M glucose concentrations.
Kreuzer et alCitation108 developed an LSPR-based rapid and cost-effective analytical method for the quantitative determination of steroid hormone (stanozolol), which is usually analyzed by chromatographic methods under standard laboratory conditions. In this assay, stanozolol-conjugated bovine serum albumin was used as a probe to specifically bind stanozolol (preincubated with stanozolol polyclonal antiserum [α-StpAb]). The detection limit of this system was determined to be 2.4 nM (0.7 μg/L) of stanozolol, a value below the maximum allowed level set by the International Olympic Committee for banned substances.
Current trends
In the past few years, researchers have developed green chemistry to synthesize GNP using a nontoxic and environmental friendly approach. Taking advantage of this approach, researchers have developed methods for in situ GNP synthesis and immobilization on sensor substrates. For example, Zheng et alCitation109 reported in situ synthesis of GNP on egg shell membrane (ESM) to develop an LSPR sensor. The formation of GNP on ESM protein fibers was attributed to the reduction of Au (III) ions to Au (0) by the aldehyde moieties of the natural ESM fibers.
The inability of sGNP to quantify micron-sized analytes has restricted their use to more qualitative detection schemes. As an illustration, one might consider the example of a recent attempt to detect Salmonella via LSPR using GNP-immobilized chips fabricated through lithography.Citation110 These chips showed a good qualitative response to the analytes, but quantification of the Salmonella cells was difficult in this sensor configuration, probably due to the fact that the maximum electromagnetic decay length (probe depth) of LSPR associated with GNP is of the order of a few nanometers. However, in the near future, the quantification of micron-sized pathogenic organisms might be possible by exploiting the capabilities of plasmonic nanorods metamaterial (probe depth = 500 nm).Citation111
Recently developed nanosandwiches (eg, gold–silica–gold, gold–cobalt–gold)Citation112,Citation113 and hybrid nanostructures (eg, Fe3O4/Au)Citation114 have demonstrated both plasmonic and magneto-optical properties. These combined functionalities in a single nanoarchitecture have introduced a platform for the advancement of magnetoplasmonic nanobiosensors.
Conclusion
In this review, we have highlighted several current research accomplishments in the domain of GNP-anchored LSPR biosensors. The precise control over the size, shape, and composition of GNP in nanostructure film plays a key role in engineering the LSPR properties that affect the biosensor response. Considerable effort has gone into synthesis/fabrication techniques to construct gold nanostructures with improved uniformity, spatial distribution, and stability. Recently developed top-down approaches have provided superior control over the uniformity and reproducibility of sensor surfaces. These nanostructured films have exhibited improvement in sensor quality parameters, such as sensitivity, reusability, reproducibility, and dynamic range. The LSPR property of anchored gold nanoparticles has been coupled with a variety of sensor substrates including glass chips, optical fibers, microfluidic channels, and even biological substrates (eg, egg shell membrane, CDMS). These sensors have harnessed the optical properties of GNP and have spawned a number of useful applications for medical diagnostics and environmental monitoring. Further, these nanostructured films have been used in multiplexed mode for simultaneous detection of multiple analytes in a given medium. These successful applications have set up a strong platform to develop versatile and portable LSPR-based biosensing devices.
Given the extensive research that has been undertaken in developing LSPR-based sensors in the past few decades, it is likely that future research in this domain will primarily concentrate on patterning nanoparticles and creation of various nanocomposites, which will allow novel sensor configurations, thereby enhancing sensitivity and/or usability. Research is underway for developing multisensor polymer chips that may be useful to detect multiple analytes at the same time by immobilizing different types of GNP on the same chip but at different sensing locations.Citation115 Printing and deposition of nanoparticles or nanoparticle-loaded films using inexpensive printers like modified deskjet printers or soft lithography-based stamping have an important role to play in obtaining highly ordered nanostructured films. Such films may be magnetoplasmonic arrays (based on, eg, gold and iron nanoparticles), which will be used for their functional advantages. Composite nanoparticles with configurations other than core-shell structures, eg, dumbbells, may play an important role by modulating the LSPR phenomenon around the fundamental gold nanoparticle.
Overall, LSPR properties of GNP have opened up new vistas in developing sensitive and cost effective sensing devices. In the current scenario, commercialization of these laboratory-based LSPR biosensing devices is still in its infancy. Cooperation among physicists, chemists, material scientists, and biomedical engineers may be valuable in delivering these devices to the consumer’s hand.
Disclosure
The authors report no conflicts of interest in this work.
References
- HomolaJPresent and future of surface plasmon resonance biosensorsAnal Bioanal Chem200337752853912879189
- HomolaJSurface plasmon resonance sensors for detection of chemical and biological speciesChem Rev200810846249318229953
- HomolaJYeeSSGauglitzGSurface plasmon resonance sensors: reviewSens Actuators B Chem199954315
- FaradayMExperimental relations of gold (and other metals) to light [abstract]Philos Trans R Soc Lond1857147145181
- MieGBeitrgeäzur Optik trüber medien, speziell kolloidaler metallösungenAnn Phys190825377445
- DraineBTFlatauPJDiscrete-dipole approximation for scattering calculationsJ Opt Soc Am A19941114911499
- FutamataMMaruyamaYIshikawaMLocal electric field and scattering cross section of Ag nanoparticles under surface plasmon resonance by finite difference time domain methodJ Phys Chem B200310776077617
- WilletsKAvan DuyneRPLocalized surface plasmon resonance spectroscopy and sensingAnnu Rev Phys Chem20075826729717067281
- UnderwoodSMulvaneyPEffect of the solution refractive index on the color of gold colloidsLangmuir19941034273430
- HaesAJvan DuyneRPA unified view of propagating and localized surface plasmon resonance biosensorsAnal Bioanal Chem200437992093015338088
- FreemanRGGrabarKCAllisonKJSelf-assembled metal colloid monolayers: an approach to SERS substratesScience19952671629163217808180
- GrubishaDSLipertRJParkHYDriskellJPorterMDFemtomolar detection of prostate-specific antigen: an immunoassay based on surface-enhanced Raman scattering and immunogold labelsAnal Chem2003755936594314588035
- KühnSHåkansonURogobeteLSandoghdarVEnhancement of single-molecule fluorescence using a gold nanoparticle as an optical nanoantennaPhys Rev Lett20069701740216907406
- LyonLAMusickMDNatanMJColloidal Au-enhanced surface plasmon resonance immunosensingAnal Chem199870517751839868916
- HeLMusickMDNicewarnerSRColloidal Au-enhanced surface plasmon resonance for ultrasensitive detection of DNA hybridizationJ Am Chem Soc200012290719077
- MirkinCALetsingerRLMucicRCStorhoffJJA -DNA-based method for rationally assembling nanoparticles into macroscopic materialsNature19963826076098757129
- NamJMThaxtonCSMirkinCANanoparticle based bio-bar codes for the ultrasensitive detection of proteinsScience20033011884188614512622
- ElghanianRStorhoffJJMucicRCLetsingerRLMirkinCASelective colorimetric detection of polynucleotides based on the distance-dependent optical properties of gold nanoparticlesScience1997277107810819262471
- DanielMCAstrucDGold nanoparticles: assembly, supramolecular chemistry, quantum size related properties, and applications toward biology, catalysis, and nanotechnologyChem Rev200410429334614719978
- HaesAJStuartDANieSvan DuyneRPUsing solution-phase nanoparticles, surface-confined nanoparticle arrays and single nanoparticles as biological sensing platformsJ Fluoresc20041435536715617378
- NathNChilkotiALabel free colorimetric biosensing using nanoparticlesJ Fluoresc20041437738915617380
- RoyDFendlerJRefection and absorption techniques for optical characterization of chemically assembled nanomaterialsAdv Mater200416479508
- HutterEFendlerJHExploitation of localized surface plasmon resonanceAdv Mater20041616851706
- GhoshSKPalTInterparticle coupling effect on the surface plasmon resonance of gold nanoparticles: from theory to applicationsChem Rev20071074797486217999554
- PingarrónJMYáñez-SedeñoPGonzález-CortésAGold nanoparticle-based electrochemical biosensorsElectrochim Acta20085358485866
- TurkevichJStevensonPCHillerJA study of the nucleation and growth processes in the synthesis of colloidal goldDiscuss Faraday Soc1951115575
- GrabarKCFreemanRGHommerMBNatanMJPreparation and characterization monolayersAnal Chem199567735743
- BrustMWalkerMBethellDSchiffrinDJWhymanRSynthesis of thiol-derivatised gold nanoparticles in a two-phase liquid–liquid systemJ Chem Soc Chem Commun19947801802
- HostetlerMJWingateJEZhongCJAlkanethiolate gold cluster molecules with core diameters from 1.5 to 5.2 nm: core and monolayer properties as a function of core sizeLangmuir1998141730
- JinYKangXSongYZhangBChengGDongSControlled nucleation and growth of surface-confined gold nanoparticles on a (3-aminopropyl)trimethoxysilane-modified glass slide: a strategy for SPR substratesAnal Chem2001732843284911467525
- FrederixFFriedtJMChoiKHBiosensing based on light absorption of nanoscaled gold and silver particlesAnal Chem2003756894690014670050
- LinTJHuangKTLiuCYDetermination of organophosphorous pesticides by a novel biosensor based on localized surface plasmon resonanceBiosens Bioelectron20062251351816769211
- AslamMFuLSuMVijayamohananKDravidVPNovel one-step synthesis of amine-stabilized aqueous colloidal gold nanoparticlesJ Mater Chem20041417951797
- SugunanAThanachayanontCDuttaJHilbornJGHeavy-metal ion sensors using chitosan-capped gold nanoparticlesSci Technol Adv Mater20056335340
- HuangKWYuCJTsengWLSensitivity enhancement in the colorimetric detection of lead(II) ion using gallic acid-capped gold nanoparticles: improving size distribution and minimizing interparticle repulsionBiosens Bioelectron20102598498919782557
- AnhNTPhuDVDuyNNDuBDHienNQSynthesis of alginate stabilized gold nanoparticles by γ-irradiation with controllable size using different Au3+concentration and seed particles enlargementRadiat Phys Chem201079405408
- JanaNRGearheartLMurphyCJWet chemical synthesis of high aspect ratio cylindrical gold nanorodsJ Phys Chem B200110540654067
- NikoobakhtBEl-SayedMAPreparation and growth mechanism of gold nanorods (NRs) using seed-mediated growth methodChem Mater20031519571962
- SauTKMurphyCJRoom temperature, high-yield synthesis of multiple shapes of gold nanoparticles in aqueous solutionJ Am Chem Soc20041268648864915250706
- SunYXiaYIncreased sensitivity of surface plasmon resonance of gold nanoshells compared to that of gold solid colloids in response to environmental changesAnal Chem2002745297530512403584
- LiuMGuyot-SionnestPMechanism of silver(I)-assisted growth of gold nanorods and bipyramidsJ Phys Chem B2005109221922220016853888
- LeeSMayerKMHafnerJHImproved localized surface plasmon resonance immunoassay with gold bipyramid substratesAnal Chem2009814450445519415896
- NehlCLLiaoHHafnerJHOptical properties of star-shaped gold nanoparticlesNano Lett2006668368816608264
- Sánchez-IglesiasAPastoriza-SantosIPérez-JusteJRodríguez-GonzálezBGarcía de AbajoFJLiz-MarzánLMSynthesis and optical properties of gold nanodecahedra with size controlAdv Mater20061825292534
- Pastoriza-SantosISánchez-IglesiasAGarcía de AbajoFJLiz-MarzánLMEnvironmental optical sensitivity of gold nanodecahedraAdv Funct Mater20071714431450
- WertsMHVLambertMBourgoinJPBrustMNanometer scale patterning of langmuir-blodgett films of gold nanoparticles by electron beam lithographyNano Lett200224347
- SuKHWeiQHZhangXMockJJSmithDRSchultzSInterparticle coupling effects on plasmon resonances of nanogold particlesNano Lett2003310871090
- CabriniSBarsottiRJCarpentieroACross beam lithography (FIB+EBL) and dip pen nanolithography for nanoparticle conductivity measurementsJ Vac Sci Technol B20052328062810
- BukasovRShumaker-ParryJSHighly tunable infrared extinction properties of gold nanocrescentsNano Lett200771113111817432920
- MalyarchukVHuaFMackNHHigh performance plasmonic crystal sensor formed by soft nanoimprint lithographyOpt Express2005135669567519498567
- KimSJungJMChoiDGJungHTYangSMPatterned arrays of Au rings for localized surface plasmon resonanceLangmuir2006227109711216893197
- HenzieJLeeMHOdomTWMultiscale patterning of plasmonic metamaterialsNat Nanotechnol2007254955418654366
- GrabarKCBrownKRKeatingCDStranickSJTangSLNatanMJNanoscale characterization of gold colloid monolayers: a comparison of four techniquesAnal Chem19976947147721639199
- FreemanRGGrabarKCAllisonKJSelf-assembled metal colloid monolayers: an approach to SERS substratesScience19952671629163217808180
- EnglebiennePUse of colloidal gold surface plasmon resonance peak shift to infer affinity constants from the interactions between protein antigens and antibodies specific for single or multiple epitopesAnalyst1998123159916039830172
- OkamotoTYamaguchiIKobayashiTLocal plasmon sensor with gold colloid monolayers deposited upon glass substratesOpt Lett20002537237418059883
- NathNChilkotiALabel-free biosensing by surface plasmon resonance of nanoparticles on glass: optimization of nanoparticle sizeAnal Chem2004765370537815362894
- RanceGAMarshDHKhlobystovANExtinction coefficient analysis of small alkanethiolate-stabilised gold nanoparticlesChem Phys Lett2008460230236
- MillerMLazaridesAASensitivity of metal nanoparticle surface plasmon resonance to the dielectric environmentJ Phys Chem B2005109215562156516853799
- EustisSEl-SayedMAWhy gold nanoparticles are more precious than pretty gold: noble metal surface plasmon resonance and its enhancement of the radiative and nonradiative properties of nanocrystals of different shapesJ Phys Chem B20061107238724816599493
- JainPKLeeKSEl-SayedIHEl-SayedMACalculated absorption and scattering properties of gold nanoparticles of different size, shape, and composition: applications in biological imaging and biomedicineJ Phys Chem B20061107238724816599493
- MarinakosSMChenSChilkotiAPlasmonic detection of a model analyte in serum by a gold nanorod sensorAnal Chem2007795278528317567106
- ChenCDChengSFChauLKWangCRCSensing capability of the localized surface plasmon resonance of gold nanorodsBiosens Bioelectron20072292693216697633
- TamFMoranCHalasNGeometrical parameters controlling sensitivity of nanoshell plasmon resonances to changes in dielectric environmentJ Phys Chem B20041081729017294
- YamamichiJIidaMOjimaTThe mesoscopic effect on label-free biosensors based on localized surface plasmon resonance of immobilized colloidal goldSens Actuators B Chem2009143349356
- HuangHHeCZengYA novel label-free multi throughput optical biosensor based on localized surface plasmon resonanceBiosens Bioelectron2009242255225919042120
- ChenKJLuCJA vapor sensor array using multiple localized surface plasmon resonance bands in a single UV-vis spectrumTalanta2010811670167520441956
- NagataniNTanakaRYuhiTGold nanoparticle-based novel enhancement method for the development of highly sensitive immunochromatographic test stripsSci Technol Adv Mater20067270275
- ZhaoWAliMMAguirreSDBrookMALiYPaper-based bioassays using gold nanoparticle colorimetric probesAnal Chem2008808431843718847216
- NathNChilkotiAA colorimetric gold nanoparticle sensor to interrogate biomolecular interactions in real time on a surfaceAnal Chem20027450450911838667
- ChengSFChauLKColloidal gold-modified optical fiber for chemical and biochemical sensingAnal Chem200375162112530813
- MorokoshiSOhhoriKMizukamiKKitanoHSensing capabilities of colloidal gold modified with a self-assembled monolayer of a glucose-carrying polymer chain on a glass substrateLangmuir2004208897890215379524
- EndoTYamamuraSNagataniNMoritaYTakamuraYTamiyaELocalized surface plasmon resonance based optical biosensor using surface modified nanoparticle layer for label-free monitoring of antigen– antibody reactionSci Technol Adv Mater20056491500
- KitanoHAnrakuYShinoharaHSensing capabilities of colloidal gold monolayer modified with a phenylboronic acid-carrying polymer brushBiomacromolecules200671065107116602722
- GuoCBoullangerPJiangLLiuTHighly sensitive gold nanoparticles biosensor chips modified with a self-assembled bilayer for detection of Con ABiosens Bioelectron2007221830183417045470
- HiepHMEndoTKermanKA localized surface plasmon resonance based immunosensor for the detection of casein in milkSci Technol Adv Mater20078331338
- EndoTYamamuraSKermanKTamiyaELabel-free cell-based assay using localized surface plasmon resonance biosensorAnal Chim Acta200861418218918420049
- KimDKKermanKHiepHMLabel-free optical detection of aptamer–protein interactions using gold-capped oxide nanostructuresAnal Biochem20083791718485275
- MayerKMLeeSLiaoHA label-free immunoassay based upon localized surface plasmon resonance of gold nanorodsACS Nano2008268769219206599
- WangYQianWTanYDingSA label-free biosensor based on gold nanoshell monolayers for monitoring biomolecular interactions in diluted whole bloodBiosens Bioelectron2008231166117018078744
- KajiuraMNakanishiTIidaHTakadaHOsakaTBiosensing by optical waveguide spectroscopy based on localized surface plasmon resonance of gold nanoparticles used as a probe or as a labelJ Colloid Interface Sci200933514014519395015
- LiXJiangLZhanQQianJHeSLocalized surface plasmon resonance (LSPR) of polyelectrolyte-functionalized gold-nanoparticles for bio-sensingColloids Surf A Physicochem Eng Asp2009332172179
- HuangCBonroyKReekmanGVerstrekenKLagaeLBorghsGAn on-chip localized surface plasmon resonance-based biosensor for label-free monitoring of antigen–antibody reactionMicroelectronic Eng20098624372441
- HuangCBonroyKReekmansGLocalized surface plasmon resonance biosensor integrated with microfluidic chipBiomed Microdevices20091189390119353272
- SaiVVRKunduTMukherjiSNovel U-bent fiber optic probe for localized surface plasmon resonance based biosensorBiosens Bioelectron2009242804280919285853
- BiNSunYTianYAnalysis of immunoreaction with localized surface plasmon resonance biosensorSpectrochim Acta A Mol Biomol Spectrosc2010751163116720079682
- HiepHMYoshikawaHTamiyaEInterference localized surface plasmon resonance nanosensor tailored for the detection of specific biomolecular interactionsAnal Chem2010821221122720073504
- ShaoYXuSZhengXWangYXuWOptical fiber LSPR biosensor prepared by gold nanoparticle assembly on polyelectrolyte multilayerSensors2010103585359622319313
- NuszGJCurryACMarinakosSMWaxAChilkotiARational selection of gold nanorod geometry for label-free plasmonic biosensorsACS Nano2009379580619296619
- NuszGJMarinakosSMCurryACLabel-free plasmonic detection of biomolecular binding by a single gold nanorodsAnal Chem20088098498918197636
- HuangHTangCZengYLabel-free optical biosensor based on localized surface plasmon resonance of immobilized gold nanorodsColloids Surf B Biointerfaces2009719610119211228
- LiaoHHafnerJHGold nanorod bioconjugatesChem Mater20051746364641
- EndoTKermanKNagataniNMultiple label-free detection of antigen–antibody reaction using localized surface plasmon resonance-based core-shell structured nanoparticle layer nanochipAnal Chem2006786465647516970322
- KimDKKermanKSaitoMLabel-free DNA biosensor based on localized surface plasmon resonance coupled with interferometryAnal Chem2007791855186417261024
- YeJBonroyKNelisDEnhanced localized surface plasmon resonance sensing on three-dimensional gold nanoparticles assembliesColloids Surf A Physicochem Eng Asp2008321313317
- GuoLChenGKimDHThree-dimensionally assembled gold nanostructures for plasmonic biosensorsAnal Chem2010825147515320469841
- MitsuiKHandaYKajikawaKOptical fiber affinity biosensor based on localized surface plasmon resonanceAppl Phys Lett20048542314233
- LeeJYLeeCWLinEHWeiPKSingle live cell refractometer using nanoparticle coated fiber tipAppl Phys Lett200893173110
- EndoTKermanKNagataniNTakamuraYTamiyaELabel-free detection of peptide nucleic acid-DNA hybridization using localized surface plasmon resonance based optical biosensorAnal Chem2005776976698416255598
- CaoZGongFCTuMPreliminary recognition of c-myc gene protein using an optical biosensor with gold colloid nanoparticles based on localized surface plasmon resonanceAnal Lett20094228202837
- EndoTIkedaDKawakamiYYanagidaYHatsuzawaTFabrication of core-shell structured nanoparticle layer substrate for excitation of localized surface plasmon resonance and its optical response for DNA in aqueous conditionsAnal Chim Acta201066120020520113736
- YooSYKimDKParkTJKimEKTamiyaELeeSYDetection of the most common corneal dystrophies caused by BIGH3 gene point mutations using a multispot gold-capped nanoparticle array chipAnal Chem2010821349135720092310
- LinTJChungMFUsing monoclonal antibody to determine lead ions with a localized surface plasmon resonance fiber-optic biosensorSensors20088582593
- LinTJChungMFDetection of cadmium by a fiber-optic biosensor based on localized surface plasmon resonanceBiosens Bioelectron2009241213121818718753
- ChauLKLinYFChengSFLinTJFiber-optic chemical and biochemical probes based on localized surface plasmon resonanceSens Actuators B Chem2006113100105
- ZhuSDuaCFuYLocalized surface plasmon resonance-based hybrid Au–Ag nanoparticles for detection of Staphylococcus aureus enterotoxin BOpt Mater20093116081613
- XuGLiHMaXJiaXDongJQianWA cuttlebone-derived matrix substrate for hydrogen peroxide/glucose detectionBiosens Bioelectron20092536236719692225
- KreuzerMPQuidantRSalvadorJPMarcoMPBadenesGColloidal-based localized surface plasmon resonance (LSPR) biosensor for the quantitative determination of stanozololAnal Bioanal Chem20083911813182018373230
- ZhengBQianLYuanHPreparation of gold nanoparticles on eggshell membrane and their biosensing applicationTalanta20108217718320685454
- FuJParkBZhaoYLimitation of a localized surface plasmon resonance sensor for Salmonella detectionSens Actuators B Chem2009141276283
- KabashinAVEvansPPastkovskySPlasmonic nanorod metamaterials for biosensingNat Mater2009886787119820701
- DmitrievAPakizehTKällMSutherlandDSGold–silica–gold nanosandwiches: tunable bimodal plasmonic resonatorsSmall2007329429917199248
- González-DíazJBGarcía-MartínAGarcía-MartínJMPlasmonic Au/Co/Au nanosandwiches with enhanced magneto-optical activitySmall2008420220518196506
- WangYShenYXieALiSWangXCaiYA simple method to construct bifunctional Fe3O4/Au hybrid nanostructures and tune their optical properties in the near-infrared regionJ Phys Chem C201011442974301
- PrabhakarAMukherjiSMicrofabricated polymer chip with integrated U-bend waveguides for evanescent field absorption based detectionLab Chip20101074875420221563