Abstract
Purpose
Motivation is an important driver of behaviour, and several frameworks distinguish the willingness of individuals to invest cognitive versus physical effort to achieve a goal. One outstanding question is whether sleep loss lowers motivation within specific domains of effort, or has a global effect on motivation across multiple domains. Here, we investigated the effects of sleep restriction on the motivation to invest cognitive or physical effort in return for reward.
Materials and Methods
24 healthy young adults (11 females) completed an effort-based decision-making task over two laboratory sessions – once while sleep restricted (three consecutive nights with a three-hour sleep opportunity), and the other while fully rested (nine-hour sleep opportunity on each night). In an initial reinforcement phase, participants were trained to ceiling performance across six levels of effort on separate cognitively and physically demanding tasks. Then, in the critical decision-making phase, participants revealed their preference for how much cognitive or physical effort they would be willing to invest for reward.
Results
Sleep restriction reduced the willingness to exert cognitive effort, but spared motivation in the physical domain. Furthermore, the reduction in cognitive motivation appeared to be a primary motivational deficit, which could not be attributed to differences in reward-likelihood of different levels of effort or the temporal structure of the task.
Conclusion
The results suggest that sleep restriction has a selective effect on cognitive over physical motivation, which has significant implications for real-world settings in which individuals must maintain high levels of cognitive motivation in the face of chronic sleep loss.
Introduction
Motivation is fundamental to daily life, and drives us to engage in effortful behaviour in pursuit of our goals – such as the cognitive effort invested by students and academics, and the physical effort invested by athletes and military personnel.Citation1–4 Chronic sleep loss can have a profound impact on daily function, with particular consequences for professions in which sleep loss is unavoidable (eg, healthcare, the military).Citation5,Citation6 It has long been recognised that the effect of sleep loss on performance may be driven, not only by its effects on cognitive and physical capacity,Citation7–9 but also through its detrimental effects on motivational drive.Citation10–15 Importantly, however, motivation is not a unitary construct, and several frameworks distinguish the motivation of individuals to invest effort in the cognitive vs the physical domains.Citation16–18 An important question, therefore, is whether the effects of sleep loss are unique to specific domains of behaviour or generalise across them. However, this topical issue remains to be directly addressed.
The majority of studies that have examined the consequences of sleep loss on motivation have focused on its impact in the cognitive domain.Citation10–12,Citation19,Citation20 Some sleep studies have simply asked the participants to report their willingness to engage in generally cognitively effortful behaviour, eg using a visual analog scale.Citation20,Citation21 However, a more sensitive approach developed in the neuroeconomic literature has been to quantify motivation as the willingness of individuals to overcome different levels of effort costs in pursuit of rewards.Citation17,Citation22–27 Such an approach allows us to determine an individual’s aversion to effort by fitting their choices to an “effort discounting” function.Citation22,Citation28–30 In the sleep literature, such effort discounting studies have tended to show that sleep loss seems to reduce the desire of individuals to engage in cognitively effortful behaviour.Citation10,Citation11,Citation19
However, an important unanswered question is the extent to which this reduction in cognitive motivation represents a primary motivational deficit, versus a secondary consequence of sleep loss on other cognitive functions. For example, previous studies have typically found that sleep loss hinders the capacity to complete cognitive tasks, and accentuates time-on-task decrements in task performance.Citation9,Citation11,Citation19,Citation31 Furthermore, many previous studies have manipulated effort by systematically varying the amount of time that individuals engage with each level of a task (eg, having to perform the psychomotor vigilance task for increasing periods of time).Citation10,Citation11,Citation19 Given that sleep loss hinders the capacity to sustain cognitive performance, previous findings showing reduced willingness to engage in cognitive tasks under sleep restriction, could be, in part, explained by the desire to avoid task failure. Consequently, it is important to confirm that any apparent reduction in cognitive motivation is indeed driven by a greater aversion to effort itself, rather than simply a disinclination to engage in activities that are less likely to be successfully completed.Citation24
Surprisingly, much less work has examined the link between sleep and physical motivation.Citation21,Citation32 These limited data suggest that sleep loss similarly reduces the motivation to invest physical effort. However, these studies are limited in having focused on targeted populations of athletes, in less controlled environments (relying solely on measures of self-reported sleep quality and/or actigraphy), and using less sensitive measures of motivation (eg, a single visual analogue rating score of willingness to perform an effortful task). The impact of sleep loss on physical motivation is therefore unclear. Furthermore, no study has examined the consequence of sleep loss on both cognitive and physical motivation using comparable tasks in the same individuals.
In this study, we examined the effects of sleep restriction on the motivation to invest cognitive vs physical effort. We tested participants in a controlled laboratory setting over two counterbalanced sessions – once while fully rested, and once while sleep restricted – and required them to make decisions on the amount of cognitive or physical effort that they were willing to exert in return for reward.Citation33,Citation34 Importantly, our task was designed to control for potential effects of restricted sleep on variables that might influence participants’ choices, including the capacity of individuals to perform each level of effort, and temporal factors.
Material and Methods
Participants
Data were collected as part of a larger study investigating the effects of sleep restriction on cognition. The final sample comprised 24 healthy adults (see ), with no history of substance use, neurological or psychiatric disease. Participants were non-smokers, consumed less than 400mg of caffeine per day and reported habitual sleep of between 7 and 9 hours with a bedtime between 22:00 and 1:00 and wake times between 6:00 and 9:00. Additionally, participants had no history of night shift work within the past 12 months. Female participants taking hormonal contraceptives were included in the study. Extreme morning and evening types were excluded from the study based on criteria from the Morning and Eveningness Questionnaire.Citation35 This final sample did not include data from two additional participants, from whom data were partially lost due to technical issues. Including the available data from these participants did not alter the main findings. Data was collected between March 2018 and March 2021. Participants were recruited via online advertisements and compensated ~ AUD 2500.
Table 1 Participant Characteristics
Procedure
Participants completed two testing sessions in a closed laboratory environment. Each participant was admitted to a private, time-isolated, light, and temperature-controlled room. Light was maintained at 100 lux during wake periods and temperature maintained at 21° (± 2°C). Each session lasted four nights (from a Sunday to a Thursday evening), and the sessions were separated by a nine-day interval. For one week prior to each session, participants maintained a regular sleep-wake schedule, based on their self-reported habitual sleep schedule, and verified with actigraphy and sleep diaries.
In one session (“Fully Rested”), participants were provided a nine-hour sleep opportunity each night. Timing was based on the at-home schedule, with time-in-bed increased equally at the start and end of the night, for those whose at-home schedule included <9 hours in bed. In the alternate session (“Sleep Restricted”), participants were provided a nine-hour sleep opportunity on their first night, but only a three-hour sleep opportunity on subsequent nights, commencing two hours after their “Fully Rested” bed time. Session order was counterbalanced across participants. The first night of the first session served as a sleep disorders screen. On the last day of each session, participants completed the experimental task four to six hours after habitual wake time, typically between 10 am and 2 pm (42 of 46 participants commenced the task between 10.57 am and 12.53 pm). Any given participant was tested at the same time of day in both testing sessions.
During the in-lab component participants were able to watch movies, play board games, and use a lab provided computer to work. Participants were not permitted to engage in any physical activity more vigorous than walking and there was no access to the internet. Activity was monitored by research staff and actigraphy. Meals and snacks with equivalent macronutrients were provided to participants at regular intervals. Participants were constantly monitored by research staff to ensure adherence to the protocol.
Effort-Based Decision-Making Task
We applied a cognitive and physical effort discounting paradigm, details of which have been previously reported.Citation33,Citation34 The paradigm consists of two phases. First, participants engaged in a Reinforcement Phase, in which they were trained on: (1) a cognitively effortful task, and (2) a physically effortful task, across separate, counterbalanced blocks. In the subsequent Choice Phase, we examined the willingness of individuals to exert cognitive or physical effort in return for reward. In both tasks, the stimuli were displayed on a 17-inch laptop monitor at a refresh rate of 60Hz positioned ~45cm from the participant. The cognitive effort task was implemented in Presentation software,Citation36 and the physical effort task in the Psychtoolbox package run in MATLAB.Citation37 Participants’ responses were registered with a standard computer keyboard for the cognitive task, and a hand-held dynamometer for the physical task (SS25LA, BIOPAC systems, USA).
Reinforcement Phase
The Reinforcement Phase for each task followed the same structure (). Each trial began with an effort cue (in the form of a pie chart presented for two seconds) indicating the amount of effort to be invested in that trial. Blue pie charts preceded cognitively effortful trials, and red pie charts physically effortful trials. These cues were followed by a period of effort exertion in the corresponding domain lasting 10 seconds, followed by a feedback display lasting two seconds indicating their success on that trial. For each task, participants completed three repetitions of each effort level delivered in a random order.
Figure 1 Experimental task. (A and B) Cognitive effort task required participants to monitor 1–6 streams of letters rapidly presented on the screen. (C and D) Physical effort task required sustaining target handgrip force (4–44% MVC). In the training phase, participants completed different effort levels presented in random order, in cognitive and physical domain (counterbalanced order). (E) In the choice phase, participants indicated their preferences between the fixed low-effort/low-reward baseline on the left and a variable high-effort/high-reward offer on the right.
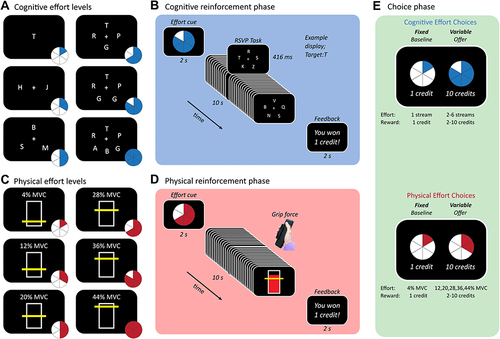
Cognitive Effort Task
The cognitive effort task required participants to monitor between one and six rapid-serial-visual-presentation (RSVP) letter streams for a target letter “T” ()Citation33,Citation34 The lowest effort level required participants to monitor one stream displayed at the centre of the screen. Higher effort levels required participants to simultaneously monitor between two to six streams, positioned equidistantly and equiangularly from fixation. Letter stimuli were displayed in Ariel 26-point font, and target letters could appear randomly in any stream. Each trial contained 24 frames, each of which lasted 416 ms, for a total trial duration of 10 seconds. The target was presented three times in each trial. A button-press response qualified as a hit if it occurred within 833ms of target presentation. Participants were rewarded with one credit if they were able to complete each trial above a threshold level of performance (>1 hit, <3 false alarms).
Physical Effort Task
The physical effort task required participants to exert one of six levels of force on a hand-held dynamometer using their dominant hand (). At the beginning of each session, we determined individuals’ maximum voluntary contraction (MVC) as the maximum of three consecutive ballistic squeezes with their dominant hand. We then defined the six effort levels for each individual as a function of their MVC (4, 12, 20, 28, 36, 44% MVC). On each trial, the target effort level was depicted by a yellow horizontal line on a vertical bar, which provided participants with real-time feedback on their generated force. To succeed, participants had to maintain their force above the target effort level for ≥50% of the 10-second duration of the trial.
Choice Phase
In the critical Choice Phase, we quantified participants’ motivation to exert cognitive or physical effort for a given reward. In each trial, participants chose between a fixed low-effort/low-reward baseline and a variable high-effort/high-reward offer within the same domain. The fixed baseline was the opportunity to perform the lowest level of effort (Level 1) in return for the lowest reward (1 credit). The variable offer was the opportunity to perform higher levels of effort (levels 2–6) for higher rewards (2, 4, 6, 8, 10 credits). The entire Effort x Reward space (5 x 5) was sampled three times in each of the two domains, for a total of 150 trials. Trials were self-paced, participants on average took 5–10 minutes to complete this phase. In keeping with previous studies, we reduced the impact of fatigue accumulation on decision-making by requiring participants to simply indicate their preferred option without executing their choices. In addition, they were explicitly told they should select the option that was most preferable to them, but that the points themselves did not alter remuneration. This protocol is consistent with previous studies and has been shown to be sensitive to changes in motivation.Citation33,Citation34,Citation38–40
Statistical Analysis
The outcomes of interest were participants’ performance in the Reinforcement Phase, and their decisions to engage in effortful behaviour in the Choice Phase. In the Reinforcement Phase, we examined the effect of sleep restriction on performance with within-subjects repeated-measures ANOVAs on the factors of Sleep Condition (fully rested vs sleep restricted) and Effort Level (1–6). We quantified performance in the cognitive effort task as a function of d′ (ZHit Rate – ZFalse Alarm Rate).Citation41 We used a log-linear correction for extreme values of hits or false alarms.Citation42 Performance in the physical effort task was measured as the proportion of time that participants were able to meet or exceed the target effort level on each trial.
In the Choice Phase, we examined the effect of sleep restriction on the proportion of trials that individuals chose the more effortful offer relative to baseline. This was accomplished with a four-way repeated-measures ANOVA on the factors of Sleep Condition (fully rested, sleep restricted), Task Domain (cognitive, physical), and the Effort (2–6) and Reward (2–10) levels associated with the more effortful option. We applied Greenhouse–Geisser corrections for violations of sphericity. All significant main effects or interactions were decomposed with pairwise comparisons corrected for multiple comparisons with a Bonferroni adjustment. We calculated effect sizes using ηp2 (partial eta-squared), a commonly used measure of effect size.Citation43 ηp2 shows the amount of total variance in the dependent variable that is explained by an independent variable while the effects of other variables and interactions are partialled out.
Further, we conducted a control analysis to examine whether participants’ choices were influenced by their capacity to perform the corresponding effort level. For each participant in each session, we used a logistic regression to predict choices as a function of Cognitive Effort, Reward, and the d` for that effort level in the Reinforcement Phase. We then compared the standardised regression coefficients for each factor to zero using a non-parametric Wilcoxon signed-rank test.
Data were analysed using MATLAB Version 2018bCitation37 and JASP Version 0.11.1.Citation44
Results
Reinforcement Phase: The Effects of Sleep Restriction on Performance
First, we examined data from the Reinforcement Phase to probe for differences in performance between the fully rested and sleep restricted conditions.
Cognitive Effort Task
The Sleep Condition x Effort ANOVA revealed a significant main effect of Effort, such that increasing effort was associated with a decrement in d` (F(3.8, 86.3) = 6.42, p < 0.001, ηp2 = 0.22). However, this was qualified by a significant Sleep x Effort interaction (Sleep x Effort, F(5, 115) = 3.25, p = 0.009, ηp2 = 0.12; Sleep, F(1, 23) = 0.13, p = 0.719, ηp2 = 0.01). Decomposing this interaction indicated a lower d` when sleep restricted vs fully rested at effort level 5, but the reverse pattern at level 6 (). Regardless, in both sessions, reinforcement rates were at ceiling, indicating that every participant was able to complete every level of effort to be rewarded on every trial ().
Figure 2 Performance in the cognitive and physical effort tasks during the Reinforcement phase. Fully rested condition is shown in black and sleep restricted condition is shown in blue (cognitive task) or red (physical task). (A) Performance in the cognitive task, quantified as d`, declined as a function of effort but was not consistently impaired under sleep restriction. (B) The reinforcement rates in the cognitive task were at ceiling level across both testing sessions. (C) Performance in the physical task, quantified as the time spent maintaining force above the target effort level, decreased as a function of effort. However, this decrease was unrelated to the sleep manipulation. (D) Finally, there was a slight decrement in the reinforcement rates in physical task at the highest effort levels, but this effect was similar across both sleep conditions.
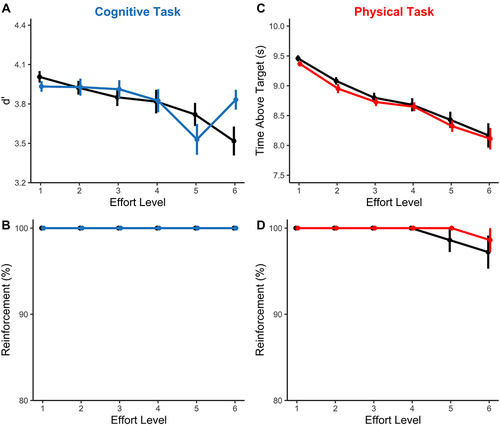
Physical Effort Task
A repeated measures ANOVA on Sleep Condition and Effort (1–6) revealed a significant main effect of Effort, such that higher effort levels led to less time being spent above the target force (F(2.2, 51.5) = 55.42, p < 0.001, ηp2 = 0.71) (). Neither the main effect of Sleep nor the Sleep x Effort interaction were significant (Sleep, F(1, 23) = 0.74, p = 0.399, ηp2 = 0.03; Sleep x Effort, F(1.9, 43.9) = 0.10, p = 0.896, ηp2 = 0.00). Similarly, the ANOVA on average reinforcement rates showed a significant main effect of Effort (F(5, 115) = 2.32, p = 0.048, ηp2 = 0.09), with a small decrement in reinforcement rates at the highest effort levels (). Importantly, however, the main effect of Sleep and the Sleep x Effort interaction were not significant (Sleep: F(1, 23) = 1.00, p = 0.328, ηp2 = 0.04; Sleep x Effort F(5, 115) = 0.39, p = 0.855, ηp2 = 0.02).
We also confirmed that there was no statistically significant difference between MVCs in the Fully Rested versus Sleep Restricted condition (t(23) = 0.69, p = 0.50).
In summary, there were no consistent differences in behaviour between the fully rested and sleep restricted conditions during the Reinforcement Phase of the task.
Choice Phase: The Effects of Sleep Restriction on Motivation
To address our principal question of whether sleep restriction differentially affected the willingness to exert cognitive vs physical effort, we examined the proportion of trials in which individuals chose the more effortful offer relative to baseline (). We conducted a four-way ANOVA on the factors of Sleep Condition, Domain, Effort and Reward. The critical result was revealed by a significant three-way interaction between Sleep Condition x Domain x Effort (F(4, 92) = 4.96, p = 0.001, ηp2 = 0.18). Decomposing this interaction revealed that sleep restriction only reduced the motivation to exert effort within the cognitive domain, but not the physical domain. Furthermore, the effect of motivation on the cognitive domain was manifest at all but the lowest level of effort (level 2, p =. 443, levels 3–6, all p-values ≤ 0.040).
Figure 3 The effects of sleep restriction on motivation. Fully rested condition is shown in black and sleep restricted condition is shown in blue (cognitive task) or red (physical task). The percentage of trials in which the high-effort/high-reward offer was chosen are plotted as a function of (A and C) effort, and (B and D) reward, for the (A and B) cognitive effort task, and the (C and D) physical effort task. Offer acceptance rates are plotted as group means, with error bars indicating one standard error. (A) Sleep restriction impaired cognitive motivation, with a more pronounced effect at the highest levels of effort and (B) across all levels of reward. (C) In contrast, sleep restriction did not change the willingness to invest any level of physical effort, (D) at any level of reward.
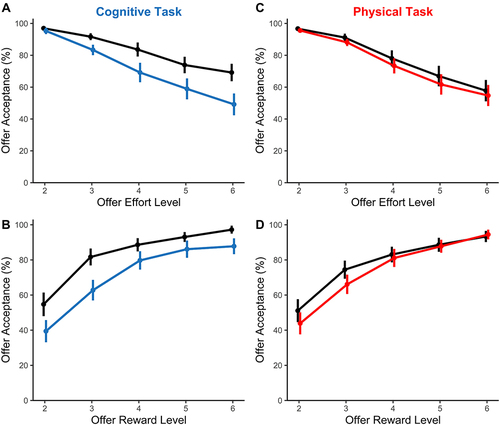
The only other significant set of results was independent of the sleep manipulation. A significant Effort x Reward interaction simply reflected the expected finding that effort discounting was greatest at the lowest levels of reward (when individuals were more sensitive to effort), and minimal at the highest levels of reward (when the rewards outweighed all effort costs) (F(4.3, 98.0) = 11.83, p < 0.001, ηp2 = 0.33). There was a trend towards Sleep Condition modulating this interaction (Sleep x Effort x Reward, F(5.6, 127.7) = 2.15, p = 0.057, ηp2 = 0.09).
Other main effects and interactions were either not significant or captured by the higher-order interactions above (Sleep, F(1, 23) = 3.79, p = 0.064, ηp2 = 0.14; Domain, F(1, 23) = 0.03, p = 0.858, ηp2 = 0.00; Effort, F(4, 92) = 62.30, p < 0.001, ηp2 = 0.73; Reward, (F(4, 92) = 45.47, p < 0.001, ηp2 = 0.66; Sleep x Domain, F(1, 23) = 7.51, p = 0.012, ηp2 = 0.25; Sleep x Effort, F(1.98, 45.6) = 1.93, p = 0.156, ηp2 = 0.08; Sleep x Reward, F(1.9, 43.2) = 2.87, p = 0.071, ηp2 = 0.11; Domain x Effort, F(1.3, 30.8) = 0.34, p = 0.629, ηp2 = 0.01; Sleep x Domain x Reward, F(2.8, 64.4) = 0.58, p = 0.617, ηp2 = 0.03; Sleep x Domain x Effort x Reward (F(6.9, 159.3) = 0.91, p = 0.562, ηp2 = 0.04).
Overall, these data indicate that sleep restriction had a differential effect on the willingness to exert effort in the cognitive domain, and not in the physical domain. In order to compare our results with those of previous effort discounting studies, we also fit participants’ choices to commonly applied computational models of effort discounting. These data reaffirm the differential impact of sleep restriction on changing patterns of cognitive effort discounting, while having no effect on effort discounting in the physical domain. Details of these analyses are presented in the Supplementary Information, Figures S1 and S2.
Control Analyses
The previous findings suggest that individuals had a lower motivation to invest cognitive effort when sleep restricted vs fully rested, which could be interpreted as evidence that sleep restriction leads to a heightened aversion to cognitively effortful behaviour. In principle, an alternate explanation could be that participants were disinclined to engage in greater effort due to a lower likelihood of completing those levels successfully. Importantly, this could not be the case here, as reinforcement rates in the cognitive effort task were at ceiling across all effort levels in both tasks (). Similarly, there was no consistent pattern of difference in performance (d`) in the fully rested vs sleep restricted conditions.
Nevertheless, in a control analysis, we formally verified that cognitive effort choices were not influenced by individuals’ capacity to perform the corresponding effort level. Importantly, performance in the cognitive task, quantified as d` for a given effort level, did not significantly explain individuals’ choices in either sleep condition (fully rested: Z = −1.17, p = 0.241; sleep restricted: Z = 0.14, p = 0.886). As expected, effort and reward both had significant effects on choice (fully rested: Effort, Z = −3.69, p = 0.002; Reward, Z = 3.97, p = 0.001; sleep restricted: Effort, Z = −4.11, p <0.001; Reward, Z = 4.17, p < 0.001).
Discussion
Increasing evidence suggests that the motivation to engage in cognitively and physically effortful behaviour is dissociable.Citation22,Citation28,Citation33 However, the question of whether the effect of sleep loss is limited to particular domains of behaviour has not been addressed. Our main finding was that sleep restriction reduced the willingness of individuals to invest cognitive effort, but spared their willingness to engage in physically effortful behaviour. Importantly, the effects of sleep restriction on cognitive motivation could not have been driven by reduced capacity of individuals to perform each level of the cognitive effort task. This finding of a behavioural dissociation in the effect of sleep restriction on cognitive and physical motivation may have significant implications for those whose professions rely on optimal cognitive performance in spite of chronic sleep loss. In contrast, those occupations relying more heavily on physical effort may not see the same decrement in motivation to perform more physically demanding tasks during sleep loss.
Our findings clarify the interpretation of recent studies which have reported that sleep loss decreases the willingness of individuals to engage in cognitively effortful tasks.Citation10,Citation11,Citation19 In interpreting the effects of sleep loss on cognitive motivation, it is important to distinguish choices based on the aversiveness of effort itself versus the capacity of individuals to adequately perform the corresponding action. More effortful actions are typically associated with a performance decrement, as shown both in our data (), and those of previous studies.Citation11,Citation19 It is therefore important to determine whether the choice to engage in the less effortful option is indeed due to an aversion to higher effort, rather than simply a desire to avoid engaging in options that the individual is less capable of completing. Importantly, participants in our task were able to accomplish all levels of cognitive effort at ceiling performance (). Furthermore, control analyses indicate that performance in the cognitive effort task could not account for the reduction in cognitive motivation caused by sleep restriction.
Relatedly, previous studies have typically been manipulated effort by increasing the time it takes to complete a cognitive task (although seeCitation20). Considering that sleep loss has been found to accentuate the time-on-task decrements in cognitive performance,Citation11,Citation19 reduced willingness to perform longer cognitive tasks under sleep restriction could be due to concerns about being able to sustain the required performance level. An important feature of our cognitive task was that we were able to manipulate attentional load while holding trial duration constant. Our results therefore serve to increase the specificity of prior findings, by showing that the effect of sleep loss on cognitive motivation likely represents a primary motivational deficit, rather than one driven by concerns about task performance.
In contrast to cognitive effort, relatively few studies have examined the question of how sleep loss affects motivation in the physical domain. Those that have suggest that a reduction in sleep quality or quantity lowers the motivation to engage in physically effortful behaviour.Citation21,Citation32 These reports may seem at odds with our results, which showed that sleep restriction had relatively little impact on motivation in the physical domain. However, key methodological differences between the current study and previous literature may explain the inconsistent results. Firstly, these earlier studies all utilised targeted groups of athletes, as opposed to the non-selective group of young adults recruited here. Unsurprisingly, previous work has shown that athletes have a higher motivation to invest physical effort relative to non-athletes.Citation28 Thus, this apparent discrepancy could simply reflect an effect of sleep loss on physical motivation in cohorts that merely had a greater capacity to demonstrate such effects in the first place. Secondly, previous work on physical motivation has relied on subjective reports of sleep quality and quantity, in contrast to the highly controlled manipulation of sleep restriction we implemented here. Thus, it will be important for future work to directly address our speculation that an individual’s baseline level of physical motivation affects their response to physical effort under sleep restricted conditions.
Our findings contribute to accumulating evidence from both behavioural tasks and neurophysiological studies that cognitive and physical motivation are dissociable.Citation22,Citation28,Citation33,Citation45 For example, studies in neurological disease indicate that certain patient groups can have selective impairments that primarily affect a single domain of motivation,Citation33 although other patient groups may demonstrate more global deficits.Citation34,Citation38 Furthermore, recent studies indicate that the neural correlates of motivated behaviour comprise a network of regions, with some areas, such as amygdala, that are sensitive to individual domains of effort,Citation22,Citation46,Citation47 and other regions that are more domain-general, including the medial prefrontal cortex and ventral striatum.Citation18,Citation48–51 Across many of these studies, it is clear that individuals vary widely in their motivation across the cognitive and physical domains. A logical extension of this work is to consider how sleep loss modulates neural activity across those areas involved in motivated behaviour – a question that is beginning to be addressed.Citation11
Limitations
In this study, we operationalised cognitive effort specifically in terms of sustained attention. This is a logical approach, given the sensitivity of attention and arousal to sleep loss.Citation19,Citation31,Citation52 However, cognitive effort can be operationalised in many different ways, and many studies have focused on cognitive tasks requiring divided attention,Citation11,Citation19,Citation31 memory,Citation53 language,Citation10 or arithmetic.Citation20 We predict therefore that our findings should generalise to other cognitive tasks and operational settings that are primarily reliant on sustained attention. However, given the impact of sleep loss varies across cognitive domains,Citation54–57 the extent to which cognitive motivation is diminished in other types of cognitive tasks remains to be empirically demonstrated.
Similarly, grip force is a commonCitation27,Citation58–62 but not the only way to manipulate physical effort in an experimental setting.Citation21,Citation32,Citation63–66 Of note, endurance tasks (eg running, cycling) appear to be more susceptible to the detrimental effects of sleep restriction than brief, strength-dependent tasks (eg weight-lifting).Citation64,Citation66,Citation67 Our task required sustaining grip force for 10 seconds. It remains to be determined to what extent our findings generalise to tasks that require maintaining physical effort for longer periods.
Conclusion
In conclusion, this study demonstrates a selective effect of sleep restriction on reducing cognitive vs physical motivation. Our data provide a compelling demonstration that sleep loss results in a primary deficit in cognitive motivation due to a heightened aversion to effort, while sparing motivation in the physical domain. These findings have significant implications for academic and professional settings that characteristically require maintaining high levels of cognitive motivation in the face of chronic sleep loss – such as in students and medical professionals. The detrimental effects of sleep loss on cognitive and physical performance are well recognised. Our data emphasise the importance of sleep, not only to optimise cognitive performance, but also to maintain the motivation to do so.
Abbreviations
MVC, maximum voluntary contraction; RSVP, rapid-serial-visual-presentation.
Data Sharing Statement
Data that support the findings of this study data are available from the corresponding authors upon reasonable request.
Ethics Approval and Consent to Participate
The study was approved by the Monash University Human Research Ethics Committee (Project ID 8032) and was conducted in accordance with relevant guidelines and regulations. All participants provided written informed consent. The study complied with the Declaration of Helsinki.
Author Contributions
M.J., S.D. and T.C. were involved in study design. M.J. and J.B. collected the data. M.J. and T.C. analysed the data. All authors contributed to writing the manuscript. All authors made a significant contribution to the work reported, whether that is in the conception, study design, execution, acquisition of data, analysis and interpretation, or in all these areas; took part in drafting, revising or critically reviewing the article; gave final approval of the version to be published; have agreed on the journal to which the article has been submitted; and agree to be accountable for all aspects of the work.
Disclosure
Professor Sean PA Drummond reports grants from Office of Naval Research Global, during the conduct of the study; advisory board via Monash University for Avecho and Philia Labs, member of Data and Safety Monitoring Board for Zelda Therapeutics, outside the submitted work. The authors report no other conflicts of interest in this work.
Acknowledgments
We would like to thank Michelle Monica Bravo for her help in collecting data.
Additional information
Funding
References
- Atkinson JW. An Introduction to Motivation. Oxford, England: Van Nostrand; 1964.
- Brehm JW, Self EA. The intensity of motivation. Ann Rev Psychol. 1989;40:109–131. doi:10.1146/annurev.ps.40.020189.000545
- Chong TT-J. Updating the role of dopamine in human motivation and apathy. Curr Opin Behav Sci. 2018;22:35–41. doi:10.1016/j.cobeha.2017.12.010
- Salamone JD, Yohn SE, López-Cruz L, San Miguel N, Correa M. Activational and effort- related aspects of motivation: neural mechanisms and implications for psychopathology. Brain. 2016;139(5):1325–1347. doi:10.1093/brain/aww050
- Capaldi VF, Balkin TJ, Mysliwiec V. Optimizing sleep in the military challenges and opportunities. Chest. 2019;155(1):215–226. doi:10.1016/j.chest.2018.08.1061
- Rosen IM, Gimotty PA, Shea JA, Bellini LM. Evolution of sleep quantity, sleep deprivation, mood disturbances, empathy, and burnout among interns. Acad Med. 2006;81:1. doi:10.1097/00001888-200601000-00020
- Vanhelder T, Radomski MW. Sleep-deprivation and the effect on exercise performance. Sports Med. 1989;7(4):235–247. doi:10.2165/00007256-198907040-00002
- Souissi N, Sesboue B, Gauthier A, Larue J, Davenne D. Effects of one night’s sleep deprivation on anaerobic performance the following day. Eur J Appl Physiol. 2003;89(3–4):359–366. doi:10.1007/s00421-003-0793-7
- Tomasi D, Wang RL, Telang F, et al. Impairment of attentional networks after 1 night of sleep deprivation. Cerebral Cortex. 2009;19(1):233–240. doi:10.1093/cercor/bhn073
- Libedinsky C, Massar SAA, Ling A, Chee W, Huettel SA, Chee MWL. Sleep deprivation alters effort discounting but not delay discounting of monetary rewards. SLEEP. 2013;36(6):899–904. doi:10.5665/sleep.2720
- Massar SAA, Lim J, Sasmita K, Ragunath BL, Chee MWL. Neurobehavioral correlates of how time-on-task and sleep deprivation modulate deployment of cognitive effort. bioRxiv. 2019;2019:865766.
- Sasmita K, Massar SAA, Lim J, Chee MWL. Reward motivation normalises temporal attention after sleep deprivation. J Sleep Res. 2018;28:e12796. doi:10.1111/jsr.12796
- Harrison Y, Horne JA. The impact of sleep deprivation on decision making: a review. J Exp Psychol Appl. 2000;6(3):236–249. doi:10.1037//1076-898x.6.3.236
- Wilkinson RT. Interaction of lack of sleep with knowledge of results, repeated testing, and individual differences. J Exp Psychol. 1961;62(3):263–271. doi:10.1037/h0048787
- Williams HL, Lubin A, Goodnow JJ. Impaired performance with acute sleep loss. Psychol Monogr. 1959;73(14):1–26. doi:10.1037/h0093749
- Husain M, Roiser JP. Neuroscience of apathy and anhedonia: a transdiagnostic approach. Nat Rev Neurosci. 2018;19(8):470–484. doi:10.1038/s41583-018-0029-9
- Westbrook A, Bosch R, Maatta JI, et al. Dopamine promotes cognitive effort by biasing the benefits versus costs of cognitive work. Science. 2020;367(6484):1362–1366. doi:10.1126/science.aaz5891
- Müller T, Apps MAJ. Motivational fatigue: a neurocognitive framework for the impact of effortful exertion on subsequent motivation. Neuropsychologia. 2018;123:141–151. doi:10.1016/j.neuropsychologia.2018.04.030
- Massar SAA, Lim J, Sasmita K, Chee MWL. Sleep deprivation increases the costs of attentional effort: performance, preference and pupil size. Neuropsychologia. 2019;123:169–177. doi:10.1016/j.neuropsychologia.2018.03.032
- Engle-Friedman M, Riela S, Golan R, et al. The effect of sleep loss on next day effort. J Sleep Res. 2003;12(2):113–124. doi:10.1046/j.1365-2869.2003.00351.x
- Ritland BM, Simonelli G, Gentili RJ, et al. Effects of sleep extension on cognitive/motor performance and motivation in military tactical athletes. Sleep Med. 2019;58:48–55. doi:10.1016/j.sleep.2019.03.013
- Chong TT-J, Apps M, Giehl K, Sillence A, Grima LL, Husain M. Neurocomputational mechanisms underlying valuation of effort costs. PLoS Biol. 2017;15(2):e1002598. doi:10.1371/journal.pbio.1002598
- Hartmann MN, Hager OM, Tobler PN, Kaiser S. Parabolic discounting of monetary rewards by physical effort. Behav Processes. 2013;100:192–196. doi:10.1016/j.beproc.2013.09.014
- Chong TT-J, Bonnelle V, Husain M. Quantifying motivation with effort-based decision-making paradigms in health and disease. Prog Brain Res. 2016;229:71–100.
- Salamone JD, Correa M, Farrar A, Mingote S. Effort- related functions of nucleus accumbens dopamine and associated forebrain circuits. Psychopharmacology. 2007;191(3):461–482. doi:10.1007/s00213-006-0668-9
- Jurgelis M, Chong WB, Atkins KJ, Cooper PS, Coxon JP, Chong TTJ. Heightened effort discounting is a common feature of both apathy and fatigue. Sci Rep. 2021;11(1):22283. doi:10.1038/s41598-021-01287-2
- Müller T, Klein-Flügge MC, Manohar SG, Husain M, Apps MAJ. Neural and computational mechanisms of momentary fatigue and persistence in effort-based choice. Nat Commun. 2021;12(1):4593. doi:10.1038/s41467-021-24927-7
- Chong TT-J, Apps MAJ, Giehl K, Hall S, Clifton CH, Husain M. Computational modelling reveals distinct patterns of cognitive and physical motivation in elite athletes. Sci Rep. 2018;8:8. doi:10.1038/s41598-017-18329-3
- Apps MAJ, Grima LL, Manohar S, Husain M. The role of cognitive effort in subjective reward devaluation and risky decision- making. Sci Rep. 2015;5:16880.
- Westbrook A, Braver TS. Cognitive effort: a neuroeconomic approach. Cogn Affect Behav Neurosci. 2015;15(2):395. doi:10.3758/s13415-015-0334-y
- Lim J, Dinges DF. Sleep deprivation and vigilant attention. Ann N Y Acad Sci. 2008;1129(1):305–322. doi:10.1196/annals.1417.002
- Engle-Friedman M, Palencar V, Riela S. Sleep and effort in adolescent athletes. J Child Health Care. 2010;14(2):131–141. doi:10.1177/1367493510362129
- Atkins KJ, Andrews SC, Stout JC, Chong TT-J. Dissociable motivational deficits in pre-manifest huntington’s disease. Cell Rep Med. 2020;1(9):100152. doi:10.1016/j.xcrm.2020.100152
- McGuigan S, Zhou SH, Brosnan MB, Thyagarajan D, Bellgrove MA, Chong TT-J. Dopamine restores cognitive motivation in Parkinson’s disease. Brain. 2019;142:719–732. doi:10.1093/brain/awy341
- Horne JA, Ostberg O. A self-assessment questionnaire to determine morningness-eveningness in human circadian rhythms. Int J Chronobiol. 1976;4(2):97–110.
- Presentation [computer program]. Version 20.0. Neurobehavioral Systems; 2017.
- MATLAB [computer program]. Version 2018b. Natick Massachusetts: MathWorks; 2018.
- Chong TT-J, Bonnelle V, Manohar S, et al. Dopamine enhances willingness to exert effort for reward in Parkinson’s disease. Cortex. 2015;69:40–46. doi:10.1016/j.cortex.2015.04.003
- Frank MJ, Seeberger LC, Reilly RC. By carrot or by stick: cognitive reinforcement learning in Parkinsonism. Science. 2004;306(5703):1940–1943. doi:10.1126/science.1102941
- Chong TT-J, Bonnelle V, Veromann KR, et al. Dissociation of reward and effort sensitivity in methcathinone‐induced Parkinsonism. J Neuropsychol. 2018;12(2):291–297. doi:10.1111/jnp.12122
- Macmillan NA. Detection Theory a User’s Guide. 2nd ed. Lawrence Erlbaum Associates; 2005.
- Hautus MJ. Corrections for extreme proportions and their biasing effects on estimated values of D’. Behav Res Methods Instr Comput. 1995;27(1):46–51. doi:10.3758/BF03203619
- Richardson JTE. Eta squared and partial eta squared as measures of effect size in educational research. Educ Res Rev. 2011;6(2):135–147. doi:10.1016/j.edurev.2010.12.001
- JASP [computer program]. Version 0.11.1. JASP Team; 2019.
- Hosking JG, Floresco SB, Winstanley CA. Dopamine antagonism decreases willingness to expend physical, but not cognitive, effort: a comparison of two rodent cost/benefit decision-making tasks. Neuropsychopharmacology. 2015;40(4):1005–1015. doi:10.1038/npp.2014.285
- Floresco SB, Ghods-Sharifi S. Amygdala-prefrontal cortical circuitry regulates effort-based decision making. Cerebral Cortex. 2007;17(2):251–260. doi:10.1093/cercor/bhj143
- Hosking JG, Cocker PJ, Winstanley CA. Prefrontal cortical inactivations decrease willingness to expend cognitive effort on a rodent cost/ benefit decision- making task. Cerebral Cortex. 2016;26(4):1529–1538. doi:10.1093/cercor/bhu321
- Westbrook A, Frank M. Dopamine and proximity in motivation and cognitive control. Curr Opin Behav Sci. 2018;22:28–34. doi:10.1016/j.cobeha.2017.12.011
- Cools R. The cost of dopamine for dynamic cognitive control. Curr Opin Behav Sci. 2015;4:152–159. doi:10.1016/j.cobeha.2015.05.007
- Schmidt L, Lebreton M, Daunizeau J, Pessiglione M. Neural mechanisms underlying motivation of mental versus physical effort. PLoS Biol. 2012;10(2):e1001266. doi:10.1371/journal.pbio.1001266
- Le Heron C, Apps MAJ, Husain M. The anatomy of apathy: a neurocognitive framework for amotivated behaviour. Neuropsychologia. 2018;118:54–67. doi:10.1016/j.neuropsychologia.2017.07.003
- Lim J, Dinges DF. A meta-analysis of the impact of short-term sleep deprivation on cognitive variables. Psychol Bull. 2010;136(3):375–389. doi:10.1037/a0018883
- Mikulincer M, Babkoff H, Caspy T, Sing H. The effects of 72 hours of sleep loss on psychological variables. Br J Psychol. 1989;80(2):145–162. doi:10.1111/j.2044-8295.1989.tb02309.x
- Boardman JM, Bei B, Mellor A, Anderson C, Sletten TL, Drummond SPA. The ability to self‐monitor cognitive performance during 60 h total sleep deprivation and following 2 nights recovery sleep. J Sleep Res. 2018;27(4):e12633–n/a. doi:10.1111/jsr.12633
- Pasula EY, Brown GG, McKenna BS, et al. Effects of sleep deprivation on component processes of working memory in younger and older adults. Sleep. 2018;41(3):1. doi:10.1093/sleep/zsx213
- Turner TH, Drummond SPA, Salamat JS, Brown GG. Effects of 42 Hr of total sleep deprivation on component processes of verbal working memory. Neuropsychology. 2007;21(6):787–795. doi:10.1037/0894-4105.21.6.787
- Frey DJ, Badia P, Wright KP. Inter‐ and intra‐individual variability in performance near the circadian nadir during sleep deprivation. J Sleep Res. 2004;13(4):305–315. doi:10.1111/j.1365-2869.2004.00429.x
- Le Bouc R, Rigoux L, Schmidt L, et al. Computational dissection of dopamine motor and motivational functions in humans. J Neurosci. 2016;36(25):6623. doi:10.1523/JNEUROSCI.3078-15.2016
- Bonnelle V, Veromann K-R, Burnett Heyes S, Lo Sterzo E, Manohar S, Husain M. Characterization of reward and effort mechanisms in apathy. J Physiol Paris. 2015;109(1–3):16–26. doi:10.1016/j.jphysparis.2014.04.002
- Bonnelle V, Manohar S, Behrens T, Husain M. Individual differences in premotor brain systems underlie behavioral apathy. Cerebral Cortex. 2016;26(2):807–819. doi:10.1093/cercor/bhv247
- Saleh Y, Le Heron C, Petitet P, et al. Apathy in small vessel cerebrovascular disease is associated with deficits in effort-based decision making. Brain. 2021;144(4):1247–1262. doi:10.1093/brain/awab013
- Lockwood PL, Abdurahman A, Gabay A, et al. Ageing increases prosocial motivation for effort. Psychol Sci. 2021;32(5):668–681. doi:10.1177/0956797620975781
- Enoka RM, Baudry S, Rudroff T, Farina D, Klass M, Duchateau J. Unraveling the neurophysiology of muscle fatigue. J Electromyogr Kinesiol. 2011;21(2):208–219. doi:10.1016/j.jelekin.2010.10.006
- Oliver SJ, Costa RJS, Laing SJ, Bilzon JLJ, Walsh NP. One night of sleep deprivation decreases treadmill endurance performance. Eur J Appl Physiol. 2009;107(2):155–161. doi:10.1007/s00421-009-1103-9
- Leiper JB, Junge A, Maughan RJ, Zerguini Y, Dvorak J. Alteration of subjective feelings in football players undertaking their usual training and match schedule during the Ramadan fast. J Sports Sci. 2008;26:55–69. doi:10.1080/02640410802538176
- Fullagar H, Skorski S, Duffield R, Hammes D, Coutts A, Meyer T. Sleep and athletic performance: the effects of sleep loss on exercise performance, and physiological and cognitive responses to exercise. Sports Med. 2015;45(2):161–186. doi:10.1007/s40279-014-0260-0
- Blumert AP, Crum JA, Ernsting SM, et al. The acute effects for twenty-four hours of sleep loss on the performance on national caliber male collegiate weightlifters. J Strength Cond Res. 2007;21(4):1146–1154. doi:10.1519/R-21606.1