Abstract
Immunotherapy provides a new treatment option for cancer. However, it may be therapeutically insufficient if only using the self-immune system alone to attack the tumor without any aiding methods. To overcome this drawback and improve the efficiency of therapy, new treatment methods are emerging. In recent years, ultrasound-mediated microbubble destruction (UMMD) has shown great potential in cancer immunotherapy. Using the combination of ultrasound and targeted microbubbles, molecules such as antigens or genes encoding antigens can be efficiently and specifically delivered into the tumor tissue. This review focuses on the recent progress in the application of UMMD in cancer immunotherapy.
Keywords:
Introduction
The incidence of and mortality due to cancer remain high. Substantial progress has been made using conventional treatments, including surgery, chemotherapy and radiotherapy; however, relapse, metastasis and drug resistance remain the major roadblocks on the path to conquering cancer.Citation1 Therefore, effective strategies to eradicate tumors are urgently needed.Citation2
Immunotherapy has been increasingly drawing clinicians’ and scientists’ attention owing to its effectiveness in cancer treatment.Citation3 Compared with other strategies for cancer therapy, immunotherapy has the unique advantage of high specificity for the tumor cell while leaving normal cells unharmed. Therefore, the adverse reactions caused by drug therapy can be avoided. Moreover, curative effects of cancer immunotherapy are its rapid onset and long duration, which are different from other strategies.
During immunotherapy, the patient’s immune system is activated and enhanced through administration of immune-stimulating substances with long-lasting therapeutic effects. In addition, the anamnestic effects of the host immune system, which account for recurrence prevention, can be induced by immunotherapy.Citation4 Immunotherapy, which characteristically has mild adverse effects and sustainable efficacy, holds great promise for cancer treatment.Citation5 Cancer vaccines, antibodies, cytokines and adoptive cell therapy (ACT) are widely used in cancer immunotherapy. Immune-activating molecules can be packaged into a recombinant protein or provided in the form of genes during drug administration.Citation6 However, the physicochemical properties of these molecules, including their surface charge, hydrophilicity and size, may affect their specificity, eventually weakening their therapeutic effects.Citation7
In recent years, with the development of materialogy, which has brought great benefits to the preparation of ultrasound (US) contrast agents, ultrasound-mediated microbubble destruction (UMMD) has become a versatile technology with great potential in cancer immunotherapy. UMMD is a target-specific, non-invasive, effective and novel gene/drug delivery system, in which the microbubbles serve as a gene/drug vehicle or gene/drug delivery enhancer. UMMD leads to acoustic cavitations and induces the generation of physical forces, such as implosions, shock waves, microstreaming and liquid jets, and these forces can induce the disruption of the cytomembrane and enhance the permeability of the cytomembrane. Therefore, drugs, genes, antibodies and cytokines can be directly delivered into the cytoplasm of immune cells, thus enhancing the immune response.Citation8 Differently from other technologies, UMMD has been shown to increase the delivery of immune-stimulating substances to tumors without causing any severe damage.
Cancer immunotherapy
For cancer patients, immunotherapy is a type of cancer therapy in which the antitumor immune response is activated so that it can act to directly attack tumor cells but leave normal cells unharmed.Citation9 Cancer immunotherapy can be mediated in different ways: via cancer vaccines; through the application of monoclonal antibodies; via delivery of cytokines, such as interferon (IFN) and interleukins (ILs); and by adoptive cell transfer, including natural killer (NK) cells and T-regulatory cells (T cells).Citation10,Citation11
In general, cancer immunotherapy can be divided into two types: active cancer immunotherapy and passive cancer immunotherapy. Active immunotherapy means that the immune response is generated by an antigen, such as a cancer vaccine, while passive immunotherapy refers to specific immune substances, such as antibodies or sensitized lymphocytes, that are reinjected into the host body to obtain specific immunity without any antigen.Citation12 Both therapeutic strategies can be enhanced by UMMD.
Effects of UMMD
UMMD is a promising technique for non-invasive, targeted drug and gene delivery, and its applications for immunomodulatory substance delivery to tumor tissues have attracted increasing interest.Citation13 The movement and destruction of US-mediated microbubbles can trigger enhanced permeability of cytomembranes, open tight junctions (eg, the blood–brain barrier [BBB], blood–tumor barrier and interendothelial junctions) and promote cell endocytosis, thereby providing a transient and invertible channel for the delivery of drugs, genes or gene vehicles and other macromolecules across the endothelial gap and across biological membranes.Citation14–Citation16
Under US irradiation, microbubbles explode and oscillation and destruction are induced.Citation17 Thus, ruptured microbubbles can serve as a tool to induce local energy release using the acoustic radiation force (ARF), microstreaming, shock waves, microjets and strains. These forces have a direct influence on the cell membrane and vascular wall, enhancing the delivery efficiency of a drug/gene into the cytoplasm.Citation18 The common mechanisms of UMMD technology include a cavitation effect, a heat effect, ARF and acoustic droplet vaporization (ADV) ().Citation19
Figure 1 UMMD enhances the microbubble payload delivery into the cell.
Abbreviations: UMMD, ultrasound-mediated microbubble destruction; US, ultrasound.
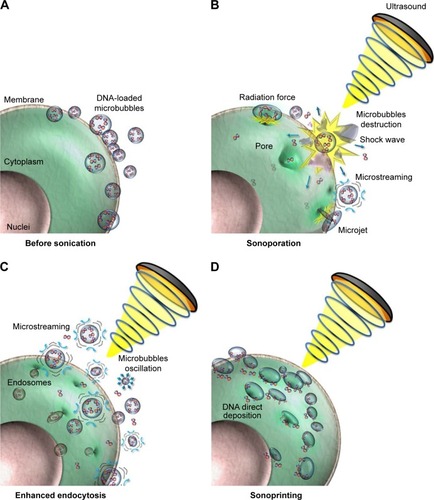
Cavitation effect
The cavitation effect is based on the interaction between microbubbles and US. Under a US field at low power, microbubbles oscillate symmetrically and linearly, implying an opposite tendency of the expansion and compression of a microbubble. The situation is different for higher US intensities. In a high-intensity US field, microbubbles behave non-linearly and expand significantly to a state larger than twice the initial size followed by rupture, causing a high acoustic pressure in a local area, which may be as high as several thousand atmospheres.Citation20 These two phenomena are also known as stable cavitation (or non-inertial cavitation) and inertial cavitation. Stable cavitation refers to the oscillation phenomenon in which a microbubble dilates at its syntonous size, creating a liquid flow called microstreams around the microbubbles. When these oscillating microbubbles reach cells, shear stress is generated, enhancing the permeability of the cell membrane.Citation21,Citation22
It is commonly recognized that inertial cavitation is a crucial mechanism of cavitation that always occurs under a high acoustic field. Inertial cavitation is characterized by sudden expansions and subsequently rapid collapses of gas microbubbles caused by microbeams.Citation23 Microbeams can produce a shear force on the surface of microtubules, causing microbubbles to deform or even rupture.Citation24 The energy induced by microbubble destruction can cause various biological effects, such as temporary holes in the cell membrane, which promote entry of drugs and genes into cells, subsequently enhancing the antitumor immune response of the host body ().
Figure 2 Schematic of enhanced delivery of UMMD.
Abbreviations: UMMD, ultrasound-mediated microbubble destruction; US, ultrasound.
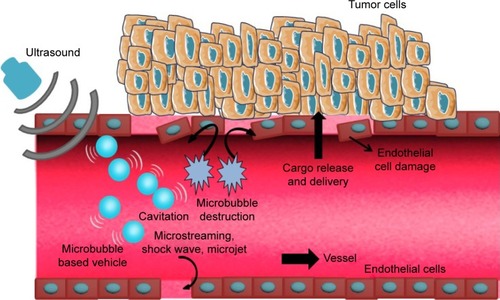
The cavitation of microbubbles can trigger many cell bioeffects, especially the enhancement of cell endocytosis.Citation25 According to a recent study, Ca2+ can immediately excite endocytosis for cytomembrane resealing.Citation26,Citation27 Fan et al found that the intracellular Ca2+ concentration was simultaneously increased with the function of cavitation and onset of sonoporation, and gradually recovered to the normal level within approximately 100 seconds.Citation28 In this way, during the time window when the Ca2+ level was increased, endocytosis was also enhanced by the cavitation and sonoporation. Moreover, several substances (eg, ceramide) were secreted by lysosomes (delivered to the damaged membrane), which can induce endocytosis and rapid formation of endosomes.Citation29
Acoustic radiation force
Oscillating microbubbles located inside the vascular structure in an acoustic field can translate toward the vascular wall through the ARF.Citation30 The microbubbles translate in the direction of wave diffusion in the case of a traveling acoustic wave.
It is not easy to translate freely with the restriction of fluid shear and the floating action in the vasculature. With the help of the pressure gradient induced by ARF, microtubules can move away from the sound source. Hence, microbubbles can be delivered to targeted areas and adhere to the targeted cells.Citation31 According to Rychak et al, ARF can promote a shift and aggregation of microbubbles toward the vascular wall, increasing the adhesion rate of targeted microbubbles 60–80-fold.Citation32
UMMD and ARF have a synergistic effect, which can cause tissue damage and reduces the side effects of intravenous administration while improving microbubble targeting to tissues. ARF impacts the fluidity of lipid bimolecular membranes, resulting in a shear force, broadening the space of endothelial cells, increasing the permeability of capillaries and promoting gene or drug delivery.Citation33
Acoustic droplet vaporization
In general, the continuous microvascular structure and dense normal endothelial gap make it difficult for macromolecules to penetrate the blood vessel wall. By contrast, the leaky blood vessels surrounding tumor tissues show heterogeneous hyperpermeability, which leads to penetration and retention of macromolecule material in the tumor bed, which is known as the enhanced permeation and retention (EPR) effect. During cancer therapy, high delivery of macromolecular drugs and genes based on the EPR effect is strongly dependent on the permeability of the tumor microvasculature.Citation34–Citation36
Owing to the inherent leakiness of the underdeveloped tumor vasculature, the enhanced permeability enables nanoparticles loaded with drugs or genes to escape the circulation and assemble at tumor tissues.Citation37 Therefore, nanodroplets that rely on the EPR effect to target tumors prolong the half-life of drugs and enhance their efficacy, with reduced side effects. EPR-based nanodroplets are widely used in medicine. For example, albumin-bound paclitaxel nanodroplets were approved by the US Food and Drug Administration to treat breast cancer in 2005.Citation38 Furthermore, paclitaxel-loaded nanodroplets were recently used in a clinical trial to treat non-small-cell lung cancer.Citation39 The liquefied gas nanodroplets are much are much smaller than microbubbles and can load much more gas. In an acoustic field of sufficient intensity, these nanodroplets are transformed into gas bubbles that are triggered by acoustic waves without obviously causing heating effects on surrounding normal tissues.Citation40 Such a phase-change phenomenon is called ADV, which was first described in the 1990s and has subsequently been widely used in imaging for preclinical trials.Citation41 Recently, the application of ADV in drug delivery has received extensive attention.Citation42 Since the diameter of these liquid droplets is sufficiently small to traverse the lungs, they can easily pass through the narrow space of tumor tissues.Citation43 Furthermore, transudatory nanodroplets vaporized into gas bubbles can be activated by acoustics, leading to cavitation in the membranes of tumor tissues to enhance drug delivery.Citation44 Thus, efficient delivery of the nanodroplets loaded with mRNA encoding a tumor antigen can induce a significant immunostimulatory effect that is meaningful for antitumor immunotherapy.
Immunotherapy assisted by UMMD
Low-frequency US combined with microbubbles simultaneously promotes dendritic cells (DCs) to differentiate and mature in the cancer microenvironment.Citation45 In addition, this phenomenon promotes T lymphocytes to trigger antitumor immunity mediated by T lymphocytes, which enhance the efficacy of angiogenesis targeting.Citation46 UMMD has been widely applied as a tool to enhance the delivery of immunomodulatory materials, such as cancer vaccines, antibodies, adoptive cells and cytokines, resulting in enhancement of the immunotherapy effect. Relevant reports of UMMD-triggered immune effects are listed in .
Table 1 Summary of experiments and immune responses after treatment with UMMD
Cancer vaccination
Cancer vaccination is attracting increasing attention as a promising therapy strategy for the prevention and treatment of tumor growth, as well as metastasis. Immunotherapy by cancer vaccination has gradually become the mainstream strategy in recent years. Cancer vaccines can be divided into the following types: tumor antigen vaccine (polypeptide vaccine and DNA vaccine), whole-cell vaccine (tumor cell vaccine and dendritic cell [DC]-based vaccine), bacterial vector vaccine, and so on.Citation47 Among these, DC-based vaccine and DNA vaccine are mostly commonly used in cancer immunotherapy. However, effective treatment by cancer vaccination needs a high delivery efficiency of cancer antigen into host antigen-presenting cells (APCs) to activate the immune response. Consequently, cancer vaccination is a targeted therapy with quite low adverse effects.
DC-based vaccination
DCs have unique capabilities involving innate and adaptive immune responses, making them an ideal APC to boost antitumor attack.Citation47,Citation48 In numerous clinical trials, DC-based vaccines have been shown to be safe and effective in cancer immunotherapy.Citation49 During DC-based vaccine therapy, loading DCs with tumor antigens and subsequently activating DCs are crucial. It has been demonstrated that transfection is significantly enhanced using microtubules, and US can activate powerful immune responses in vivo, which can be applied as a potential method for further clinical trials ().Citation50 Oda et al used microbubbles combined with US irradiation to deliver antigens that were extracted from tumor cells into DCs and then investigated the therapeutic effect of the treated DCs on a mouse model of lung metastasis. The results demonstrated that prophylactic immunization with this strategy showed significant suppression (a four-fold decrease) of melanoma lung metastases.Citation51
Figure 3 Schematic of the use of US with mRNA-loaded microbubbles.
Abbreviations: DC, dendritic cell; US, ultrasound.
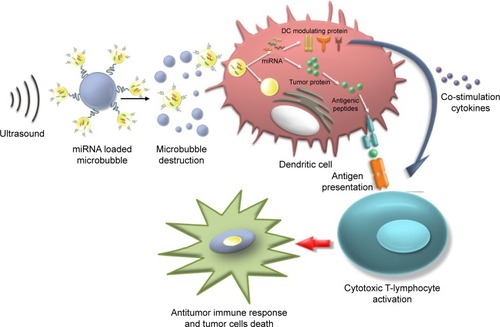
DNA vaccination
Several studies have reported that DNA vaccination can activate the immune response, including humoral immunity and cellular immunity, using cancer antigens encoded by exogenous tumor-associated genes.Citation48,Citation52 An exogenous gene encoding cancer antigens is called a DNA vaccine.Citation53,Citation54 To achieve the full therapeutic effects of DNA vaccination, it is necessary to divert the antigen-coding gene selectively and effectively into APCs (macrophages and DCs), which play a critical role in the initiation, programming and regulation of antitumor immune responses.Citation55
Many researchers have demonstrated that the effects of cancer vaccination can be improved by UMMD-triggered gene transfection technologies.Citation56 Un et al developed a mannose-modified gene carrier called Man-PEG2000 [mannose–poly(ethylene glycol) 2000] bubble lipoplexes to deliver a DNA vaccine into APCs, resulting in high antitumor effects ().Citation57 With the help of these transfection methods, it is possible to deliver a large amount of gene-loaded antigen as well as antigen peptides into APCs.Citation58 Yoshida et al synthesized a US-responsive gene carrier (doxorubicin-encapsulated poly(ethylene glycol)-modified liposome microbubble) to deliver the DNA vaccine. The results suggested that the combination of US and the DNA vaccination-loaded liposome microbubble can increase the delivery of DNA vaccine, inducing an effective therapeutic outcome for cancer immunotherapy.Citation59
Figure 4 Schematic representation of the treatment method of US exposure combined with pDNA-loaded microbubbles.
Abbreviations: Man, mannose; PEG2000, poly(ethylene glycol) 2000; NT, not treatment; SC, subcutaneous; US, ultrasound.
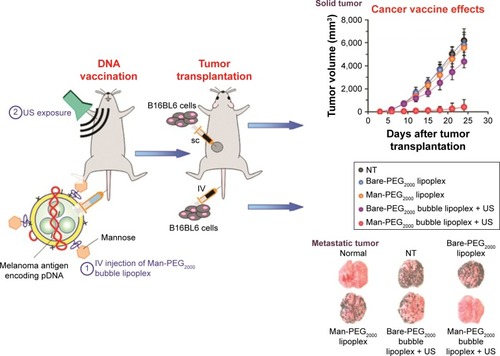
Antibody-based immunotherapy
Because immunoregulation of antitumor treatments is often used clinically today, therapeutic antibodies that can distinguish tumor cells have been developed in recent years. Antibodies can provide effective treatment by targeting specific molecular targets, thus inhibiting tumor cell growth.Citation60
Bevacizumab is a drug that targets vascular endothelial growth factor (VEGF) and tumor neovascularization, subsequently inhibiting the biological function of VEGF.Citation61 Liu et al reported enhanced delivery of the antiangiogenic antibody bevacizumab into the central nervous system using the combination of focus US, microbubble and magnetic resonance imaging (MRI) monitoring.Citation62 Rituximab is another antibody that can specifically bind to CD20+ lymphoma cells and induce cell apoptosis.Citation63 In 2017, Ishijima et al developed a phase-change nanodroplet conjugated with an antitumor antibody (9E5), and in vitro experiments showed that the antibody was delivered to 97.8% of high-epiregulin-expressing cancer cells and that 57% of those cancer cells were killed with US irradiation ().Citation64
Figure 5 Schematic explaining the synergistic treatment of ultrasound with targeted liquid fluorocarbon droplets.
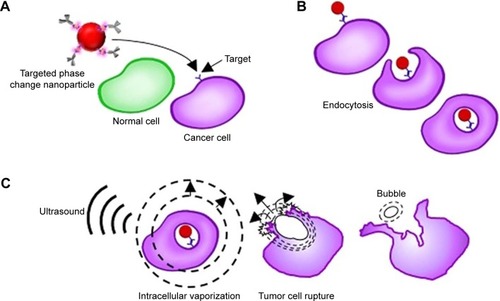
However, antibody-based immunotherapy is less efficient in solid tumors because it is difficult to enrich antibodies within the tumor and the bioavailability in the tumor is very poor.Citation65 Thus, systemic and repeated delivery of a high antibody dose is essential to reach the therapeutic concentration, which increases the side effects and costs.Citation66 Monoclonal antibodies targeting the HER2 protein, such as trastuzumab, have the potential to prolong the survival of patients with HER2+.Citation67 Trastuzumab is a humanized monoclonal antibody that is widely used in clinical treatment. However, several recent studies have reported that the use of a large amount of trastuzumab resulted in an increasing incidence of brain metastases.Citation68
Antibodies and other drugs are often unsatisfactory for the treatment of brain metastases because the BBB and blood–tumor barrier prevent most drugs entering the brain.Citation69 To effectively treat brain metastases and reduce side effects, drugs or genes must be delivered efficiently to the brain.Citation70 In the study by Kobus et al, HER2-targeting antibodies combined with US and Optison (GE Healthcare, Milwaukee, WI, USA)™ were utilized to inhibit the growth rate of a tumor model derived from HER2-positive breast cancer metastasis to the brain. The results demonstrated that the antitumor effects of antibodies can be significantly enhanced by the synergism of US and Optison.Citation71
Adoptive cell therapy
As a highly individualized cancer therapy, ACT involves the regulation of the tumor-bearing host’s immune cells with direct antitumor activity.Citation72,Citation73 ACT has been shown to be more effective than other cancer immunotherapies, although it relies on abundant antitumor immune cells with high activity, including regulatory T cells (Tregs) and NK cells.Citation74
Most importantly, adoptive cell transfer provides a beneficial microenvironment to support antitumor immunity. Until now, the most widely used immune cells in ACT have been NK cells. Transferred adoptive cells can proliferate in the new host and retain their antitumor ability. First, reinjection of a large amount of adoptive cells leads to unpredictable side effects, such as pyrexia and anaphylaxis.Citation75 Second, the number of adoptive cells delivered to the targeted areas is always too low to play an effective role in killing tumor cells. Furthermore, the normal functions of adoptive cells may be influenced by the ex vivo expansion strategy as well as the immunosuppressive effect of the self-tumor microenvironment.Citation76
NK-cell-based immunotherapy
NK cells are a type of cytotoxic T-lymphocyte that play an important antitumor role in the innate immune system. In addition, NK cells can regulate immune function as well as kill tumor cells.Citation77 Furthermore, NK cells can induce tumor cell apoptosis in an antigen-dependent method when the antibody is adherent to the receptors. NK-92 is a human NK cell line, which has been demonstrated to have connection with tumor-associated antigens in tumor tissues. However, in clinical trials, the effectiveness of NK cells in treating cerebral tumors is restricted by their poor ability to traverse the BBB.Citation78 The BBB limits the passage of most substances, including cells, nucleic acids and antibodies, from the blood circulation into the brain tissue, thus suppressing their treatment effect.
Fortunately, recent studies have shown the potential to transiently open the BBB, enabling enhanced permeation of drugs or genes. O’Reilly et al investigated the time taken for the BBB to close and the opening volume on the time scale of closure after focused US exposure; no significant differences were detected on MRI between large- and small-volume sonications, suggesting that safe BBB opening can be achieved by US combined with microbubbles.Citation79 Lin et al demonstrated that the BBB can be successfully opened by US-triggered microbubble destruction and thus the delivery of exogenous substances can be significantly improved, although the specific mechanisms are still unclear.Citation80 So far, the following statements can be made: the ARF pushes microbubbles toward the vascular wall and promotes an impact on vascular cells that induces a loose intercellular gap; and microbubbles inside the vasculature can produce microstreams and shock waves, thus compromising the stability of the vascular wall. After the safe BBB disruption with focused ultrasound (FUS) and microbubbles (), NK-92 cells are largely delivered into brain tumor tissues and exert their anticancer effects, causing a higher suppression of tumor growth and longer survival time in a mouse brain tumor model compared to the non-treatment prototype.Citation81 Alkins et al labeled HER2 high-expressing breast tumor cells with superparamagnetic iron oxide (SPIO) and implanted them into nude rats. Following transcranial FUS irradiation and intravenous injection of SPIO-labeled NK cells, MRI showed a remarkable spark drop, indicating successful homing clustering of NK cells.Citation82
Figure 6 Schematic of FUS-induced BBB opening to enhance IL-12 delivery in brain glioma treatment.
Abbreviations: BBB, blood–brain barrier; FUS, focused ultrasound; IL-12, interleukin-12.
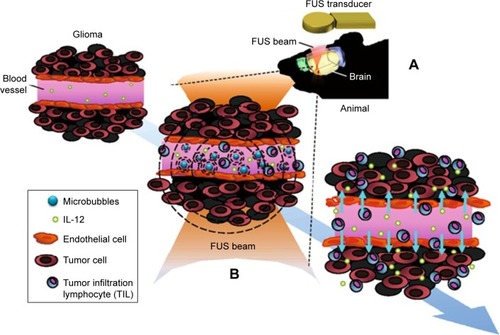
T-cell-based immunotherapy
Tregs are known to produce ILs and transforming growth factors, but they have an inhibitory effect on tumor-associated APCs because tumor-associated APCs, such as DCs, lose their co-stimulatory ligands, causing an inability to support T-cell activation.Citation83 In addition, CD4+CD25+ Tregs are reported to have an important effect on antitumor immune responses.Citation84 Tregs may induce immune tolerance to self-antigens and suppress the self-immune response against cancer by suppressing reactive immune cells. In addition, the poor clinical efficacy of antitumor immunotherapy may result from the excessive presence of Tregs.Citation85,Citation86 Therefore, the strategy of using targeted Tregs will be an effective treatment to enhance antitumor immunotherapy.Citation87 Shi et al studied the treatment effects on the viability of CD4+CD25+ Tregs induced by the combination of US and SonoVue® (Bracco Co. Geneva, Switzerland) microbubbles, and explored the optimal US parameters for Treg transfection. Their results suggest that US and microbubbles have a synergistic effect on the enhancement of Treg proliferation, and the optimal Treg transfer rate was acquired by irradiation with US for 150/180 seconds under a US mechanical index of 1.4 in the presence of 10% microbubbles.Citation88–Citation90
Cytokine immunotherapy
The aim of cytokine immunotherapy is to deliver cytokines to a local area at a high concentration to induce a forceful antitumor immune response. In the recent literature on cytokine immunotherapy triggered by US and microbubbles, several trials have delivered cytokines, including IL-2, IL-10 and IL-13, as well as tumor growth factors, to stimulate an immune response.Citation72,Citation91
IL-based immunotherapy
ILs have been reported to have an antitumor effect because they can serve as immunostimulatory molecules that can trigger an antitumor immune response. Among the cytokines mentioned in the previous paragraph, IL-12 plays the most important role in immunity and tumor angiogenesis; thus, it has garnered the most attention. IL-12 is a heterodimeric protein consisting of p35 and p40 subunits produced by DCs and macrophages, and has multiple immunoregulatory and antitumor effects.Citation92
In turn, activated T cells increase the level of IFN-γ, which triggers positive feedback on APCs to secrete IL-12.Citation93 Suzuki et al assessed the utility of the combination of IL-12-loaded microbubbles and US in cancer therapy and found that this system can induce a T-cell-dependent immune response that can dramatically suppress tumor growth.Citation94 In addition, Chen et al applied FUS to induce BBB opening and subsequent safe IL-12 delivery, and found that this method activated local immune responses to enhance antitumor effects.Citation95
IFN-based immunotherapy
IFN has several biological effects, such as immunoregulation and anti-proliferative activities, on some cancer cells. Moreover, IFN induces cell apoptosis, resulting in tumor inhibition. Sakakima et al examined tumor suppression after IFN gene transfection with the combination of US and a mixture of IFN and microbubbles. The results revealed that the tumor size was significantly reduced after IFN gene transfection, indicating that IFN-based antitumor immunotherapy with sonoporation may be a new treatment option for tumors.Citation96,Citation97
Conclusions and future prospects
UMMD is now considered to be a promising non-viral gene/drug delivery system. This technology combines the advantages of microbubbles/nanodroplets and US in such a way that US-triggered microbubbles or nanodroplet destruction induces a series of physical effects. Thus, the permeability of physiological barriers can be instantaneously enhanced, allowing immunostimulatory materials (ie, antibodies, antigens, immune cells and vaccines) to traverse across the barriers to exert their effects.Citation98 Antitumor immune responses have been achieved by delivering immunostimulatory substances using the combination of US and microbubbles and nanoparticles. UMMD-mediated immunotherapy is in its infancy, but provides promising strategies for cancer treatment.
Recent research progress concerning the dynamic and complicated interactions between the immune system and cancer plays a role in guiding cancer immunotherapy, which will be available for innovative cancer therapy. Numerous studies have utilized microbubbles/nanoparticles as delivery vehicles in combination with US to transport antibodies, antigens and immunostimulatory molecules to APCs, which have shown enhanced CD4+ and CD8+ T responses against tumors. Likewise, other macromolecules, including miRNA and pDNA, can be delivered into tumor tissues in this way.
However, both microbubbles and nanoparticles have two major disadvantages for utilization as delivery carriers to tumor cells. First, they are too large to easily and effectively traverse many barriers. Second, the half-life of gas-filled microbubbles or nanobubbles is fairly short in vivo, resulting in a poor US-triggered ability. Furthermore, the low systemic cytotoxicity, high specificity and lasting efficacy, as well as the good bioavailability of gene/drug vehicles, are clinically challenging for optimizing delivery technology.Citation99
The improvement of this method relies on several main strategies. The first possible solution to this issue is replacing the gas in the microbubble with perfluorooctylbromide. Although some researchers have explored this idea and generated results, further study is required.Citation100 Another potential method is using targeting ligands specific to immune cells,Citation101 so that effective immunotherapy can be optimized. Finally, when using UMMD-based immunotherapies, the differences between liquid and solid tumors should be considered.
Acknowledgments
This work is supported by the Research Projects of the National Natural Science Foundation of China (number 81671707), the Natural Science Foundation of Guangdong Province (number 2016A030311054), the Research Projects of Guangzhou Technology Bureau (number 201607010201) and the Research Fund for Lin He’s Academician Workstation of New Medicine and Clinical Translation.
Disclosure
The authors report no conflicts of interest in this work.
References
- TorreLABrayFSiegelRLFerlayJLortet-TieulentJJemalAGlobal cancer statistics, 2012CA Cancer J Clin20156528710825651787
- LindauDGielenPKroesenMWesselingPAdemaGJThe immunosuppressive tumour network: myeloid-derived suppressor cells, regulatory T cells and natural killer T cellsImmunology2013138210511523216602
- MillerJFAPSadelainMThe journey from discoveries in fundamental immunology to cancer immunotherapyCancer Cell201527443944925858803
- KnutsonKLKaryampudiLLamichhanePPrestonCTargeted immune therapy of ovarian cancerCancer Metastasis Rev2015341537425544369
- TopalianSLDrakeCGPardollDMImmune checkpoint blockade: a common denominator approach to cancer therapyCancer Cell201527445046125858804
- WangWXiaXWuSCancer immunotherapy: A need for peripheral immunodynamic monitoringAm J Reprod Immunol201720Pt 1e12793
- Madorsky RowdoFPBaronAUrrutiaMMordohJImmunotherapy in Cancer: A Combat between Tumors and the Immune System; You Win Some, You Lose SomeFront Immunol201562312725859247
- KapadiaCHPerryJLTianSLuftJCDeSimoneJMNanoparticulate immunotherapy for cancerJ Control Release201521916718026432555
- YadavMDelamarreLIMMUNOTHERAPY. Outsourcing the immune response to cancerScience201635262911275127627284181
- RosenbergSADecade in review-cancer immunotherapy: entering the mainstream of cancer treatmentNat Rev Clin Oncol2014111163063225311350
- YuBWangJHeCCytokine-induced killer cell therapy for modulating regulatory T cells in patients with non-small cell lung cancerExp Ther Med201714183184028673007
- FontanaFLiuDHirvonenJSantosHADelivery of therapeutics with nanoparticles: what’s new in cancer immunotherapy?Wiley Interdiscip Rev Nanomed Nanobiotechnol201791e1421
- ChenZ-YLiangKQiuR-XTargeted gene delivery in tumor xenografts by the combination of ultrasound-targeted microbubble destruction and polyethylenimine to inhibit survivin gene expression and induce apoptosisJ Exp Clin Cancer Res201029115221092274
- AryalMParkJVykhodtsevaNZhangY-ZMcDannoldNEnhancement in blood-tumor barrier permeability and delivery of liposomal doxorubicin using focused ultrasound and microbubbles: evaluation during tumor progression in a rat glioma modelPhys Med Biol20156062511252725746014
- ChenZ-YYangFLinYNew development and application of ultrasound targeted microbubble destruction in gene therapy and drug deliveryCurr Gene Ther201313425027423721204
- LuoMHYehCKSituBYuJSLiBCChenZYMicrobubbles: A Novel Strategy for ChemotherapyCurr Pharm Des201723233383339028088911
- KangSTYehCKUltrasound microbubble contrast agents for diagnostic and therapeutic applications: current status and future designChang Gung Med J201235212513922537927
- CarsonARMctiernanCFLaveryLUltrasound-targeted microbubble destruction to deliver siRNA cancer therapyCancer Res201272236191619923010078
- FanCHLinCYLiuHLYehCKUltrasound targeted CNS gene delivery for Parkinson’s disease treatmentJ Control Release201726124626228690161
- VanbavelEEffects of shear stress on endothelial cells: Possible relevance for ultrasound applicationsProg Biophys Mol Biol2007931–337438316970981
- WuJNyborgWLUltrasound, cavitation bubbles and their interaction with cellsAdv Drug Deliv Rev200860101103111618468716
- MauriGNicosiaLXuZFocused ultrasound: tumor ablation and its potential to enhance immunological therapy to cancerBr J Radiol20189110832017064129168922
- JinQKangS-TChangY-CZhengHYehC-KInertial cavitation initiated by polytetrafluoroethylene nanoparticles under pulsed ultrasound stimulationUltrason Sonochem2016321727150739
- LiuH-LFanC-HTingC-YYehC-KCombining microbubbles and ultrasound for drug delivery to brain tumors: current progress and overviewTheranostics20144443244424578726
- QinPHanTYuAXuLMechanistic understanding the bioeffects of ultrasound-driven microbubbles to enhance macromolecule deliveryJ Control Release201827216918129305924
- AndrewsNWCorrotteMCastro-GomesTAbove the fray: Surface remodeling by secreted lysosomal enzymes leads to endocytosis-mediated plasma membrane repairSemin Cell Dev Biol201545101726433178
- FanPZhangYGuoXCell-cycle-specific Cellular Responses to SonoporationTheranostics20177194894490829187912
- FanZKumonREParkJDengCXIntracellular delivery and calcium transients generated in sonoporation facilitated by microbubblesJ Control Release20101421313919818371
- TamCIdoneVDevlinCExocytosis of acid sphingomyelinase by wounded cells promotes endocytosis and plasma membrane repairJ Cell Biol201018961027103820530211
- KooimanKVosHJVersluisMde JongNAcoustic behavior of microbubbles and implications for drug deliveryAdv Drug Deliv Rev20147222284824667643
- KuznetsovaLACoakleyWTApplications of ultrasound streaming and radiation force in biosensorsBiosens Bioelectron20072281567157716979887
- RychakJJKlibanovALHossackJAAcoustic radiation force enhances targeted delivery of ultrasound contrast microbubbles: in vitro verificationIEEE Trans Ultrason Ferroelectr Freq Control200552342143315857050
- HerbstEBUnnikrishnanSWangSKlibanovALHossackJAMauldinFWJrThe Use of Acoustic Radiation Force Decorrelation-Weighted Pulse Inversion for Enhanced Ultrasound Contrast ImagingInvest Radiol20175229510227495188
- FangRHZhangLNanoparticle-Based Modulation of the Immune SystemAnnu Rev Chem Biomol Eng20167130532627146556
- KumariPGhoshBBiswasSNanocarriers for cancer-targeted drug deliveryJ Drug Target201624317919126061298
- FreyABSuppression of T cell responses in the tumor microenvironmentVaccine201533517393740026403368
- KooHHuhMSSunI-CIn Vivo Targeted Delivery of Nano-particles for TheranosisAcc Chem Res201144101018102821851104
- GradisharWJTjulandinSDavidsonNPhase III trial of nanoparticle albumin-bound paclitaxel compared with polyethylated castor oil-based paclitaxel in women with breast cancerJ Clin Oncol200523317794780316172456
- Paz-AresLRossHO’BrienMPhase III trial comparing paclitaxel poliglumex vs docetaxel in the second-line treatment of non-small-cell lung cancerBr J Cancer200898101608161318475293
- BlumNTYildirimAChattarajRGoodwinAPNanoparticles Formed by Acoustic Destruction of Microbubbles and Their Utilization for Imaging and Effects on Therapy by High Intensity Focused UltrasoundTheranostics20177369470228255360
- ZhouYWangZChenYMicrobubbles from gas-generating perfluorohexane nanoemulsions for targeted temperature-sensitive ultrasonography and synergistic HIFU ablation of tumorsAdv Mater201325304123413023788403
- SheeranPSDaytonPAPhase-change contrast agents for imaging and therapyCurr Pharm Des201218152152216522352770
- HoYJChangYCYehCKImproving Nanoparticle Penetration in Tumors by Vascular Disruption with Acoustic Droplet VaporizationTheranostics20166339240326909113
- ZhouYApplication of acoustic droplet vaporization in ultrasound therapyJ Ther Ultrasound2015312026566442
- YangQNanayakkaraGKDrummerCLow-Intensity Ultrasound-Induced Anti-inflammatory Effects Are Mediated by Several New Mechanisms Including Gene Induction, Immunosuppressor Cell Promotion, and Enhancement of Exosome Biogenesis and DockingFront Physiol2017881829109687
- ZhangWShouW-DXuY-JBaiW-KHuBLow-frequency ultrasound-induced VEGF suppression and synergy with dendritic cell-mediated anti-tumor immunity in murine prostate cancer cells in vitroSci Rep201771577828720900
- MoyerTJZmolekACIrvineDJBeyond antigens and adjuvants: formulating future vaccinesJ Clin Invest2016126379980826928033
- SchreibeltGBolKFWestdorpHEffective Clinical Responses in Metastatic Melanoma Patients after Vaccination with Primary Myeloid Dendritic CellsClin Cancer Res20162292155216626712687
- TjoaBALodgePASalgallerMLBoyntonALMurphyGPDendritic cell-based immunotherapy for prostate cancerCA Cancer J Clin199949211712811198886
- DewitteHVan LintSHeirmanCThe potential of antigen and TriMix sonoporation using mRNA-loaded microbubbles for ultrasound-triggered cancer immunotherapyJ Control Release201419412283625151979
- OdaYSuzukiROtakeSProphylactic immunization with Bubble liposomes and ultrasound-treated dendritic cells provided a four-fold decrease in the frequency of melanoma lung metastasisJ Control Release2012160236236622192573
- KutzlerMAWeinerDBDNA vaccines: ready for prime time?Nat Rev Genet200891077678818781156
- StifterKSchusterCSchlosserMBoehmBOSchirmbeckRExploring the induction of preproinsulin-specific Foxp3+CD4+ Treg cells that inhibit CD8+ T cell-mediated autoimmune diabetes by DNA vaccinationSci Rep201662941927406624
- GongY-FZhouQ-BLiaoY-DOptimized construction of MUC1-VNTRn DNA vaccine and its anti-pancreatic cancer efficacyOncol Lett20171342198220628454381
- MeliefCJMCancer Immunotherapy by Dendritic CellsImmunity200829337238318799145
- SuzukiROdaYUtoguchiNA novel strategy utilizing ultrasound for antigen delivery in dendritic cell-based cancer immunotherapyJ Control Release2009133319820519000727
- UnKKawakamiSSuzukiRMaruyamaKYamashitaFHashidaMSuppression of melanoma growth and metastasis by DNA vaccination using an ultrasound-responsive and mannose-modified gene carrierMol Pharm20118254355421250746
- UnKKawakamiSSuzukiRMaruyamaKYamashitaFHashidaMDevelopment of an ultrasound-responsive and mannose-modified gene carrier for DNA vaccine therapyBiomaterials201031307813782620656348
- YoshidaMKawakamiSKonoYEnhancement of the anti-tumor effect of DNA vaccination using an ultrasound-responsive mannose-modified gene carrier in combination with doxorubicin-encapsulated PEGylated liposomesInt J Pharm20144751–240140725218184
- WeinerLMMurrayJCShuptrineCWAntibody-based immunotherapy of cancerCell201214861081108422424219
- SandlerAGrayRPerryMCPaclitaxel-carboplatin alone or with bevacizumab for non-small-cell lung cancerN Engl J Med2006355242542255017167137
- LiuH-LHsuP-HLinC-YFocused Ultrasound Enhances Central Nervous System Delivery of Bevacizumab for Malignant Glioma TreatmentRadiology201628119910827192459
- MarcusRImrieKBelchACVP chemotherapy plus rituximab compared with CVP as first-line treatment for advanced follicular lymphomaBlood200510541417142315494430
- IshijimaAMinamihataKYamaguchiSSelective intracellular vaporisation of antibody-conjugated phase-change nano-droplets in vitroSci Rep201774407728333127
- ZhouSZhangXWangCRituximab-conjugated, doxorubicin-loaded microbubbles as a theranostic modality in B-cell lymphomaOncotarget20168347604772
- MinchintonAITannockIFDrug penetration in solid tumoursNat Rev Cancer20066858359216862189
- NutiMBellatiFViscontiVImmune Effects of TrastuzumabJ Cancer20112131732321716848
- HortonJKHalleJFerraroMRadiosensitization of chemotherapy-refractory, locally advanced or locally recurrent breast cancer with trastuzumab: a phase II trialInt J Radiat Oncol Biol Phys2010764998100419560883
- ChenP-YWeiK-CLiuH-LNeural immune modulation and immunotherapy assisted by focused ultrasound induced blood-brain barrier openingHum Vaccin Immunother201511112682268726378609
- CentellesMNWrightMGedroycWThanouMFocused ultrasound induced hyperthermia accelerates and increases the uptake of anti-HER-2 antibodies in a xenograft modelPharmacol Res201611414415127771465
- KobusTZervantonakisIKZhangYMcDannoldNJGrowth inhibition in a brain metastasis model by antibody delivery using focused ultrasound-mediated blood-brain barrier disruptionJ Control Release201623828128827496633
- AndersenRDoniaMBorchTAdoptive cell therapy with tumor infiltrating lymphocytes and intermediate dose IL-2 for metastatic melanomaJ Immunother Cancer20142Suppl 3P1
- PaluckaKUenoHBanchereauJRecent developments in cancer vaccinesJ Immunol201118631325133121248270
- SmithDMSimonJKBakerJRJrApplications of nanotechnology for immunologyNat Rev Immunol201313859260523883969
- BudhuSWolchokJMerghoubTThe importance of animal models in tumor immunity and immunotherapyCurr Opin Genet Dev2014244465124657536
- SingerBDKingLSD’AlessioFRRegulatory T cells as immunotherapyFront Immunol201454624575095
- ChengMChenYXiaoWSunRTianZNK cell-based immunotherapy for malignant diseasesCell Mol Immunol201310323025223604045
- Sta MariaNSBarnesSRWeistMRColcherDRaubitschekAAJacobsRELow Dose Focused Ultrasound Induces Enhanced Tumor Accumulation of Natural Killer CellsPLoS One20151011e014276726556731
- O’ReillyMAHoughOHynynenKBlood-Brain Barrier Closure Time After Controlled Ultrasound-Induced Opening Is Independent of Opening VolumeJ Ultrasound Med201736347548328108988
- LinC-YHsiehH-YPittWGFocused ultrasound-induced blood-brain barrier opening for non-viral, non-invasive, and targeted gene deliveryJ Control Release20152121926071631
- AlkinsRBurgessAKerbelRWelsWSHynynenKFranciaGEarly treatment of HER2-amplified brain tumors with targeted NK-92 cells and focused ultrasound improves survivalNeuro Oncol201618797498126819443
- AlkinsRBurgessAGangulyMFocused ultrasound delivers targeted immune cells to metastatic brain tumorsCancer Res20137361892189923302230
- MunnDHBronteVImmune suppressive mechanisms in the tumor microenvironmentCurr Opin Immunol2016391626609943
- ReadSMalmströmVPowrieFCytotoxic T lymphocyte-associated antigen 4 plays an essential role in the function of CD25+ CD4+ regulatory cells that control intestinal inflammationJ Exp Med2000192229530210899916
- MatheuMPOthySGreenbergMLImaging regulatory T cell dynamics and CTLA4-mediated suppression of T cell primingNat Commun201561621925653051
- ShuZShenYSongYYingYHow to detour Treg cells in T cell-based antitumor immune therapyOnco Targets Ther201361243124724043948
- SchabowskyRHMadireddiSSharmaRYolcuESShirwanHTargeting CD4+CD25+FoxP3+ regulatory T-cells for the augmentation of cancer immunotherapyCurr Opin Investig Drugs200781210021008
- ShiCZhangYYangHCombined effect of ultrasound/SonoVue microbubble on CD4+CD25+ regulatory T cells viability and optimized parameters for its transfectionUltrasonics2015629710226048174
- ZhouQZhangYDuJDifferent-Sized Gold Nanoparticle Activator/Antigen Increases Dendritic Cells Accumulation in Liver-Draining Lymph Nodes and CD8+ T Cell ResponsesACS Nano20161022678269226771692
- RosaliaRACruzLJvan DuikerenSCD40-targeted dendritic cell delivery of PLGA-nanoparticle vaccines induce potent anti-tumor responsesBiomaterials201540889725465442
- MittalSKRochePASuppression of antigen presentation by IL-10Curr Opin Immunol201534222725597442
- AlatrashGHutsonTEMoltoLClinical and immunologic effects of subcutaneously administered interleukin-12 and interferon alfa-2b: phase I trial of patients with metastatic renal cell carcinoma or malignant melanomaJ Clini Oncol2004221428912900
- CiccheleroLDeniesSHaersHIntratumoural interleukin 12 gene therapy stimulates the immune system and decreases angiogenesis in dogs with spontaneous cancerVet Comp Oncol20161541187120527506827
- SuzukiRNamaiEOdaYCancer gene therapy by IL-12 gene delivery using liposomal bubbles and tumoral ultrasound exposureJ Control Release2009358245250
- ChenP-YHsiehH-YHuangC-YFocused ultrasound-induced blood–brain barrier opening to enhance interleukin-12 delivery for brain tumor immunotherapy: a preclinical feasibility studyJ Transl Med20151319325784614
- SakakimaYHayashiSYagiYHayakawaATachibanaKNakaoAGene therapy for hepatocellular carcinoma using sonoporation enhanced by contrast agentsCancer Gene Ther2005121188488915891773
- LiuS-YWeiWYueHNanoparticles-based multi-adjuvant whole cell tumor vaccine for cancer immunotherapyBiomaterials201334338291830023910466
- EscoffreJMDeckersRBosCMoonenCBubble-Assisted Ultrasound: Application in Immunotherapy and VaccinationAdv Exp Med Biol201688024326126486342
- ZangXZhaoXHuHQiaoMDengYChenDNanoparticles for tumor immunotherapyEur J Pharm Biopharm201711524325628323111
- SinghRHusseiniGAPittWGPhase transitions of nanoemulsions using ultrasound: experimental observationsUltrason Sonochem20121951120112522444691
- ZhuLZhaoHZhouZPeptide-Functionalized Phase-Transformation Nanoparticles for Low Intensity Focused Ultrasound-Assisted Tumor Imaging and TherapyNano Lett20181831831184129419305