Abstract
Self-calcifying, self-replicating nanoparticles have been isolated from calcified human tissues. However, it is unclear if these nanoparticles participate in disease processes. Therefore, this study was designed to preliminarily test the hypothesis that human-derived nanoparticles are causal to arterial disease processes. One carotid artery of 3 kg male rabbits was denuded of endothelium; the contralateral artery remained unoperated as a control. Each rabbit was injected intravenously with either saline, calcified, or decalcified nanoparticles cultured from calcified human arteries or kidney stones. After 35 days, both injured and control arteries were removed for histological examination. Injured arteries from rabbits injected with saline showed minimal, eccentric intimal hyperplasia. Injured arteries from rabbits injected with calcified kidney stone- and arterial-derived nanoparticles occluded, sometimes with canalization. The calcified kidney stone-derived nanoparticles caused calcifications within the occlusion. Responses to injury in rabbits injected with decalcified kidney stone-derived nanoparticles were similar to those observed in saline-injected animals. However, decalcified arterial-derived nanoparticles produced intimal hyperplasia that varied from moderate to occlusion with canalization and calcification. This study offers the first evidence that there may be a causal relationship between human-derived nanoparticles and response to injury including calcification in arteries with damaged endothelium.
Introduction
Self-replicating, self calcifying nanoparticles (NPs, formerly called nanobacteria) ranging in size of 0.08–0.5 μm which form a biofilm containing carbonate or hydroxylapatite have been isolated and propagated from mammalian blood (CitationKajander et al 1997) and calcified human arterial tissue and kidney stones (CitationMiller et al 2004; CitationShiekh et al 2006). Although the exact nature of these nano-sized particles remains controversial, their propagation is reduced by certain antibiotics and inhibitors of oxidative metabolism (CitationBjorklund et al 1998; CitationCiftcioglu et al 2002; CitationKumar et al 2006). They incorporate radiolabeled uridine and phosphate into their structures and some sequences of nucleic acids have been isolated from biofilms containing nanosized structures (CitationKajander et al 1997; CitationCisar et al 2000; CitationMiller et al 2004; CitationKumar et al 2006). Propagation seems to be accelerated in environments simulating microgravity (CitationCiftcioglu et al 2005).
In addition to calcified renal and arterial tissue, similar structures are found in human urine, renal cyst fluid (CitationCiftcioglu et al 2002), capsules from breast implants (CitationDeva and Chang 1999), prostatitis (CitationShoskes et al 2005), gall stones (CitationWang et al 2006), dental plaque (CitationCiftcioglu et al 2003), intestinal tissue affected by inflammatory bowel disease (CitationMeissner et al 2006), and ovarian cancer (CitationSedivy and Battistutti 2003). In spite of these associations, a cause and effect relationship between NPs and disease pathogenesis remains unclear. Therefore, in order to show a causal relationship between human-derived NPs and disease, Koch’s postulates dictate that particles (organisms) must be identified in diseased tissue, isolated and propagated from diseased tissue, and inoculation of an animal with the isolates should recapitulate the disease. Therefore, this pilot study was designed to test the hypothesis that inoculation of naïve animals with different preparations of self-replicating, self-calcifying, human-derived NPs would initiate disease processes, specifically arterial calcification, in rabbits.
Methods
Nanoparticle culture and collection
Self-replicating, self calcifying NPs derived from human calcified aortic aneurysms and kidney stones (CitationMiller et al 2004) were used in these experiments. NPs for injection were isolated from mature cultures which had formed a characteristic biofilm of calcified domes adhering to the bottom of polystyrene culture flasks. Culture flasks contained Dulbecco’s Modified Eagle Medium (DMEM 10-013, Mediatech Inc, Manassas, VA) supplemented with 50 μM beta-mercaptoethanol (Sigma Chemical Co, St. Louis, MO), 10% γ-irradiated fetal calf serum (Atlanta Biologicals Inc, Lawrenceville, GA), and CaCl2 (Sigma) to 3.6 mM.
Calcified human-derived NPs were collected by gently washing 10 confluent flasks twice with 10 ml sterile phosphate-buffered saline (PBS). The flasks were scraped to loosen NP colonies from the flask and the scrapings collected and centrifuged (20 min, 4 °C, 20,000 g). After each spin, tubes were consolidated in 0.5 ml PBS and centrifuged again. Once all pellets had been consolidated to a single tube, the pellet was washed twice with Tris buffer (10 mM) and the final pellet was suspended in 1 ml sterile saline for injection into the animal.
Decalcified human-derived NPs were collected by gently washing 3 confluent flasks twice with 10 ml sterile PBS containing 0.5 M ethylendiaminetetraacetic acid (EDTA) and then adding 1 ml of 0.5 M EDTA to the flasks for 1 h. The flasks were then scraped and the resulting solution was centrifuged (30 min, 4 °C, 60,000 g). Pellets were consolidated, washed, and re-suspended as described above for the calcified NPs. NPs ranged in size from 50–200 nm and in some situations formed clumps (please see CitationMiller et al 2004).
Animals and surgery
Adult male (3 kg) New Zealand white rabbits (n = 9 total) were fed LabDiet hi-fiber rabbit chow ad libitum and were maintained in individual cages (12 hr light/12 hr dark cycle). Once animals were inoculated with NPs, they were maintained in a Biohazard Level 2 safety facility. All protocols were approved by the Animal Care and Use Committee and Biosafety Committee of the Mayo Clinic.
The left carotid artery of each animal was denuded of endothelium using balloon injury. Briefly, animals were sedated intramuscularly using a mixture of ketamine (35 mg/kg, Ketaset, Fort Dodge Animal Health, Fort Dodge, IA), xylazine (5 mg/kg, VetTek, Blue Springs, MO) and acepromazine (1 mg/kg, Boehringer Ingelheim Vetmedica Inc, St. Joseph, MO). They received a prophylactic dose of 0.25 ml penicillin (Pen-aqueous, Durvet, Inc., Blue Springs, MO) IM to reduce infection related to the surgery. This antibiotic does not interfere with NP replication in culture (CitationCiftcioglu et al 2002). Saline (60 ml, 0.9% NaCl, Baxter Healthcare Corp, Deerfield, IL) was administered subcutaneously to prevent dehydration during the surgery. Using universal aseptic techniques, the left carotid artery was exposed and mobilized by gentle blunt dissection through a ventral midline incision in the neck. Small side branches were exposed and cauterized at least 5 mm away from the common carotid. Heparin (Baxter Healthcare Corp, Deerfield, IL) was administered (1000 U/kg) through a catheter in the marginal ear vein. The carotid artery was then temporarily occluded proximally and distally with microvascular clamps.
Monofilament suture (8–0 Ethilon; Ethicon, Somerville, NJ) was used to create a purse string suture in the anterior region of the occluded artery. A 3.0 French Fogarty (Edwards Lifesciences, Irvine, CA) angioplasty catheter was introduced into the lumen through a small incision made in the center of the purse-string suture. The balloon was inflated until the artery was distended to 150%. The catheter was withdrawn and advanced three times in order to denude the endothelium. The incision was closed with the purse-string suture. The injured area was marked by placing one small suture at the distal end of the artery where the balloon had been extended. The vascular clamps were then removed. The remaining carotid artery was not exposed, serving as the unoperated contralateral control. The surgical site was closed in three layers, the final closure being a subcuticular stitch so that the animal could not chew the sutures.
Inoculation
While still under anesthesia, and within 3 hrs after the surgery, animals were injected with one of the following solutions (total volume of inoculant and saline washes was 5 ml) via a catheter placed in the marginal ear vein:
Saline (Control; n = 2). This group was the standard for the healing response.
Calcified arterial-derived nanoparticles derived from 10 flasks (n = 1).
Calcified kidney stone-derived nanoparticles derived from 10 flasks (n = 1).
Decalcified arterial-derived nanoparticles derived from three flasks (n = 3).
Decalcified kidney stone-derived nanoparticles derived from three flasks (n = 2).
Tissue collection
At 35 days post-operative, animals were anesthetized and carotid arteries were removed, fixed in formalin, embedded in paraffin, sectioned, and examined histologically. Adjacent sections (5 micron) from each artery were evaluated by light microscopy for general anatomical features such as intima:media ratio, presence of a fibrous cap, and calcification using elastin van Giesen, hematoxylin and eosin, and von Kossa stains, respectively. Slides were viewed on an Axioplan 2 upright microscope equipped with a Plan-Neofluar 5×, 0.15 na objective lens (Zeiss, Thornwood, NY). Images were digitized with an AxioCam HRc camera (Zeiss) mounted to the microscope with a 0.63 × c-mount and interfaced through KS400 image analysis software (Zeiss). Lumen, intima, and media borders were interactively selected and the intimal and medial areas were calculated with the KS400 software.
Histological analysis
The extent of myointimal hyperplasia was evaluated based on intima:media ratios and a qualitative analysis of the condition of the internal elastic lamina and media in arterial cross-sections. One section per stain was evaluated from each animal (three sections/animal).
Results
All animals remained in good health throughout the study and none showed anorexia or signs of neurological deficits (eye drooping, immobility, etc). The uninjured artery from all rabbits in all groups showed normal anatomy with a single layer of endothelial cells, intact internal elastic lamina and media, and an adventitia devoid of inflammatory cells (, upper left panel). The injured artery of animals injected with saline showed eccentric intimal hyperplasia (, upper right panel); the intima:media ratios for the injured arteries from the two animals injected with saline were 0.39 and 1.33.
Figure 1 Representative light micrographs of sections (5 μm) of an uninjured (upper left panel) carotid artery from a rabbit inoculated with human arterial-derived nanoparticles, and the injured carotid artery of a rabbit inoculated with saline (control; upper right panel); calcified human arterial-derived (middle left panel) and calcified human kidney stone-derived (middle right panel and bottom right panel) nanoparticles; decalcified human arterial-derived nanoparticles (bottom left panel). All tissue was collected 5 weeks post-inoculation and sections are stained with either elastin van Giesen stain (upper and middle panels) or von Kossa stain (brown-black; lower panels). Sections are shown at the lowest (5X) magnification in order to show the entire artery. Uninjured arteries of rabbits in each treatment group are indistinguishable from that shown. Arrows indicate internal elastic lamina, bar indicates intimal thickening.
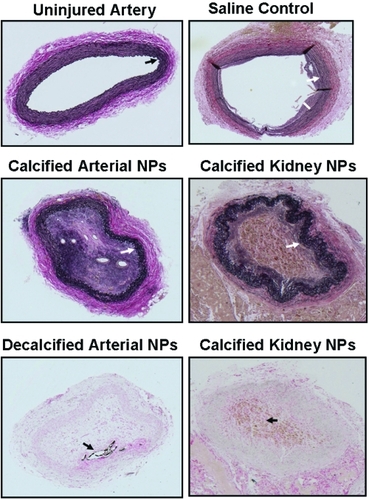
The lumen of the injured artery of each rabbit inoculated with calcified arterial- or kidney-stone-derived NPs was filled with acellular and cellular material which was canalized with blood vessels. The media layer lost cohesion even though the internal elastic lamina remained intact (; middle panels).
The extent of vascular healing and intimal hyperplasia in injured carotid arteries of rabbits injected with decalcified arterial-derived NPs was variable. In one animal, there was minimal intimal hyperplasia consistent with that observed in the injured arteries of animals injected with saline (, left panel). The art ery from another rabbit inoculated with decalcified arterial-derived NPs had greater myointimal hyperplasia than observed in saline-injected animals and the internal elastic lamina was discontinuous (, middle panel). In the third rabbit injected with decalcified arterial-derived NPs, the denuded artery occluded (with canalization) and a plaque, which stained positive for calcium phosphate, formed along the intima-medial border where the internal elastic lamina was disrupted (, right panel and , bottom left panel). Injured arteries from the two animals inoculated with decalcified NP of kidney stone origin had milder injury responses similar to the two animals inoculated with decalcified NP of aneurysmal origin shown in the left and center panels of .
Figure 2 Representative light micrographs of sections (5 μm) of injured carotid arteries of three rabbits inoculated with decalcified human arterial-derived nanoparticles. All tissue was collected 5 weeks post-inoculation and stained with an elastin van Giesen stain. Sections are shown at the lowest (5X) magnification in order to show the entire artery. Uninjured arteries in these rabbits are indistinguishable from that shown in , upper left panel. Injured arteries from rabbits injected with decalcified human arterial-derived nanoparticles exhibited a spectrum of vascular healing, ranging from disruption of the internal elastic lamina with minimal development of intimal hyperplasia (left panel), disruption of the internal elastic lamina and greater intimal hyperplasia than developed in the saline-injected rabbits (middle panel), to complete occlusion with canalization and calcification (right panel). Arrows indicate internal elastic lamina, bars indicate intimal thickening.
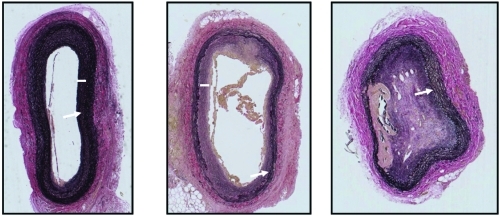
Diffuse staining for calcium phosphate by von Kossa stain was identified within the occluded injured artery of the animal injected with calcified NPs of kidney stone origin (, lower right panel). A defined area of calcification was present in the occlusion of one animal injected with decalcified NPs of aneurysmal origin (, lower left panel).
Discussion
Results of this study, while preliminary, provide the first evidence of a causal relationship between human-derived NPs and arterial disease processes including accelerated development of intimal hyperplasia and calcification. Evidence has been published to support a causal relationship between NPs and black pigment gall stones (CitationWang et al 2006) and renal lithogenesis (CitationCiftcioglu et al 2005; CitationShiekh et al 2006). However, the gall stone study utilized direct injection of NPs into the target organ. Intravenous injection of kidney-derived NPs localized to the kidney in the nephrolithiasis study (CitationShiekh et al 2006). The present study extends these observations to demonstrate that an intravenous injection of kidney stone- and arterial-derived NPs accelerates a pathological process initiated by other stimuli, in this case, mechanical endothelial denudation. This systemic exposure to NPs might be comparable to that resulting from an environmental exposure as could occur in humans. The systemic route of administration, and perhaps an immune response to the NPs, may explain some of the variability in the vascular healing response observed in animals inoculated with the human-derived NPs. Whether NPs would accelerate the response to vascular injury caused by other means, ie, endothelial dysfunction due to hyperlipidemia, remains to be determined.
Variability in vascular responses in animals exposed to human-derived NPs may also have resulted from the way inoculants were prepared. Calcified NPs were derived from combined scrapings of biofilm from 10 flasks and, even with several washing of the scraped biofilm, some proteins in the culture media may remain adhered to hydroxyapatite shells of the NPs, initiating an immune response. Future experiments could investigate the contribution of inorganic hydroxyapatite as a carrier of mitogenic proteins and stimuli of inflammation (CitationNadra et al 2008).
To eliminate the potential contribution of the hydroxyapatite shell, NPs were decalcified. However, variability in vascular remodeling was still evident. Thus, the response to injury observed in NP-inoculated animals does not appear to be mediated only by the calcium phosphate shell and/or nonspecifically associated proteins.
These experiments have several shortcomings. First, the number of animals in this pilot study was small. However, there were clear differences in responses of animals injected with saline compared with those injected with NPs and the intimal hyperplasia in saline-injected rabbits was comparable with that seen in other studies of endothelial damage in rabbits (CitationOrlandi et al 2002), rats (CitationChen and Mehta 1996), and pigs (CitationShimokawa et al 1989). Second, the inoculant was not standardized beyond using material initially derived from the same number of culture flasks. This method does not take into account differences in the quality of the cultures or number of viable NP colonies in each flask. Differences in NP preparations could account for some of the variability in responses among animals. In the future, methods to standardize inoculants need to be developed including evaluating responses to free floating, “planktonic” NPs rather than those isolated from established biofilm. Furthermore, complete removal of the hydroxyapatite shell from the decalcified NPs could not be verified using the current process. Therefore, reliable methods of isolating or producing uncalcified NPs must be developed. Finally, it is not known whether inoculated animals developed an immune-type response to the NPs that would be comparable to that of animals exposed to other infectious agents (eg, Chlamydia pneumonia cytomegalovirus) implicated in arterial disease (CitationMattila et al 1993; CitationMuhlestein 1998; CitationEpstein et al 2000; CitationBurnett et al 2001; CitationMuhlestein and Anderson 2003). Future experiments will need to explore the immunological responses evoked by NPs.
In spite of these short comings, results of this study provide exciting proof-of-principle that human-derived, self-replicating, self-calcifying NPs accelerate vascular wound healing and may lead to pathological calcification. Although the exact chemical, physical and biological nature of these human-derived NPs remains to be elucidated (CitationMartin et al 1965; CitationMiller et al 2004; CitationBenzerara et al 2006), these results support the need for ongoing investigation of the medical and pathophysiological consequences of NPs in humans. Regardless of whether or not NPs represent a pleiotropic form of bacteria or biofilm (CitationDomingue and Schlegel 1977; CitationAkerman et al 1993; CitationDomingue and Woody 1997; CitationCosterton et al 1999; CitationCisar et al 2000; CitationVali et al 2001), the cumulative evidence from this and other studies (CitationShiekh et al 2006; CitationWang et al 2006) suggest that human-derived NPs participate in disease processes. Even if NPs are ultimately found not to be a life form in the classic sense, these experiments suggest a novel mechanism of disease propagation, namely that endogenous or exogenously derived calcified NPs can deliver pathogenic factors to a diseased segment of vessel and stimulate atherogenesis. Determining when and how humans may be exposed to these particles and the inter-individual variability in response to such an exposure could signify a paradigm shift in the diagnosis and treatment of some diseases, including arterial calcification.
Acknowledgements
This work was funded by the Mayo Foundation, Wilson Foundation, and NIH grant DK 60201. The authors report no conflicts of interest.
References
- AkermanKKJuronenIKajanderEOScanning electron microscopy of nanobacteria – novel biofilm producing organisms in bloodScanning Electron Microscopy199315sIII9091
- BenzeraraKMillerVMFarellGSearch for microbial signatures within human and microbial calcifications using soft-X-ray spectromicroscopyJ Invest Med20065436779
- BjorklundMCiftciogluNKajanderEOExtraordinary survival of nanobacteria under extreme conditionsSPIE199834411239
- BurnettMSGaydosCAMadicoGEAtherosclerosis in apoE knockout mice infected with multiple pathogensJ Infect Dis20011832263111120928
- ChenLYMehtaJLFurther evidence of the presence of constitutive and inducible nitric oxide synthase isoforms in human plateletsJ Cardiovasc Pharmacol1996271548656650
- CiftciogluNHaddadRWGoldenCDA potential cause for kidney stone formation during space flights: Enhanced growth of nanobacteria in microgravityKidney Int2005674839115673296
- CiftciogluNMcKayDSKajanderEOAssociation between nanobacteria and periodontal diseasesCirculation2003108e5812939248
- CiftciogluNMiller-HjelleMAHjelleJTInhibition of nanobacteria by antimicrobial drugs as measured by a modified microdilution methodAntimicrob Agents Chemother20024620778612069958
- CisarJOXuD-QThompsonJAn alternative interpretation of nanobacteria-induced biomineralizationProc Natl Acad Sci USA200097115111511027350
- CostertonJWStewartPSGreenbergEPBacterial biofilms: a common cause of persistent infectionsScience199928413182210334980
- DevaAChangLBacterial biofilms: A cause for accelerated capsular contracture?Aesth Surg J1999191303
- DomingueGJSchlegelJUNovel bacterial structures in human blood: Cultural isolationInfect Immun1977156217844907
- DomingueGJSrWoodyHBBacterial persistence and expression of diseaseClin Microbiol Rev199710320449105757
- EpsteinSEZhuJBurnettMSInfection and atherosclerosis: potential roles of pathogen burden and molecular mimicryArtevioscler Thromb Vasc Biol200020141720
- KajanderEOKuronenIAkermanKKNanobacteria from blood, the smallest culturable autonomously replicating agent on EarthSPIE199731114208
- KumarVFarellGYuSCell biology of pathologic renal calcification: contribution of crystal transcytosis, cell-mediated calcification, and nanoparticlesJ Investig Med20065441224
- MartinDSCassisiNJPickensJLEndotoxin shock: a collective reviewRev Surg19652231195318544
- MattilaKJValleMSNieminenMSDVE-IJN-0000-0801-2473SCH-005Dental infections and coronary atherosclerosisAtherosclerosis1993103205118292096
- MeissnerYPellequerYLamprechtANanoparticles in inflammatory bowel disease: Particle targeting versus pH-sensitive deliveryInt J Pharm20063161384316675176
- MillerVMRodgersGCharlesworthJAEvidence of nanobacterial-like structures in human calcified arteries and cardiac valvesAm J Physiol: Heart Circ Physiol2004287H1115H112415142839
- MompeoBTscheuschilsurenGAustGEstrogen receptor expression and synthesis in the human internal thoracic arteryAnn Anat2003185576512597128
- MuhlesteinJBBacterial infections and atherosclerosisJ Investig Med199846396402
- MuhlesteinJBAndersonJLChronic infection and coronary artery diseaseCardiol Clin2003213336214621450
- NadraIBoccacciniARPhilippidisPEffect of particle size on hydroxyapatite crystal-induced tumor necrosis factor alpha secretion by macrophagesAtherosclerosis20081969810517350022
- OrlandiAMarcelliniMPesceDPropionyl-L-carnitine reduces intimal hyperplasia after injury in normocholesterolemic rabbit carotid artery by modulating proliferation and caspase 3-dependent apoptosis of vascular smooth muscle cellsAtherosclerosis200216081911755925
- SedivyRBattistuttiWBNanobacteria promote crystallization of psammoma bodies in ovarian cancerAPMIS2003111951414616547
- ShiekhFAKhullarMSinghSKLithogenesis: Induction of renal calcifications by nanobacteriaUrol Res20063453716425019
- ShimokawaHFlavahanNAVanhouttePMNatural course of the impairment of endothelium-dependent relaxations after balloon endothelium removal in porcine coronary arteriesCirc Res198965740532504508
- ShoskesDAThomasKDGomezEAnti-nanobacterial therapy for men with chronic prostatitis/chronic pelvic pain syndrome and prostatic stones: Preliminary experienceJ Urol2005173474715643213
- StatonCABrownNJRodgersGRAlphastatin, a 24-amino acid fragment of human fibrinogen, is a potent new inhibitor of activated endothelial cells in vitro and in vivoBlood2004103601614512300
- ValiHMcKeeMDCifticiogluNNanoforms: A new type of protein-associated mineralizationGeochimica et Cosmochimica Acta2001656374
- WangLShenWWenJAn animal model of black pigment gallstones caused by nanobacteriaDigestive Dis Sci200651112632