Abstract
The presence of inflammation decreases local anesthetic efficacy, especially in dental anesthesia. Although inflammatory acidosis is most frequently cited as the cause of such clinical phenomena, this has not been experimentally proved. We verified the acidosis mechanism by studying the drug and membrane lipid interaction under acidic conditions together with proposing an alternative hypothesis. Liposomes and nerve cell model membranes consisting of phospholipids and cholesterol were treated at different pH with lidocaine, prilocaine and bupivacaine (0.05%–0.2%, w/v). Their membrane-interactive potencies were compared by the induced-changes in membrane fluidity. Local anesthetics fluidized phosphatidylcholine membranes with the potency being significantly lower at pH 6.4 than at pH 7.4 (p < 0.01), supporting the acidosis theory. However, they greatly fluidized nerve cell model membranes even at pH 6.4 corresponding to inflamed tissues, challenging the conventional mechanism. Local anesthetics acted on phosphatidylserine liposomes, as well as nerve cell model membranes, at pH 6.4 with almost the same potency as that at pH 7.4, but not on phosphatidylcholine, phosphatidylethanolamine and sphingomyelin liposomes. Since the positively charged anesthetic molecules are able to interact with nerve cell membranes by ion-paring with anionic components like phosphatidylserine, tissue acidosis is not essentially responsible for the local anesthetic failure associated with inflammation. The effects of local anesthetics on nerve cell model membranes were inhibited by treating with peroxynitrite (50 μM), suggesting that inflammatory cells producing peroxynitrite may affect local anesthesia.
Introduction
Inflammatory diseases alter the pharmacokinetics and pharmacodynamics of various drugs, resulting in their decreased clinical effects and increased adverse effects (CitationSlaviero et al 2003; CitationSattari et al 2003; CitationAitken et al 2006). Such an inflammation-induced alteration is well known especially in dental anesthesia. Local anesthetic failure or difficulty to obtain satisfactory analgesia commonly occurs in the situations of pulpitis and apical periodontitis (CitationLópez and Diago 2006). The anesthetic efficacies of lidocaine and mepivacaine injections are remarkably affected in the teeth with irreversible pulpitis (CitationDunbar et al 1996; CitationReisman et al 1997). The presence of pulpitis has been estimated to cause inferior alveolar nerve block to fail in approximately 30%–45% of cases (CitationCohen et al 1993; CitationPotočnik and Bajrović 1999). Although a variety of mechanistic hypotheses were proposed for explaining the decreased effects of local anesthetics in the presence of inflammation, the most cited is the theory that the acidosis of inflamed tissues reduces the anesthetic potencies of administered drugs (CitationMeechan 1999; CitationBecker and Reed 2006).
Since clinically used local anesthetics are structurally the tertiary amines with aromatic rings, they exist as charged and uncharged molecules with the relative amounts depending on their medium pH and pKa values. While both species are relevant to pharmacological activities, local anesthetics diffuse in uncharged form through nerve sheaths and penetrate into cell membranes to reach the cytoplasmic binding sites or receptors on transmembrane channels. In addition to the blockade of voltage-gated sodium channels, the mode of action of anesthesia includes the drug and membrane lipid interaction which induces the changes in membrane physicochemical property, fluidization or disordering (CitationJastak et al 1995; CitationFrangopol and Mihǎilescu 2001). These pharmacological features, together with a correlation between anesthetic potency and lipid solubility (CitationCovino 1986), strongly suggest that the drugs interact with membrane lipid bilayers to induce local anesthesia. Lactic acid and acidic by-products are increasingly produced and concentrated in and near inflamed tissues, causing the acidosis which lowers the tissue pH at least the order of 0.5–1.0 pH unit (CitationPunnia-Moorthy 1987; Citationde Backer 2003). The pKa values of almost local anesthetics in clinical use are larger than 7.5 (CitationJastak et al 1995). According to the Henderson-Hasselbalch equation (Log10 [uncharged drug]/[charged drug] = pH − pKa), a greater proportion of administered drugs should be the charged molecules with much less membrane interactivity and permeability under acidic conditions corresponding to inflamed tissues. Therefore, local anesthetic effects would be decreased in the presence of inflammation, leading to the hypothetic mechanism based on tissue acidosis. This hypothesis seems to be so theoretical and understandable that it has been conventionally accepted despite being not experimentally confirmed.
The hydrophobic interaction underlies the effects of local anesthetics on lipid bilayers (CitationOhki and Ohshima 1996; CitationFrangopol and Mihǎilescu 2001; CitationTsuchiya et al 2005). However, the drug action on biomembranes is not identical to that speculated from the organic: aqueous phase partition. Unlike experimental membrane specimens with the simple lipid composition, nerve cell membranes consist of different phospholipid and sterol components (CitationSvennerholm et al 1992). Their compositional variations greatly affect the membrane interactivities of drugs (CitationTsuchiya et al 2007).
Therefore, we attempted to verify the conventional acidosis mechanism for the local anesthetic failure associated with inflammation. We comparatively studied the interactions of local anesthetics with membrane lipids by lowering the reaction pH and varying the membrane lipid composition. Of particular interest were whether local anesthetics could change membrane fluidity at acidic pH by interacting with lipid bilayers and whether any of lipid components were specifically responsible for the possible membrane interaction. For this purpose, we used the liposome system in which reaction conditions and membrane lipids were readily manipulated to examine the influence of pH and the importance of individual lipids (CitationChatterjee and Agarwal 1988). Based on the verification results, we propose an alternative hypothesis which was speculated from the potential reactivity of peroxynitrite pathologically relevant to inflammation.
Materials and methods
Reagents
Lidocaine, prilocaine and bupivacaine, all in hydrochloride salt form, were purchased from Sigma (St. Louis, MO, USA), and 1,2-dipalmitoylphosphatidylcholine (DPPC), 1-palmitoyl-2-oleoylphosphatidylcholine (POPC), 1-palmitoyl-2-oleoylphosphatidylethanolamine (POPE), 1-palmitoyl-2-oleoylphosphatidylserine (POPS) and sphingomyelin (SM) from Avanti Polar Lipids (Alabaster, AL, USA). Cholesterol, 1,6-diphenyl-1,3,5-hexatriene (DPH) and peroxynitrite were obtained from Wako Pure Chemicals (Osaka, Japan), Molecular Probes (Eugene, OR, USA) and Dojindo (Kumamoto, Japan), respectively. The concentration of peroxynitrite was spectrophotometrically determined according to manufacturer’s directions, and then diluted to be 10 mM with 0.1 M NaOH. The prepared peroxynitrite solutions were stored at −80 ºC and consumed within three weeks after preparation. Water of liquid chromatographic grade (Kishida, Osaka, Japan) and dimethyl sulfoxide of spectroscopic grade (Kishida) were used for preparing reagent solutions. All other chemicals were of the highest grade available commercially.
Liposome preparation
DPH-labeled liposomes of the lipid bilayer structure (total lipids of 0.14 mM) were prepared by hydrating the lipid dry film with 20 mM sodium phosphate buffer of pH 7.4 and 6.4 (Wako Pure Chemicals) containing 100 mM KCl as reported previously (CitationTsuchiya et al 2005). The molar ratio of DPH to membrane lipids was adjusted to be 1 : 200. The lipid composition of liposomal membranes was as follows: DPPC (100 mol%), POPC and cholesterol (55 : 45, mol%), POPE and cholesterol (55 : 45, mol%), POPS and cholesterol (55 : 45, mol%), and SM and cholesterol (55 : 45, mol%). Nerve cell model membranes were prepared with POPC, POPE, POPS, SM and cholesterol (11 : 16.5 : 11 : 16.5 : 45, mol%) according to the composition of major membrane lipids of peripheral nerve cells (CitationSvennerholm et al 1992).
Membrane lipid interaction
Local anesthetics dissolved in dimethyl sulfoxide (20 μL) were added to the liposome preparations (3.98 mL) of pH 7.4 and 6.4 so that a final concentration was 0.2% (w/v) for lidocaine, 0.2% (w/v) for prilocaine and 0.05% (w/v) for bupivacaine. The concentration of dimethyl sulfoxide was adjusted to be less than 0.5% (v/v) of the total volume so as not to influence the membrane fluidity of liposomes. After reaction for 15 min at 37 ºC, DPH fluorescence polarization was measured by an RF-540 spectrofluorometer (Shimadzu, Kyoto, Japan) equipped with a polarizer and a cuvette thermo-controller as reported previously (CitationTsuchiya 2001). Compared with control values (dimethyl sulfoxide vehicle added), the decrease of DPH polarization means the increase of fluidity (membrane fluidization) in the hydrocarbon cores of lipid bilayers, reflecting the acting site of tested drugs (CitationMizogami et al 2002). The potencies to interact with liposomal membranes were compared on the basis of the changing degree of polarization values relative to controls.
Peroxynitrite treatment
Local anesthetics dissolved in dimethyl sulfoxide (20 μL) were added to the nerve cell model membrane preparations (3.93 mL) of pH 7.4 and 6.4 to be a final concentration of 0.2% (w/v) for lidocaine, 0.2% (w/v) for prilocaine and 0.05% (w/v) for bupivacaine. Immediately after that, peroxynitrite in 0.1 M NaOH (50 μL) was added to be 50 μM with vortex-mixing for 5 sec, followed by reaction for 15 min at 37 ºC to induce the membrane effects of local anesthetics. The corresponding volume of 0.1 M NaOH vehicle was added to controls. Thereafter, DPH polarization was measured as described above. The influence of peroxynitrite was evaluated by comparing the membrane-fluidizing effects of local anesthetics treated with and without peroxynitrite.
Statistical analysis
Results are expressed as mean ± SE (N = 6–8). Data were statistically analyzed by Student’s t-test (StatView 5.0; SAS Institute, Cary, NC, USA). A P value <0.05 was considered significant.
Results
Lidocaine, prilocaine and bupivacaine acted on DPPC liposomal membranes at pH 7.4 to increase their fluidity as shown by DPH polarization decreases (). At pH 6.4, however, their membrane-fluidizing effects were significantly weakened. Compared with pH 7.4, the decreasing values of polarization were reduced to 30.8 ± 1.2% for lidocaine, 32.5 ± 0.9% for prilocaine and 32.5 ± 0.5% for bupivacaine.
Figure 1 Effects of local anesthetics on DPPC liposomal membranes at different pH. Lidocaine (0.2%, w/v), prilocaine (0.2%, w/v) and bupivacaine (0.05%, w/v) were reacted with DPPC liposomes for 15 min at 37 ºC at pH 7.4 and 6.4, followed by DPH fluorescence polarization measurements. Each result represents mean ± SE (N = 6–8). **p <0.01, compared with pH 7.4.
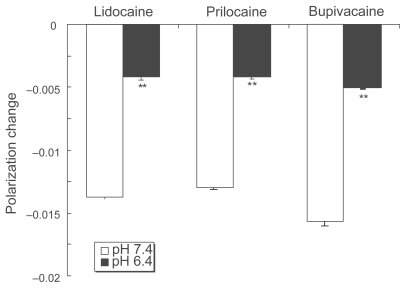
Even if the pH was lowered from 7.4 to 6.4, local anesthetics more greatly fluidized nerve cell model membranes compared with DPPC liposomal membranes (). While the polarization decreasing values at pH 6.4 relative to pH 7.4 were 69.6 ± 2.1% for lidocaine, 70.5 ± 1.9% for prilocaine and 71.8 ± 2.9% for bupivacaine, their potencies to interact with nerve cell model membranes at pH 6.4 were 2.2–2.3 times those with DPPC liposomal membranes.
Figure 2 Effects of local anesthetics on nerve cell membranes at different pH. Lidocaine (0.2%, w/v), prilocaine (0.2%, w/v) and bupivacaine (0.05%, w/v) were reacted with nerve cell model membranes for 15 min at 37 ºC at pH 7.4 and 6.4, followed by DPH fluorescence polarization measurements. Each result represents mean ± SE (N = 6–8). **p <0.01, compared with pH 7.4.
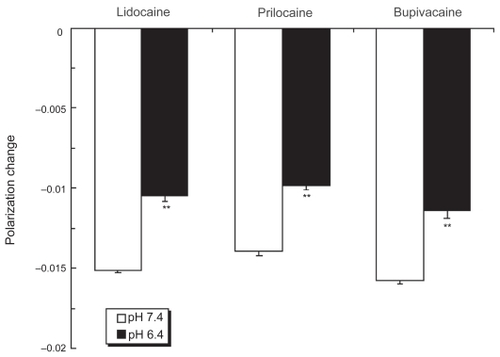
The effects of pH lowering remarkably depended on the kind of membrane component phospholipids. The membrane-interactive potencies of lidocaine, prilocaine and bupivacaine were greatly diminished at pH 6.4 in POPC, POPE and SM liposomal membranes (). However, all the tested anesthetics fluidized POPS liposomal membranes at pH 6.4 with almost the same potency as that at pH 7.4.
Figure 3 Effects of local anesthetics on liposomal membranes consisting of different phospholipids. Lidocaine (0.2%, w/v), prilocaine (0.2%, w/v) and bupivacaine (0.05%, w/v) were reacted with POPC, POPE, SM or POPS liposomes for 15 min at 37 ºC at pH 7.4 and 6.4, followed by DPH fluorescence polarization measurements. Each result represents mean ± SE (N = 6–8). **p <0.01, compared with pH 7.4.
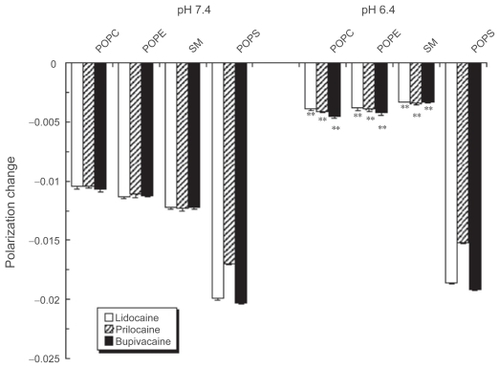
The relative potencies of local anesthetics to interact with liposomal membranes at pH 6.4 to pH 7.4 are shown in , together with the relative concentrations of uncharged molecules which were calculated by the Henderson-Hasselbalch equation using the pKa values of 7.9 for lidocaine, 7.8 for prilocaine and 8.1 for bupivacaine (CitationCourtney 1980).
Table 1 Relative concentrations of uncharged anesthetic molecules calculated by the Henderson-Hasselbalch equation and relative potencies to interact with different liposomal membranes (mean ± SE, N = 6–8)
The fluidizing effects of lidocaine, prilocaine and bupivacaine on nerve cell model membranes were inhibited by treating with peroxynitrite (). The inhibition occurred at both pH 7.4 and pH 6.4, which showed no differences in inhibition potency.
Figure 4 Influence of peroxynitrite on local anesthetic membrane effects. Lidocaine (0.2%, w/v), prilocaine (0.2%, w/v) and bupivacaine (0.05%, w/v) were treated with 50 μM peroxynitrite for 5 sec at pH 7.4 and 6.4. Their effects on nerve cell model membranes were determined after reaction for 15 min at 37 ºC by measuring DPH fluorescence polarization. Each result represents mean ± SE (N = 6–8). **p < 0.01, compared with control (not treated with peroxynitrite).
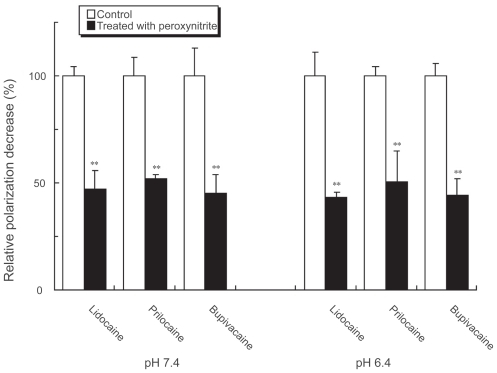
Discussion
Representative anesthetics lidocaine, prilocaine and bupivacaine were subjected to the membrane interactions at clinically used concentrations (CitationJastak et al 1995). All of them fluidized DPPC liposomal membranes in 15 min, being consistent with their onset time (CitationUckan et al 1998; CitationSun et al 1999). However, their membrane-interactive potencies were significantly decreased at pH 6.4 which is almost comparable to inflamed tissue conditions (CitationPunnia-Moorthy 1987). Such pH-dependence may provide experimental support for the conventional mechanism based on tissue acidosis.
Local anesthetics must traverse ensheathing perineuria which are hydrophobic (lipoid) barriers against the drugs to reach nerve fibers. They also must penetrate into or across neuronal cell membranes consisting of lipid bilayers to exert their pharmacological activities. When blocking voltage-gated sodium channels, local anesthetics also permeate cell membranes to bind to the receptors. Inflammation causes metabolic acidosis which lowers the pH of affected tissues (CitationPunnia-Moorthy 1987; Citationde Backer 2003). Such inflammatory conditions would alter the pharmacokinetics and pharmacodynamics of local anesthetics by reducing their interactivities with lipid bilayers and/or membrane lipids.
There is a systematic relationship between anesthetic potency and hydrophobicity to determine the membrane interactivity (CitationCourtney 1980). The hydrophobicity of local anesthetics is characterized by their partition coefficients, usually in an octanol:buffer system (CitationStrichartz et al 1990). While tertiary amine local anesthetics exist in uncharged (free base) or charged form depending on their pKa values and medium pH, the uncharged molecules are much more membrane-interactive than the charged ones. As shown in , the relative potencies to interact with DPPC liposomal membranes decrease in all the tested drugs by lowering the pH from 7.4 to 6.4, which is correlated to the decreasing relative concentrations of uncharged molecules.
The effects of lidocaine, prilocaine and bupivacaine on nerve cell model membranes at pH 6.4 were much greater than presumed from their relative concentrations of uncharged molecules (). Although the rank order is different between relative potency and concentration, this is interpretable by the difference in hydrophobicity of local anesthetics. The hydrophobic interaction with phosphatidylcholine membranes is estimated to be prilocaine < lidocaine < bupivacaine in increasing order of intensity based on their chromatographic behaviors in the reversed-phase partition system (CitationTsuchiya et al 2005). The partition coefficients are greatest in the order of prilocaine < lidocaine << bupivacaine for both uncharged and charged molecules (CitationStrichartz et al 1990). The results of nerve cell model membranes never support the acidosis mechanism, indicating that the tissue acidification is not essentially responsible for the decreased effects of local anesthetics in the presence of inflammation.
Unlike DPPC liposomal membranes consisting of phosphatidylcholine alone, nerve cell model membranes were prepared with different phospholipids (POPC, POPE, POPS and SM) and cholesterol to resemble peripheral nerve cells (CitationSvennerholm et al 1992). Such lipid composition may explain why local anesthetics are able to interact with membrane lipids under inflammatory acidic conditions. As well as DPPC liposomes, liposomal membranes consisting of POPC, POPE and SM showed much less fluidization at pH 6.4 compared with pH 7.4. However, only POPS liposomal membranes were significantly fluidized even at pH 6.4 by lidocaine, prilocaine and bupivacaine despite that their charged molecules predominated. With respect to structural difference of these phospholipids, DPPC, POPC, POPE and SM are zwitterionic, whereas POPS is acidic. Positively charged (cationic) drugs show much higher affinity to acidic (anionic) phospholipids like POPS compared with zwitterionic ones (Citationde Wolf et al 1990; CitationTsuchiya et al 2007). Not only uncharged but also charged anesthetics are able to interact with lipid bilayers, the former deeply in the hydrophobic core, but the latter in the head-group regions of phospholipids (CitationPasenkiewicz-Gierula et al 2003). Local anesthetics in charged form electrostatically interact with the negatively charged sites in membranes at pH lower than the pKa values and their adsorbed amounts depend on the membrane surface charge (CitationOhki and Ohshima 1996). In this study, local anesthetics with pKa over 7.8 showed less fluidizing effects on DPPC, POPC, POPE and SM liposomal membranes at pH 6.4 as the uncharged molecules decreased. On the other hand, their effects on POPS liposomal membranes were significantly great even at pH 6.4, contradicting to the relative decrease of uncharged molecules, but correlating to the relative increase of charged molecules. As known in certain amphiphilic drugs (CitationŠtolc et al 1989), the cationic moieties of local anesthetics possibly pair with the anionic head-groups of acidic phospholipids to form the seemingly uncharged ion-pairs, which could penetrate into lipid bilayers and modify membrane fluidity. Acidic phosphatidylserine is referred to as a counter-ion to pair with positively charged anesthetics.
Local anesthetics have been verified to interact with nerve cell membranes at the pH corresponding to inflammatory acidosis. Tissues can buffer the excess acidity (CitationWennberg et al 1982) and such buffering is more potent in inflamed tissues (CitationPunnia-Moorthy 1988). These pathophysiological features are also unfavorable to the conventional mechanism based on tissue acidosis, requiring an alternative theory for the local anesthetic failure associated with inflammation.
Inflammatory cells produce peroxynitrite (CitationRódenas et al 1995), which has been implicated in the pathogenesis of various diseases including inflammation (CitationDemiryürek et al 1998). Inflamed tissues are characterized by the production of nitric oxide and superoxide anion which react to form peroxynitrite. Inflammatory diseases influence the effects of different drugs due to the formation of nitric oxide (CitationMayo et al 2000; CitationKulmatycki et al 2001). Several local anesthetics, including lidocaine and bupivacaine, react with peroxynitrite (CitationGunaydin and Demiryurek 2001; CitationGünaydin and Demiryürek 2003). Peroxynitrite is also able to inhibit Na+/K+-ATPase activity through the modification of membrane fluidity (CitationMuriel and Sandoval 2000). Therefore, our hypothesis is that inflammatory peroxynitrite may interact with local anesthetics to affect their membrane interactivities.
In the membrane interaction experiments, peroxynitrite has been revealed to inhibit the anesthetic-induced fluidization of nerve cell model membranes. The reactivity of peroxynitrite is greater at acidic to neutral pH than at alkaline pH (CitationRadi et al 1991). While local anesthetics were reacted with 50 μM peroxynitrite for 5 sec at pH 7.4 and 6.4, these experimental conditions are not in conflict with the peroxynitrite exposure by activated inflammatory cells (CitationIschiropoulos et al 1992).
Since venules and capillaries are dilated in inflamed tissues, the increased blood flow due to vasodilatation would promote the absorption and removal of local anesthetics from their administered sites (CitationPunnia-Moorthy 1988; CitationJastak et al 1995). The presence of inflammation modifies the activities of sensory nerves and ion channels to possibly induce afferent hyperexcitability and hyperalgesia (CitationFlake and Gold 2005; CitationWang et al 2007). In addition to these pathophysiological changes, it is hypothesized that inflammatory peroxynitrite could participate in the decrease of local anesthetic effects.
In conclusion, inflammatory acidosis is not as important for the decreased efficacy of local anesthesia as recognized conventionally. As a novel mechanistic hypothesis, peroxynitrite relevant to inflammation may interact with local anesthetics to modify their pharmacological activities.
Acknowledgments
This study was partly supported by a Grant-in-Aid for Scientific Research 20592381 (to H.T.) from the Japan Society for the Promotion of Science.
Disclosures
None of the authors disclose conflicts of interest.
References
- AitkenAERichardsonTAMorganET2006Regulation of drug-metabolizing enzymes and transporters in inflammationAnnu Rev Pharmacol Toxicol461234916402901
- BeckerDEReedKL2006Essentials of local anesthetic pharmacologyAnesth Prog539810917175824
- ChatterjeeSNAgarwalS1988Liposomes as membrane model for study of lipid peroxidationFree Radic Biol Med451722830175
- CohenHPChaBYSpångbergLS1993Endodontic anesthesia in mandibular molars: a clinical studyJ Endod1937038245762
- CourtneyKR1980Structure-activity relations for frequency-dependent sodium channel block in nerve by local anestheticsJ Pharmacol Exp Ther21311496965720
- CovinoBG1986Pharmacology of local anaesthetic agentsBr J Anaesth58701162425835
- de BackerD2003Lactic acidosisMinerva Anestesiol69281412766720
- de WolfFAMaliepaardMvan DorstenF1990Comparable interaction of doxorubicin with various acidic phospholipids results in changes of lipid order and dynamicsBiochim Biophys Acta109667802268686
- DemiryürekATCakiciIKanzikI1998Peroxynitrite: a putative cytotoxinPharmacol Toxicol8211379553987
- DunbarDReaderANistR1996Anesthetic efficacy of the intraosseous injection after an inferior alveolar nerve blockJ Endod2248169198431
- FlakeNMGoldMS2005Inflammation alters sodium currents and excitability of temporomandibular joint afferentsNeurosci Lett384294915908117
- FrangopolPTMihǎilescuD2001Interactions of some local anesthetics and alcohols with membranesColloids Surf B Biointerfaces2232211438236
- GunaydinBDemiryurekAT2001Interaction of lidocaine with reactive oxygen and nitrogen speciesEur J Anaesthesiol188162211737181
- GünaydinBDemiryürekAT2003Effects of tetracaine and bupivacaine on chemiluminescence generated by formyl-methionyl-leucyl-phenylalanine-stimulated human leukocytes and cell-free systemsInt Immunopharmacol37576412757744
- IschiropoulosHZhuLBeckmanJS1992Peroxynitrite formation from macrophage-derived nitric oxideArch Biochem Biophys298446511329657
- JastakJTYagielaJADonaldsonD1995Local Anesthesia of the Oral CavityPhiladelphiaWB Saunders
- KulmatyckiKMAbouchehadeKSattariS2001Drug-disease interactions: reduced β-adrenergic and potassium channel antagonist activities of sotalol in the presence of acute and chronic inflammatory conditions in the ratBr J Pharmacol1332869411350865
- LópezABDiagoMP2006Failure of locoregional anesthesia in dental practice. Review of the literatureMed Oral Patol Oral Cir Bucal11E510317072256
- MayoPRSkeithKRussellAS2000Decreased dromotropic response to verapamil despite pronounced increased drug concentration in rheumatoid arthritisBr J Clin Pharmacol506051311136300
- MeechanJG1999How to overcome failed local anaesthesiaBr Dent J186152010028738
- MizogamiMTsuchiyaHHaradaJ2002Membrane effects of ropivacaine compared with those of bupivacaine and mepivacaineFundam Clin Pharmacol163253012570022
- MurielPSandovalG2000Nitric oxide and peroxynitrite anion modulate liver plasma membrane fluidity and Na+/K+-ATPase activityNitric Oxide43334210944417
- OhkiSOhshimaH1996Distribution of local anesthetics in lipid membranesColloids Surf B Biointerfaces5291305
- Pasenkiewicz-GierulaMRógTGrochowskiJ2003Effects of a carane derivative local anesthetic on a phospholipid bilayer studied by molecular dynamics simulationBiophys J8512485812885668
- PotočnikIBajrovićF1999Failure of inferior alveolar nerve block in endodonticsEndod Dent Traumatol152475110825834
- Punnia-MoorthyA1987Evaluation of pH changes in inflammation of the subcutaneous air pouch lining in the rat, induced by carrageenan, dextran and Staphylococcus aureusJ Oral Pathol1636442435877
- Punnia-MoorthyA1988Buffering capacity of normal and inflamed tissues following the injection of local anaesthetic solutionsBr J Anaesth6115493415887
- RadiRBeckmanJSBushKM1991Peroxynitrite-induced membrane lipid peroxydation: the cytotoxic potential of superoxide and nitric oxideArch Biochem Biophys28848171654835
- ReismanDReaderANistR1997Anesthetic efficacy of the supplemental intraosseous injection of 3% mepivacaine in irreversible pulpitisOral Surg Oral Med Oral Pathol8467682
- RódenasJMitjavilaMTCarbonellT1995Simultaneous generation of nitric oxide and superoxide by inflammatory cells in ratsFree Radic Biol Med18869757797094
- SattariSDrydenWFEliotLA2003Despite increased plasma concentration, inflammation reduces potency of calcium channel antagonists due to lower binding to the rat heartBr J Pharmacol1399455412839868
- SlavieroKAClarkeSJRivoryLP2003Inflammatory response: an unrecognised source of variability in the pharmacokinetics and pharmacodynamics of cancer chemotherapyLancet Oncol42243212681266
- ŠtolcSNemčekVSzöcsováH1989Local anesthetics: lipophilicity, charge, diffusion and site of action in isolated neuronEur J Pharmacol164249562547635
- StrichartzGRSanchezVArthurGR1990Fundamental properties of local anesthetics. II. Measured octanol:buffer partition coefficients and pKa values of clinically used drugsAnesth Analg71158702375517
- SunRHamiltonRCGimbelHV1999Comparison of 4 topical anesthetic agents for effect and corneal toxicity in rabbitsJ Cataract Refract Surg251232610476507
- SvennerholmLBoströmKFredmanP1992Membrane lipids of human peripheral nerve and spinal cordBiochim Biophys Acta1128171390872
- TsuchiyaHMizogamiMTakakuraK2005Reversed-phase liquid chromatographic retention and membrane activity relationships of local anestheticsJ Chromatogr A1073303815909534
- TsuchiyaHMizogamiMUenoT2007Interaction of local anaesthetics with lipid membranes under inflammatory acidic conditionsInflammopharmacology151647017701019
- TsuchiyaH2001Structure-specific membrane-fluidizing effect of propofolClin Exp Pharmacol Physiol28292911251643
- UckanSGulerNSumerM1998Local anesthetic efficacy for oral surgery: Comparison of diphenhydramine and prilocaineOral Surg Oral Med Oral Pathol Oral Radiol Endod8626309690241
- WangJ-GStrongJAXieW2007Local inflammation in rat dorsal root ganglion alters excitability and ion currents in small diameter sensory neuronsAnesthesiology1073223217667578
- WennbergEHaljamäeHEdwallG1982Effects of commercial (pH ~ 3.5) and freshly prepared (pH ~ 6.5) lidocaine-adrenaline solutions on tissue pHActa Anaesth Scand2652477148369