Abstract
Purpose:
Interleukin-1β (IL-1β) and S100B calcium binding protein B (S100B) have been implicated in the pathogenesis of Alzheimer’s disease. Both are present in and around senile plaques and have been shown to increase levels of amyloid precursor protein (APP) mRNA in vitro. However, it is not known how either of these substances affects APP in vivo.
Methods:
We have studied the effects of IL-1β and S100B on the expression and processing of APP using a retinal-vitreal model. We have also investigated the effect of amyloid beta peptide (Aβ) on APP in the same system and the regulation of S100B production by Aβ and IL-1β from retinal glial cells.
Results:
Retinal ganglion cells constitutively express APP. However, after intravitreal injection of IL-1β or Aβ there was a marked reduction in APP levels as detected by Western blotting and IL-1β produced a decrease in APP immunoreactivity (IR). Nissl staining showed that the integrity of the injected retinas was unchanged after injection. Two days after S100B injection, there was a small reduction in APP-IR but this was accompanied by the appearance of some intensely stained large ganglion cells and there was some up-regulation in APP holoprotein levels on Western blot. Seven days post-S100B injection, these large, highly stained cells had increased in number throughout the retina. Injection of Aβ and IL-1β also caused an increase in S100B production within the retinal Müller glial cells.
Conclusion:
These results support the hypothesis that S100B (a glial-derived neurotrophic factor) and IL-1β (a pro-inflammatory cytokine) can modulate the expression and processing of APP in vivo and so may contribute to the progression of Alzheimer’s disease.
Introduction
The neuropathological hallmarks of Alzheimer’s disease (AD) are senile plaques and neurofibrillary tangles. The plaques are comprised of a variety of peptides, the most abundant of which is amyloid beta peptide (Aβ) and it is thought the abnormal cleavage of amyloid precursor protein (APP) by β- and then γ-secretase yields this pro-aggregatory Aβ peptide (CitationHardy 1997). These plaques are intimately associated with activated microglial cells interdigitating the plaques and astrocytes surrounding the plaques. It has been shown that these cells over-express pro-inflammatory factors such as S100B and interleukin-1 beta (IL-1β) (CitationGriffin et al 1995; CitationMrak et al 1996).
S100B is a small 10 kDa protein belonging to the EF-hand family of calcium binding proteins and is released from astrocytes and Schwann cells (CitationDonato 2001). At lower doses (high picomolar to low nanomolar), S100B displays neurotrophic properties by increasing neuronal survival and promoting neurite outgrowth (CitationKligman et al 1985; CitationBhattacharyya et al 1992). However, at higher doses, S100B stimulates astrocytes and microglia to produce pro-inflammatory cytokines including IL-1β (CitationKim et al 2004). Studies from CitationSheng and colleagues (1996) suggest that early plaques are accompanied by large numbers of astrocytes expressing S100B whilst at later stages there are far fewer S100B-positive astrocytes, implying a role for this factor in early plaque development. This is further supported by evidence showing that in the APP-over expressing mouse, APPV717F mice, increased levels of S100B precede the deposition of Aβ (CitationSheng et al 2000). Whether S100B causes a direct increase in Aβ production or whether it is a secondary increase remains to be determined.
Interleukin-1β (17 kDa) is a pro-inflammatory cytokine having a multitude of different actions. Microglial cells within senile plaques have been shown to increase their production of IL-1β and this expression increases with plaque pathology; finally disappearing at the end stage “burnt-out” plaques (CitationMrak and Griffin 2001). Again, it would appear that IL-1β may be involved in production of Aβ, but whether it is cause or effect is still unclear. IL-1β increases the expression of APP mRNA in cultured cells by acting on its promoter (CitationGoldgaber et al 1989; CitationBuxbaum et al 1992) and may regulate the increase in APP expression after traumatic brain injury (CitationCiallella et al 2002). Accumulation of APP may lead to increased production of Aβ – the central core of amyloid plaques. Increased Aβ could then cause the stimulation of glial cells resulting in further expression of S100B. S100B may stimulate the release of IL-1β from microglia and so a “cytokine cycle” is initiated, perpetuating the damage (CitationGriffin et al 1998). However, the direct actions of S100B and IL-1β on APP production and processing in vivo are unclear. To address this issue, we have used a rat retinal-vitreal model to study the effects of these cytokines on APP expression in vivo. This is a potentially useful model because retinal ganglion cells (RGC) constitutively express APP (CitationMorin et al 1993) and the closed nature of the system means that the injected substances remain in situ rather than being rapidly cleared, giving them the opportunity to act locally. Furthermore, the retina contains most of the nerve cells that express AD-associated molecules, all the major neurotransmitter-producing neurons and most of the glial populations found in the brain (CitationGuo et al 1991, Citation1992a, Citation1992b; CitationYang et al 1992; CitationChen et al 1996; CitationBresciani et al 2002; CitationWalsh et al 2005; CitationWatts et al 2007), thus enabling us to study the interplay of these cytokines within a central nervous system setting.
Materials and methods
All experimental procedures were carried out in accordance with the Animals (Scientific Procedures) Act 1986. Female Sprague-Dawley rats (6–8 weeks old) were anaesthetised with sodium pentobarbital (0.6 ml/100 g) and underwent unilateral intravitreal injection with either S100B (Sigma, UK; 2 and 5 nmol in 3 μl vehicle; n = 6 per dose); rat recombinant IL-1β (Serotec, UK; 150 and 1500U in 3 μl; n = 9 per dose), the higher dose based on a study by CitationBamforth and colleagues (1997); aggregated Aβ1–42 (Bachem, UK; 2 nmol in 3 μl; n = 12) or phosphate-buffered saline (PBS) (n = 6). Aβ1–42 was aggregated by incubation at 37 °C for 4 days in PBS (CitationJen et al 1998; CitationWalsh et al 2002). Before injection, some of the vitreous humour was removed to avoid increased intraocular pressure. At 2 and 7 days after injection, animals were either killed by cervical dislocation and the retinas removed for Western blotting or they were transcardially perfused with saline followed by 4% paraformaldehyde (PFA) and processed for immunohistochemistry (IHC; n = 3 for each treatment). Aβ1–42-injected animals were killed at 2 days.
For Western blotting (n = 3 for each treatment), retinas were rapidly removed and snap frozen in liquid nitrogen. Retinas were homogenised in RIPA buffer (150 mM NaCl, 1% NP-40, 0.5% sodium deoxycholate, 0.1% SDS, in 50 mM Tris, pH 8.0). A cocktail of protease inhibitors (CompleteMini™, Roche) was always added fresh to the lysis buffer to avoid nonspecific digestion of proteins. Retinal homogenates (30 μg/lane) underwent electrophoresis on 4%–12% BisTris gels (NuPAGE System, Invitrogen) and were then blotted onto nitrocellulose membranes. Blots were blocked in 5% dried skimmed milk in PBS/0.1% Tween 20 (blocking buffer) for at least 1 hour prior to incubation with either CT695 anti-APP antibody (Zymed, Laboratories Inc, USA; 1:200 dilution) or anti-S100B antibody (Sigma Chemical Company Ltd, Poole, UK; 1:1000 dilution) overnight at 4 °C. Blots were washed and then incubated for 1 hour with peroxidase-conjugated anti-rabbit antibody (Sigma Chemical Company Ltd, Poole, UK; 1:3000) in blocking buffer. Bound immunoglobulins were detected with the ECL system. Some blots were stripped of the antibodies using ReBlot™ (Chemicon, Harrow, UK) and then re-probed with alpha-actin antibody (Roche, Lewes, UK; 1:2000) to visualize the protein load per lane.
For IHC, eyes were removed, post-fixed in 4% PFA (4 h) and sunk in sucrose overnight. The eyes were then embedded in OCT and frozen in isopentane pre-cooled in liquid nitrogen. Sections were cut at a 20 μm thickness and mounted onto SuperFrost® Plus slides (VWR International). Sections were washed in 0.1 mM PBS/0.3% Triton X-100 (Sigma, UK); in methanol; endogenous peroxidases were blocked with H2O2 and microwaved in EDTA, at 40% power for 4 min. Sections were then blocked in normal serum before addition of the primary antibody overnight, either CT695 anti-APP (1:1000 dilution) or anti-S100B (1:1000 dilution). Sections were washed and incubated with biotinylated secondary antibody (Vector, Peterborough, UK) for 45 minutes followed by further washing and incubation in Vectastain Elite ABC kit for 45 minutes (Vector, Peterborough, UK). Staining was visualized using the diaminobenzidine (DAB) peroxidise substrate kit (Vector, Peterborough, UK). APP-staining also included nickel enhancement. For staining with anti-S100B antibody, the microwave step was omitted. Sections were dehydrated in an increasing alcohol gradient, cleared in xylene and mounted with DPX resin (BDH Chemicals Ltd, Poole, UK).
For Nissl staining to reveal the cytoarchitecture, sections were stained with cresyl fast violet (Raymond A Lamb, East-bourne, UK) for 5 minutes, washed in PBS and dehydrated in an increasing alcohols gradient and mounted with DPX.
Results
APP immunoreactivity is altered by IL-1β and S100B
We have set out to address how APP processing is affected by IL-1β and S100B in projection neurons of the rat retina. In control animals, APP, as detected with a C-terminal directed antibody (CT695, Zymed), is constitutively expressed in the RGC throughout the ganglion cell layer with some staining seen in the axons of these cells. This pattern of staining was unchanged by intravitreal injection of PBS at 2 days (Figure and b) or by injection of the lower dose of IL-1β (150 U/3 μl, not shown) and S100B (2 nmoles/3 μl, not shown). However, 2 days after intravitreal injection with higher dose IL-1β (1500 U/3 μl) there was a marked decrease in immunoreactivity (IR) throughout the ganglion cell layer with loss of staining in the RGC (Figure ). In the retinas injected with a higher dose of S100B (5 nmol/3 μl), there appeared to be a small decrease in the overall staining within the ganglion cell layer but this was accompanied by the appearance of a small number of ganglion cells that showed very strong APP-IR (Figure and h). Seven days after injection with S100B, the number of these intensely stained RGCs appeared to increase (Figure and j). Similar large, heavily stained cells also became apparent 7 days after injection with high dose IL-1β (Figure and f). As the injected Aβ1–42 cross-reacted with the CT695 antibody, we were unable to ascertain the changes in APP-IR after injection of the peptide. Since we only noted changes with the higher doses of cytokines tested, we continued to use these doses for further studies.
Figure 1 Photomicrographs showing APP immunoreactivity in rat retinas 2 days post-PBS injection at low (a) and high (b) magnification. Note staining is seen in the GCL with some axonal staining. Low magnification photomicrograph showing that APP immunoreactivity is decreased in retinas injected with IL-1β after 2 days (c) compared with low magnification micrograph the retina from the corresponding noninjected eye (d). Low (e) and high (f) magnifications of retinas 7 days post-IL-1β injection show recovery of APP staining of RGCs. Low and high magnifications of retinas 2 days post-S100B injection show sporadic RGCs strongly stained for APP. Low (i) and high (j) magnifications of retina 7 days post-S100B injection indicating an increase in RGC immunoreactivity.
Abbreviations: APP, amyloid precursor protein; GCL, granule cell layer; IL, interleukin; RGC, retinal ganglion cells; PBS, phosphate-buffer solution.
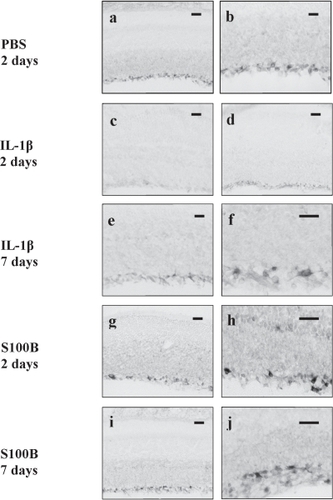
To ensure that our injection procedures were not depleting cells within the granule cell layer (GCL), we looked at Nissl staining of sections injected with PBS, IL-1β, and S100B. The staining revealed that there was no change in the cytoarchitecture of the retinas after injection with S100B and we could not detect any pyknotic bodies. After injection with IL-1β, there was the appearance of a cellular infiltrate in some of the injected animals but the cells within the GCL appeared healthy with no sign of pyknotic bodies (Figure ).
Figure 2 Nissl staining of (a) normal retina, (b) retina 2 days after injection with S100B and (c) retina 2 days after injection with IL-1β. Note that the tissue architecture is not affected by the injection and that the GCL layer appears intact without loss of the large ganglion cells.
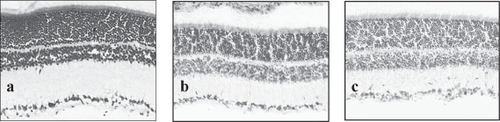
Differential effects of IL-1β, S100B, and Aβ1–42 on APP processing
We have used IHC to look at the cellular profile of APP changes but this does not allow us to study its processing. We therefore used Western blotting to investigate how each cytokine affected the processing of APP. Using the same antibody in the Western blots, APP appears as a cluster of bands at 98 kDa and a single band at 54 kDa, as shown previously (CitationCroucher et al 2003). Two days after injection with IL-1β or Aβ1–42 there was a marked decrease in the 54 kDa band in the injected as compared with noninjected eyes (Figure and Figure , respectively) and a decrease in the 98 kDa band after Aβ1–42 (Figure ). After injection with Aβ1–42 there was also the appearance of lower molecular weight bands at around 13 kDa and a band at approx 82 kDa (Figure ). Interestingly, we were not able to detect the same band pattern in retinas from eyes injected with S100B, even though there was an up-regulation of the 98 kDa band (Figure ). Figure also shows faint band fragments at approximately 30 kDa and at 37 kDa in injected and noninjected eyes. Seven days after injection of IL-1β, the 98 and 54 kDa bands had returned to the same levels as the noninjected eyes (not shown). Again, seven days after S100B injection, there was no difference in the levels and band pattern of APP between injected and noninjected eyes (not shown).
Figure 3 Western blotting of APP in retinas injected with (a) IL-1β, (b) S100B, (c) Aβ1–42 peptide, and (d) PBS 2 days after injection. Each panel shows 2 animals per treatment. Both IL-1β and Aβ1–42 show a marked reduction in APP levels in the injected left eye (L) compared with the noninjected right eye (R), particularly in the 54 kDa band. There was no down-regulation after injection with S100B or PBS. Note also the appearance of bands around 13 kDa and 82 Kda after injection with Aβ1–42.
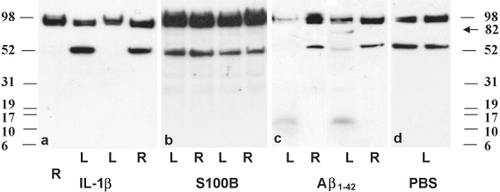
S100B is up-regulated by Aβ1–42 and IL-1β in vivo
As S100B appeared to be up-regulating APP expression in a sub-population of the RGCs and has been implicated in AD, we wanted to see how this cytokine is itself regulated by IL-1β and Aβ1–42. In naïve, untreated retinas, S100B is constitutively expressed in the Müller glial cell end feet in the ganglion cell layer (Figure ). Injection of PBS caused a slight increase in S100B staining (Figure ). After injection with aggregated Aβ1–42 (2 nmol/3 μl, 2 days post-injection) there was a marked up-regulation of S100B in the Müller cell end feet and also the Müller cell bodies within the inner nuclear layer as well as the appearance of staining throughout the Müller cell radial processes (Figure ). Interestingly, we also noted an increase in S100B staining in the contralateral retina (not shown). Western blotting with an anti-S100B antibody showed the appearance of a strong band around 15 kDa, corresponding to S100B after injection with Aβ1–42 (Figure ). The 7 kDa band seen in lanes loaded with recombinant S100B probably represents the monomer, whereas the 15 kDa band in the left (injected) eye probably represents the biologically active homodimer of S100B. There was no increase in S100B staining 2 days after injection of IL-1β (data not shown). However, there was a marked increase in S100B in the Müller glial cells 7 days after injection of IL-1β (Figure ) and, again, we noted a contralateral effect with increased S100B in the noninjected eye (data not shown).
Figure 4 S100B immunostaining in (a) noninjected rat retina shows that there is normal expression of the protein in the Müller cell endfeet. (b) S100B is only slightly increased after injection of PBS. (c) 2 days after injection of Aβ1–42 there is a marked up-regulation of S100B in the Müller cell endfeet, cell bodies (arrowhead) and processes (arrows) throughout the retina. (d) There was also a marked increase in S100B immunoreactivity 7 days after injection of IL-1β. (e) The increase in S100B after Aβ1–42 injection was confirmed by Western blotting. Recombinant bovine S100B is shown in lanes 1–3 and forms two bands at 7 and 19 kDa. Injection of Aβ1–42 caused strong expression of a single 15 kDa band in the injected left eye (L) compared with a comparatively weak expression in the noninjected, right eye (R).
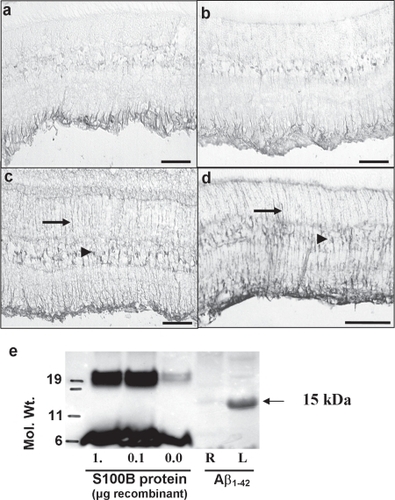
Discussion
This study has highlighted the differential effects of two amyloid plaque-associated factors and Aβ1–42 on the expression and processing of APP. Injection of IL-1β resulted in the reduction of APP-IR in RGCs and, along with injection of Aβ1–42, a reduction on Western blot. However, injection of S100B appears to increase APP levels in a population of the RGC (Figure –j) and an up-regulation of the 98 kDa APP band (Figure ) as well as the appearance of faint protein bands at approximately 30 kDa and 37 kDa.
The reduction in APP induced by IL-1β and Aβ1–42 appears to be widespread throughout the ganglion cell layer. The reason for this APP down-regulation is unclear but it may be that it is a stress response of the peptide itself. In vivo, it has previously been demonstrated that some cytokines can down-regulate APP as well as the anti-apoptotic protein, Bcl-2, levels in the rat retina (CitationChen et al 1999). This appears to contradict studies that have shown that IL-1β can increase mRNA for APP (CitationGoldgaber et al 1989; CitationBuxbaum et al 1992), but may reflect the differences between the in vitro and in vivo systems. Whether we are observing a decrease in the production of APP, an increase in metabolism or both remains to be fully established, but we have observed, particularly after Aβ1–42 injection, lower molecular weight bands that may represent breakdown products. In vitro evidence has shown that IL-1β alone and in combination with other cytokines can induce the production of soluble APPs (sAPPs) or α-cleaved APPs (αAPPs) from a neuronal cell line stably transfected with APP. In combination with interferon-γ, there was also an increase in secreted Aβ peptides in the same system (CitationSastre et al 2003). These changes occurred in the absence of a change in the cell-associated APP levels and so are likely to be a direct consequence of APP processing.
The APP band pattern produced in the retinas exposed to Aβ1–42 is of particular interest since it appears similar to that of retinas exposed to the broad spectrum metabotropic glutamate (mGlu) receptor agonist, (1S,3R)-1-aminocyclopentane-1,3-dicarboxylic acid ((1S,3R)-ACPD) (CitationCroucher et al 2003). The APP fragment that appears between the 98 kDa holoprotein and the 54 kDa fragment may be an 82 kDa non-amyloidogenic soluble fragment (sAPP) formed from metabolism of APP by α-secretase leading to proteolyic cleavage within the Aβ region (Figure ). CitationCroucher and colleagues (2003) also demonstrated that fragments of APP metabolism can be secreted into the vitreous fluid of rat eyes after stimulation with this mGlu receptor agonist and, in view of the similarity in the retinal APP Western blot band pattern, it is likely that the same is happening here.
IL-1β has been shown to cause changes in APP levels in other cell systems in vitro. In U-373 MG astrocytoma cells, IL-1β caused an increase in αAPPs (CitationGitter et al 2000), whilst in the PC12 cell line, IL-1β enhanced the secretion of N-terminal fragments of APP, again reflecting increased processing of APP (CitationDash and Moore 1995).
Our Western blots showed a greater reduction in the 54 kDa band compared with the 98 kDa, especially after IL-1β injection (Figure ). It would appear that this band is more sensitive to the degradation process than the 98 kDa band. Interestingly, this 54 kDa band has also been identified in the neuromuscular junction, which, like the retina, is rich in cholinergic innervations (CitationDash and Moore 1995) and may imply that cholinergic innervation exerts some control over APP levels. It remains to be seen if the IL-1β- or Aβ1–42-induced metabolism of APP yields amyloidogenic Aβ1–42 in this in vivo model.
S100B induced a small decrease in APP-IR in the RGC (Figure and h), though not as marked as with IL-1β (Figure and d), suggesting that S100B may not be initiating such a severe response as the other mediator. However, we also noted the appearance of some large, intensely APP-IR cells within the retinal ganglion cell layer (Figure g–j). This was accompanied by marked up-regulation the 98 kDa APP holoprotein in the injected and noninjected eyes of rats treated with S100B as determined by Western blot (Figure ); that is, S100β induced a contralateral effect on APP biosynthesis. It may be that the increase in the APP levels in these large ganglion cells is masking the small loss of APP seen in IHC. The appearance of faint bands at approximately 30 kDa and 37 kDa in Figure might reflect a different APP metabolism to that produced by injection of Aβ1–42.
To our knowledge, this is the first study that directly links S100B to increases in APP in vivo. S100B has been shown to cause an increase in APP protein and message in neurones from primary culture of rat foetal cortex (CitationLi et al 1998). At low doses, S100B promotes neurite outgrowth (CitationKligman and Marshak 1985) and enhances neuronal survival after injury and during development (CitationWinningham-Major et al 1989). Given its role in axonal transport, the appearance of increased APP may indicate that that these cells are undergoing some aberrant sprouting/neuritic outgrowth. The observation of increased APP in a subset of projection neurons may have important implications in AD. Astrocytes over-expressing S100B have been found to correlate with the proliferation of dystrophic neurites over-expressing APP in the cortex and within plaques in AD patients (CitationMrak et al 1996) and here we provide direct evidence that S100B can induce increases in neuronal APP in an animal model. Interestingly, we noted the appearance of a small number of densely stained RGCs 7 days after the injection of IL-1β. Our results show that an intravitreal injection of IL-1β causes a prolonged increase in S100B staining in Müller glia (Figure ) and exposure to Aβ1–42 up-regulates retinal S100β two days after injection (Figure ). This may suggest that IL-1β-stimulated release of S100B is causing this delayed increase in cells over-expressing APP – an observation that warrants further investigation. The increase in S100B staining seen after IL-1β and Aβ1–42 seen here is similar to the increase in GFAP in Müller glia seen after Aβ1–42 treatment (CitationBamford et al 1997; CitationWalsh et al 2002), optic nerve crush, and intravitreal lipopolysaccharide injection (data not shown) and highlights that this response is not specific to any particular treatment and is a general marker of astrocytic activation. However, this raises the possibility that activation of glial cells, by whatever means, may exacerbate the disease progression.
Using a retinal-vitreal system, we have shown that intravitreal injection of either IL-1β or Aβ1–42 induce a reduction in APP levels probably due to altered metabolism while S100B increased APP in a subset of neurons in a time-dependent fashion. We have also shown that both Aβ1–42 and IL-1β up-regulate S100B itself. These changes may have important implications in the pathogenesis of AD. Both IL-1β and S100B have been shown to be associated with reactive astrocytes and dystrophic neurites over-expressing APP and here we provide in vivo evidence of a direct link between these mediators and APP production and metabolism. It has been hypothesised that in AD, a “cytokine cycle” may underlie the progression of the disease (CitationGriffin et al 1998). In this cycle, within the environs of senile plaques, Aβ1–42 and soluble APP cause the release of IL-1β from activated microglia. This in turn stimulates the release of S100B from astrocytes and has direct effects on APP levels in neurons. Our in vivo evidence suggests that S100B is secreted by glial Müller cells in the retina; that S100B is able to increase APP expression; and that both IL-1β and Aβ1–42 increase the processing of APP. Our retinal-vitreal system functions as an ideal in vivo system to study the interplay between IL-1β, S100B, Aβ1–42 and APP. Future work will determine the identities of the APP protein fragments seen in the Western blots published in this paper. It may be that the IL-1β-S100B cytokine cycle described above, in up-regulating APP levels, leads to increased production of the fibrillogenic Aβ1–42 and the formation of amyloid plaques.
Acknowledgements
This work was supported by the American Alzheimer’s Association (IRG-00-2441) and The National Institutes of Health (AG12411) and GlaxoSmithKline.
References
- AkaabouneMAllinquantBFarzaH2000Developmental regulation of amyloid precursor protein at the neuromuscular junction in mouse skeletal muscleMol Cell Neurosci153556710845772
- BamforthSDLightmanSLGreenwoodJ1997Interleukin-1 beta-induced disruption of the retinal vascular barrier of the central nervous system is mediated through leukocyte recruitment and histamineAm J Pathol150329409006348
- BhattacharyyaAOppenheimRWPrevetteD1992S100 is present in developing chicken neurons and Schwann cells and promotes motor neuron survival in vivoJ Neurobiol23451661634890
- BrescianiLGWalshDTGentlemanSM2002Developmental regulation and possible alternative cleavage of presenilin 1 in the rat retinaMol Cell Neurosci212394912401445
- BuxbaumJDOishiMChenHI1992Cholinergic agonists and interleukin 1 regulate processing and secretion of the Alzheimer beta/A4 amyloid protein precursorProc Natl Acad Sci USA891007581359534
- ChenSTGentlemanSMGareyLJ1996Distribution of beta-amyloid precursor and B-cell lymphoma protooncogene proteins in the rat retina after optic nerve transection or vascular lesionJ Neuropathol Exp Neurol551073828858004
- ChenSTJenAGentlemanSM1999Effects of bFGF and TGFbeta on the expression of amyloid precursor and B-cell lymphoma protooncogene proteins in the rat retinaNeuroreport105091210208580
- CiallellaJRIkonomovicMDPaljugWR2002Changes in expression of amyloid precursor protein and interleukin-1beta after experimental traumatic brain injury in ratsJ Neurotrauma1915556712542857
- CroucherMJPatelHWalshDT2003Up-regulation of soluble amyloid precursor protein fragment secretion in the rat retina in vivo by metabotropic glutamate receptor stimulationNeuroreport142271414625461
- DashPKMooreAN1995Enhanced processing of APP induced by IL-1 beta can be reduced by indomethacin and nordihydroguaiaretic acidBiochem Biophys Res Commun20854287695605
- DonatoR2001S100: a multigenic family of calcium-modulated proteins of the EF-hand type with intracellular and extracellular functional rolesInt J Biochem Cell Biol336376811390274
- GitterBDBoggsLNMayPC2000Regulation of cytokine secretion and amyloid precursor protein processing by proinflammatory amyloid beta (A beta)Ann N Y Acad Sci9171546411268339
- GoldgaberDHarrisHWHlaT1989Interleukin 1 regulates synthesis of amyloid beta-protein precursor mRNA in human endothelial cellsProc Natl Acad Sci USA867606102508093
- GriffinWSShengJGRobertsGW1995Interleukin-1 expression in different plaque types in Alzheimer’s disease: significance in plaque evolutionJ Neuropathol Exp Neurol54276817876895
- GriffinWSShengJGRoystonMC1998Glial-neuronal interactions in Alzheimer’s disease: the potential role of a ‘cytokine cycle’ in disease progressionBrain Pathol865729458167
- GuoQXChauRMYangSZ1991Development of choline acetyltransferase-immunoreactive neurons in normal and intracranially transplanted retinas in ratsBrain Res Dev Brain Res6217787
- GuoQXYuMCGareyLJ1992aDevelopment of parvalbumin immunoreactive neurons in normal and intracranially transplanted retinas in the ratExp Brain Res90359681397149
- GuoQXYangSZLiangCL1992bTyrosine-hydroxylase-immunoreactive neurons in retinal transplants in the ratBiol Signals146561364035
- HardyJ1997Amyloid, the presenilins and Alzheimer’s diseaseTrends Neurosci2015499106355
- JenLSHartAJJenA1998Alzheimer’s peptide kills cells of retina in vivoNature39214019515959
- KimSHSmithCJVan EldikLJ2004Importance of MAPK pathways for microglial pro-inflammatory cytokine IL-1[beta] productionNeurobiol Aging25431915013563
- KligmanDMarshakDR1985Purification and characterization of a neurite extension factor from bovine brainProc Natl Acad Sci USA82713693863143
- LiYWangJShengJG1998S100 beta increases levels of beta-amyloid precursor protein and its encoding mRNA in rat neuronal culturesJ Neurochem71142189751173
- MorinPJAbrahamCRAmaratungaA1993Amyloid precursor protein is synthesized by retinal ganglion cells, rapidly transported to the optic nerve plasma membrane and nerve terminals, and metabolizedJ Neurochem61464737687653
- MrakREShengJGGriffinWS1996Correlation of astrocytic S100 beta expression with dystrophic neurites in amyloid plaques of Alzheimer’s diseaseJ Neuropathol Exp Neurol5527398786385
- MrakREGriffinWS2001The role of activated astrocytes and of the neurotrophic cytokine S100B in the pathogenesis of Alzheimer’s diseaseNeurobiol Aging229152211754999
- SastreMDewachterILandrethGE2003Nonsteroidal anti-inflammatory drugs and peroxisome proliferator-activated receptor-gamma agonists modulate immunostimulated processing of amyloid precursor protein through regulation of beta-secretaseJ Neurosci23979680414586007
- ShengJGMrakRERovnaghiCR1996Human brain S100 beta and S100 beta mRNA expression increases with age: pathogenic implications for Alzheimer’s diseaseNeurobiol Aging17359638725896
- ShengJGMrakREBalesKR2000Overexpression of the neuritotrophic cytokine S100beta precedes the appearance of neuritic beta-amyloid plaques in APPV717F miceJ Neurochem7429530110617132
- WalshDTBrescianiLSaundersD2005Amyloid beta peptide causes chronic glial cell activation and neuro-degeneration after intravitreal injectionNeuropathol Appl Neurobiol3149150216150120
- WalshDTMonteroRMBrescianiLG2002Amyloid-beta peptide is toxic to neurons in vivo via indirect mechanismsNeurobiol Dis1020712079400
- WattsHRVinceVWalshDT2007Alterations in presenilin 1 processing by amyloid-beta peptide in the rat retinaExp Brain Res181697717333007
- Winningham-MajorFStaeckerJLBargerSW1989Neurite extension and neuronal survival activities of recombinant S100 beta proteins that differ in the content and position of cysteine residuesJ Cell Biol1093063712592414
- YangSZGuoQXTsangD1992Development of gamma-aminobutyric acid-immunoreactive neurons in normal and intracranially transplanted retinas in ratsBrain Res Bull28543501617437