Abstract
Chronic kidney disease (CKD) is estimated to affect almost 10% of individuals worldwide and is one of the leading causes of morbidity and mortality. Renal fibrosis, a central pathway in CKD progression (irrespective of etiology), is associated with shortened or dysfunctional telomeres in animal studies. Telomeres are specialized nucleoprotein structures located at the chromosome end that maintain genomic integrity. The mechanisms of associations between telomere length and CKD have not yet been fully elucidated, however, CKD patients with shorter telomere length may have decreased renal function and a higher mortality rate. A plethora of ongoing research has focused on possible therapeutic applications of telomeres with the overall goal to preserve telomere length as a therapy to treat CKD.
Plain language summary
Chronic kidney disease or CKD is one of the leading causes of illness and death worldwide. Scarring of the kidney tissue that occurs in CKD has been associated with shorter telomeres in studies using rats. Telomeres, said to act as the cellular ‘shoelace caps’, maintain the structure of chromosomes, allowing for genetic material inside cells to divide correctly. The length of telomeres (TL) is influenced by diverse factors such as genetics and lifestyle. The underlying processes for the associations between TL and CKD are still not understood, however, patients with CKD and shorter TL have reduced kidney function and an increased death rate. Therefore, research is focused on possible ways to preserve TL and treat CKD.
Tweetable abstract
Preserving telomere length may reduce rates of chronic kidney disease, thereby decreasing morbidity & mortality worldwide.
The kidneys maintain body homeostasis by regulating fluid, electrolyte and acid-base balance. They are also involved in blood pressure regulation, endocrine functions, blood filtration, metabolism and waste removal [Citation1]. In a healthy adult, about 100–110 ml of glomerular filtrate flows through the kidneys per min, which can decline to <15 ml/min during kidney disease [Citation2]. Chronic kidney disease (CKD) is defined as a glomerular filtration rate (GFR) <60 ml/min per 1.73 m2 decrease in GFR by ≥35%, increase in serum creatinine by >50% or markers of kidney damage lasting >3 months [Citation3]. It is a progressive disease that has become a global public health issue due to its increasingly high prevalence [Citation4–6]. A total of 9.1% of the world’s population is estimated to have CKD [Citation7]. CKD is known to cluster in families [Citation8] and eGFR shows a strong heritable component [Citation9].
Despite the associated morbidity and mortality, the current management strategy for CKD is essentially based on the management of existing comorbidities, such as diabetes, hypertension and obesity, to delay its progression [Citation10,Citation11]. The heterogeneous pathogenesis of CKD poses additional challenges for therapeutic interventions [Citation4,Citation12]. The most prevalent risk factors for CKD are family history, diabetes mellitus (DM), hypertension and obesity (), for a review, see Kazancioğlu [Citation13]. CKD patients may be asymptomatic in the early stages.
As renal function decreases, there is an accumulation of urea, toxins and other waste products resulting in uremia. Uremia induces immune deficiency, oxidative stress and inflammation. Oxidative stress, inflammation and immune deficiency are associated with increased telomere attrition. Shorter telomeres predispose to kidney fibrosis and decrease the tissue repair capacity of the kidney in response to injury. This increases susceptibility to onset of CKD and exacerbates existing CKD.
CKD: Chronic kidney disease.
Image created with BioRender.com.
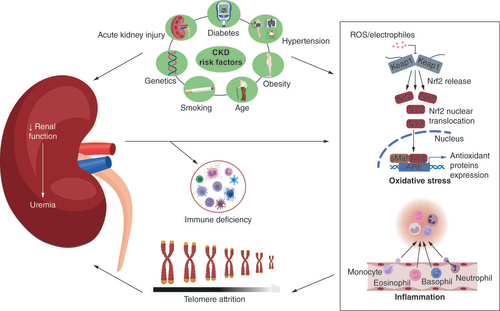
Regardless of the origin, there is a progressive loss of nephrons at the onset of CKD. As nephrons become irreversibly lost, there are fewer functional nephrons, leading to compensatory hyperfiltration and increased glomerular filtration pressure. The ensuing proteinuria and renin–angiotensin–aldosterone system (RAAS) activation results in interstitial inflammation, tubular fibrosis and glomerular sclerosis. Together these further accelerate the rate of nephron loss. This declining renal function leads to accumulation of urea and toxins and elevation of blood urea nitrogen and serum creatinine, hallmarks of uremia. Decreased renal clearance also alters general homeostasis, impacting other organs in the body. A causal player in the progression of CKD may be inherent in the loss of integrity of renal cells leading to tubulointerstitial fibrosis. In that regard, telomeres that protect from DNA damage, cell senescence and/or apoptosis play an important role (). This review focuses on the role of telomeres in the pathophysiology of CKD and the prospects of telomere therapy in CKD management.
Telomeres
Telomeres are specialized nucleoprotein structures located at the chromosome end. They maintain genomic integrity and are essential in the replication and proliferation of cells. Telomeric DNA contains a double-stranded region and terminates with a single-stranded region that consists of hundreds of G-rich base-pair strands () [Citation14,Citation15]. The telomere cap is a highly conserved six nucleotide repeat of 5′-TTAGGG-3′ at the end of the chromosome that binds to domains of the shelterin complex to form a protective telomeric loop that maintains telomere integrity and stability [Citation16]. The binding of the six-member shelterin protein complex to telomeric DNA protects the chromosomes from degradation and fusion with one another. It also prevents the stimulation of the DNA double-strand-break repair machinery in telomeres [Citation16,Citation17].
Due to the end replication problem, telomeres progressively shorten with each cell division.
Image created with BioRender.com.
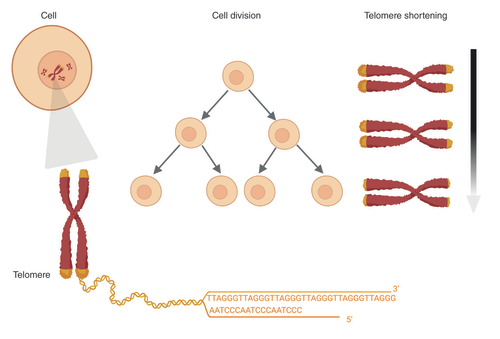
In linear chromosomes, DNA polymerase cannot completely replicate the DNA end and telomeres serve as buffers against the end replication problem [Citation18]. Therefore, telomere attrition occurs with each DNA replication because the end of the lagging strand of the telomeric DNA is not replicated () [Citation18,Citation19]. Telomeres progressively shorten with each cell division until they reach a critical short length that activates the DNA damage response (DDR) [Citation20,Citation21]. The DDR induces cell cycle arrest by inhibiting cyclin-dependent kinases (CDKs) via the p53/p21 or p16/Rb pathways resulting in replicative senescence or apoptosis [Citation22,Citation23].
The telomerase enzyme can maintain telomere length (TL) by adding single-stranded G-rich sequences (5′-TTAGGG-3′) to extend the 3′ overhang region [Citation15,Citation24,Citation25]. Human telomerase is an enzymatic ribonucleoprotein complex made up of a catalytic subunit human TERT, a template for reverse transcription human TERC and regulatory protein subunit dyskerin [Citation15,Citation24]. Baseline TL and rate of telomere shortening vary among individuals and across different organs in the same individual [Citation26]. TL is influenced by genetic and lifestyle factors including lack of physical activity, stress, smoking and alcohol abuse [Citation27,Citation28]. Oxidative damage induced by inflammation and oxidative stress can accelerate telomere shortening [Citation29–32]. Thus, the rate of telomere shortening is dependent on both cell replication and DNA damage.
Telomeres & CKD
Evidence from animal studies
As shown in , there are a few studies reported in animal models on CKD and telomeres, the general consensus of which is that telomeres play an important role in maintaining healthy kidney structure and function. In a mouse model of telomere dysfunction, a recent study from the Blasko group demonstrated that deletion of Trf1, a shelterin component, induced kidney fibrosis, a precursor to end-stage kidney disease [Citation33]. Telomerase-deficient mice (G3 TERT-/-) with short telomeres developed kidney fibrosis and severe kidney dysfunction after the administration of low-dose folic acid [Citation33]. A similar dose of folic acid did not induce fibrosis in wild-type mice [Citation33], indicating that mild kidney injury could increase the predisposition to develop kidney failure in the presence of shortened telomeres. Deprotected telomeres either due to telomere shortening or shelterin dysfunction undergo changes in their epigenetic pattern [Citation34]. The lncRNAs, telomeric repeat-containing RNA (TERRA) and telomere dysfunction-induced lncRNA (tdilncRNA), are transcribed at deprotected telomeres [Citation35,Citation36]. Growing evidence shows that lncRNAs may be implicated in the pathogenesis of renal fibrosis [Citation37] and TERRA has been associated with pulmonary fibrosis [Citation38]. TERRA and tdilncRNA may mediate telomere dysfunction and induce renal fibrosis.
Table 1. Studies of associations between telomeres and chronic kidney disease.
To determine whether telomere shortening or dysfunction could impair the regenerative capacity of the kidney, TERT and TERC knockout mice have been used as models. TERT and TERC knockout significantly delayed renal recovery after ischemia/reperfusion-induced renal injury and the animals with relatively longer TL showed better recovery [Citation39,Citation40]. Concurrent occurrences of shortened TL and conditions that increase the functional load of the kidney such as hypertension and diabetes may accelerate the development of CKD. In addition, pre-existing CKD may lead to telomere shortening in other organs, which may cause further complications in CKD. Wong et al. [Citation41] reported that kidney failure after myocardial infarction (MI) did not lead to excessive cardiac telomere shortening compared with that observed in kidney failure-only rats [Citation41]. An association between CKD and shorter renal TL has also been reported in cats [Citation42,Citation43].
The association between telomerase and CKD remains unclear in animal studies. Cyclosporine-induced oxidative stress in non-CKD rats led to renal telomere shortening without altering renal telomerase activity [Citation44]. This indicates the possibility of oxidative stress modulating TL independent of the telomerase pathway. In cats, kidney telomerase activity was similar across CKD and control groups, while liver telomerase activity was highest in the CKD group compared with the control group [Citation42]. The increase in liver telomerase activity may be compensatory, as uremic toxins are known to directly inhibit enzyme activation in the liver. On the other hand, severe kidney failure and uremia resulted in cardiac telomere shortening in rats [Citation41,Citation45]. Although oxidative stress has been implicated in animal models, the pathways involved are yet to be fully clarified. The shorter aortic TL of uremic rats has been correlated with increased oxidative stress and treatment with an antioxidant (vitamin C) resulted in longer TL in the treated group [Citation45]. Conversely, similar antioxidant treatment did not decrease oxidative stress in nonuremic aging rats [Citation45]. These findings suggest that uremia-induced oxidative stress may initiate telomere shortening in rats. Cyclosporine-induced oxidative stress has also been associated with nephrotoxicity and renal telomere shortening in non-CKD rats [Citation44]. Other factors that increase oxidative stress may accelerate kidney aging and telomere shortening.
Human studies
CKD patients: positive associations between TL & CKD
Shorter leukocyte TL (LTL) has been associated with decreased renal function in CKD patients [Citation46–48]. A study by Raschenberger et al. [Citation49] found a U-shaped association between LTL and CKD such that patients who have had CKD for between 6 months and 5 years exhibit shorter LTL than those patients who have CKD for <6 months and >5 years [Citation49]. This result suggests that TL change may not be unidirectional and may be related to the CKD stage. On the other hand, CKD may exacerbate telomere shortening in patients, accounting for the decreased TL between 6 months and 5 years of CKD. Longer telomeres in patients with CKD for >5 years may be due to survival bias. Shorter peripheral blood mononuclear cells (PBMCs) TL and LTL have also been reported to be independent predictors of morbidity and mortality in CKD patients [Citation50,Citation51]. Therefore, patients with shorter TL may have a higher mortality rate that may result in patients with long disease duration having comparably longer telomeres.
Incident kidney disease resulting in TL shortening and a further decline in renal function is another hypothesis. Betjes et al. [Citation46] observed that shortened T-cell TL in kidney failure may be responsible for the uremia-associated immune deficiency seen in CKD patients [Citation46]. In 222 patients at different CKD stages, the uremic state was associated with shorter PBMC TL and immune senescence [Citation50]. In this study, there was no difference in DNA damage, which is a marker of telomere-independent immune senescence, between uremic and nonuremic CKD patients [Citation50]. It was concluded that uremia-induced immune senescence might depend on telomere replicative senescence and not on telomere-independent immune senescence. Uremia may accelerate telomere shortening through inflammation and immunodeficiency.
Furthermore, other factors could contribute to this process. Renal replacement therapies, hemodialysis (HD) and kidney transplantation, for stage 4 and 5 CKD patients are linked with telomere attrition. A longer duration of HD correlates with shorter PBMC TL, possibly due to increased oxidative stress because of recurring exposure of blood within the extracorporeal circuit [Citation52]. Studies have reported that decreased LTL and PBMC TL are associated with inflammation biomarkers observed in patients on HD, which may contribute to telomere attrition [Citation53,Citation54]. Increased oxidative stress due to both the uremic state characteristic of kidney disease and persistent inflammation resulting from dialysis therapy may be largely responsible for the observed telomere attrition.
Patients with increased baseline leukocyte count have been reported to have longer LTL after the first year of dialysis therapy while those with longer baseline LTL had higher telomere attrition [Citation47]. This may be reflective of leukocyte proliferation in patients with high leukocyte count at the onset, highlighting the role of dialysis in oxidative stress in CKD. In a case-control study among HD patients, antioxidant vitamin D supplementation resulted in longer PBMC TL compared with untreated HD patients [Citation55]. This further underscores the possible role of oxidative stress in inducing telomere shortening due to HD therapy. For stage 4 and 5 CKD patients on HD, the use of antioxidants to attenuate the effect of oxidative stress and prevent further telomere shortening may be explored.
Reduction of the prooxidative and proinflammatory state in CKD alone may not result in immediate attenuation of telomere attrition. Kidney transplantation stabilizes the uremic milieu and increases long-term survival compared with dialysis therapy; however, kidney transplantation has been linked with increased telomere attrition compared with dialysis [Citation56]. The choice of immunosuppressive therapy may correlate with the degree of telomere shortening. Increased telomere attrition is observed in blood cells of kidney transplant patients on mycophenolic acid compared with those on less potent immunosuppressants such as azathioprine or mTOR inhibitors [Citation56,Citation57]. Furthermore, immunological aging and a decrease in T-cell TL associated with uremia are not reversed by kidney transplantation [Citation58]. In kidney transplant patients, while increased telomere attrition in blood cells of more immunosuppressed patients may be due to immune deficiency, factors such as comorbidities and donor characteristics may also have implications.
Patients with comorbidities: positive associations
Shorter LTL in Type 1/Type 2 DM (T1DM/T2DM) and cardiovascular disease (CVD) patients [Citation59–62] has been associated with decreased renal function. Several studies (see ) also show that telomere shortening could be an independent predictor of deteriorating renal function in T1DM and T2DM patients [Citation48,Citation59,Citation60,Citation63]. This suggests that telomere shortening may be involved in the pathogenesis of kidney disease in DM patients. A plausible mechanism is through oxidative stress characteristic of the hyperglycaemic milieu. Exposure of human kidney cells to a stressful environment of high glucose, resulting in increased oxidative stress, led to shorter TL [Citation64]. Similarly, other conditions that induce oxidative stress may accelerate telomere shortening and kidney disease progression. A strong association was observed between LTL and CKD progression in CKD patients who were also active smokers and/or DM patients [Citation65]. In addition, shorter TL in CKD patients may predispose them to the development of comorbidities. Shorter LTL and renal TL have been associated with renal arteriosclerosis [Citation66,Citation67]. The resulting endothelial dysfunction and arteriosclerosis may play a role in the pathogenesis of CVD.
CKD patients: lack of associations
Some studies have reported that the association between renal function and TL may be age-dependent in CKD patients [Citation51,Citation68,Citation69]. As discussed, TL may vary with CKD stage and the relationship between telomere shortening and renal function might be CKD-stage dependent. Variation of CKD stage among study participants could be responsible for the lack of association observed. The complex pathogenesis of renal disease, which could affect the rate of initial decline in renal function in the early stages of CKD, may also explain the discrepancies.
General population
A recent Mendelian randomization study using data from the CKDGen consortium and UK Biobank showed a causal link between genetically predicted telomere attrition and increased CKD risk [Citation70]. Shorter LTL has been associated with decreased renal function in healthy participants [Citation71]. For example, Eguchi et al. [Citation72] found that the correlation between LTL and eGFR is stronger in non-CKD patients, which may further explain the age-dependent association reported in some CKD patients. Although, an age-dependent association [Citation73] and a lack of associations [Citation74,Citation75] between LTL and renal function in non-CKD patients have been reported. These observations may occur because age is associated with both telomere attrition and renal function decline. In addition, the correlation between TL and renal function may differ depending on the renal function parameter assessed. In a cohort of healthy participants, the association between TL and some measures of renal function, such as glomerular filtration, urea and creatinine, was age-dependent while TL was independently correlated with albuminuria [Citation73]. TL may only be indicative of renal function using specific parameters in healthy people.
CKD patients: telomerase activity
Telomerase activity may provide insights into TL changes across CKD stages. Kidir et al. [Citation76] observed increased telomerase activity in CKD patients compared with controls with the highest telomerase activity in stage 5 CKD patients. In contrast, another study reported low telomerase activity in CKD patients with the highest telomerase activity in controls [Citation77]. Since the latter study analyzed telomerase activity in all CKD groups together, varying TL across CKD stages may have affected the overall results. Patients with longer LTL at baseline have been shown to experience higher telomere attrition [Citation47,Citation75], suggesting that telomerase activity may be more pronounced in short telomeres. Telomerase activity seems to decrease in the PBMCs of HD patients compared with controls and inversely correlates with the duration of HD treatment [Citation78]. More recently, a study reported no difference in PBMC telomerase activity between HD patients and healthy controls [Citation52]. Here, the long duration of HD treatment was associated with positive telomerase activity and relatively longer PBMC TL among HD patients [Citation52]. Increased oxidative stress during dialysis may stimulate telomerase activity. An increase in telomerase activity over the long duration of HD may further explain the longer LTL in patients with CKD for >5 years compared with patients with CKD for between 6 months and 5 years. A prospective study found no difference in PBMC telomerase activity between CKD and non-CKD patients and no correlation between telomerase activity and TL in PBMCs [Citation50]. Hence, telomerase activity may not be indicative of the development of CKD.
Current therapeutic applications of telomeres
Telomere biology has been implicated in the pathophysiology of a wide range of diseases with a plethora of ongoing research focused on possible therapeutic applications of telomeres. The goal is to increase or maintain TL in diseases associated with aging, whereas the opposite is true in cancer, where the focus is on reducing the proliferative capacity of tumor cells. Telomerase, TERT, TERC and telomere-associated proteins are current therapeutic targets as a result. Adeno-associated viruses for cardiac-specific TERT expression increased TL and improved prognosis without increasing cancer susceptibility in a preclinical mouse model of MI [Citation80]. Polysaccharides of Cistanche deserticola increased telomerase activity in mice hearts and brains [Citation81]. Female mice on dietary supplementation with telomerase activator TA-65 extracted from Astragalus membranaceus had enhanced health span and lengthening of short telomeres with no increase in the risk of developing cancer [Citation82]. These effects may be exerted through a telomerase-dependent pathway since TA-65 increased average TL and decreased the percentage of critically short telomeres in mouse embryonic fibroblasts (MEFs) with a single copy of the telomerase RNA TERC gene (G3 TERC+/-) but not in telomerase-deficient G3 TERC-/- MEFs [Citation82].
Adults on a health maintenance program consisting of TA-65 (10–50 mg daily) and a dietary supplement pack had a lengthening of critically short telomeres after 1 year [Citation83]. Low dose TA-65 (250 U) also increased LTL among healthy cytomegalovirus-positive participants over a 1-year period [Citation84]. High-dose TA-65 (1000 U) did not result in a statistically significant increase in TL [Citation84], suggesting a dose-dependent effect of telomerase activators. Ginkgo biloba extracts increased telomerase activity in cultured human endothelial progenitor cells [Citation85]. Telomerase activity of human HeLa cells increased after treatment with root extracts of Ashwagandha (Withania somnifera) [Citation86].
Metformin inhibited telomerase activity in endometrial and human breast cancer cell lines [Citation87–89]. Despite their telomerase inhibiting activity in cancer cells, evidence shows that antidiabetic agents may promote telomere maintenance in noncancerous cells. Sitagliptin significantly increased LTL in newly diagnosed T2DM patients after 2 months of therapy [Citation90]. Decreased leukocyte telomere attrition rate was reported among T2DM patients using antidiabetic agents compared with those not using medication [Citation91]. However, specific antidiabetic agents may not be protective against telomere attrition. Independent of glycemic control, patients whose antidiabetic therapy incorporated the use of acarbose had a higher telomere attrition rate compared with patients using acarbose-free antidiabetic therapy [Citation91]. A 6-year follow-up study of T2DM patients receiving oral antidiabetic therapy and/or insulin therapy showed that, despite good glycemic control in all study participants, 70% of patients showed LTL attrition while 30% of patients had an increase in LTL [Citation92]. Insulin was independently associated with the telomere attrition observed in these patients [Citation92]. Acarbose and insulin may promote telomere attrition, while metformin and some other antidiabetic agents may decrease telomere shortening.
Hypertensive BPH/2J mice show renal telomere attrition only after the development of hypertension [Citation93]. Alleviation of telomere attrition in hypertension may prove beneficial to delay hypertension-induced renal damage. Treatment with losartan resulted in significantly longer renal TL in young spontaneously hypertensive rats [Citation94]. These effects were sustained for a prolonged period even after discontinuation of therapy. Zhang et al. [Citation95] reported increased LTL and accompanying lower systolic blood pressure and pulse pressure in patients on calcium-channel blocker (CCB) or angiotensin-receptor blocker (ARB) therapy but not on diuretic therapy during a 2-year follow-up. A study on postmenopausal women with arterial hypertension found that, independent of blood pressure control, moxonidine therapy increased telomerase activity in blood monocytes while bisoprolol decreased telomerase activity [Citation96]. Diuretics, β-blockers and ARBs but not CCBs nor angiotensin-converting enzyme inhibitors are independent protective factors against decreasing TERT expression in peripheral blood leukocytes [Citation97]. These studies suggest that antihypertensives are associated with telomere maintenance; however, their action on telomere biology may be class specific.
In cancer therapy, oligonucleotides and small molecule inhibitors such as BIBR1532 that prevent telomere extension by direct inhibition of telomerase activity have been identified [Citation98,Citation99]. Telomere-specific antisense oligonucleotides that target lncRNAs transcribed at telomeres hold potential for use in diseases such as CKD by mitigating the effect of fibrosis and other phenotypic changes resulting from deprotected telomeres [Citation35]. In addition, senolytics that selectively clear senescent cells attenuated renal fibrosis in obese mice and there are ongoing clinical trials on their use in CKD [Citation100,Citation101]. TERT oncolytic adenovirus and vaccines targeting TERT tumor-associated antigens and G-quadruplex stabilizing compounds that alter telomerase function are also being studied for their anticancer effects [Citation98]. Telomerase is being combined with existing therapies to form new nanoplatforms that can increase the specificity of tumor cell identification and combat chemotherapy multidrug resistance [Citation102–105]. Natural telomerase inhibitors such as telomestatin in combination with chemotherapeutic agents potentiated anticancer effects in K562 leukemia cell lines [Citation15]. Despite the prospects that telomeres hold, the only telomere therapeutics in advanced stages of clinical trials are for the treatment of cancer. Imetelstat (GRN163L), an oligonucleotide telomerase inhibitor that competitively inhibits telomerase catalytic activity by binding to TERC, has completed phase II clinical trials for breast cancer, non-small cell lung cancer and multiple myeloma [Citation106]. Human TERT-based vaccines (GV1001, GRNVAC1 and combinations Vx-001) that induce the killing of telomerase-positive tumor cells through CD4+ and CD8+ T-cell cytotoxic responses with no significant toxicity to noncancerous cells are also being tested in different stages of clinical trials [Citation106].
Limitations
Most of the reviewed studies on telomeres and CKD in humans measured TL or telomerase activity in the blood (LTL and PBMCs). A validation study from the Genotype-Tissue Expression Consortium has shown that LTL can be a proxy for renal TL [Citation79]. This allows for the use of LTL for noninvasive measurement and prognosis management of CKD in the future. Furthermore, many of the studies discussed had a small number of subjects, therefore, not precluding the possibility of false associations.
Conclusion
With almost 10% of the world’s population impacted by CKD, there is a need to find new effective therapies. Several studies indicate a correlation between shorter length of telomere and onset of CKD. Although many of these studies were conducted using leukocytes rather than kidney tissue and had small samples, there is compelling evidence for this association. This association has brought to the foreground the important therapeutic role of the enzyme telomerase, which can maintain TL. Studies have demonstrated that TERT knockout significantly delays renal recovery. The causality of the association between CKD and shorter telomeres also remains unclear, but fibrosis seems to play a central role here. Another intriguing piece of data suggests that CKD patients with shorter TL may have a higher mortality rate, again supporting the evidence that maintaining TL is a treatment avenue. Further work to clarify these associations and their therapeutic potential is warranted.
Future perspective
With increasing investigation of the associations between telomeres and CKD, novel management and treatment strategies for CKD are on the horizon. Telomerase, shelterin protein complex and telomere maintenance genes are potential therapeutic targets (). Telomere-specific antisense oligonucleotides that deplete TERRA and tdilncRNAs may be a strategy to alleviate renal fibrosis. TL assessment could also serve as a diagnostic biomarker for the early detection of CKD.
Treatment possibilities include gene therapy using genes in the telomerase subunits TERT and TERC, proteins from the shelterin complex (TRF1, TRF2, TIN2, POT1, RAP1, TPP1) and other telomere maintenance genes, including PARN, CTC1, TCBA1, NHP2, RTEL1, NOP10, DKC1 and NAF1. Use of telomerase activators such as Astragalus membranaceus, Centella asiatica, oleanolic acid and maslinic acid can also be explored.
Image created with BioRender.com.
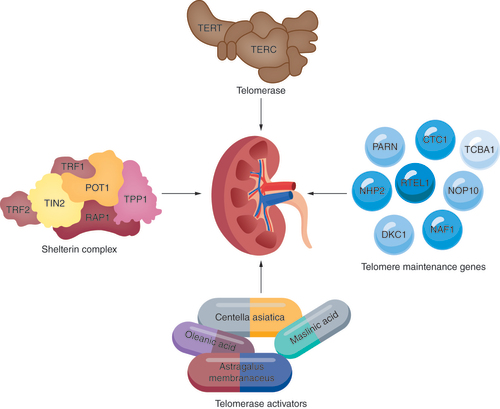
Chronic kidney disease (CKD) is a complex multifactorial condition with increasing global prevalence. Regardless of the etiology, renal fibrosis is an integral pathway for CKD progression.
Telomeres & CKD: evidence from animal studies
Renal fibrosis is associated with shortened or dysfunctional telomeres and may provide insights into the role of telomeres in CKD pathogenesis.
Telomeres & CKD: human studies
Telomeres have been linked with the development and progression of CKD and shorter telomeres may predispose to the development of CKD complications.
Current therapeutic applications of telomeres
Telomerase activator supplements have increased telomere length without increasing the risk of developing cancer.
Future directions
Telomeres and telomere maintenance genes are potential therapeutic intervention targets for the early detection and management of CKD.
Author contributions
OA Akinnibosun: Conception and design; literature search; manuscript draft; critical revision; final approval of published version; agreement to be accountable for all aspects of the work. MC Maier: literature search; manuscript draft; final approval of published version; agreement to be accountable for all aspects of the work. J Eales: literature search; critical revision; final approval of published version; agreement to be accountable for all aspects of the work. M Tomaszewski: literature search; critical revision; final approval of published version; agreement to be accountable for all aspects of the work. FJ Charchar: conception and design; critical revision; final approval of published version; agreement to be accountable for all aspects of the work.
Financial & competing interests disclosure
The British Heart Foundation project grant (PG/06/097/21331) and National Health Medical Research Foundation of Australia project grant (GNT1123472) both fund the open access of this paper. OA Akinnibosun is funded by Destination Australia Scholarship. The authors have no other relevant affiliations or financial involvement with any organization or entity with a financial interest in or financial conflict with the subject matter or materials discussed in the manuscript apart from those disclosed.
No writing assistance was utilized in the production of this manuscript.
Additional information
Funding
References
- Gekle M . Kidney and aging-a narrative review. Exp. Gerontol.87, 153–155 (2017).
- Glassock RJ , RuleAD. Aging and the kidneys: anatomy, physiology and consequences for defining chronic kidney disease. Nephron134(1), 25–29 (2016).
- Levey AS , EckardtK-U , DormanNMet al. Nomenclature for kidney function and disease: report of a Kidney Disease: Improving Global Outcomes (KDIGO) Consensus Conference. Kidney Int.97(6), 1117–1129 (2020).
- Eckardt K-U , CoreshJ , DevuystOet al. Evolving importance of kidney disease: from subspecialty to global health burden. Lancet382(9887), 158–169 (2013).
- Jha V , Garcia-GarciaG , IsekiKet al. Chronic kidney disease: global dimension and perspectives. Lancet382(9888), 260–272 (2013).
- Jager KJ , KovesdyC , LanghamR , RosenbergM , JhaV , ZoccaliC. A single number for advocacy and communication-worldwide more than 850 million individuals have kidney diseases. Kidney Int.34(11), 1803–1805 (2019).
- Bikbov B , PurcellCA , LeveyASet al. Global, regional, and national burden of chronic kidney disease, 1990–2017: a systematic analysis for the Global Burden of Disease Study 2017. Lancet395(10225), 709–733 (2020).
- Zhang J , ThioCH , GansevoortRT , SniederH. Familial aggregation of CKD and heritability of kidney biomarkers in the general population: the Lifelines Cohort Study. Am. J. Kidney Dis.77(6), 869–878 (2021).
- Debiec R , ChristofidouP , DenniffMet al. Urotensin-II system in genetic control of blood pressure and renal function. PLOS ONE8(12), e83137 (2013).
- Levin A , StevensPE , BilousRWet al. Kidney Disease: Improving Global Outcomes (KDIGO) CKD Work Group. KDIGO 2012 clinical practice guideline for the evaluation and management of chronic kidney disease. Kidney Int. Suppl.3(1), 1–150 (2013).
- Peng SY , HeJW , HuangJSet al. Self-management interventions for chronic kidney disease: a systematic review and meta-analysis. BMC Nephrol.20(1), 142 (2019).
- Keith DS , NicholsGA , GullionCM , BrownJB , SmithDH. Longitudinal follow-up and outcomes among a population with chronic kidney disease in a large managed care organization. Arch. Intern. Med.164(6), 659–663 (2004).
- Kazancioğlu R . Risk factors for chronic kidney disease: an update. Kidney Int. Suppl.3(4), 368–371 (2013).
- Allshire RC , DempsterM , HastieND. Human telomeres contain at least three types of G-rich repeat distributed non-randomly. Nucleic Acids Res.17(12), 4611–4627 (1989).
- Bajaj S , KumarMS , PetersGJ , MayurYC. Targeting telomerase for its advent in cancer therapeutics. Med. Res. Rev.40(5), 1871–1919 (2020).
- Griffith JD , ComeauL , RosenfieldSet al. Mammalian telomeres end in a large duplex loop. Cell97(4), 503–514 (1999).
- Stewart JA , ChaikenMF , WangF , PriceCM. Maintaining the end: roles of telomere proteins in end-protection, telomere replication and length regulation. Mutat. Res.730(1-2), 12–19 (2012).
- Levy MZ , AllsoppRC , FutcherAB , GreiderCW , HarleyCB. Telomere end-replication problem and cell aging. J. Mol. Biol.225(4), 951–960 (1992).
- Lormand JD , BuncherN , MurphyCTet al. DNA polymerase δ stalls on telomeric lagging strand templates independently from G-quadruplex formation. Nucleic Acids Res.41(22), 10323–10333 (2013).
- Wright WE , ShayJW. Telomere biology in aging and cancer. J. Am. Geriatr. Soc.53(Suppl. 9), S292–S294 (2005).
- D’souza Y , LauzonC , ChuTW , AutexierC. Regulation of telomere length and homeostasis by telomerase enzyme processivity. J. Cell Sci.126(2), 676–687 (2013).
- Hayflick L , MoorheadPS. The serial cultivation of human diploid cell strains. Exp. Cell Res.25(3), 585–621 (1961).
- Jacobs JJ , DeLange T. Significant role for p16INK4a in p53-independent telomere-directed senescence. Curr. Biol.14(24), 2302–2308 (2004).
- Jiang J , ChanH , CashDDet al. Structure of Tetrahymena telomerase reveals previously unknown subunits, functions, and interactions. Science350(6260), (2015).
- Nguyen THD , TamJ , WuRAet al. Cryo-EM structure of substrate-bound human telomerase holoenzyme. Nature557(7704), 190–195 (2018).
- Oeseburg H , DeBoer RA , Van GilstWH , VanDer Harst P. Telomere biology in healthy aging and disease. Pflugers Arch.459(2), 259–268 (2010).
- Blackburn EH , EpelES , LinJ. Human telomere biology: a contributory and interactive factor in aging, disease risks, and protection. Science350(6265), 1193–1198 (2015).
- Saretzki G . Telomeres, telomerase and ageing. Biochem. Cell Biol. Aging90, 221–308 (2018).
- Von Zglinicki T . Oxidative stress shortens telomeres. Trends Biochem. Sci.27(7), 339–344 (2002).
- Kawanishi S , OikawaS. Mechanism of telomere shortening by oxidative stress. Ann. NY Acad. Sci.1019(1), 278–284 (2004).
- Jurk D , WilsonC , PassosJFet al. Chronic inflammation induces telomere dysfunction and accelerates ageing in mice. Nat. Commun.5(1), 1–14 (2014).
- Zhang J , RaneG , DaiXet al. Ageing and the telomere connection: an intimate relationship with inflammation. Aging Res. Rev.25, 55–69 (2016).
- Saraswati S , MartínezP , Graña-CastroO , BlascoMA. Short and dysfunctional telomeres sensitize the kidneys to develop fibrosis. Nat. Aging1(3), 269–283 (2021).
- Galati A , MicheliE , CacchioneS. Chromatin structure in telomere dynamics. Front. Oncol.3, 46 (2013).
- Aguado J , DiFagagna FDA , WolvetangE. Telomere transcription in ageing. Aging Res. Rev.62, 101115 (2020).
- Porro A , FeuerhahnS , DelafontaineJ , RiethmanH , RougemontJ , LingnerJ. Functional characterization of the TERRA transcriptome at damaged telomeres. Nat. Commun.5(1), 1–13 (2014).
- Lin J , JiangZ , LiuCet al. Emerging roles of long non-coding RNAs in renal fibrosis. Life10(8), 131 (2020).
- El-Desoky MM , HewidyAA , FoudaAM , HishamFA. Telomeric repeat-containing ribonucleic acid (TERRA) expression in patients with idiopathic pulmonary fibrosis. Egypt. J. Med. Hum. Genet.22(1), 1–9 (2021).
- Westhoff JH , SchildhornC , JacobiCet al. Telomere shortening reduces regenerative capacity after acute kidney injury. J. Am. Soc. Nephrol.21(2), 327–336 (2010).
- Cheng HF , FanXF , LawsonWE , PaueksakonP , HarrisRC. Telomerase deficiency delays renal recovery in mice after ischemia-reperfusion injury by impairing autophagy. Kidney Int.88(1), 85–94 (2015).
- Wong LS , WindtWA , RoksAJet al. Renal failure induces telomere shortening in the rat heart. Neth. Heart J.17(5), 190–194 (2009).
- Quimby JM , MaranonDG , BattagliaCLR , MclelandSM , BrockWT , BaileySM. Feline chronic kidney disease is associated with shortened telomeres and increased cellular senescence. Am. J. Physiol. Renal Physiol.305(3), F295–F303 (2013).
- Quimby J , EricksonA , MclelandSet al. Renal senescence, telomere shortening and nitrosative stress in feline chronic kidney disease. Vet. Sci.8(12), 314 (2021).
- Raeisi S , GhorbanihaghjoA , ArganiHet al. Oxidative stress-induced renal telomere shortening as a mechanism of cyclosporine-induced nephrotoxicity. J. Biochem. Mol. Toxicol.32(8), e22166 (2018).
- Carracedo J , BuendiaP , MerinoAet al. Cellular senescence determines endothelial cell damage induced by uremia. Exp. Gerontol.48(8), 766–773 (2013).
- Betjes MGH , LangerakAW , VanDer Spek A , DeWit EA , LitjensNHR. Premature aging of circulating T cells in patients with end-stage renal disease. Kidney Int.80(2), 208–217 (2011).
- Kato S , ShielsPG , McguinnessDet al. Telomere attrition and elongation after chronic dialysis initiation in patients with end-stage renal disease. Blood Purif.41(1-3), 25–33 (2016).
- Gurung RL , YiamunaaM , LiuS , LiuJJ , LimSC , StudySD. Short leukocyte telomere length predicts albuminuria progression in individuals with Type 2 diabetes. Kidney Int. Rep.3(3), 592–601 (2018).
- Raschenberger J , KolleritsB , TitzeSet al. Do telomeres have a higher plasticity than thought? Results from the German Chronic Kidney Disease (GCKD) study as a high-risk population. Exp. Gerontol.72, 162–166 (2015).
- Crepin T , LegendreM , CarronCet al. Uraemia-induced immune senescence and clinical outcomes in chronic kidney disease patients. Nephrol. Dial. Transplant.35(4), 624–632 (2018).
- Fazzini F , LaminaC , RaschenbergerJet al. Results from the German Chronic Kidney Disease (GCKD) study support association of relative telomere length with mortality in a large cohort of patients with moderate chronic kidney disease. Kidney Int.98(2), 488–497 (2020).
- Stefanidis I , VoliotisG , PapanikolaouVet al. Telomere length in peripheral blood mononuclear cells of patients on chronic hemodialysis is related with telomerase activity and treatment duration. Artif. Organs39(9), 756–764 (2015).
- Ramírez R , CarracedoJ , SorianoSet al. Stress-induced premature senescence in mononuclear cells from patients on long-term hemodialysis. Am. J. Kidney Dis.45(2), 353–359 (2005).
- Carrero JJ , StenvinkelP , FellstromBet al. Telomere attrition is associated with inflammation, low fetuin-A levels and high mortality in prevalent haemodialysis patients. J. Intern. Med.263(3), 302–312 (2008).
- Borras M , PanizoS , SarroF , ValdivielsoJM , FernandezE. Assessment of the potential role of active vitamin D treatment in telomere length: a case-control study in hemodialysis patients. Clin. Ther.34(4), 849–856 (2012).
- Luttropp K , NordforsL , McguinnessDet al. Increased telomere attrition after renal transplantation-impact of antimetabolite therapy. Transplant. Direct2(12), e116 (2016).
- Petrara MR , SerrainoD , DiBella Cet al. Immune activation, immune senescence and levels of Epstein Barr Virus in kidney transplant patients: impact of mTOR inhibitors. Cancer Lett.469, 323–331 (2020).
- Meijers RWJ , LitjensNHR , DeWit EA , LangerakAW , BaanCC , BetjesMGH. Uremia-associated immunological aging is stably imprinted in the T-cell system and not reversed by kidney transplantation. Transpl. Int.27(12), 1272–1284 (2014).
- Tentolouris N , NzietchuengR , CattanVet al. White blood cells telomere length is shorter in males with type 2 diabetes and microalbuminuria. Diabetes Care30(11), 2909–2915 (2007).
- Fyhrquist F , TiituA , SaijonmaaO , ForsblomC , GroopPH. Finndiane Study G. Telomere length and progression of diabetic nephropathy in patients with type 1 diabetes. J. Intern. Med.267(3), 278–286 (2010).
- Van Der Harst P , WongLSM , DeBoer RAet al. Possible association between telomere length and renal dysfunction in patients with chronic heart failure. Am. J. Cardiol.102(2), 207–210 (2008).
- Wong LSM , VanDer Harst P , DeBoer RAet al. Renal dysfunction is associated with shorter telomere length in heart failure. Clin. Res. Cardiol.98(10), 629–634 (2009).
- Mazidi M , RezaieP , CovicAet al. Telomere attrition, kidney function, and prevalent chronic kidney disease in the United States. Oncotarget8(46), 80175–80181 (2017).
- Cao DW , JiangCM , WanCet al. Upregulation of MiR-126 delays the senescence of human glomerular mesangial cells induced by high glucose via telomere-p53-p21-Rb signaling pathway. Curr. Med. Sci.38(5), 758–764 (2018).
- Raschenberger J , KolleritsB , RitchieJet al. Association of relative telomere length with progression of chronic kidney disease in two cohorts: effect modification by smoking and diabetes. Sci. Rep.5, 11887 (2015).
- De Vusser K , PietersN , JanssenBet al. Telomere length, cardiovascular risk and arteriosclerosis in human kidneys: an observational cohort study. Aging7(10), 766–775 (2015).
- De Vusser K , MartensD , LerutE , KuypersD , NawrotTS , NaesensM. Replicative senescence and arteriosclerosis after kidney transplantation. Nephrol. Dial. Transplant.35(11), 1984–1995 (2020).
- Raschenberger J , KolleritsB , TitzeSet al. Association of relative telomere length with cardiovascular disease in a large chronic kidney disease cohort: The GCKD study. Atherosclerosis242(2), 529–534 (2015).
- Bansal N , WhooleyMA , ReganMet al. Association between kidney function and telomere length: the heart and soul study. Am. J. Nephrol.36(5), 405–411 (2012).
- Park S , LeeS , KimYet al. A Mendelian randomization study found causal linkage between telomere attrition and chronic kidney disease. Kidney Int.100(5), 1063–1070 (2021).
- Zhang WG , WangY , HouKet al. A correlation study of telomere length in peripheral blood leukocytes and kidney function with age. Mol. Med. Rep.11(6), 4359–4364 (2015).
- Eguchi K , HonigLS , LeeJH , HoshideS , KarioK. Short telomere length is associated with renal impairment in Japanese subjects with cardiovascular risk. PLOS ONE12(4), e0176138 (2017).
- Pykhtina VS , StrazheskoID , TkachevaONet al. Association of renal function, telomere length, and markers of chronic inflammation in patients without chronic kidney and cardiovascular diseases. Adv. Gerontol.6(3), 217–223 (2016).
- Ma C , HeS , LiP , ZhangH , LiW , LiY. Negative association between caloric intake and estimated glomerular filtration rate in a chinese population: mediation models involving mitochondrial function. Gerontology66(5), 439–446 (2020).
- Zhang WG , JiaLP , MaJet al. Peripheral blood leukocyte telomere length is associated with age but not renal function: a cross-sectional follow-up study. J. Nutr. Health Aging22(2), 276–281 (2018).
- Kidir V , AynaliA , AltuntasA , InalS , AridoganB , SezerMT. Telomerase activity in patients with stage 2-5D chronic kidney disease. Nefrologia37(6), 592–597 (2017).
- Kordinas V , TsirpanlisG , NicolaouCet al. Is there a connection between inflammation, telomerase activity and the transcriptional status of telomerase reverse transcriptase in renal failure? Cell. Mol. Biol. Lett. 20(2), 222–236 (2015).
- Tsirpanlis G , ChatzipanagiotouS , BoufidouFet al. Telomerase activity is decreased in peripheral blood mononuclear cells of hemodialysis patients. Am. J. Nephrol.26(1), 91–96 (2006).
- Demanelis K , JasmineF , ChenLSet al. Determinants of telomere length across human tissues. Science369(6509), (2020).
- Bar C , DeJesus BB , SerranoRet al. Telomerase expression confers cardioprotection in the adult mouse heart after acute myocardial infarction. Nat. Commun.5, 5863 (2014).
- Zhang H , LiY , SongYJCPJ. Effect of polysaccharides of Cistanche deserticola on immune cells and telomerase activity in aging mice. J. Chin. Pharm. Sci.46(14), 1081–1083 (2011).
- Bernardes De Jesus B , VeraE , SchneebergerKet al. Telomerase gene therapy in adult and old mice delays aging and increases longevity without increasing cancer. EMBO Mol. Med.4(8), 691–704 (2012).
- Harley CB , LiuW , BlascoMet al. A natural product telomerase activator as part of a health maintenance program. Rejuvenation Res.14(1), 45–56 (2011).
- Salvador L , SingaraveluG , HarleyCB , FlomP , SuramA , RaffaeleJM. A natural product telomerase activator lengthens telomeres in humans: a randomized, double blind, and placebo controlled study. Rejuvenation Res.19(6), 478–484 (2016).
- Dong XX , HuiZJ , XiangWX , RongZF , JianS , ZhuCJ. Ginkgo biloba extract reduces endothelial progenitor-cell senescence through augmentation of telomerase activity. J. Cardiovasc. Pharmacol.49(2), 111–115 (2007).
- Raguraman V , SubramaniamJR. Withania somnifera root extract enhances telomerase activity in the human HeLa cell line. Biotechnology7(4), 199–204 (2016).
- Cantrell LA , ZhouC , MendivilA , MalloyKM , GehrigPA , Bae-JumpVL. Metformin is a potent inhibitor of endometrial cancer cell proliferation-implications for a novel treatment strategy. Gynecol. Oncol.116(1), 92–98 (2010).
- Hanna RK , ZhouC , MalloyKMet al. Metformin potentiates the effects of paclitaxel in endometrial cancer cells through inhibition of cell proliferation and modulation of the mTOR pathway. Gynecol. Oncol.125(2), 458–469 (2012).
- Javidfar S , Pilehvar-SoltanahmadiY , FarajzadehRet al. The inhibitory effects of nano-encapsulated metformin on growth and hTERT expression in breast cancer cells. J. Drug Deliv. Sci. Technol.43, 19–26 (2018).
- Ma DL , YuY , YuXF , ZhangMX , YangY. The changes of leukocyte telomere length and telomerase activity after sitagliptin intervention in newly diagnosed type 2 diabetes. Diabetes Metab. Res. Rev.31(3), 256–261 (2015).
- Liu JH , GeYL , WuSet al. Association between antidiabetic agents use and leukocyte telomere shortening rates in patients with type 2 diabetes. Aging11(2), 741–755 (2019).
- Zeng JB , LiuHB , PingF , LiW , LiYX. Insulin treatment affects leukocyte telomere length in patients with type 2 diabetes: 6-year longitudinal study. J. Diabetes Complications33(5), 363–367 (2019).
- Chiu CL , HearnNL , PaineD , SteinerN , LindJM. Does telomere shortening precede the onset of hypertension in spontaneously hypertensive mice?Twin Res. Hum. Genet.19(5), 422–429 (2016).
- Baumann M , BartholomeR , Peutz-KootstraCJ , SmitsJFM , Struijker-BoudierHaJ. Sustained tubulo-interstitial protection in SHRs by transient losartan treatment: an effect of decelerated aging?Am. J. Hypertens.21(2), 177–182 (2008).
- Zhang S , LiR , YangYet al. Longitudinal association of telomere attrition with the effects of antihypertensive treatment and blood pressure lowering. Aging Dis.11(3), 494–508 (2020).
- Dudinskaya EN , TkachevaON , BazaevaEVet al. New possibilities of using moxonidin for blood pressure control in female patients with osteopenia. Kardiologiya58, 36–45 (2018).
- Martynowicz H , GaćP , Kornafel-FlakOet al. The relationship between the effectiveness of blood pressure control and telomerase reverse transcriptase concentration, adipose tissue hormone concentration and endothelium function in hypertensives. Heart Lung Circ.29(8), e200–e209 (2020).
- Guterres AN , VillanuevaJ. Targeting telomerase for cancer therapy. Oncogene39(36), 5811–5824 (2020).
- Schrank Z , KhanN , OsudeCet al. Oligonucleotides targeting telomeres and telomerase in cancer. Molecules23(9), (2018).
- Kim SR , JiangK , OgrodnikMet al. Increased renal cellular senescence in murine high-fat diet: effect of the senolytic drug quercetin. Transl. Res.213, 112–123 (2019).
- Hickson LJ , PrataLGL , BobartSAet al. Senolytics decrease senescent cells in humans: preliminary report from a clinical trial of dasatinib plus quercetin in individuals with diabetic kidney disease. EBioMedicine47, 446–456 (2019).
- Miao L , LiuC , GeJet al. Antitumor effect of TRAIL on oral squamous cell carcinoma using magnetic nanoparticle-mediated gene expression. Cell Biochem. Biophys.69(3), 663–672 (2014).
- Liu Z , ZhaoJ , ZhangRet al. Cross-platform cancer cell identification using telomerase-specific spherical nucleic acids. ACS Nano12(4), 3629–3637 (2018).
- Wu YH , ZhongD , LiYHet al. Tumor-oriented telomerase-terminated nanoplatform as versatile strategy for multidrug resistance reversal in cancer treatment. Adv. Healthc. Mater.9(7), (2020).
- Wu Y , ZhongD , LiYet al. A tumor-activatable peptide supramolecular nanoplatform for the delivery of dual-gene targeted siRNAs for drug-resistant cancer treatment. Nanoscale13(9), 4887–4898 (2021).
- Wu LL , FidanK , UmJY , AhnKS. Telomerase: key regulator of inflammation and cancer. Pharmacol. Res.155, 104726 (2020).