Abstract
Purpose: To investigate the effect of heat shock protein (HSP) modulation on tumour coagulation by combining radiofrequency (RF) ablation with adjuvant liposomal quercetin and/or doxorubicin in a rat tumour model.
Methods: Sixty R3230 breast adenocarcinoma tumours/animals were used in this IACUC-approved study. Initially, 60 tumours (n = 6, each subgroup) were randomised into five groups: (1) RF alone, (2) intravenous (IV) liposomal quercetin alone (1 mg/kg), (3) IV liposomal quercetin followed 24 h later with RF, (4) RF followed 15 min later by IV liposomal doxorubicin (8 mg/kg), (5) IV liposomal quercetin 24 h before RF followed by IV liposomal doxorubicin 15 min post-ablation. Animals were sacrificed 4 or 24 h post-treatment and gross coagulation diameters were compared. Next, immunohistochemistry staining was performed for Hsp70 and cleaved caspase-3 expression. Comparisons were performed by using Student t-tests or ANOVA.
Results: Combination RF-quercetin significantly increased coagulation size compared with either RF or liposomal quercetin alone (13.1 ± 0.7 mm vs. 8.8 ± 1.2 mm or 2.3 ± 1.3 mm, respectively, P < 0.001 for all comparisons). Triple therapy (quercetin-RF-doxorubicin) showed larger coagulation diameter (14.5 ± 1.0 mm) at 24 h than quercetin-RF (P = 0.016) or RF-doxorubicin (13.2 ± 1.3 mm, P = 0.042). Combination quercetin-RF decreased Hsp70 expression compared with RF alone at both 4 h (percentage of stained cells/hpf 22.4 ± 13.9% vs. 38.8 ± 16.1%, P < 0.03) and 24 h (45.2 ± 10.5% vs. 81.1 ± 3.6%, P < 0.001). Quercetin-RF increased cleaved caspase-3 expression at both 4 h (percentage of stained cells/hpf 50.7 ± 13.4% vs. 41.9 ± 15.1%, P < 0.03) and 24 h (37.4 ± 7.8% vs. 33.2 ± 6.5%, P = 0.045); with, triple therapy (quercetin-RF-doxorubicin) resulting in the highest levels of apoptosis (45.1 ± 10.7%) at 24 h. Similar trends were observed for rim thickness.
Conclusions: Suppression of HSP production using adjuvant liposomal quercetin can increase apoptosis and improve RF ablation-induced tumour destruction. Further increases in tumour coagulation can be seen including an additional anti-tumour adjuvant agent such as liposomal doxorubicin.
Introduction
Minimally invasive, percutaneous treatment using radiofrequency (RF) ablation is increasingly used to treat focal tumours in a range of organs, and has been incorporated into treatment paradigms for focal tumours of the liver, lung, bone, and kidney Citation[1]. Yet, a key limitation of RF ablation has been difficulties in achieving a complete ablative margin for larger tumours, where residual, untreated tumour leads to local tumour progression Citation[2–5]. Most often, tumour cells survive ablation because of the biophysical limitations of the procedure, such as perfusion-mediated tissue cooling, that prevent uniform heating of the entire tumour volume to a temperature sufficient for inducing coagulation necrosis (50–60°C), especially in the peripheral area of ablation Citation[5], Citation[6]. Therefore, strategies that can increase the uniformity and completeness of RF tumour destruction, even for small lesions, are required.
One successful strategy to improve completeness of RF ablation has been to target these residual viable cells with adjuvant chemotherapy or radiation Citation[7], Citation[8]. The rationale of this combined approach is to increase tumour destruction occurring within the sizable peripheral zone of sub-lethal, temperatures (i.e. largely reversible cell damage induced by mildly elevating tissue temperatures to 41–45°C) surrounding the heat-induced coagulation Citation[7]. Improved tumour cytotoxicity is also likely to reduce the rate of local tumour progression at the treatment site. Indeed, several studies have reported increased tumour destruction and associated increased intratumoural drug accumulation with RF ablation and adjuvant intravenous (IV) liposomal doxorubicin in both experimental animal and pilot clinical studies Citation[9], Citation[10].
Most recently, several mechanisms accounting for the increased tumour destruction of combination therapy have been identified, most notably increased cell stress (in part, due to up-regulation of nitrative and oxidative pathways) leading to apoptosis in a rim of periablational tissue exposed to sublethal hyperthermia Citation[11]. Interestingly, increased heat-shock protein (HSP) production was also observed in a concentric ring of still-viable tumour surrounding the ablation zone, and immediately peripheral to the rim of apoptosis. These HSPs have known cellular protective effects against apoptosis through several pathways, including blocking of cytochrome c release from mitochondria Citation[12], inhibition of procaspase 9 activation, apoptosome formation Citation[13] and kinase JNK phosphorylation Citation[14]. The presence of a rim of increased HSP expression beyond the ablation zone with co-localisation to persistently viable tumour suggests that protective HSP effects may be inhibiting additional gains in tumour destruction at the ablation margin.
There are several pharmaceutical agents with known inhibitory effects on HSP expression. One in particular, quercetin, has known suppressive effects on the synthesis of heat shock proteins in a variety of cell lines Citation[15]. Underlying mechanisms include suppression of heat shock protein gene expression through reduced availability of heat shock transcription factor and the suppression of initiation and elongation of the Hsp70 mRNA Citation[16]. Therefore, combining RF ablation with adjuvant quercetin-loaded liposomes may reduce the previously observed HSP up-regulation, and increase thermal injury and tumour coagulation after RF ablation. Accordingly, the purpose of this study was to (1) determine whether RF ablation combined with IV liposomal quercetin and/or liposomal doxorubicin increased tumour coagulation over either therapy alone, (2) characterise the effects of adjuvant liposomal quercetin and/or liposomal doxorubicin on RF-induced HSP production and apoptosis using immunohistochemistry staining.
Materials and methods
Experimental overview
This study was performed in two phases to systematically investigate the potential effects of RF ablation with adjuvant liposomal quercetin and/or doxorubicin.
Phase I. Effect of RF ablation combined with liposomal quercetin/doxorubicin on tumour destruction
A total of 60 tumours (n = 6, each subgroup) were randomised to receive one of five therapeutic regimens including standardised RF ablation (conventional monopolar 1-cm tip; 5 min; 70°C) and/or IV liposomal quercetin (0.29 mg in 0.5 mL) or doxorubicin (1 mg in 0.5 mL). Treatment groups included: (1) RF ablation alone, (2) IV liposomal quercetin alone, (3) IV liposomal quercetin followed 24 h later with RF ablation, (4) RF ablation followed 15 min later by IV liposomal doxorubicin, (5) IV liposomal quercetin 24 h before RF ablation which was followed by IV liposomal doxorubicin 15 min post-ablation. Gross pathologic analysis was performed for all groups with 2% 2,3,5-triphenyl staining. In sections below, references to ‘quercetin’ and ‘doxorubicin’ imply liposomal encapsulation as no free drug (either quercetin or doxorubicin) was administered in this study.
Phase II. Effect of combination RF/liposomal quercetin or doxorubicin on apoptosis and heat shock protein production
For all tumour samples (4 h and 24 h post-treatment) from the five groups (RF alone, quercetin alone, quercetin-RF, RF-doxorubicin, quercetin-RF-doxorubicin), pathological change was assessed by haematoxylin and eosin (H&E) staining and expression of heat shock protein and apoptosis were evaluated by immunohistochemistry (IHC) staining. At least five samples from each of the five treatment groups underwent IHC staining for cleaved caspase-3 and Hsp70 (as described in detail below). Specific time points (4 h and 24 h after RF ablation) were selected for this study based upon the results of prior studies that demonstrated that HSP production started at 4 h and peaked at 24 h after RF ablation Citation[11].
Animal model
Approval of the Institutional Animal Care and Use Committee was obtained before the start of this study. For all experiments and procedures, anaesthesia was induced with intraperitoneal injection of a mixture of ketamine (50 mg/kg, Ketaject; Phoenix Pharmaceutical, St Joseph, MO) and xylazine (5 mg/kg, Bayer, Shawnee Mission, KS). Animals were sacrificed with an overdose (0.2 mL/kg) of pentobarbital sodium (Nembutal; Abbott Laboratories, North Chicago, IL).
Experiments were performed with a well-characterised (22,24,29) established R3230 mammary adenocarcinoma cell line used in multiple prior studies Citation[7], Citation[17]. Sixty fisher rats (120 ± 20 g; 13–14 weeks old, Taconic Farms, Germantown, NY) with R3230 tumours were used in this study. Fresh tumour was harvested from a live carrier and homogenised in a tissue grinder (Model 23; Kontes Glass, Vineland, NJ) and suspended in Roswell Park Memorial Institution (RPMI) 1640 medium (Biomedicals, Aurora, IL). One tumour was implanted into each animal by slowly injecting 0.3–0.4 mL of tumour suspension into the mammary fat pad of each animal via an 18-gauge needle. Animals were monitored every 2–3 days to measure tumour growth. Solid non-necrotic tumours of 1.2–1.6 cm, as determined at ultrasonography (absence of more than three separate cystic spaces >0.5 mm in diameter within the entire tumour) at the time of treatment, were used for this study.
RF application
Conventional monopolar RF was applied by using a 500-kHz RFA generator (Model 3E; Radionics, Burlington, MA). To complete the RF circuit, the animal was placed on a standardised metallic grounding pad (Radionics). Contact was assured by shaving the animal's back and by liberally applying electrolytic contact gel. Initially, the 1-cm tip of a 21-gauge electrically insulated electrode (SMK electrode; Radionics) was placed at the centre of the tumour. RF was applied for 5 min with the generator output titrated to maintain a designated tip temperature (70 ± 2°C, mean 91.3 ± 22.5 mA, range 48–155 mA). This standardised method of RFA application has been demonstrated previously to provide reproducible coagulation volumes with use of this conventional RFA system Citation[17].
Preparation and administration of adjuvant intravenous liposomal agents
A commercially available preparation of liposomal doxorubicin (Doxil; ALZA Pharmaceuticals, Palo Alto, CA) was used. Quercetin-loaded liposomes were prepared such that the lipid composition in these liposomes was identical to Doxil, as has been described in previous work Citation[11]. Briefly, 0.29 mg of quercetin (1 mg/mL solution in methanol) was added to hydrogenated soy phosphatidylcholine, cholesterol and polyethyleneglycol phosphatidylethanolamine (PEG2000-PE) (57.25:37.57:5.18 mol%, respectively) solutions in chloroform, and a lipid film was formed in a round-bottomed flask by solvent removal on a rotary evaporator. The lipid film was then rehydrated with 1 mL of phosphate buffered saline, pH 7.4 and the preparation was probe-sonicated with a sonic dismembrator (Model 100, Fisher Scientific, Pittsburgh, PA) at a power output of 7 W for 30 min. To remove any titanium particles which may have been shed from the tip of the probe during sonication, the sample was centrifuged for 10 min at 2000 rpm. Liposomes were loaded with 5 mol% quercetin (not to saturation), and the liposomal loading efficiency of quercetin was 100% (as noted above, 0.29 mg of quercetin was loaded in each administered dose). The liposomal size was 115 nm ± 43 nm. The zeta-potential was −34.7 ± 5.5 mV. The liposomal formulation was tested and stable for 1 week at 4°C in phosphate buffered saline (PBS) at a pH of 7.4 and for 24 h at 37°C in PBS with 10% foetal bovine serum (FBS). When prepared for the experiment, all formulations were administered within 24 h of preparation. Finally, the polydispersity coefficient was 0.315.
Liposomal quercetin was administered slowly (for 30 s via a 27-gauge needle) via tail vein injection 24 h pre-RF ablation in the combined therapy group at the maximum liposomal saturation dose (0.29 mg in 0.5 mL). Liposomal doxorubicin was injected with the same approach (1 mg in 0.5 mL IV per animal), but at a different time point (15 min post-RF), based upon prior studies demonstrating maximum tumour coagulation and intratumoural drug accumulation with this administration regimen.
Gross histopathological and IHC analysis
Gross measurements of tumour destruction were performed by staining for mitochondrial enzyme activity by incubating representative tissue sections for 30 min in 2% 2,3,5-triphenyl measured by callipers Citation[17], Citation[18]. Coagulation diameter (longest measurement perpendicular to the inserted electrode Citation[19]) was determined by blinded consensus of three observers (W.Y., M.A, S.N.G., with 2, 8, and 12 years experience, respectively). Previous studies have documented close correlation between gross pathologic and histopathological findings for RF-induced coagulative necrosis Citation[17].
Harvested tumours were sectioned perpendicularly to the direction of electrode insertion and placed in cassettes containing the central section of tumour. All tissue was fixed in 10% formalin overnight at 4oC, embedded in paraffin, and sectioned at a thickness of 5 µm. Tissues were stained with H&E for gross pathology.
Immunohistochemical staining
At least five samples from each of the five treatment groups underwent IHC staining for cleaved caspase-3 and Hsp70. For each stain, a cut-section of the treated tumour was prepared on a single slide from each of the five tumours assigned to each of the five treatment groups for each time point. Immunohistochemistry was performed using antibodies to cleaved caspase-3, a marker of apoptosis Citation[20] (Cell Signaling Technology, Danvers, MA), and Hsp70, a key product of the HSP chain (Stressgen, Chicago, MI) Citation[21], to detect evidence of apoptosis and HSP production, respectively, using previously described techniques Citation[11]. Five-micron sections were placed on charged plus slides (Fisher Scientific, Pittsburgh, PA) and incubated overnight at 37°C, and 1 h at 58°C. Upon paraffin removal and tissue rehydration, slides were heated via microwave oven at 97°C for 10 min for retrieval of antigenic activity. Sections were incubated with hydrogen peroxide at room temperature for 5 min to inactivate endogenous peroxidase. Immunohistochemistry assays were performed using antibodies on each sample to detect cleaved caspase-3 and Hsp70. Sections were incubated with primary antibodies at room temperature for 60 min, and washed with PBS. Sections were then incubated with species-matched secondary antibodies at room temperature for 30 min. Nuclear counterstaining was performed lightly with hematoxylin. Sections were dehydrated, and cover slips applied using Permount (Richard-Allan Scientific, Kalamazoo, MI).
Specimen slides were imaged and analysed using a Micromaster I microscope and Micron imaging software (Westover Scientific, Mill Creek, WA). Temporal evolution of cellular morphology and the spatial distribution of protein expression were determined in relation to the central zone of RF coagulation and to one another. Additionally, comparison of regions of cleaved caspase-3 and Hsp70 staining was performed by both a side-by-side comparison and overlaying the slides on top of each other to visualise spatial differences in staining in the periablational rim. Quantitative analysis was performed using two metrics: the average thickness of the rim of staining (as measured in three separate sites) and the percentage of cells that stain per high-powered field (hpf, 40×) within the selected areas in the rim of tumour/tissue surrounding the central ablation zone (as it has been previously shown that the maximum effects of apoptosis and Hsp70 expression occur in this location from combination therapy Citation[11]. Five random high power fields were analysed for a minimum of five specimens for each parameter and scored in a blinded fashion to remove observer bias.
As an additional control to ensure uniformity of staining, whenever direct comparisons were made, IHC was repeated with all relevant comparison slides stained at the same time. Accuracy of the final data was verified by the senior author (S.N.G.), who was blinded to treatment group.
Statistical analysis
The SPSS 13.0 software package (SPSS, Chicago, IL) was used for statistical analysis. All data were provided as mean plus or minus SD. Tumour destruction and immunohistochemistry results were compared using analysis of variance (ANOVA). Additional post-hoc analysis was performed with paired, two-tailed Student's t-test, if and only if, the analysis of variance achieved statistical significance. A P value of less than 0.05 was considered significant.
Results
Phase I. Effect of RF ablation combined with liposomal quercetin/doxorubicin on tumour destruction
The zone of coagulation (i.e. induced tumour necrosis) at gross pathological examination for all animals sacrificed 4 h and 24 h after last therapy was within measurement error (<1 mm) of the results of TTC staining (i.e. absent mitochondrial staining) in all cases. IV injection of liposomal quercetin alone showed scattered tiny areas of necrosis (2.3 ± 1.3 mm). RF ablation alone produced 8.8 ± 1.2 mm of coagulation at 24 h (P < 0.001, compared with quercetin alone). Greater coagulation (13.1 ± 0.7 mm) was observed in combination of liposomal quercetin and RF (P < 0.001, compared with RF alone). Triple therapy (quercetin-RF-doxorubicin) resulted in the largest coagulation diameter (14.5 ± 1.0 mm), compared to quercetin-RF (P = 0.016) or RF-doxorubicin (13.2 ± 1.3 mm, P = 0.042) at 24 h (). The amount of tumour coagulation significantly increased from 4 h to 24 h for all treatment groups except for quercetin alone (P < 0.001 for all comparisons) ().
Figure 1. Gross pathologic specimens of RF-induced coagulation. Tumours treated with (A) RF alone, (B) liposomal quercetin 24 h pre-RF, (C) liposomal doxorubicin 15 min post-RF, and (D) triple therapy consisting of liposomal quercetin 24 h pre-RF and followed doxorubicin 15 min post-RF are presented. The central white area represents treatment-induced tumour necrosis/coagulation (as noted by the white arrows in each image), with viable tumour staining red. Greater coagulation was observed with RF-quercetin (13.1 ± 0.7 mm) and RF-doxorubicin (13.2 ± 1.3 mm) compared to RF alone (p < 0.001 for both comparisons). The amount of tumour coagulation further increased for triple therapy (quercetin-RF-doxorubicin) (14.5 ± 1.0 mm), compared with quercetin-RF (p = 0.016).
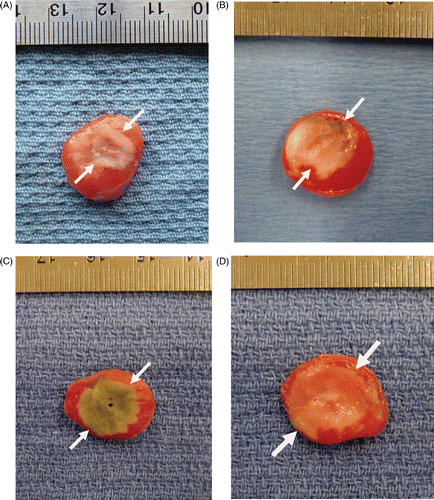
Figure 2. Comparison of tumour coagulation diameter at 4 h and 24 h post-RF ablation in different treatments. The bar graph demonstrates marked differences in coagulation obtained with varied treatment. Greatest coagulation is seen for triple therapy. The amount of tumour coagulation significantly increased from 4 to 24 h for all treatment groups except for quercetin alone (p < 0.001 for all comparisons).
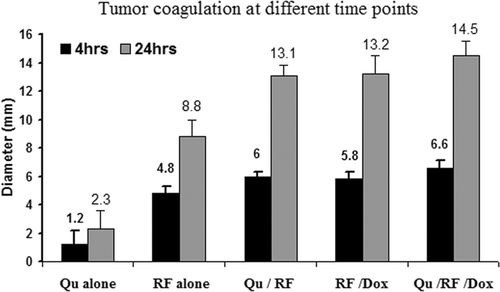
Phase II. Effect of combination RF and liposomal quercetin/doxorubicin on apoptosis and heat shock protein expression
At H&E staining, tissues treated with RF ablation alone exhibited characteristic tumour necrosis in the zone of ‘white coagulation’ surrounded by a process of vascularisation and early inflammatory reaction that progressed until 24 h (as has been described previously Citation[11]. At 24 h, classic findings of coagulation necrosis, including pyknotic nuclei and streaming cytoplasm, were visualised in the ablation zone for all samples treated with RF ablation. H&E staining for tumours treated with combination RF ablation and liposomal quercetin or doxorubicin exhibited increased periablational vascularisation. Although minimal scattered cellular apoptosis was seen at 4 h or 24 h in tumours treated with liposomal quercetin alone, there was no defined pattern of spatial distribution for apoptosis or heat shock protein staining. However, for RF alone and combination therapy with RF, IHC revealed bands of discreet cleaved caspase-3 staining immediately adjacent to the coagulation zone, surrounded by a ring of Hsp70 immediately peripheral to the rim of cleaved caspase-3 staining.
There was a minimal to slight rim of Hsp70 at the ablative margin for combination quercetin-RF compared to RF alone at 4 h (rim thickness 0.26 ± 0.08 mm, stained cells/hpf 22.4 ± 13.9% vs. 0.35 ± 0.18 mm, 38.8 ± 16.1%, P < 0.03, ). RF-doxorubicin increased Hsp70 staining at 4 h, compared with RF alone (0.58 ± 0.16 mm, 55.4 ± 11.5% vs. 0.35 ± 0.18 mm, 38.8 ± 16.1%, P < 0.001). The Hsp70 expression with triple therapy (quercetin-RF-doxorubicin) (0.36 ± 0.14 mm, 44.5 ± 18.4%) was between that of quercetin-RF and RF-doxorubicin at 4 h. At 24 h post-treatment, Hsp70 expression was increased in all groups. The lowest level of Hsp70 staining was observed for quercetin-RF (0.31 ± 0.12 mm, 45.2 ± 10.5%, ). Quercetin-RF-doxorubicin had less HSP production compared to RF-doxorubicin alone (0.52 ± 0.16 mm, 58.4 ± 19.0% vs. 0.61 ± 0.13 mm, 73.7 ± 11.5%, P < 0.05) ().
Figure 3. Effect of liposomal quercetin-RF on Hsp70 expression at 4 h. Tumour tissue from quercetin-RF (A, B) stained for Hsp70 demonstrates a weaker band of staining (black arrows) at the periphery of the ablation zone with a lower percentage of positive staining cells compared to RF alone (C, D), noting a marked decrease in heat shock protein formation at 4 h. (A, C = 4×; B, D = 40×).
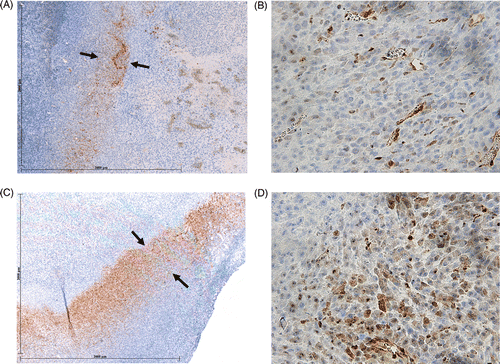
Figure 4. Effect of liposomal quercetin-RF on Hsp70 expression at 24 h. Tumour tissue from quercetin-RF (A, B) stained for Hsp70 demonstrates a comparatively weaker band of staining (black arrows) at the periphery of the ablation zone with a lower percentage of positive staining cells compared to RF alone (C, D), noting a persistent decrease in heat shock protein formation at 24 h. (A, C = 4×; B, D = 40×).
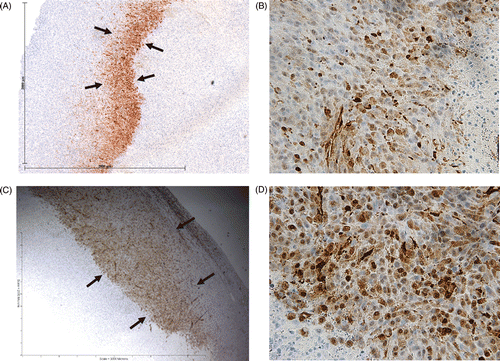
Table 1. Quantitative immunohistochemical staining for heat shock protein expression. There was significantly less Hsp70 expression at the ablative margin for quercetin-RF compared to RF alone at 4 h (rim thickness 0.26 ± 0.08 mm, percentage of stained cells/hpf 22.4 ± 13.9% vs. 0.35 ± 0.18 mm, 38.8 ± 16.1%, P < 0.03). Additionally, RF-doxorubicin resulted in the greatest Hsp70 expression compared to all other groups, including triple therapy (quercetin-RF-doxorubicin) (for both rim thickness and percentage of stained cells/hpf for all comparisons, P < 0.05). Similarly, at 24 h post-treatment, decreased Hsp70 expression was observed for treatments that included quercetin (more so with quercetin-RF than with triple therapy) compared to both RF alone and RF-doxorubicin (for both rim thickness and % stained cells/hpf for all comparisons, P < 0.05).
Greater cleaved caspase-3 staining was observed for quercetin-RF at 4 h, compared to RF alone (0.48 ± 0.19 mm, 50.7 ± 13.4% vs. 0.35 ± 0.18 mm, 41.9 ± 15.1%, P < 0.04, ). RF-doxorubicin and quercetin-RF-doxorubicin also showed greater cleaved caspase-3 staining than RF alone (P < 0.001, P < 0.03, respectively). Specifically, combination groups resulted in greater rim thickness (RF-doxorubicin: 0.49 ± 0.10 mm, quercetin-RF-doxorubicin: 0.48 ± 0.08 mm) and percentage of positive cells (RF-doxorubicin: 56.7 ± 10.5%, quercetin-RF-doxorubicin: 51.2 ± 12.4%) compared to the RF alone group (0.35 ± 0.18 mm, 41.9 ± 15.1%) at 4 h (P < 0.04). At 24 h, cleaved caspase-3 expression decreased in all groups (P < 0.001). Quercetin-RF and RF-doxorubicin had comparatively greater cleaved caspase-3 expression than RF alone (P < 0.05). Triple therapy with quercetin-RF-doxorubicin had a similar positive cell percentage (45.1 ± 10.8%), to RF-doxorubicin, and was significantly greater compared with other groups (P < 0.03, ).
Figure 5. Effect of liposomal quercetin-RF on apoptosis at 4 h. Tumour tissue from liposomal quercetin-RF (A, B) stained for cleaved caspase-3 demonstrates a thicker band of staining (black arrows) at the periphery of the ablation zone with a greater percentage of positive staining cells compared to RF alone (C, D), noting a marked increase in apoptotic activity at 4 h (A, C = 4×; B, D = 40×).
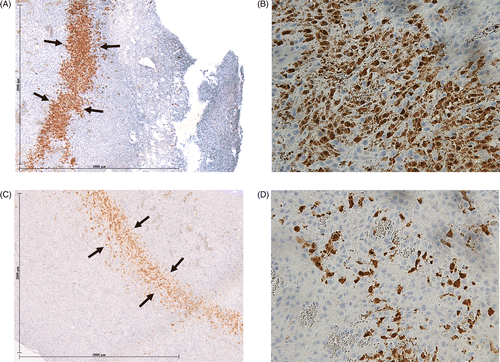
Table 2. Quantitative immunohistochemical staining for apoptosis. Greater cleaved caspase-3 staining was observed for quercetin-RF, RF-doxorubicin, and quercetin-RF-doxorubicin at 4 h, compared to RF alone (for both rim thickness and percentage of stained cells/hpf for all comparisons, P < 0.04 for all comparisons). At 24 h, cleaved caspase-3 expression decreased in all groups (P < 0.001). Quercetin-RF and RF-doxorubicin had comparatively greater cleaved caspase-3 expression than RF alone (for percentage of stained cells/hpf, P < 0.05). Triple therapy with quercetin-RF-doxorubicin had a similar positive cell percentage (45.1 ± 10.8%), to RF-doxorubicin, and was significantly greater compared with other groups (P < 0.03).
Discussion
Exposure to low, non-lethal levels of hyperthermia, especially in the periphery of the ablation zone, results in reversible cellular injury and up-regulation of cellular protective pathways. One such pathway, mediated by the heat shock protein family and the intracellular Hsp70 protein in particular, has known protective effects in the face of cellular injury from exposure to hyperthermia and chemotherapeutic agents. Several studies have demonstrated increased Hsp70 expression after RF ablation. For example, Yang et al. observed increased Hsp70 expression 4 and 10 h after RF ablation to temperatures >45°C in a colon cancer xenograft model implanted in nude mice Citation[22]. Similarly, elevated Hsp70 levels were also detected in biopsy specimens obtained 24 h after RF ablation of human hepatocellular carcinoma Citation[23]. Along these lines, in our study we demonstrated increased Hsp70 expression for tumours treated with RF ablation alone compared to control tumours. As anticipated, the predominant expression occurred in the transitional zone at the ablation margin where the greatest exposure to sub-lethal hyperthermia occurs. This corresponds to prior studies that have observed increased Hsp70 expression in the periablational transitional zone up to five days after RF ablation Citation[24], Citation[25].
More importantly, our results demonstrate that the Hsp70 pathway can be specifically and successfully targeted to increase overall RF-induced coagulation. Combining RF ablation with adjuvant liposomally encapsulated quercetin, a flavonoid agent that has known inhibitory effects on HSP expression and function Citation[15], significantly increased the ablation zone compared to RF alone. This correlated to an early (4 h) reduction in Hsp70 expression and increased apoptosis for combination quercetin-RF compared to RF alone. These results correspond to findings reported in a recent study by Yang et al. in which partial Hsp70 suppression was achieved when adjuvant liposomal paclitaxel was combined with RF ablation Citation[26].
Interestingly, in this study, the addition of quercetin to RF alone or RF-doxorubicin (triple therapy) results in only partial suppression of Hsp70 production, especially at later time points (∼24 h). There are several possible explanations for this. Firstly, the HSP suppressive effect of quercetin may be temporally limited, as early HSP suppression by quercetin may not have been able to keep up with progressive increases in HSP production, suggesting a role for either longer-acting HSP suppression or a multiple dosing algorithm to maximise anti-Hsp70 effects of quercetin. Additionally, liposome deposition occurs predominantly within the periablational tumour, such that HSP suppression may be limited by lack of significant drug deposition farther away from the RF zone. Nevertheless, the presence of residual HSP production at all time-points suggests that further investigation into additional methods of HSP suppression is still warranted. Finally, we also observed that different treatments had a variable effect on both cleaved caspase-3 and Hsp70 staining depending on whether the outcome measure was percentage of positive stained cells (a surrogate for drug effect or concentration) or rim thickness (which reflects geographic drug distribution). This underscores the heterogeneity of the periablational zone and the need for continued characterisation to maximise drug delivery, and ultimately, tumour destruction.
A number of experimental and clinical studies have demonstrated the benefits of combining RF ablation with IV liposomal doxorubicin, including increases in tumour coagulation and intratumoural drug accumulation Citation[7], Citation[10]. Most recently, histopathological analysis demonstrated increased Hsp70 expression in the periablational rim after RF ablation, especially when combined with adjuvant liposomal doxorubicin Citation[11]. For RF + Doxil, simultaneous staining for apoptosis and Hsp70 expression demonstrated a clear, defined zone of increased apoptotic cell death which was immediately peripherally surrounded by a rim of Hsp70 (located at a greater distance from the electrode than for treatment of RF alone). This suggested that using adjuvant agents that specifically target HSP pathways may increase tumour destruction even further and formed the basis of our rationale for combining RF ablation with both adjuvant liposomal quercetin and doxorubicin.
In our study, triple therapy increased tumour coagulation compared to RF/single adjuvant agent. Yet, based upon immunohistochemical staining, there was less HSP suppression compared to quercetin-RF. Similarly, the caspase expression and apoptosis observed for RF-doxorubicin was greater than that observed for triple therapy. This latter finding suggests that apoptotic mechanisms may already be largely saturated by doxorubicin and that the benefit of triple therapy may also involve other pathways. Thus, the increased tumour destructive effects of combining adjuvant quercetin and doxorubicin may be multifactorial. Indeed, in addition to our initial hypothesis, several in vitro studies using breast tumour and spleen cells suggest that quercetin may improve the therapeutic index and efficacy of doxorubicin by reversing cell resistance in hypoxic conditions Citation[27]. While quercetin acts predominantly through anti-HSP effects, adjuvant doxorubicin has been shown to both increase apoptosis and HSP expression Citation[11], suggesting that to some degree, both agents target different cellular stress/injury pathways. Regardless, these findings underscore the potential role of combining multiple adjuvant agents with RF ablation to maximise gains in overall tumour destruction, as reported in a recent study combining RF with liposomal doxorubicin and paclitaxel Citation[26].
While our results demonstrated that quercetin-RF increased tumour coagulation at 24 h and triple therapy with doxorubicin could further improve the result, the long-term outcome of combination therapy requires continued investigation. Extracellular HSP expression up-regulates the immune-mediated response to tumour cells Citation[28–30], and therefore, gains in tumour coagulation from intracellular HSP suppression may not translate directly to improvements in animal endpoint survival. As such, continued investigation into optimal HSP modulation is required. Such discord was noted In a recent study combining RF ablation with adjuvant liposomal paclitaxel, where improvements in tumour coagulation at 24 h and intratumoural drug accumulation did not translate into improved endpoint animal survival Citation[26]. This underscores that endpoint survival and delayed evaluation of tumour coagulation need to be viewed as important outcome measures. Indeed, while early evaluation of coagulation diameter can identify temporal differences between groups, the actual time it takes for a full effect to take place may vary.
There are several limitations to this study worthy of mention. Although the R3230 tumour model was selected because it is a well-characterised vascular solid adenocarcinoma whose results can be compared to other studies of RF ablation and liposomal chemotherapy performed in this model, careful interpretation and application of the results is required. For example, the differences identified between quercetin and doxorubicin-based regimens likely reflect in part underlying tumour susceptibility to each of these agents for this particular model, and so further study will be required in other models to determine which tumour types will respond to RF combined with either or both liposomal agents. Specifically, Hsp70 expression is variable between different tumour types (such as primary liver or lung tumours which currently undergo treatment with RF ablation), and therefore, the degree to which Hsp70 suppression would impact tumour coagulation will likely vary from tumour to tumour. Additionally, the administration of liposomal quercetin 24 h before RF ablation was selected based upon the need for intratumoural drug accumulation prior to RF ablation. Similarly, a single standardised quercetin dose was selected for this study. Incorporating higher doses of quercetin into the delivery vehicle could potentially result in even greater Hsp70 suppression, and therefore, further gains in tumour destruction. Finally, we have selected a commercially available pegylated stealth liposomal agent (doxorubicin) and prepared similarly constructed quercetin-containing liposomes. Prior studies have demonstrated good success with these preparations, such as increased tumour destruction and intratumoural drug accumulation while reducing tumour growth rates Citation[7]. As it has been previously shown that the empty liposome itself contributes to increases in observed tumour destruction, the continued use of this delivery vehicle allows a standardised comparison of the effects of different chemotherapy agents. However, additional characterisation and optimisation of the delivery vehicle and timing of the agent administration in future studies may improve in vivo drug delivery. We also acknowledge that other approaches, such as the incorporation of thermosensitive liposomal preparations into our combination therapy algorithm may yield different, and perhaps improved, results Citation[31], Citation[32]. However, given the timing of anti-HSP and pro-apoptosis effects that we have observed (4–12 h), it is far from clear that an additional early burst of drug release (0–1 h), both spatially and temporally, will definitely increase tumour destruction compared to a slower, more prolonged drug release. In particular, the paradigm of tissue heating (both timing and variances in temperature ranges in the treatment zone) vary significantly between focal high-temperature RF tumour ablation and the low-temperature conventional hyperthermia that has been combined with thermosensitive liposomes in existing literature – such that, it is likely too early to assume that thermosensitive liposomal preparations will definitely be ‘better’ than non-temperature-sensitive agents. Clearly, further investigation into identifying the delivery vehicle characteristics and administration paradigm is still required.
In conclusion, combining RF ablation with adjuvant IV liposomal chemotherapeutic agents, such as quercetin, that target heat shock protein pathways can increase overall tumour coagulation by suppressing HSP expression and increasing apoptosis in the periablational rim. Even further gains in tumour coagulation can be achieved with the addition of a second additional adjuvant agent such as liposomal doxorubicin. Further work is required to determine whether this combination therapy can increase animal endpoint survival, along with characterisation in different animal and tumour models with optimisation of administration regimens.
Declaration of interest: This study was supported by grants from the National Cancer Institute, National Institutes of Health, Bethesda, MD (R01CA133114, R01 CA100045, and 2R01 HL55519, CCNE 1U54CA151881-01). W.Y. is a recipient of the Chinese National High Technology Research and Development Program 863, Commission No. 2007AA02Z4B8. The authors alone are responsible for the content and writing of the paper.
References
- Dupuy D, Goldberg S. Image-guided radiofrequency tumor ablation: Challenges and opportunities – Part II. JVIR 2001; 12: 1135–1148
- Rossi S, Garbagnati F, Lencioni R, et al. Percutaneous radio-frequency thermal ablation of nonresectable hepatocellular carcinoma after occlusion of tumor blood supply. Radiology 2000; 217: 119–126
- Chen T, Guo J, Han C, Yang M, Cao X. Heat shock protein 70, released from heat-stressed tumor cells, initiates antitumor immunity by inducing tumor cell chemokine production and activating dendritic cells via TLR4 pathway. J Immunol 2009; 182: 1449–1459
- Livraghi T, Goldberg SN, Lazzaroni S, et al. Hepatocellular carcinoma: Radio-frequency ablation of medium and large lesions. Radiology 2000; 214: 761–768
- Goldberg SN, Hahn PF, Tanabe KK, et al. Percutaneous radiofrequency tissue ablation: Does perfusion-mediated tissue cooling limit coagulation necrosis?. J Vasc Interv Radiol 1998; 9: 101–111
- Patterson EJ, Scudamore CH, Owen DA, Nagy AG, Buczkowski AK. Radiofrequency ablation of porcine liver in vivo: Effects of blood flow and treatment time on lesion size. Ann Surg 1998; 227: 559–565
- Ahmed M, Goldberg SN. Combination radiofrequency thermal ablation and adjuvant IV liposomal doxorubicin increases tissue coagulation and intratumoural drug accumulation. Int J Hyperthermia 2004; 20: 781–802
- Horkan C, Dalal K, Coderre JA, et al. Reduced tumor growth with combined radiofrequency ablation and radiation therapy in a rat breast tumor model. Radiology 2005; 235: 81–88
- Goldberg SN, Kamel IR, Kruskal JB, et al. Radiofrequency ablation of hepatic tumors: Increased tumor destruction with adjuvant liposomal doxorubicin therapy. AJR Am J Roentgenol 2002; 179: 93–101
- Monsky WL, Kruskal JB, Lukyanov AN, et al. Radio-frequency ablation increases intratumoral liposomal doxorubicin accumulation in a rat breast tumor model. Radiology 2002; 224: 823–829
- Solazzo S, Ahmed M, Schor-Bardach R, et al. Liposomal doxorubicin increases radiofrequency ablation-induced tumor destruction by increasing cellular oxidative and nitrative stress and accelerating apoptotic pathways. Radiology 2010; 255: 62–74
- Polla BS, Kantengwa S, Francois D, et al. Mitochondria are selective targets for the protective effects of heat shock against oxidative injury. Proc Natl Acad Sci USA 1996; 93: 6458–6463
- Xanthoudakis S, Nicholson DW. Heat-shock proteins as death determinants. Nat Cell Biol 2000; 2: E163–165
- Gabai VL, Yaglom JA, Volloch V, et al. Hsp72-mediated suppression of c-Jun N-terminal kinase is implicated in development of tolerance to caspase-independent cell death. Mol Cell Biol 2000; 20: 6826–6836
- Hansen RK, Oesterreich S, Lemieux P, Sarge KD, Fuqua SA. Quercetin inhibits heat shock protein induction but not heat shock factor DNA-binding in human breast carcinoma cells. Biochem Biophys Res Commun 1997; 239: 851–856
- Lee YJ, Erdos G, Hou ZZ, et al. Mechanism of quercetin-induced suppression and delay of heat shock gene expression and thermotolerance development in HT-29 cells. Mol Cell Biochem 1994; 137: 141–154
- Goldberg SN, Kruskal JB, Oliver BS, Clouse ME, Gazelle GS. Percutaneous tumor ablation: Increased coagulation by combining radiofrequency ablation and ethanol instillation in a rat breast tumor model. Radiology 2000; 217: 827–831
- Liszczak TM, Hedley-Whyte ET, Adams JF, et al. Limitations of tetrazolium salts in delineating infarcted brain. Acta Neuropathol (Berl) 1984; 65: 150–157
- Goldberg SN, Girnan GD, Lukyanov AN, et al. Percutaneous tumor ablation: Increased necrosis with combined radio-frequency ablation and intravenous liposomal doxorubicin in a rat breast tumor model. Radiology 2002; 222: 797–804
- Liu Y, Wu X, Zhou H, Liu X, Zhang F, Yang J. The fluorescence enhancement of quercetin-nucleic acid system and the analytical application. Luminescence 2009; 24: 416–421
- Theriault JR, Adachi H, Calderwood SK. Role of scavenger receptors in the binding and internalization of heat shock protein 70. J Immunol 2006; 177: 8604– 8611
- Yang WL, Nair DG, Makizumi R, et al. Heat shock protein 70 is induced in mouse human colon tumor xenografts after sublethal radiofrequency ablation. Ann Surg Oncol 2004; 11: 399–406
- Schueller G, Kettenbach J, Sedivy R, et al. Heat shock protein expression induced by percutaneous radiofrequency ablation of hepatocellular carcinoma in vivo. Int J Oncol 2004; 24: 609–613
- Rai R, Richardson C, Flecknell P, Robertson H, Burt A, Manas DM. Study of apoptosis and heat shock protein (HSP) expression in hepatocytes following radiofrequency ablation (RFA). J Surg Res 2005; 129: 147–151
- Schueller G, Kettenbach J, Sedivy R, et al. Expression of heat shock proteins in human hepatocellular carcinoma after radiofrequency ablation in an animal model. Oncol Rep 2004; 12: 495–499
- Yang W, Ahmed M, Elian M, et al. Do Liposomal Apoptotic Enhancers IncreaseTumor Coagulation and End-Point Survival in Percutaneous Radiofrequency Ablation of Tumors in a Rat Tumor Model?. Radiology 2010; 257: 685–696
- Du G, Lin H, Wang M, et al. Quercetin greatly improved therapeutic index of doxorubicin against 4T1 breast cancer by its opposing effects on HIF-lalpha in tumor and normal cells. Cancer Chemother Pharmacol 2009; 65: 277–287
- Blachere NE, Li Z, Chandawarkar RY, et al. Heat shock protein-peptide complexes, reconstituted in vitro, elicit peptide-specific cytotoxic T lymphocyte response and tumor immunity. J Exp Med 1997; 186: 1315–1322
- den Brok, MH Sutmuller, RP Nierkens, S, et al. Efficient loading of dendritic cells following cryo and radiofrequency ablation in combination with immune modulation induces anti-tumour immunity. Br J Cancer 2006; 95: 896–905
- Dromi SA, Walsh MP, Herby S, et al. Radiofrequency ablation induces antigen-presenting cell infiltration and amplification of weak tumor-induced immunity. Radiology 2009; 251: 58–66
- Poon RT, Borys N. Lyso-thermosensitive liposomal doxorubicin: A novel approach to enhance efficacy of thermal ablation of liver cancer. Expert Opin Pharmacother 2009; 10: 333–343
- Frich L, Bjornerud A, Fossheim S, Tillung T, Gladhaug I. Experimental application of thermosensitive paramagnetic liposomes for monitoring magnetic resonance imaging guided thermal ablation. Magn Reson Med 2004; 52: 1302–1309