Abstract
Over the past 20 years advances in radiological technology have led to dramatic changes in cervical cancer management. External beam radiotherapy has evolved radically as a result of these changes. More recently, though, three-dimensional (3D) image guidance and volume-based prescribing has been adopted into cervical cancer brachytherapy. This overview covers the science and technology behind magnetic resonance imaging (MRI)-guided adaptive brachytherapy and explains why it is now considered the new international standard of care.
Introduction
Brachytherapy plays a fundamental role in the treatment of cervical carcinoma. Internationally, concomitant chemoradiation followed by brachytherapy is regarded as the standard of care for patients with tumours staged between IB2 and IVA. The addition of chemotherapy to external beam radiotherapy (EBRT) has been found to improve overall survival by approximately 12% Citation[1–3] but at the cost of increased gastrointestinal (GI) and haematological (only one use) toxicity. In patients unsuitable for concurrent chemoradiotherapy, studies examining the addition of hyperthermia to radiotherapy have shown improved local control rates and overall survival in patients with advanced tumours Citation[4], Citation[5]. The radiobiological and radiophysical principles of brachytherapy though allow a higher dose of radiation to be delivered to the cervical tumour whilst minimising toxicity to the organs at risk (OAR).
The principle of brachytherapy is that the radiation source is placed directly into the area to be treated thus delivering an intense high dose which dissipates rapidly according to the inverse square law. The procedure has been well developed over many years with traditional applicator systems which have subsequently been adapted for use with modern afterloading techniques. Afterloading is now universally employed allowing safe and accurate placement of applicators prior to radiation delivery and minimising the radiation exposure to staff and patients. Conventional applicator systems are based on a central intrauterine tube (the tandem) and a vaginal applicator which may be either a pair of ovoids within each fornix or a ring placed at the top of the vagina. These are usually rigid applicators, fixing together in a defined format which enables accurate and reproducible dose distributions. An alternative method which has been developed in Paris is to use a vaginal mould technique Citation[6], Citation[7]. This method involves the creation of a customized vaginal mould for each patient, which houses one intrauterine and two vaginal catheters. The position and length of the catheters within the mould are based upon the patient's anatomy and the size and extent of their tumour. Following insertion of the mould under general anaesthetic the patient is then scanned in the MRI scanner and the necessary planning volumes delineated.
The radiation can be delivered using either low dose rate (LDR), high dose rate (HDR) or pulsed dose rate brachytherapy (PDR). The dose rate refers to the rate at which radiation energy is release from the source and is expressed in Gray per hour (Gy/h). Although the equivalent prescribed biological dose is the same no matter which dose rate is used, a correction is required with a reduction in total physical dose as the dose rate increases, and for HDR, fractionated delivery of the dose over 3 or 4 fractions. Typically in LDR and PDR the source stays in situ for up to 24–48 h before being removed, while in HDR this time is typically a few minutes Citation[8]. Selection of dose rate depends upon the centre's brachytherapy equipment and staff experience, but LDR machines have now largely been phased out and modern practice is based on either HDR or PDR. Published outcomes using all three dose rates show high local control rates with acceptable levels of toxicity Citation[6], Citation[9], Citation[10]. It has been postulated that PDR brachytherapy allows a wider range of treatment optimisation and facilitates the treatment of a larger volume of tissue without increasing toxicity [10]. Lower rates of local failure when compared with LDR brachytherapy have been reported but will need to be confirmed with larger studies Citation[6].
The historical convention when delivering cervical brachytherapy is to prescribe the radiation dose to a point termed Point A and to use the International Commission on Radiation Units and Measurements (ICRU) Report 38 for OAR point dose reporting Citation[11]. The combination of this prescription convention with radiographic 2D imaging has consistently shown high local control rates for early stage disease, but unfortunately these rates reduce significantly when the disease becomes bulkier and more advanced Citation[12]. Studies have shown that by escalating the brachytherapy dose delivered using conventional non-adaptive 2D X-ray imaging there is an improvement in local control rates but at the cost of significantly higher rates of toxicity Citation[13–16]. Interest has therefore grown in discovering ways to improve local control while maintaining or reducing existing OAR toxicity. This has led to the development of high-precision computed tomography (CT) and MRI-guided volume-based brachytherapy for carcinoma of the cervix.
Search strategies used and sources of information
PubMed was searched for articles published before January 2012 containing the following terms: cervix cancer, cervical cancer, brachytherapy, MRI-guided, Royal College of Radiologists, RCR, CT, PET, and MRI. All relevant and any related articles were then fully reviewed.
Imaging technologies used in treating cervical cancer
Important prognostic factors associated with cervical carcinoma are tumour size, tumour extension and nodal involvement Citation[17–19]. Hence radiological imaging in combination with physical examination plays a vital role in the diagnosis, treatment and follow-up of patients with carcinoma of the cervix. This applies at every step of the management process and embraces ultrasound (US), CT, MRI and positron emission tomography (PET). Over the past 20 years there has been a significant evolution in the application of imaging technology to the planning and delivery of radiotherapy for cervical carcinoma.
Since the early 1990s CT has been adopted into the routine planning and delivery of EBRT. This has led to the creation of what is termed conformal radiotherapy which describes a volume-based approach to EBRT planning whereby the tumour and other clinically important areas are radiologically visualised and delineated on the CT to create 3D volumes. ICRU Reports 50, 62, 71, and 78 define the structures and terminology used to represent and report these volumes Citation[20–23]. The structures normally delineated on the CT planning scan are the gross tumour volume (GTV), clinical target volume (CTV), planning target volume (PTV) and OAR. Once these volumes have been delineated on the planning CT then advanced planning software is used to shape the radiotherapy dose so that it conforms to the PTV.
This volume-based approach has been shown to improve dose homogeneity across the tumour while helping to minimise unwanted normal tissue toxicity by the adoption of volumetric dose constraints for OAR Citation[24], Citation[25]. In one study comparing 3D conformal radiotherapy planning with older conventional radiotherapy planning, the dose received by the critical organs, the rectum and bowel, was approximately 50% lower (96.82% versus 38.23% and 61.37% versus 30.79% respectively) Citation[26].
Despite the benefits of CT leading to significant advances in EBRT planning and delivery it still remains inferior to MRI with regard to soft tissue visualisation. With MRI it is possible to alter the contrast of different tissues to improve tissue plane visualisation and detect subtle abnormalities. In cervical carcinoma this means that the relationship of the tumour to the uterus and vagina can be accurately determined and any early invasion of the parametria identified. Compared with CT, MRI has been evaluated as the more accurate imaging modality for cervical cancer staging, 86% versus 63% respectively Citation[27], with a sensitivity of 93% for early tumour detection and an overall staging accuracy of 86% Citation[28]. MRI staging accuracy has also been assessed by comparing it with findings found at the time of surgery. MRI was found to accurately estimate tumour size to within ±5 mm and correctly identify parametrial invasion in 88% of cases Citation[29–31].
Although MRI offers a distinct advantage over CT in terms of tumour visualisation it has not replaced CT in EBRT planning. MRI relies upon the signals derived from different water densities in tissue so unlike CT it does not measure the electron density of tissues. Also, due to the low water density of cortical bone, MRI cannot accurately detect a signal from it. Because of these differences MRI cannot be used in EBRT planning because it lacks the information required for dose calculations. Hence the data provided by CT is still regarded as vital in EBRT treatment planning.
In brachytherapy planning however, tissue electron density data is of less importance since dosimetry is dependent primarily upon the inverse square law. Consequently MRI can be adopted directly for brachytherapy using its superior tumour visualisation. Dimopoulos et al. demonstrated that, unlike CT, MRI for brachytherapy planning permitted the precise delineation of the GTV, CTV, OAR and applicators Citation[32]. Hence there has been a transition from Point A-based 2D dosimetry to MR-based 3D dosimetry for cervical cancer which has required significant changes to methods of dose prescribing and reporting.
An introduction to MRI-guided adaptive brachytherapy
The first reports on the use of 3D imaging in pelvic brachytherapy were in the late 1980s Citation[33]. At the time clinicians were using CT-based imaging to calculate brachytherapy dose data for the tumour and OAR. With the advent of 3D conformal EBRT, a similar approach was being explored in brachytherapy which would allow dose escalation while minimising unwanted toxicity. Early proponents of MRI-guided brachytherapy showed using small case series that this technique could offer those potential benefits Citation[34–37].
These early studies revealed that the traditional method of using point doses rather than volumes for dose prescription and reporting was unsatisfactory. The studies which had used CT-based imaging to facilitate brachytherapy had discovered that when the dose delivered to point A using the CT technique was compared with the dose delivered to point A using the traditional radiographic technique that the traditional technique could lead to tumour under-dosage Citation[38], Citation[39]. Kim et al. discovered that the traditional radiographic method of prescribing to point A overestimated the dose received by the tumour and that the tumour stage seemed to be inversely proportional to the volume of GTV covered by the prescription dose. By examining the dose volume histograms created using their CT-based brachytherapy data they reported that for FIGO stages IB1, IB2, IIB and IIIB the dose prescribed to point A encompassed on average 98.5%, 89.5%, 79.5% and 59.5% of the GTV volume respectively Citation[39]. These studies also revealed that the traditional method of using ICRU Report 38 to report the OAR doses was inaccurate and underestimated the maximum dose received by the bladder and rectum when compared with the new CT volume-based OAR data Citation[38], Citation[40]. As a result a new system of tumour voluming, dose prescription and dose reporting was developed to replace the traditional point-based system Citation[41], Citation[42].
Defining standards in image-guided brachytherapy
These developments led to the publication of several different recommendations and guidelines. Following the American Brachytherapy Society's 2004 recommendations Citation[43] the Groupe Européen de Curiethérapie-European Society for Therapeutic Radiology and Oncology (GEC-ESTRO) working group published their own recommendations in 2005 and 2006 Citation[41], Citation[42]. Their published recommendations covered all aspects of tumour target and OAR contouring and provided guidance on the reporting of dose volume parameters for image-guided brachytherapy (IGBT). Their recommendations defined an individualised approach to cervical brachytherapy whereby image guidance was used to help conform the dose to the tumour volume and pelvic topography while balancing the organ at risk dose volume constraints Citation[41], Citation[42]. In 2005 it was agreed internationally that the GEC-ESTRO recommendations would form the basis of future work in gynaecological IGBT Citation[44].
GEC-ESTRO sets out clear recommendations with regard to tumour and OAR voluming. They advocate the delineation of three specific volumes relating to the tumour: GTV, high risk (HR) CTV, intermediate risk (IR) CTV and OAR. The organs at risk are defined as the rectum, bladder, sigmoid colon and any adjacent bowel loops which are considered relevant to the treatment target volume. As is the case with conformal EBRT, it is hypothesised that the risk of microscopic disease varies with tumour cell density. Consequently the GTV, HR-CTV and IR-CTV are considered as having different reducing radiotherapy dose requirements based on this hypothesis.
At the time of brachytherapy planning, the patient is scanned in the MRI scanner with the brachytherapy applicators in situ. Then, using the clinical examination findings, initial MRI staging information and the brachytherapy imaging data, the treating clinician delineates these volumes on the planning scan. Any areas of residual palpable disease which are visible as areas of high signal intensity on the T2 weighted planning MRI scan are delineated and defined as the HR-CTV. Any areas at high risk of containing tumour cells are also included in the HR-CTV. These are characteristically described as ‘grey zones’ on the MRI scan and represent areas of disease regression following chemoradiotherapy.
The IR-CTV is normally determined by the original volume of the tumour and any initial pelvic extension. Typically it is defined by a margin of 10–15 mm around the HR-CTV Citation[41]. Once the HR and IR-CTV's have been volumed then the clinician creates volumes for the OARs and the applicators in situ which are tracked and defined ().
Figure 1. The image shows an axial slice from a T2 weighted MRI-guided adaptive brachytherapy planning scan. Delineated on the scan are the GTV (green), HR-CTV (red), IR-CTV (blue) and OAR (sigmoid colon (yellow), bladder (purple) and rectum (green)).
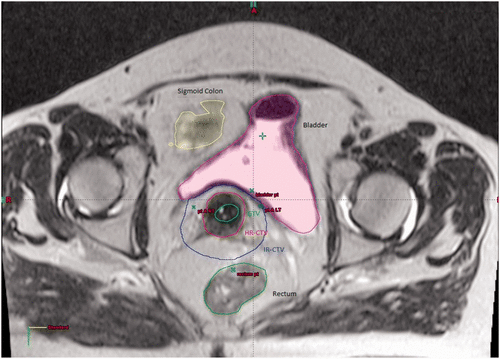
Once these planning volumes have been created it is then the role of the brachytherapy physicist to determine an appropriate radiation delivery strategy. The aim of treatment planning is to ensure that the HR-CTV receive an adequate dose to the D90 (dose to 90% of the HRCTV) while observing the OAR dose constraints. Delivery of an equivalent dose in 2 Gy per fraction (EQD2) of ≥87 Gy to the HR-CTV D90 is recommended as this has been shown by Dimopoulos et al. to correlate with a local pelvic control rate of >90% Citation[39], Citation[45]. If it is not possible to cover the HR-CTV D90 volume adequately using a standard delivery technique e.g. using a tandem ring applicator, then it has been shown that the addition of parametrial interstitial needles to the intracavity treatment can improve dose coverage and homogeneity Citation[46], Citation[47] ().
Figure 2. Axial and sagittal T2 weighted images from two different patients MRI planning scans. The top two images show the dose wash from a standard tandem ring applicator technique (red, high dose; blue, low dose). The bottom two images demonstrate how the addition of interstitial needles to the standard tandem ring applicator technique can help conform the dose around the IR-CTV (note how the interstitial needles on the right and left help extend the high dose coverage out towards the pelvic side walls).
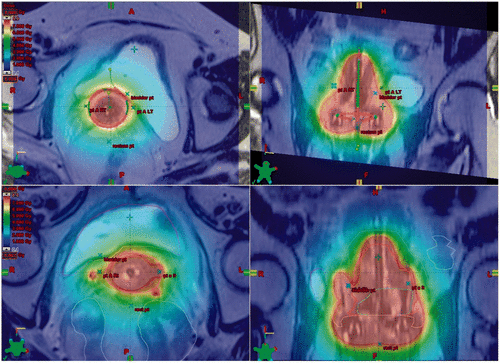
Adequate coverage of the HR-CTV D90 must be balanced against achieving the OAR dose constraints. It is well recognised from historical case series using 2D non-adaptive treatment techniques that the incidence of late radiation toxicity is determined by the total radiation dose delivered to the pelvic organs, the volume of normal tissue treated and the rate of dose delivery Citation[48–51].
The GEC-ESTRO recommendations changed the way in which OAR doses were reported by recommending the use of dose volume histograms (DVH). Now that OAR structures were volumed it was possible to calculate the maximum dose received by 2 cm3, 1 cm3 and 0.1 cm3 of OAR tissue. GEC-ESTRO termed these volumes as D2cc, D1cc and D0.1cc and defined them as the maximum dose received by that specified volume of OAR tissue Citation[41], Citation[42].
Recently, Georg et al. published work correlating the DVH parameters defined by GEC ESTRO with OAR toxicity following chemoradiotherapy and MRI-guided adaptive brachytherapy. They found that the D2cc had good predictive value for late rectal and bladder toxicities graded II–IV Citation[52]. They had difficulty finding a correlation between D2cc and sigmoid colon toxicity owing to a paucity of bowel toxicity events within their data series Citation[53].
Based on the GEC-ESTRO recommendations and more recently published reports, the current dose constraints are 70–75 Gy for the rectum and sigmoid colon and 85–90 Gy for the bladder Citation[12], Citation[42], Citation[52], Citation[53].
The future of MRI-guided adaptive brachytherapy
MRI-guided adaptive brachytherapy is now considered the gold standard technique for treating cervical carcinoma and is recommended in numerous published guidelines Citation[41], Citation[42], Citation[44], Citation[54–56]. Pötter et al. published an update on their MRI-guided brachytherapy patient series (n = 156) in 2011 which included details on local control and toxicity rates. They reported a complete remission rate of 97% (151/156) with local control rates at 3 years of 95% overall and 98% for tumours 2–5 cm and 92% for tumours >5 cm Citation[57]. Notably, the improved local control rates seen for tumours >5 cm (92% versus 67%/64% from the Vienna historical series Citation[9], Citation[58]) seemed to impact on cancer-specific survival (CSS) rates at 3 years. The CSS rate improved from 57% in 1993–1997 and 40% in 1998–2000 to 70% in 2001–2008 Citation[9], Citation[57], Citation[58]. The CSS rate at 3 years for tumours 2–5 cm remained unchanged during the same time period. They advised caution in drawing conclusions from this trend because their length of follow up was only 3 years and might be explained by a number of other important factors and not just adaptive brachytherapy. Nevertheless it is an important finding that will need to be investigated through future prospective studies.
Despite these recently published findings and those of several other smaller international series which have shown improvements in local control rates (∼20%) without any significant increase in toxicity Citation[10], Citation[46], Citation[59], MRI-guided brachytherapy still remains a new and relatively under-utilised approach.
In the UK the Royal College of Radiologists published recommendations on the implementation of image-guided brachytherapy in 2009. Since their publication there has been a significant increase in the number of UK centres using image-guided brachytherapy techniques. Tan et al. surveyed UK oncology centres in both 2008 and 2011 and found a 27% increase in centres using some form of 3D imaging (51% versus 78%) and a 16% increase (4% versus 20%) in centres using MRI-guided brachytherapy Citation[60]. This would suggest that certainly in the UK clinicians are adopting image-guided treatment techniques into the management of cervical carcinoma.
In 2008 an international collaborative study named EMBRACE [61] was launched which aims at improving MRI-guided brachytherapy implementation and quality assurance across the globe. Through prospective data collection on local control rates and toxicity it hopes to further validate the role of MRI-guided brachytherapy in the management of cervical cancer using the GYN-GEC-ESTRO recommendations. Thus far it has collected data on over 600 treated patients and is on track to reach the target recruitment number. It is hoped that the findings when published will reinforce the future of MRI-guided adaptive brachytherapy in gynaecological brachytherapy. Needless to say their final results are eagerly awaited by the radiation oncology gynaecological community.
Declaration of interest: The authors report no conflicts of interest. The authors alone are responsible for the content and writing of the paper.
References
- Green J, Kirwan J, Tierney J, Vale C, Symonds P, Fresco L, et al. Concomitant chemotherapy and radiation therapy for cancer of the uterine cervix. Cochrane Database Syst Rev 2005; 3: CD002225
- Thomas GM. Improved treatment for cervical cancer – Concurrent chemotherapy and radiotherapy. New Engl J Med 1999; 340: 1198–1200
- Vale C. Reducing uncertainties about the effects of chemoradiotherapy for cervical cancer: A systematic review and meta-analysis of individual patient data from 18 randomized trials. J Clin Oncol 2008; 26: 5802–5812
- Lutgens L, van der Zee J, Pijls-Johannesma M, De Haas-Kock DF, Buijsen J, Mastrigt GA, et al. Combined use of hyperthermia and radiation therapy for treating locally advanced cervix carcinoma. Cochrane Database Syst Rev 2010; 3: CD006377
- van der Zee J, Gonzalez Gonzalez D, van Rhoon GC, van Dijk JD, van Putten WL, Hart AA. Comparison of radiotherapy alone with radiotherapy plus hyperthermia in locally advanced pelvic tumours: A prospective, randomised, multicentre trial. Dutch Deep Hyperthermia Group. Lancet 2000; 355: 1119–1125
- Haie-Meder C, Chargari C, Rey A, Dumas I, Morice P, Magne N. MRI-based low dose-rate brachytherapy experience in locally advanced cervical cancer patients initially treated by concomitant chemoradiotherapy. Radiother Oncol 2010; 96: 161–165
- Georg D, Kirisits C, Hillbrand M, Dimopoulos J, Potter R. Image-guided radiotherapy for cervix cancer: High-tech external beam therapy versus high-tech brachytherapy. Int J Radiat Oncol Biol Phys 2008; 71: 1272–1278
- Hoskin PJ, Coyle C. Radiotherapy in Practice: Brachytherapy. Oxford University Press, Oxford 2005
- Pötter R, Dimopoulos J, Georg P, Lang S, Waldhausl C, Wachter-Gerstner N, et al. Clinical impact of MRI assisted dose volume adaptation and dose escalation in brachytherapy of locally advanced cervix cancer. Radiother Oncol 2007; 83: 148–155
- Chargari C, Magne N, Dumas I, Messai T, Vicenzi L, Gillion N, et al. Physics contributions and clinical outcome with 3D-MRI-based pulsed-dose-rate intracavitary brachytherapy in cervical cancer patients. Int J Radiat Oncol Biol Phys 2009; 74: 133–139
- International Commission on Radiation Units and Measurements (ICRU) Report 38. Dose and Volume Specification for Reporting Intracavitary Therapy in Gynecology. ICRU, Bethesda, MD 1985
- Gerbaulet A, Potter R, Haie-Meder C. The GEC ESTRO handbook of brachytherapy. GEC ESTRO, Brussels 2002
- Perez CA, Breaux S, Madoc-Jones H, Camel HM, Purdy J, Sharma S, et al. Correlation between radiation dose and tumor recurrence and complications in carcinoma of the uterine cervix: Stages I and IIA. Int J Radiat Oncol Biol Phys 1979; 5: 373–382
- Perez CA, Fox S, Lockett MA, Grigsby PW, Camel HM, Galakatos A, et al. Impact of dose in outcome of irradiation alone in carcinoma of the uterine cervix: Analysis of two different methods. Int J Radiat Oncol Biol Phys 1991; 21: 885–898
- Perez CA, Grigsby PW, Chao KS, Mutch DG, Lockett MA. Tumor size, irradiation dose, and long-term outcome of carcinoma of uterine cervix. Int J Radiat Oncol Biol Phys 1998; 41: 307–317
- Sinistrero G, Sismondi P, Rumore A, Zola P. Analysis of complications of cervix carcinoma treated by radiotherapy using the Franco-Italian glossary. Radiother Oncol 1993; 26: 203–211
- Barillot I, Horiot JC, Maingon P, Bone-Lepinoy MC, D'Hombres A, Comte J. Carcinomes du col uterin localement avances (II distaux, barillet et III) traites par irradiation exclusive: Impact de la planification individuelle des raitements sur les resultats, les sequelles et les complications. [Locally advanced carcinoma of the cervix (distal II, barrel-shaped and III) treated by irradiation alone: Impact of individual treatment planning on results, sequelae and complications]. Bull Cancer Radiother 1996; 83: 364–369
- Gerbaulet A, Haie C, Michel G, Castaigne D, Prade M, Kunkler I, et al. Combined radio-surgical treatment in early invasive cervix carcinoma according to prognostic factors. Experience of the Gustave-Roussy Institute. Eur J Gynaecol Oncol 1992; 13: 256–261
- Perez CA, Grigsby PW, Nene SM, Camel HM, Galakatos A, Kao MS, et al. Effect of tumor size on the prognosis of carcinoma of the uterine cervix treated with irradiation alone. Cancer 1992; 69: 2796–2806
- International Commission on Radiation Units and Measurements (ICRU). Prescribing, Recording and Reporting Photon Beam Therapy. ICRU, Bethesda, MD 1993, Report 50
- International Commission on Radiation Units and Measurements (ICRU). Prescribing, Recording and Reporting Photon Beam Therapy. ICRU, Bethesda, MD 1999, Supplement to Report 50
- International Commission on Radiation Units and Measurements (ICRU). Prescribing, Recording and Reporting Photon Beam Therapy. Oxford University Press, Oxford 2004, Report 50
- International Commission on Radiation Units and Measurements (ICRU). Prescribing, Recording and Reporting Photon Beam Therapy. Oxford University Press, Oxford 2007, Report 50
- Yamazaki A, Shirato H, Nishioka T, Hashimoto S, Kitahara T, Kagei K, et al. Reduction of late complications after irregularly shaped four-field whole pelvic radiotherapy using computed tomographic simulation compared with parallel-opposed whole pelvic radiotherapy. Jap J Clin Oncol 2000; 30: 180–184
- Taylor A, Powell ME. Conformal and intensity-modulated radiotherapy for cervical cancer. Clin Oncol 2008; 20: 417–425
- Włodarczyk H, Roszak A, Cikowska-Woźniak E, Bratos K, Wojciechowska-Łącka A, Skrobała A. Does conformal therapy improve dose distribution in comparison to old techniques in teleradiotherapy of cervical cancer patients?. Rep Pract Oncol Radiother 2008; 13: 195–200
- Pannu HK, Corl FM, Fishman EK. CT evaluation of cervical cancer: Spectrum of disease. Radiographics 2001; 21: 1155–1168
- Bipat S, Glas AS, van der Velden J, Zwinderman AH, Bossuyt PM, Stoker J. Computed tomography and magnetic resonance imaging in staging of uterine cervical carcinoma: A systematic review. Gynecol Oncol 2003; 91: 59–66
- Hricak H, Lacey CG, Sandles LG, Chang YC, Winkler ML, Stern JL. Invasive cervical carcinoma: Comparison of MR imaging and surgical findings. Radiology 1988; 166: 623–631
- Subak LL, Hricak H, Powell CB, Azizi L, Stern JL. Cervical carcinoma: Computed tomography and magnetic resonance imaging for preoperative staging. Obstet Gynecol 1995; 86: 43–50
- Kaur H, Silverman PM, Iyer RB, Verschraegen CF, Eifel PJ, Charnsangavej C. Diagnosis, staging, and surveillance of cervical carcinoma. Am J Roentgenol 2003; 180: 1621–1631
- Dimopoulos JC, Schard G, Berger D, Lang S, Goldner G, Helbich T, et al. Systematic evaluation of MRI findings in different stages of treatment of cervical cancer: Potential of MRI on delineation of target, pathoanatomic structures, and organs at risk. Int J Radiat Oncol Biol Phys 2006; 64: 1380–1388
- Ling CC, Schell MC, Working KR, Jentzsch K, Harisiadis L, Carabell S, et al. CT-assisted assessment of bladder and rectum dose in gynecological implants. Int J Radiat Oncol Biol Phys 1987; 13: 1577–1582
- Schoeppel SL, Ellis JH, LaVigne ML, Schea RA, Roberts JA. Magnetic resonance imaging during intracavitary gynecologic brachytherapy. Int J Radiat Oncol Biol Phys 1992; 23: 169–174
- Pötter R, Fidarova E, Kirisits C, Dimopoulos J. Image-guided adaptive brachytherapy for cervix carcinoma. Clin Oncol 2008; 20: 426–432
- Tardivon AA, Kinkel K, Lartigau E, Masselot J, Gerbaulet AP, Vanel D. MR imaging during intracavitary brachytherapy of vaginal and cervical cancer: Preliminary results. Radiographics 1996; 16: 1363–1370
- Pötter R, Kovacs G, Lenzen B. Technique of MRI assisted brachytherapy treatment planning. Activity. Select Brachyther J 1991; 5: 145–148
- Datta NR, Srivastava A, Maria Das KJ, Gupta A, Rastogi N. Comparative assessment of doses to tumor, rectum, and bladder as evaluated by orthogonal radiographs vs computer enhanced computed tomography-based intracavitary brachytherapy in cervical cancer. Brachytherapy 2006; 5: 223–229
- Kim RY, Pareek P. Radiography-based treatment planning compared with computed tomography (CT)-based treatment planning for intracavitary brachytherapy in cancer of the cervix: Analysis of dose-volume histograms. Brachytherapy 2003; 2: 200–206
- Schoeppel SL, LaVigne ML, Martel MK, McShan DL, Fraass BA, Roberts JA. Three-dimensional treatment planning of intracavitary gynecologic implants: Analysis of ten cases and implications for dose specification. Int J Radiat Oncol Biol Phys 1994; 28: 277–283
- Haie-Meder C, Potter R, Van Limbergen E, Briot E, De Brabandere M, Dimopoulos J, et al. Recommendations from Gynaecological (GYN) GEC-ESTRO Working Group (I): Concepts and terms in 3D image based 3D treatment planning in cervix cancer brachytherapy with emphasis on MRI assessment of GTV and CTV. Radiother Oncol 2005; 74: 235–245
- Pötter R, Haie-Meder C, Van Limbergen E, Barillot I, De Brabandere M, Dimopoulos J, et al. Recommendations from gynaecological (GYN) GEC ESTRO working group (II): Concepts and terms in 3D image-based treatment planning in cervix cancer brachytherapy-3D dose volume parameters and aspects of 3D image-based anatomy, radiation physics, radiobiology. Radiother Oncol 2006; 78: 67–77
- Nag S, Cardenes H, Chang S, Das IJ, Erickson B, Ibbott GS, et al. Proposed guidelines for image-based intracavitary brachytherapy for cervical carcinoma: Report from Image-Guided Brachytherapy Working Group. Int J Radiat Oncol Biol Phys 2004; 60: 1160–1172
- Nag S. Controversies and new developments in gynecologic brachytherapy: Image-based intracavitary brachytherapy for cervical carcinoma. Sem Radiat Oncol 2006; 16: 164–167
- Dimopoulos J, Georg P, Kirisits C, Lang S, Berger D, Pötter R. The predictive value of dose volume parameters on local tumor control in MRI assisted brachytherapy of cervix cancer. Radiother Oncol 2006; 81: 539
- Dimopoulos JC, Kirisits C, Petric P, Georg P, Lang S, Berger D, et al. The Vienna applicator for combined intracavitary and interstitial brachytherapy of cervical cancer: Clinical feasibility and preliminary results. Int J Radiat Oncol Biol Phys 2006; 66: 83–90
- Kirisits C, Lang S, Dimopoulos J, Berger D, Georg D, Potter R. The Vienna applicator for combined intracavitary and interstitial brachytherapy of cervical cancer: Design, application, treatment planning, and dosimetric results. Int J Radiat Oncol Biol Phys 2006; 65: 624–630
- Perez CA, Breaux S, Bedwinek JM, Madoc-Jones H, Camel HM, Purdy JA, et al. Radiation therapy alone in the treatment of carcinoma of the uterine cervix. II. Analysis of complications. Cancer 1984; 54: 235–246
- Barillot I, Horiot JC, Maingon P, Truc G, Chaplain G, Comte J, et al. Impact on treatment outcome and late effects of customized treatment planning in cervix carcinomas: Baseline results to compare new strategies. Int J Radiat Oncol Biol Phys 2000; 48: 189–200
- Perez CA, Grigsby PW, Lockett MA, Chao KS, Williamson J. Radiation therapy morbidity in carcinoma of the uterine cervix: Dosimetric and clinical correlation. Int J Radiat Oncol Biol Phys 1999; 44: 855–866
- Chen SW, Liang JA, Yang SN, Liu RT, Lin FJ. The prediction of late rectal complications following the treatment of uterine cervical cancer by high-dose-rate brachytherapy. Int J Radiat Oncol Biol Phys 2000; 47: 955–961
- Georg P, Potter R, Georg D, Lang S, Dimopoulos JC, Sturdza AE, et al. Dose effect relationship for late side effects of the rectum and urinary bladder in magnetic resonance image-guided adaptive cervix cancer brachytherapy. Int J Radiat Oncol Biol Phys 2012; 82: 653–657
- Georg P, Pötter R, Georg D, Berger D, Lang S, Dimopoulos JC, et al. Dose–volume histogram parameters and late side effects in magnetic resonance image–guided adaptive cervical cancer brachytherapy. Int J Radiat Oncol Biol Phys 2011; 79: 356–362
- Bidmead M, Briot E, Burger J, Ferreira I, Grusell E, Kirisits C, et al., A practical guide to quality control of brachytherapy equipment, J. Venselaar and J. Perez-Calatayud, Editors. 2004, ESTRO Publications.
- Board of the Faculty of Clinical Oncology. Implementing Image-Guided Brachytherapy for Cervix Cancer in the UK. Royal College of Radiologists, London 2009
- Barillot I, Reynaud-Bougnoux A. The use of MRI in planning radiotherapy for gynaecological tumours. Cancer Imaging 2006; 6: 100–106
- Pötter R, Georg P, Dimopoulos JC, Grimm M, Berger D, Nesvacil N, et al. Clinical outcome of protocol based image (MRI) guided adaptive brachytherapy combined with 3D conformal radiotherapy with or without chemotherapy in patients with locally advanced cervical cancer. Radiother Oncol 2011; 100: 116–123
- Pötter R, Knocke TH, Fellner C, Baldass M, Reinthaller A, Kucera H. Definitive radiotherapy based on HDR brachytherapy with iridium 192 in uterine cervix carcinoma: Report on the Vienna University Hospital findings (1993–1997) compared to the preceding period in the context of ICRU 38 recommendations. Cancer Radiother 2000; 4: 159–172
- Tan LT, Coles CE, Hart C, Tait E. Clinical impact of computed tomography-based image-guided brachytherapy for cervix cancer using the tandem-ring applicator – The Addenbrooke's experience. Clin Oncol 2009; 21: 175–182
- Tan LT. Implementation of image-guided brachytherapy for cervix cancer in the UK: Progress update. Clin Oncol 2011; 23: 681–684
- EMBRACE Trial Website. Available from: https://www.embracestudy.dk/