Abstract
Purpose: This paper examines X-ray CT, to serve as an image-guiding thermal monitoring modality for high intensity focused ultrasound (HIFU) treatment of fatty tissues. Materials and methods: Six ex vivo porcine fat tissue specimens were scanned by X-ray CT simultaneously with the application of HIFU. Images were acquired during both heating and post-ablation stages. The temperature at the focal zone was measured simultaneously using a thermocouple. The mean values of the Hounsfield units (HU) at the focal zone were registered and plotted as a function of temperature. Results: In all specimens studied, the HU versus temperature curves measured during the heating stage depicted a characteristic non-linear parabolic trajectory (R2 > 0.87). The HU-temperature trajectory initially decreased to a minimum value at about 44.5 °C and then increased substantially as the heating progressed. The occurrence of this nadir point during the heating stage was clearly detectable. During post-ablation cooling, on the other hand, the HU increased monotonically with the decreasing temperature and depicted a clearly linear trajectory (R2 ≥ 0.9). Conclusions: Our results demonstrate that the HU-temperature curve during HIFU treatment has a characteristic parabolic trajectory for fat tissue that might potentially be utilised for thermal monitoring during HIFU ablation treatments. The clear detection of 44.5 °C, presumably marking the onset of hyperthermic injury, can be detected non-invasively as an occurrence of a minimum on the HU-time curve without any need to relate the HU directly to temperature. Such features may be helpful in monitoring and optimising HIFU thermal treatment for clinically applicable indications such as in the breast by providing a non-invasive monitoring of tissue damage.
Introduction
High intensity focused ultrasound (HIFU) treatments for non-invasive tissue ablation have become widespread for many applications in recent years, including for the treatment of fibroadenomas and other breast pathology [Citation1–3]. This may be mainly attributed to the ability of ultrasound waves to selectively heat tissue regions within the body without damaging surrounding tissues and healthy organs located along the acoustic path. The resulting temperature elevation at the focal zone leads to tissue destruction in fat [Citation4] as well as other tissue types [Citation5]. The evolving focusing capabilities of the ultrasound beam today allows high precision and localising effect of the acoustic wave energy. Yet, thermal monitoring in such treatments would be desired for safety and efficiency [Citation6].
Currently, magnetic resonance imaging (MRI) is the only US Food and Drug Administration approved modality for HIFU treatment thermal monitoring [Citation7–9], but some research has demonstrated that MRI thermometry based on proton resonance frequency shift (PRFS) is prone to inaccuracy in some tissues such as fat [Citation10–12]. This may be particularly important in breast tumour HIFU treatments [Citation4], where the surrounding tissue is mainly comprised of fatty tissue. In such procedures an indication of the tumour boundary destruction may be of utmost importance in indicating the success of the treatment. Another motivation for investigating thermal monitoring of HIFU application to fat tissue stems from the growing market for non-invasive body contouring where fat tissue is ablated for cosmetic reasons [Citation13,Citation14].
X-ray CT does not share MRI’s three major disadvantages, which are: (1) a relatively expensive imaging modality, (2) very restrictive RF shielding requirements, and (3) limited access to the patient. Hence, assuming of course that radiation levels are acceptable, X-ray CT could be a beneficial modality which could provide an alternative method for effective and non-invasive thermometry, in fat as well as in other types of tissues. Moreover, CT is a much more cost-effective modality that also allows excellent spatial and temporal resolution imaging.
Changes of the X-ray CT Hounsfield unit (HU) intensity stemming from temperature changes are already known [Citation15–17]. However, most previous studies have commonly attempted to relate the HU to temperature. Nonetheless, additional information regarding the onset of hyperthermic injury and the condition of the tissue, particularly at or about when ablation/protein denaturation occurs, would be desirable for improved and more comprehensive monitoring of tissue condition during a thermal treatment [Citation18]. Accordingly, this study was designed to explore the potential of X-ray CT to non-invasively detect the temperature at which the onset of hyperthermic injury and significant degradation in cell viability of fat tissue occurs [Citation4,Citation19], and hence serve as an imaging tool for ablation monitoring.
Materials and methods
Experimental set-up
The experimental set-up comprised a special apparatus for holding the tissue specimen along with a HIFU system and a thermocouple (see ), positioned atop the bed of a CT scanner. All the scans were acquired with a single source dual energy multi-slice CT machine (Philips Healthcare, Cleveland, OH), and the images were reconstructed using the full spectrum. All the axial scans were acquired using the same scanning protocol: tube voltage 120 kVp, tube current 500 mA, scan coverage 2 cm, reconstructed slice thickness 2.5 mm, rotation time 1 s/rotation, scan resolution standard, reconstruction filter B (standard), image matrix size 512 × 512 pixels, image field of view 250 mm. These scanning parameters are commonly used in clinical applications such as biopsies [Citation20] except for the higher current which was chosen in this experiment in order to improve signal-to-noise ratio.
Figure 1. The experimental apparatus was assembled from an ultrasonic thermal therapy transducer (A), which transmitted waves through a focusing lens (B) such that its focus zone would be located at the mid depth of the fat specimen (C). A vertical slider (D) mounted on a lateral slider (E) was used to position the probe (F) at the focal zone.
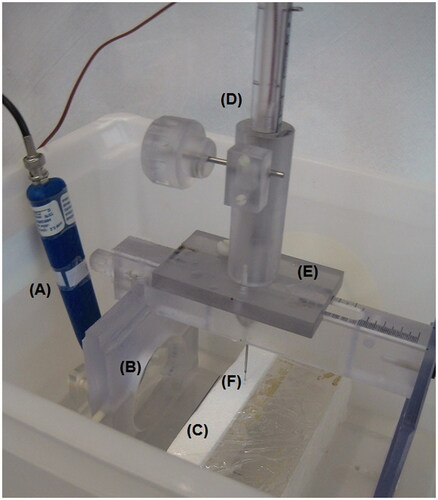
HIFU heating was accomplished using an ultrasonic thermal therapy device (Mettler Electronics Sonicator 730, Anaheim, CA). The device operated at 3 MHz frequency using a 5 cm2 applicator set for continuous wave transmission and maximal intensity of 2.2 W/cm2. A Plexiglas concave acoustic lens was mounted on top of the applicator, to focus the ultrasonic beam at a distance of 80 mm.
As porcine tissue is a good model for human tissue [Citation18], six specimens of ex vivo porcine subcutaneous fat tissue samples purchased at a local butcher were studied. The specimens were fresh (less than 24 h), and kept refrigerated. Each specimen was cut into a block of about 20 mm in thickness, 20 cm in width and 17 cm in height. It was placed inside the apparatus as shown in . The tissue was strapped to a plastic block to prevent it from floating and then submerged in water. The thermocouple probe was then inserted into the centre of the planned HIFU focal zone and the temperature was measured at 1 s intervals throughout the entire experiment. The specially designed apparatus was used to position the tissue specimen in front of the HIFU beam in such a way that the beam focal zone would be located at the mid depth of the specimen. The apparatus was built from polycarbonate and Plexiglas parts and was designed to position the HIFU lens in place. A ‘bridge’ between the lens wall and its opposite wall (the rear wall of the box) enabled the horizontal movement of a detachable guiding element to hold a thermocouple probe (TJC36-CPSS-020U-6-SMP-M, Omega Engineering, Manchester, UK) along the ultrasonic beam axis. The guiding element allowed the thermocouple probe to be left inside the tissue specimen by using a ratchet wheel to control the vertical movement of the conductor for the thermocouple, which could also be retracted. This facilitated precise positioning of the tip of the probe at the HIFU focal zone. Finally, the assembly was placed atop the X-ray CT scanner bed.
Experimental procedure
For each of the fat specimens, after the specimen and thermocouple probe were correctly positioned, HIFU was activated to heat the tissue for a predetermined duration (which was varied over the experiments in order to achieve different thermal doses). Then the HIFU was turned off, and after sufficient cooling time (at least 30 min) the specimen was repositioned laterally by about 5 cm to retain the same distance from the HIFU and similar conditions, but with an untreated, fresh portion of tissue located at the HIFU focal zone (to avoid re-heating the same location). Following this step, the tissue was heated and cooled again under the exact same conditions as before but this time without inserting the thermocouple probe. This was done in order to obtain a set of images without any image artefacts stemming from the thermocouple probe tip. Each tissue was heated only twice, once with the thermocouple probe and once without (on a fresh part of it).
Reference temperature measurements, as well as a reference set of images, were acquired prior to HIFU sonication. These images served as a baseline for measuring the HU changes stemming from the heating and post-ablation stages. X-ray CT scanning was performed simultaneously during the heating and post-ablation stages. Scan interval was 10–15 s during the first 3 min, and 29 s intervals during the rest of the heating and post-ablation. The HIFU was activated for different durations (up to 29 min) for each of the studied specimens to obtain different maximal temperature, ranging from 32 °C to about 60 °C. Scanning continued for an additional 30–45 min, after the termination of the sonication, to obtain images of the post-ablation cooling stage as well. CT scan acquisition times as well as the activation times of the HIFU were registered, to enable the synchronisation of the scan time with the temperature measurements acquired by the thermocouple.
A selected small region of interest (ROI) located at the focal zone was defined, and the mean HU within that ROI were then calculated and plotted versus time. The temperature was recorded by a thermocouple probe to allow data integration and plot curves of HU versus temperature.
Data analysis
HU–temperature curves
The position of the tip of the thermocouple probe was detected from the reference scans of the first part of the experiment for each of the tissue specimens studied. This position was later used to mark the central pixel of a ROI on the CT images acquired during the second part of the experiment (i.e. without the thermocouple probe), under the exact same conditions. A square ROI of 3 × 3 pixels was then defined around the location of the thermocouple tip to calculate the HU value. The calculated HU value was the mean value of the ROI after the image was filtered with a 5-pixel width Gaussian filter.
For each case, plots of the HU versus time and the temperature versus time curves were registered. These curves were then integrated in order to depict the HU as a function of temperature; this process was performed for all of the studied fat tissue specimens.
Finally, for each of the generated curves, the heating stage was approximated analytically using higher order functions including a second-degree polynomial function by implementing least squares regression and determining goodness of fit. The post-ablation stage, on the other hand, was approximated by applying linear regression.
Indices for tissue condition assessment
As prior studies of HU–temperature curves depict a hysteresis phenomenon whereby the heating trajectory differs substantially from the post-ablation stage trajectory [Citation21], four hysteresis indices for quantifying this data were performed, as illustrated in .
Enclosed region: This index measures the region bounded by the pair of heating and post-ablation curves.
Total range: This index measures the total difference in HU values, between the minimal and maximal HU values, which is the total HU range during heating and post-ablation cool-down.
Maximal disparity: This index measures the maximal disparity between HU values for the same temperature, measured once during heating and once during post-ablation cool-down.
Indentation depth: This index measures the indentation depth of HU values, calculated as the difference between the HU value of the nadir point of the curve, HU(Tnadir) and the highest HU value measured during the rest of the heating process, HU(TSonication_off). It defines the ‘depth’ of the indentation of the heating parabolic curve.
Figure 2. Schematic illustration of the indices, found from the HU vs. temperature plot: (A) depicts the total HU range; (B) depicts the maximal HU disparity; and (C) depicts the indentation depth, which is the difference between the minimal value and the value at the end of the heating. The enclosed region between the heating and post-ablation trajectories is shaded.
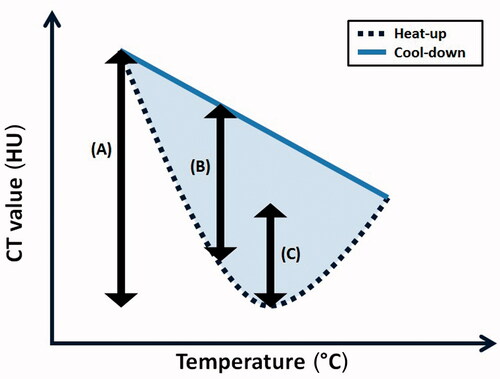
Results
HU–temperature curves: HU versus time and temperature versus time were measured () and then integrated to yield a HU–temperature curve depicted in .
Figure 3. An example of HU versus time curve measured on ex vivo porcine fat tissue during heating and cooling post-ablation stages and its matching temperature versus time profile measured under the same conditions. The error bars represent the standard deviation around the average value.
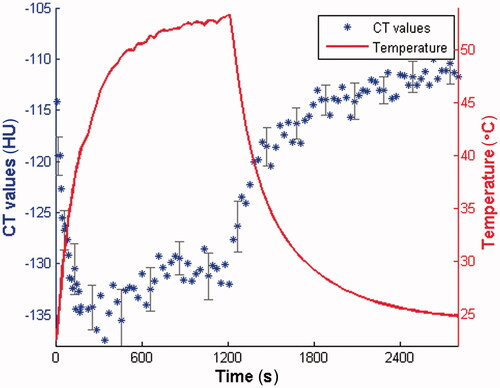
Figure 4. HU versus temperature curves obtained for each of the six specimens studied. For clarity, arrows indicating the time progression are depicted in the bottom right figure. Note the non-linear behaviour in the heating stage and the linear behaviour of the post-ablation stage. The vertical line indicates the maximal discrepancy between heating and post-ablation values.
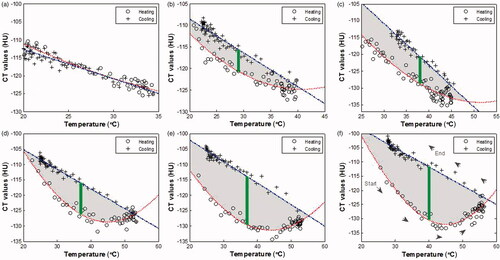
When observing the HU–temperature curves of all the specimens that exceeded 44.5 °C (), a non-monotonic behaviour was detected. At the beginning of the heating stage, as the measured temperature increased, the HU values decreased to a defined nadir. At this point a non-monotonic behaviour was demonstrated, where the HU values started to increase with the temperature.
For each of the tissue specimens a polynomial function of the second degree was fitted to HU–temperature data of the heating stage using least squares regression. This approximation has yielded the best correlation coefficients for all cases (R2 ≥ 0.87). In the cases where the temperature exceeded 44 °C a minimum point of the curve was found at around this temperature (Mean Tnadir = 44.5 ± 0.57 °C), as depicted in . In addition, a second degree polynomial function was fitted to the combined data (solid line in , R2 ≥ 0.75). Finally, as an additional proof of consistency, for comparable cases such as numbers 4 and 5 where the final temperature differed by only 1.5 °C, we have calculated the discrepancy between the curves and have documented a very small average difference in temperature during the heating and cool-down period of 0.18 °C (less than 1%). In addition, the average difference in CT HU value during heating and cool-down was 2.9 HU, well within the standard deviation of measurements.
Figure 5. Heating curves of specimens that were heated beyond hyperthermic injury point demonstrate a non-monotonic behaviour, with a minimum at about 44.5 °C.
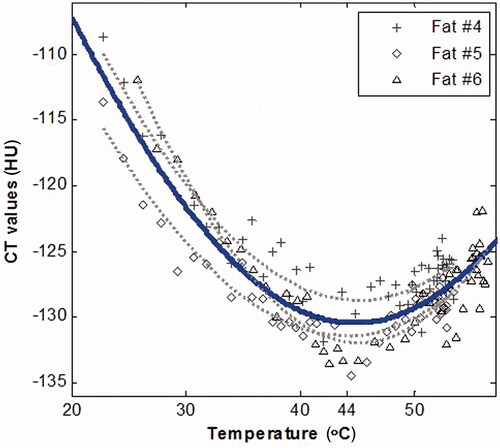
This combined data curve later served as a calibration curve for the temperature calculation to yield thermal maps of the ablated area (see ).
Figure 6. CT image with thermal mapping at different progressive times during the heating phase where darker (colour) pixels represent temperature above 18 °C and pixels below that are shown in (lighter) greyscale. (The low temperature is due to the refrigerated storage of the fat). The first image (top left) was acquired 18 s after HIFU activation. The time interval between the images is approximately 2.5 min (top-left corner to bottom-right corner). The black bar is 5 mm.
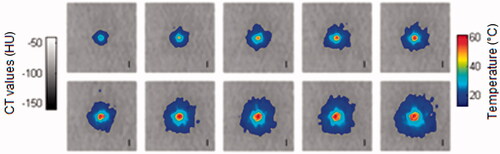
During the cooling post-ablation stage, the HU values increased as well, but along a clear linear curve. Likewise, linear fits yielded very strong correlation (R2 ≥ 0.9) for temperature for all of the cases.
Assessment of tissue damage
summarises the calculated results obtained for each of the hysteresis indices versus maximal temperature. As can be noted, the calculated indices increase as the temperature increases, and they are highly correlated. This is shown in .
Table I. Summary of the quantified results of fatty tissues damage, for each of the suggested methods.
As for the indentation-depth index, calculation was made also directly from the HU versus time curve, i.e. without any need for the temperature measurements, as shown for two examples in .
Discussion
In recent years, HIFU treatments for non-invasive tissue ablation have become prevalent in many applications, most notably for the treatment of breast neoplasms that are often surrounded by fatty tissue [Citation22,Citation23]. The main technical challenge for these types of treatment is the difficulty in obtaining non-invasive thermal monitoring during the process. This is especially true for fatty tissues, since MRI, which is currently considered the leading modality for non-invasive internal temperature monitoring [Citation7,Citation8], is less accurate in fat than desired when using the PRFS method for MRI thermometry during ablation treatments [Citation11,Citation24]. The method suggested here for monitoring HIFU treatment in fat tissue with X-ray CT aims to address this problem. The results obtained in this study provide strong indication that indeed it has the potential to serve as the basis for development of a new clinical tool.
CT HU changes during heating have already been observed for many years [Citation25,Citation26]. Previous works assumed that HU changes stemming from heating are attributed mainly to thermal expansion. Moreover, it was assumed that the thermal expansion coefficient remains constant during the heating process. Consequently, this has led to the assumption that the HU are linearly related to the temperature [Citation27,Citation28]. In a recent study [Citation21], it was revealed that a very prominent non-linear behaviour of HU versus temperature exists and this is associated with a significant hysteresis phenomenon. This leads to the conclusion that the linear assumption is insufficient to adequately describe the physical process.
Indeed, the findings reported here further support the hypothesis that the HU–temperature curve hysteresis phenomena is associated with the amount of thermal dose administered to the tissue and hence to the damage induced. This can be verified by observing the HU–temperature curves depicted in , and especially for the case where temperatures did not exceed 35 °C (). As can be noted, the HU values during the heating and post-ablation stages are very similar, indicating that no irreversible change occurred, and that the heating process was reversible. On the other hand, as the maximal temperature reached has increased, the discrepancy between the heating curve and the cooling curve (as indicated by the enclosed region index [HU°C]) becomes more prominent. This can also be observed quantitatively in . Both observations are consistent with the above hypothesis and previous findings [Citation21].
The results obtained in this study during HIFU heating of the ex vivo porcine fat tissue produced a reproducible HU–temperature heating curve. These values can serve as a calibration curve for thermal mapping of the tissue during treatment. illustrates an exemplary set of images, whereby the temperature evaluation for each pixel of the CT images is depicted as a colour-coded graphic overlay atop the anatomical grey-level images, similar to the manner used in MRI thermometry [Citation29]. The temperatures were calculated according to the calibration curve. These time progression images demonstrate how the heat dissipates around the centre of the HIFU focal spot zone while the temperature rises.
However, contrary to findings in liver tissue [Citation21], where the heat-up curve displayed a monotonic concave shape, in this case of fat tissue, a convex non-monotonic curve was observed, with a defined nadir point at about 44.5 °C. Moreover, when combined with the post-ablation trajectory the plots described a counter-clockwise trajectory for fat tissue (marked in ), which is fundamentally different from the clockwise trajectory observed in liver and chicken serum albumin experimentally studied [Citation21]. These findings imply that the observed hysteresis phenomenon is tissue-type dependent.
Summarising the major finding in this study, it was observed that in the course of the first heating phase, the curve behaviour demonstrates a persistent decrease in HU. However, when reaching the temperature of about 44.5 °C, a nadir point is observed. This temperature is related to the onset of hyperthermic injury, and coagulation and significant degradation in cell viability of fat tissue occurs [Citation19]. From that point on, an increase of HU values during the remaining heating phase is noted. Our suggested explanation is that this behaviour of fat stems from two opposing mechanisms. During the first phase of heating, thermal expansion is probably the dominant mechanism. This mechanism leads to a decrease in fat density and consequently to a decrease in the X-ray attenuation coefficient values, as is observed for many tissues. However, above 44.5 °C, when notable hyperthermic injury is observed, fat has been shown by previous investigators to undergo structural and morphological changes [Citation4] that presumably make it more desiccated, and hence denser. This results in the observed increase in attenuation and the corresponding HU values during the second phase of heating.
Our results demonstrate a pattern of HU–temperature values during HIFU heating that can be described by a second degree polynomial equation. This pattern could serve as a useful tool for assessing tissue damage levels during thermal ablation therapy. Making use of the fact that the nadir point always occurs at about 44.5 °C, the obtained heating curves can also be used as a calibration means for calculating the temperature from the HU values of the CT image during treatment (as demonstrated in ). Since temperature alone is only ancillary information to the physician, displaying the tissue condition as well as temperature and accumulative damage in a quantitative manner during treatment would be more beneficial than showing merely the temperature alone.
A potential clinical application could be developed based on the presented findings and the suggested hysteresis quantifying indices. demonstrates an exemplary clinical use of the suggested indentation-depth methodology. Indeed, in such a case the detection of the nadir point of the tumour’s margins may indicate the termination time of the treatment. Thus, by implementing this method, one does not have to rely on the absolute temperature measurement or HIFU heating protocol. Another potential advantage is that reliance on the identification of the HU minima utilises only the heating stage of the fat, providing shorter monitoring time, and consequently reduces the number of X-ray scans needed, and radiation level. Accordingly, one may conceive an application by which a tumour surrounded by fatty tissue (such as breast tumours) is ablated by HIFU.
Figure 8. Illustration of overlay damage level of the fat tissue on top of the CT image. The indentation depth index was calculated for each pixel around the focal zone of a CT image, obtained immediately after HIFU treatment termination, regardless of the temperature data. The results were analysed and transformed into three levels, as assessed by the indentation depth index values, marked by three contours. Each contour represents an area with a different level of tissue damage, where the most inner contour (red) indicates higher estimated damage level. The black bar is 5 mm.
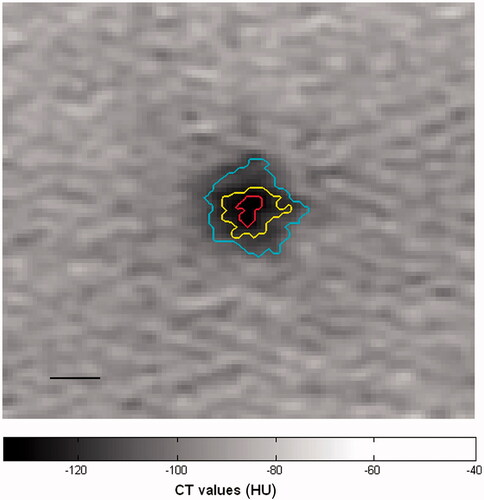
In practice, however, during HIFU treatment of fat monitored by X-ray CT, the HU–temperature curve is not available. Hence, importantly, we argue that monitoring of the HU values versus time would suffice to assess the induced thermal damage. This can be done by detection of the minimal HU instant, i.e. the nadir point in the HU–time curve (), which is the estimated point of hyperthermic injury onset. As explained above, this nadir point implies not only that the temperature of T = 44.5 °C has been reached, but likely that irreversible changes in tissue properties have occurred. Indeed, as shown by previous studies [Citation19], for adipocyte cells when exceeding this temperature cell viability rapidly decreases. They have shown that at 45 °C viability drops from 89% after 1 min to 40% after 3 min. The rate of hyperthermic injury is even further accelerated for higher temperatures. Heating fat tissue with a temperature of 75 °C would result in cellular death, and no viable cells [Citation4]. Thus, an indication of the success of the treatment might be that the regions around the tumour have reached 44.5 °C. This will indicate that the tissue inside the tumour (which is closer to the HIFU focal zone and so naturally warmer) has exceeded the hyperthermic injury point.
According to the indentation depth parameter introduced above, once the minimum has been detected (i.e. Tnadir), the HU values can be measured until the deactivation of the HIFU (i.e. TSonication_off). The difference between the two HU values may serve as an index by real-time measurement of the increase in HU during the remainder of the heating phase, once the hyperthermic injury onset was detected. With this index it would be possible to provide a quantitative index for the damage induced (i.e. the reaching of ablation/tissue denaturation). Again, it is emphasised that this method can be used without any need for direct temperature measurements. Once the nadir point in the HU–time curve is detected, the magnitude of the indentation parameter is constantly registered. This is demonstrated by the solid red line of , in the HU–time profile. When its value has exceeded a predefined threshold, it could potentially be concluded that damage has been achieved and the treatment could be terminated. To conclude the process, a graphic overlay could be superimposed on top of the CT images, in real time, as illustrated in to depict the size and level of the damaged area. In order to establish the applicability of this method for potential clinical monitoring, further work and research is still needed.
Our study has several limitations. We examined ex vivo fat tissue and our results need to be verified in vivo. Moreover, the applicability of X-ray CT for thermography will be challenging, as CT uses ionizing radiation, hence the lowest possible dose delivered to the human tissue will need to be found first in future ex vivo studies and later in in vivo studies.
In summary, in fat tissues the point where a temperature of 44.5 °C has been reached can be clearly detected non-invasively from the occurrence of a minimum point (nadir point) of the HU–time curve. This may be indicative for the onset of irreversible ablative denaturation, and can potentially be utilised to develop a new method for thermal monitoring with the aid of X-ray CT. With the proposed method for thermal monitoring implemented on X-ray CT for HIFU treatments of fat tissues, it might be possible to evaluate non-invasively and in real time the level of tissue damage in a quantifiable manner, hence providing better evaluation of the ablated tissue size and helping to optimise clinical treatments.
Declaration of interest
The authors report no conflicts of interest. The authors alone are responsible for the content and writing of the paper.
References
- Azhari H. Basics of Biomedical Ultrasound for Engineers. 1st edition. New York: Wiley-IEEE Press, 2010
- Huber PE, Jenne JW, Rastert R, Simiantonakis I, Sinn H, Strittmatter H, et al. A new noninvasive approach in breast cancer therapy using magnetic resonance imaging-guided focused ultrasound surgery. Cancer Res 2001;61:8441–7
- ter Haar G. High intensity focused ultrasound: Physical principles and devices. Int J Hyperthermia 2007;23:89–104
- Merckel LG, Deckers R, Baron P, Bleys RL, van Diest PJ, Moonen CT, et al. The effects of magnetic resonance imaging-guided high-intensity focused ultrasound ablation on human cadaver breast tissue. Eur J Pharmacol 2013;717:21–30
- Diederich CJ, Hynynen K. Ultrasound technology for hyperthermia. Ultrasound Med Biol 1999;25:871–87
- Buy X, Tok C, Szwarc D, Bierry G, Gangi A. Thermal protection during percutaneous thermal ablation procedures: Interest of carbon dioxide dissection and temperature monitoring. Cardiovasc Intervent Radiol 2009;32:529–34
- Hynynen K, McDannold N. MRI guided and monitored focused ultrasound thermal ablation methods: A review of progress. Int J Hyperthermia 2004;20:725–37
- Furusawa H, Namba K, Thomsen S, Akiyama F, Bendet A, Tanaka C, et al. Magnetic resonance-guided focused ultrasound surgery of breast cancer: Reliability and effectiveness. J Am Coll Surg 2006;203:54–63
- Rieke V, Butts Pauly K. MR thermometry. J Magn Reson Imaging 2008;27:376–90
- McDannold N, Hynynen K, Wolf D, Wolf G, Jolesz F. MRI evaluation of thermal ablation of tumors with focused ultrasound. J Magn Reson Imaging 1998;8:91–100
- Hynynen K, McDannold N, Mulkern RV, Jolesz FA. Temperature monitoring in fat with MRI. Magn Reson Med 2000;43:901–4
- Soher BJ, Wyatt C, Reeder SB, MacFall JR. Noninvasive temperature mapping with MRI using chemical shift water–fat separation. Magn Reson Med 2010;63:1238–46
- Moreno-Moraga J, Valero-Altés T, Riquelme AM, Isarria-Marcosy M, Royo de la Torre J. Body contouring by non-invasive transdermal focused ultrasound. Lasers Surg Med 2007;39:315–23
- Chang S, Huang Y, Lee M, Chang C, Chung W, Wu E, et al Combination therapy of focused ultrasound and radio-frequency for noninvasive body contouring in Asians with MRI photographic documentation. Lasers Med Sci 2013:1–8
- Bruners P, Levit E, Penzkofer T, Isfort P, Ocklenburg C, Schmidt B, et al. Multi-slice computed tomography: A tool for non-invasive temperature measurement? Int J Hyperthermia 2010;26:359–65
- Pandeya GD, Greuter MJ, de Jong KP, Schmidt B, Flohr T, Oudkerk M. Feasibility of noninvasive temperature assessment during radiofrequency liver ablation on computed tomography. J Comput Assist Tomogr 2011;35:356–60
- Schena E, Saccomandi P, Giurazza F, Caponero M, Mortato L, Di Matteo F, et al. Experimental assessment of CT-based thermometry during laser ablation of porcine pancreas. Phys Med Biol 2013;58:5705–16
- Martinez AA, Meshorer A, Meyer JL, Hahn GM, Fajardo LF, Prionas SD. Thermal sensitivity and thermotolerance in normal porcine tissues. Cancer Res 1983;43:2072–5
- Franco W, Kothare A, Ronan SJ, Grekin RC, McCalmont TH. Hyperthermic injury to adipocyte cells by selective heating of subcutaneous fat with a novel radiofrequency device: Feasibility studies. Lasers Surg Med 2010;42:361–70
- Paulson EK, Sheafor DH, Enterline DS, McAdams HP, Yoshizumi TT. CT fluoroscopy-guided interventional procedures: Techniques and radiation dose to radiologists. Radiology 2001;220:161–7
- Weiss N, Goldberg SN, Sosna J, Azhari H. Temperature–density hysteresis in X-ray CT during HIFU thermal ablation: Heating and cooling phantom study. Int J Hyperthermia 2014;30:27–35
- Kennedy J, Ter Haar G, Cranston D. High intensity focused ultrasound: Surgery of the future? Br J Radiol 2003;76:590–9
- Coon J, Payne A, Roemer R. HIFU treatment time reduction in superficial tumours through focal zone path selection. Int J Hyperthermia 2011;27:465–81
- Sprinkhuizen SM, Konings MK, van der Bom MJ, Martijn J, Viergever MA, Bakker CJ, et al. Temperature induced tissue susceptibility changes lead to significant temperature errors in PRFS based MR thermometry during thermal interventions. Magn Reson Med 2010;64:1360–72
- Bydder GM, Kreel L. The temperature dependence of computed tomography attenuation values. J Comput Assist Tomogr 1979;3:506–10
- Fallone B, Moran P, Podgorsak E. Noninvasive thermometry with a clinical X-ray CT scanner. Med Phys 1982;9:715–21
- Pandeya G, Klaessens J, Greuter M, Schmidt B, Flohr T, van Hillegersberg R, et al. Feasibility of computed tomography based thermometry during interstitial laser heating in bovine liver. Eur Radiol 2011;21:1733–8
- Bruners P, Pandeya GD, Levit E, Roesch E, Penzkofer T, Isfort P, et al. CT-based temperature monitoring during hepatic RF ablation: Feasibility in an animal model. Int J Hyperthermia 2012;28:55–61
- Rieke V, Butts Pauly K. MR thermometry. J Magn Reson Imaging 2008;27:376–90