Abstract
Purpose: Exposure to increased environmental temperatures is commonly used as a non-pharmacological treatment modality in ankylosing spondylitis (AS). We aimed to investigate systemic immunological effects of moderate whole body hyperthermia in patients with AS compared to healthy control subjects. Materials and methods: Ten healthy control subjects and six AS patients underwent whole body hyperthermia treatment with 38.7–39 °C body core temperature over 60 min. Numbers of polymorphonuclear leucocytes and lymphocyte subsets, plasma concentrations of several acute phase reactants and cytokines, and gene expression levels of toll-like receptor 4 (TLR-4), interleukin 10 (IL-10) and heat shock protein beta 1 (HSPB1) were determined during and up to 24 h after treatment. Results: TLR-4, IL-10 and HSPB1 gene expression increased significantly up to 3 h post treatment, with an earlier, higher and more pronounced increase of IL-10 in patients with AS. An increase of natural killer cells and CD8+ T lymphocytes was noted during active heating, with a subsequent decrease up to 2 h after treatment. CD4+ T lymphocytes showed a short increase during active treatment in AS patients, while decreasing immediately after start of treatment in control subjects. Neutrophil granulocytes increased significantly up to 3 h after treatment, monocytes and B lymphocytes remained unchanged. Likewise, no significant changes were found concerning systemic cytokine concentrations and acute phase reactants. Conclusions: Our data support the concept of systemic immunological effects of moderate whole body hyperthermia in patients with AS.
Introduction
Ankylosing spondylitis (AS) is a chronic inflammatory disease of the sacroiliac joints, spine and entheses affecting about 0.1–0.25% of the general population. In addition to pharmacological treatment, current guidelines recommend physiotherapy including regular exercise, preferably in combination with spa therapy [Citation1,Citation2]. Continued improvement of pain scores and function has been demonstrated over a period of 6 months following serial moderate whole body hyperthermia treatment in natural hot caves with additional low dose radon exposure in several controlled clinical trials [Citation3,Citation4]. In addition to immediate physical hyperthermia effects such as muscle relaxation and consecutive pain reduction, immunological mechanisms may play a role in prolonged improvement of pain and function in AS patients following serial moderate hyperthermia treatment, and may therefore lead to altered systemic cytokine and acute phase reactant levels. Elevated systemic levels of tumour necrosis factor α (TNF-α), interleukin 6 (IL-6) and interleukin 10 (IL-10) have been reported in AS patients compared to healthy controls, while soluble interleukin 2 receptor (sIL-2R) levels were decreased [Citation5]. Previous in vitro and in vivo studies with core temperatures up to 42 °C showed an increase of circulating interleukin 1 β (IL-1β), IL-6, interleukin 8 (IL-8) and TNF-α [Citation6–11], whereas moderate hyperthermia treatment around 39 °C enhanced anti-inflammatory T cell functions [Citation8,Citation12–18]. Prolonged T cell activation up to 48 h after whole body hyperthermia has been demonstrated, accompanied by a marked shift of T cells into lymphoid tissues [Citation12]. Numbers of natural killer (NK) cells and CD8+ T lymphocytes increased during whole body hyperthermia, while numbers of CD4+ T lymphocytes decreased [Citation13,Citation15]. Further effects were noted concerning systemic levels of acute phase reactants, with increased levels of transferrin and C-reactive protein (CRP) and inconsistent data for α-1 antitrypsin (α1-AT) [Citation19,Citation20].
In order to further elucidate physiological mechanisms underlying the empirical success of therapeutic hyperthermia, we investigated a number of immunological parameters that appeared promising according to previous studies.
Materials and methods
In a pilot study design we determined numbers of polymorphonuclear leucocytes and lymphocyte subsets, plasma levels of IL-1β, sIL-2R, IL-6, IL-10, IL-12, interferon γ (IFN-γ), TNF-α, CRP, α1-AT, haptoglobin, transferrin, complement 3c (C3c), complement 4 (C4) and gene expression levels of heat shock protein β 1 (HSPB1), toll-like receptor 4 (TLR-4) and IL-10 as markers of heat stress, innate immune system activation and adaptive anti-inflammatory activity.
Patients
The study was approved by the institutional review board of the Medical University of Graz (SRN 20–132 ex 08/09, SRN 21–363 ex 09/10), and written informed consent was obtained from all study participants. Ten healthy control subjects, median age 24.5 years (range 22–32), median CRP 1.0 mg/L (range 1–3), all male, and six patients diagnosed with AS according to the modified New York criteria, median age 42 years (range 25–46), median bath ankylosing spondylitis disease activity index (BASDAI) 2.2 (range 0.2–5.5), median bath ankylosing spondylitis functional index (BASFI) 1.85 (range 0.2–4.9), median CRP 5.8 mg/L (range 0.6–25.7), median erythrocyte sedimentation rate 9 mm (range 2–60), one female, were included in the study. Individuals diagnosed with autoimmune diseases other than AS, chronic infectious diseases, cardiac or pulmonary conditions or other severe concomitant disorders were excluded from the study. All participants were clinically examined to rule out acute infections or other acute medical conditions. Alcohol, coffee or nicotine consumption and intake of non-steroidal anti-inflammatory drugs (NSAIDs), analgesics, corticosteroids, opioids or tranquillisers were not allowed immediately before and during the study. No anaesthetics or analgesics were administered during hyperthermia treatment.
Whole body hyperthermia treatment
Moderate whole body hyperthermia was induced by water-filtered infrared-A radiation (wIRA) using a Heckel HT 3000 hyperthermia device (Hydrosun, Esslingen, Germany). Study participants entered the preheated device in a supine position after installation of ECG, blood pressure and oxygen saturation monitors, and wIRA radiation was started immediately. Body core temperature was continually measured using transrectal temperature probes, aiming to reach and maintain body core temperatures of 38.7–39 °C. Median time until achievement of target temperature was 55 min (range 43–74). During the heat retention period, target temperature was maintained for 1 h by wrapping study participants in the flexible walls of the hyperthermia equipment according to the instruction manual. Blood samples were taken using intravenous lines before hyperthermia treatment around 7:00 a.m., at the end of active heating (achievement of target body core temperature), and 1, 2, 3 and 24 h after treatment. Study participants drank 1–2 L of tap water during hyperthermia treatment and were allowed to get up during the observation period.
Differential blood count and lymphocyte subsets
Blood samples were collected into EDTA tubes at room temperature and polymorphonuclear leucocyte counts were obtained by routine laboratory methods. Lymphocyte subsets (B lymphocytes, CD4+ and CD8+ T lymphocytes, NK cells and monocytes) were determined using FACS Calibur (Beckton Dickinson, NJ), MultiSET Test kit, MultiSET (BD Biosciences, San Jose, CA) software and commercially available antibodies according to protocol.
Acute phase reactants
Whole blood samples were collected into pre-chilled EDTA tubes, centrifuged immediately at 4 °C, 1000 g for 10 min, and plasma was aliquoted and stored at −80 °C. Haptoglobin, C3c, C4 and α1-AT levels were determined by immunonephelometry using a Behring Nephelometer II (Siemens, Marburg, Germany). CRP and transferrin levels were measured by immunoturbidimetry using a cobas 8000 analyser (Roche Diagnostics, Mannheim, Germany).
Systemic cytokines
Whole blood samples were collected into pre-chilled EDTA tubes, centrifuged immediately at 4 °C, 1000 g for 10 min, and plasma was aliquoted and stored at −80 °C for batch cytokine analysis. A multiplex suspension bead array immunoassay (Bio-Plex analyser, Bio-Rad, Hercules, CA) was used to simultaneously measure levels of IFN-γ, IL-1β, sIL-2R, IL-6, IL-10, IL-12 and TNF-α according to protocol. Cytokine concentrations were calculated using a five-parameter standard curve derived from three measurements of reference cytokine concentrations supplied by the manufacturer.
End point reverse transcriptase polymerase chain reaction
Whole blood samples were collected into pre-chilled Tempus blood RNA tubes (Applied Biosystems, Foster City, CA) and frozen immediately after collection. mRNA was extracted using a Tempus (Applied Biosystems) spin RNA isolation kit. To avoid potential DNA contamination, DNA was digested with 1 U DNase (Fermentas, Burlington, Canada) per µg RNA for 30 min at 37 °C. A total of 0.5–1 µg of RNA was then reverse-transcribed using a RevertAid cDNA synthesis kit (Fermentas) and random hexamer primers according to the manual. For real-time reverse transcriptase polymerase chain reaction (RT-PCR) analysis, a ready-to-use master mix containing SYBR® green (Invitrogen, Carlsbad, CA) and primers were used. A standard curve was constructed from a pool of all 111 cDNA samples to allow quantification. All samples were run in triplicate with glyceraldehyde 3-phosphate dehydrogenase (GAPDH), β-2 microglobuline (B2M) and hypoxanthine phosphoribosyl transferase (HPRT1) serving as reference genes for internal control. The real-time PCR protocol consisted of 40 cycles of amplification followed by a temperature gradient to verify amplified sequences by their melting temperature. Real-time PCR was performed with AB7900HT (Applied Biosystems). The initial amount of cDNA was calculated using ABI prism sequence-detecting software (Applied Biosystems) according to the manufacturer’s manual, and was based on fixed quantities for the standard. The mean quantity of a given triplicate for the target gene was compared to the geometric mean of the housekeeping genes GAPDH, B2M and HPRT1, resulting in a normalised and comparable ratio [Citation21].
Statistical analysis
Repeated measurements were evaluated descriptively by quantiles and box-and-whiskers plots and analysed according to a non-parametric longitudinal factorial design [Citation22]. By this method, all measurements of a variable are rank-transformed, and a repeated measures analysis of variance (ANOVA) is performed on the relative ranks (ranks divided by the number of measurements). Means of relative ranks are called relative treatment effects (RTE). ANOVA type statistical tests [Citation22] were performed for group effect, time effect and time by group interaction. For each variable, three kinds of statistical tests were calculated: one testing for differences between control subjects and patients concerning average RTE (group effect), one testing for changes over time with respect to average RTE of control subjects and patients (time effect) and one testing for differences in kinetics (group by time interaction). Correction for multiple testing was achieved by using the false discovery rate (FDR), which is the probability of a wrongly significant test result within all test results declared to be significant. The corrected significance level was chosen so that FDR would be 0.05. Analogous to the p-value of a statistical test, a q-value is reported such that all statistical tests with a q-value below 0.05 would imply an FDR less than 0.05. Q-values were calculated according to the method of Benjamini [Citation23,Citation24]. R 15.1 [Citation25], R code [Citation25] and R package multtest 2.12.0 were used for calculations.
Results
All measurements were conducted on blood samples taken immediately before hyperthermia treatment, at the end of active heating, and 1, 2, 3 and 24 h after treatment, respectively.
Polymorphonuclear leucocytes and lymphocyte subsets
Investigation of absolute leucocyte cell counts showed a significant increase of neutrophils (q = 3.8 E–12) and basophils (q = 0.045) and a significant decrease of eosinophils (q = 0.0018) and lymphocytes (q = 0.0077) following hyperthermia treatment. Further analysis of lymphocyte subsets yielded a significant decrease of absolute numbers of T lymphocytes (q = 0.0073) with a decrease of both CD4+ (q = 0.018) and CD8+ (q = 0.015) T lymphocytes and a significant decrease of NK cells (q = 1.4 E-07). No significant treatment effects were found concerning numbers of monocytes (q = 0.21) and B lymphocytes (q = 0.07). Overall, neutrophil (q = 0.018) and monocyte counts (q = 0.016) were significantly higher in AS patients compared to control subjects, and CD4+ T lymphocytes showed significantly different kinetics in both study groups, with an initial increase in AS patients during active treatment (, and . No figures shown for B-lymphocytes and monocytes).
Figure 1. Polymorphonuclear leukocyte counts. Symbols and abbreviations: Δ patients; ○ healthy control subjects;
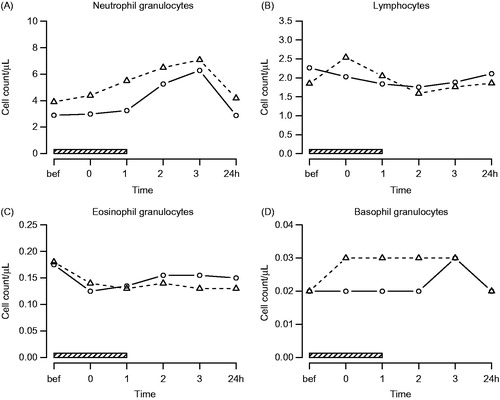
Figure 2. Lymphocyte subsets. Symbols and abbreviations: Δ patients; ○ healthy control subjects;
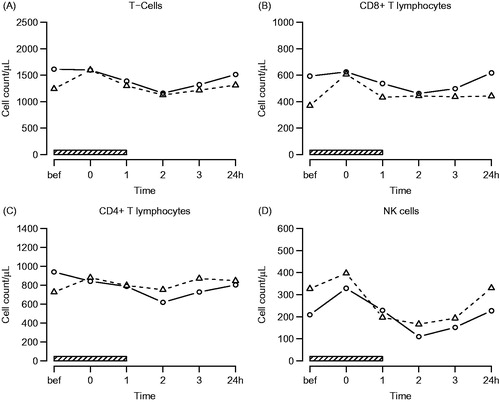
Table 1. Effects of hyperthermia on polymorphonuclear leucocytes and lymphocyte subsets (q-values).
TLR-4, IL-10 and HSPB1 gene expression
A significant increase of IL-10 (q = 4.6 E–13), TLR-4 (q = 0.00099) and HSPB1 (q = 1.6 E–59) gene expression levels was found following hyperthermia treatment in AS patients and control subjects. Kinetics of TLR-4 (q = 0.44) and HSPB1 (0.092) were similar in both groups, while IL-10 showed a significantly earlier, higher and more sustained increase in patients compared to control subjects (q = 0.073). No significant differences were found in TLR-4 (q = 0.11) and HSPB1 (q = 0.27) gene expression between both study groups (, ).
Figure 3. TLR-4, IL-10 and HSPB1 gene expression. Symbols and abbreviations: Δ patients; ○ healthy control subjects;
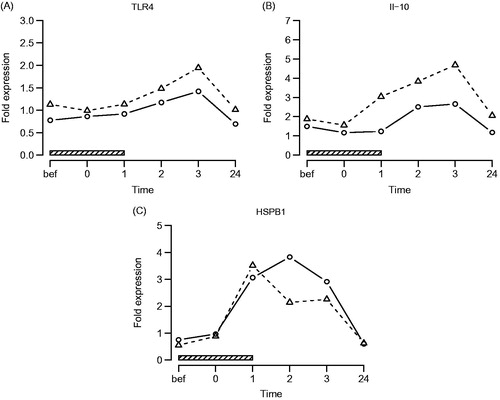
Table 2. Effects of hyperthermia treatment on TLR-4, IL-10 and HSPB1 gene expression (q-values).
Systemic cytokines
No significant changes in levels of IL-1β (q = 0.44), sIL-2R (q = 0.42), IL-6 (q = 0.27), IL-10 (q = 0.42), IL-12 (q = 0.32), IFN-γ (q = 0.31) and TNF-α (q = 0.27) were found during and up to 24 h after treatment. Plasma levels of sIL-2R (q = 0.0022) were significantly lower in AS patients compared to control subjects (, ).
Figure 4. Systemic cytokines. Symbols and abbreviations: Δ patients; ○ healthy control subjects;
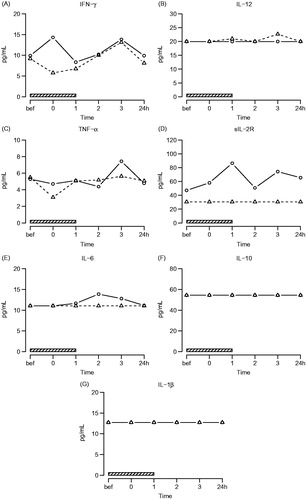
Table 3. Effects of hyperthermia treatment on plasma cytokine concentrations (q-values).
Acute phase reactants
No significant changes in systemic levels of CRP (q = 0.055), α1-AT (q = 0.39), haptoglobin (q = 0.07), transferrin (q = 0.32), C3c (q = 0.27) and C4 (q = 0.39) were observed during and up to 24 h after treatment. CRP (q = 0.02), α1-AT (q = 0.0029), C3c (q = 0.019) and haptoglobin (q = 0.0074) concentrations were significantly higher in patients compared to control subjects (, ).
Figure 5. Acute phase reactants. Symbols and abbreviations: Δ patients; ○ healthy control subjects;
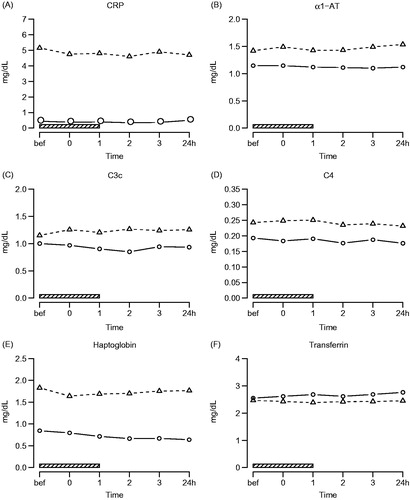
Table 4. Effects of hyperthermia treatment on acute phase reactants (q-values).
Discussion
Our aim was to investigate systemic immunological effects of moderate whole body hyperthermia in patients with AS compared to healthy control subjects. Significant changes were observed in both study groups concerning numbers of polymorphonuclear leucocytes and lymphocyte subsets as well as TLR-4, IL-10 and HSPB1 gene expression after a single treatment course.
We found a significant increase of neutrophils and basophils up to 3 h after treatment and a slight decrease of eosinophils during the whole observation period. An increase of NK cells and CD8+ T lymphocytes was noted during active heating, with a subsequent decrease up to 2 h after treatment. CD4+ T lymphocytes decreased significantly 1–2 h after achievement of target temperature, with different kinetics in both study groups. Our data largely concur with the existing literature, describing an increase of circulating neutrophils following hyperthermia treatment due to stress induced mobilisation from the vessel walls [Citation26]. Moreover, tissue trafficking and homing to lymph nodes, but also apoptosis, may account for the decrease in T lymphocytes and NK-cells [Citation12,Citation27]. Monocytes have previously been shown to be activated by hyperthermia, exhibiting reduced extravasation in order to be able to respond to pathogens in the bloodstream [Citation28]. Accordingly, we did not find significant changes in monocyte numbers during and after hyperthermia treatment. However, at higher body core temperatures up to 40 °C over 6 h, one study also detected elevated monocyte counts [Citation26]. Concerning the kinetics of lymphocyte subsets, our data confirm the results from previous studies [Citation13,Citation26].
In addition, we found a significant increase in TLR-4, IL-10 and HSPB1 gene expression following hyperthermia treatment. An earlier, higher and more sustained increase of IL-10 mRNA was detected in AS patients compared to healthy control subjects, with TLR-4 and HSPB1 showing similar kinetics in both study groups. HSPB1 gene expression already increased during active heating, while TLR-4 gene expression started increasing during the heat retention period. mRNA levels of all tested genes returned to baseline values 24 h after treatment. It has been demonstrated that activation of the innate immune system via HSP peptide complexes led to subsequent release of proinflammatory cytokines [Citation29,Citation30], with Hsp70 being widely involved in the regulation of immune cell activity [Citation31,Citation32]. TLR-4 gene expression by antigen presenting cells and LPS-induced cytokine production has been found to be increased following moderate hyperthermia treatment, suggesting an activation of the innate immune response [Citation33,Citation34]. Interestingly, we also found a striking increase in IL-10 gene expression, which was even more pronounced in AS patients compared to healthy control subjects. Augmented IL-10 production has been demonstrated in vitro and in vivo in the context of extreme hyperthermia [Citation9,Citation11], but might also play a role in moderate hyperthermia indicating an emerging anti-inflammatory cytokine response.
Several in vitro and in vivo studies showed an elevation of acute phase reactants and cytokines in the context of extreme hyperthermia up to 42 °C [Citation8–11,Citation19,Citation20,Citation35]. Only one study reported reductions of serum levels of IL-1β, IL-6 and TNF-α were reported in AS patients undergoing one treatment cycle of balneotherapeutic hyperthermia at 38.5 °C [Citation36]. We did not detect significant changes of cytokine or acute phase reactant levels following a single moderate hyperthermia treatment.
Conclusion
Our data suggest an additional systemic immunological effect of moderate whole body hyperthermia in patients with AS. Of note, we found a significant increase in TLR-4, IL-10 and HSPB1 gene expression following hyperthermia treatment with an earlier, higher and more sustained increase of IL-10 mRNA detected in AS patients. Concerning kinetics of differential cell counts and lymphocyte subsets, our data largely confirm the results from previous studies. However, a single moderate hyperthermia treatment might not be sufficient to affect systemic cytokine levels and acute phase reactants. Overall, this study may provide a basis for further investigation of serial moderate whole body hyperthermia treatment in AS patients.
Declaration of interest
This study was funded by an unrestricted grant from Research Institute Gastein, Paracelsus Medical University, Salzburg, Austria. The Heckel HT3000 hyperthermia device was provided by Erwin Braun Stiftung, Basel, Switzerland. The authors alone are responsible for the content and writing of the paper.
Acknowledgements
We thank Yvonne Fuchs for assistance in data collection, administration and coordination of hyperthermia treatments, and Marie-Luise Kremser and Daniela Setznagl for providing excellent technical help.
References
- Braun J, van den Berg R, Baraliakos X, Boehm H, Burgos-Vargas R, Collantes-Estevez E, et al. 2010 update of the ASAS/EULAR recommendations for the management of ankylosing spondylitis. Ann Rheum Dis 2011;70:896–904
- Dagfinrud H, Kvien TK, Hagen KB. Physiotherapy interventions for ankylosing spondylitis. Cochrane Database Syst Rev 2008;23:CD002822
- Falkenbach A, Kovacs J, Franke A, Jörgens K, Ammer K. Radon therapy for the treatment of rheumatic diseases − Review and meta-analysis of controlled clinical trials. Rheumatol Int 2005;25:205–10
- Van Tubergen A, Landewe R, van der Heijde D, Hidding A, Wolter N, Asscher M, et al. Combined spa–exercise therapy is effective in patients with ankylosing spondylitis: A randomized controlled trial. Arthritis Rheum 2001;45:430–38
- Bal A, Unlu E, Bahar G, Aydog E, Eksioglu E, Yorgancioglu R. Comparison of serum IL-1 beta, sIL-2R, IL-6, and TNF-alpha levels with disease activity parameters in ankylosing spondylitis. Clin Rheumatol 2007;26:211–15
- Ostberg JR, Taylor SL, Baumann H, Repasky EA. Regulatory effects of fever-range whole-body hyperthermia on the LPS-induced acute inflammatory response. J Leukoc Biol 2000;68:815–20
- Fairchild KD, Viscardi RM, Hester L, Singh IS, Hasday JD. Effects of hypothermia and hyperthermia on cytokine production by cultured human mononuclear phagocytes from adults and newborns. J Interferon Cytokine Res 2000;20:1049–55
- Katschinski DM, Wiedemann GJ, Longo W, d’Oleire FR, Spriggs D, Robins HI. Whole body hyperthermia cytokine induction: A review, and unifying hypothesis for myeloprotection in the setting of cytotoxic therapy. Cytokine Growth Factor Rev 1999;10:93–7
- Matsui T, Kakeda T. IL-10 production is reduced by hypothermia but augmented by hyperthermia in rat microglia. J Neurotrauma 2008;25:709–15
- Bouchama A, al-Sedairy S, Siddiqui S, Shail E, Rezeig M. Elevated pyrogenic cytokines in heatstroke. Chest 1993;104:1498–502
- Robins HI, Kutz M, Wiedemann GJ, Katschinski DM, Paul D, Grosen E, et al. Cytokine induction by 41.8 °C whole body hyperthermia. Cancer Lett 1995;97:195–201
- Dieing A, Ahlers O, Hildebrandt B, Kerner T, Tamm I, Possinger K, et al. The effect of induced hyperthermia on the immune system. Prog Brain Res 2007;162:137–52
- Tomiyama-Miyaji C, Watanabe M, Ohishi T, Kanda Y, Kainuma E, Bakir HY, et al. Modulation of the endocrine and immune systems by well-controlled hyperthermia equipment. Biomed Res 2007;28:119–25
- Appenheimer MM, Chen Q, Girard RA, Wang WC, Evans SS. Impact of fever-range thermal stress on lymphocyte–endothelial adhesion and lymphocyte trafficking. Immunol Invest 2005;34:295–323
- Kappel M, Diamant M, Hansen MB, Klokker M, Pedersen BK. Effects of in vitro hyperthermia on the proliferative response of blood mononuclear cell subsets, and detection of interleukins 1 and 6, tumour necrosis factor-alpha and interferon-gamma. Immunology 1991;73:304–8
- Downing JF, Martinez-Valdez H, Elizondo RS, Walker EB, Taylor MW. Hyperthermia in humans enhances interferon-gamma synthesis and alters the peripheral lymphocyte population. J Interferon Res 1988;8:143–50
- Ostberg JR, Kabingu E, Repasky EA. Thermal regulation of dendritic cell activation and migration from skin explants. Int J Hyperthermia 2003;19:520–33
- Peng JC, Hyde C, Pai S, O’Sullivan BJ, Nielsen LK, Thomas R. Monocyte-derived DC primed with TLR agonists secrete IL-12p70 in a CD40-dependent manner under hyperthermic conditions. J Immunother 2006;29:606–15
- Bannikov AV, Dorofeikov VV, Freidlin TS, Freidlin IS, Shustov EB, Shcherbak IG, et al. Characteristics of the acute phase reaction in humans with various types of autonomic nervous system regulation during simulated hyperthermia. Ross Fiziol Zh Im I M Sechenova 2000;86:41–5
- Hietala J, Nurmi T, Uhari M, Pakarinen A, Kouvalainen K. Acute phase proteins, humoral and cell mediated immunity in environmentally-induced hyperthermia in man. Eur J Appl Physiol Occup Physiol 1982;49:271–6
- Vandesompele J, De Preter K, Pattyn F, Poppe B, Van Roy N, De Paepe A, et al. Accurate normalization of real-time quantitative RT-PCR data by geometric averaging of multiple internal control genes. Genome Biol 2002;18:7
- Brunner E, Puri ML. Nonparametric methods in factorial designs. Stat Pap 2001;42:1–52
- Benjamini Y, Hochberg Y. On the adaptive control of the false discovery rate in multiple testing with independent statistics. J Educ Behav Stat 2000;25:60–83
- Hwang YT, Chu SK, Ou ST. Evaluations of FDR-controlling procedures in multiple hypothesis testing. Stat Comput 2011;21:569–83 www.rproject.org
- The R project for statistical computing. http://www.r-project.org/ (accessed 2 May 2013)
- Kraybill WG, Olenki T, Evans SS, Ostberg JR, O’Leary KA, Gibbs JF, et al. A phase I study of fever-range whole body hyperthermia (FR-WBH) in patients with advanced solid tumours: Correlation with mouse models. Int J Hyperthermia 2002;18:253–66
- Peer AJ, Grimm MJ, Zynda ER, Repasky EA. Diverse immune mechanisms may contribute to the survival benefit seen in cancer patients receiving hyperthermia. Immunol Res 2010;46:137–54
- Zellner M, Hergovics N, Roth E, Jilma B, Spittler A, Oehler R. Human monocyte stimulation by experimental whole body hyperthermia. Wien Klin Wochenschr 2002;114:102–7
- Milani V, Noessner E, Ghose S, Kuppner M, Ahrens B, Scharner A, et al. Heat shock protein 70: role in antigen presentation and immune stimulation. Int J Hyperthermia 2002;18:563–85
- Asea A, Kraeft SK, Kurt-Jones EA, Stevenson MA, Chen LB, Finberg RW. Hsp70 stimulates cytokine production through a CD14-dependant pathway, demonstrating its dual role as a chaperone and cytokine. Nat Med 2000;6:435–42
- Pockley AG. Heat shock proteins are regulators of the immune response. Lancet 2003;362(9382):469–76
- Singh IS, Hasday JD. Fever, hyperthermia and the heat shock response. Int J Hyperthermia 2013;29:423–35
- Yan X, Xiu F, An H, Wang X, Wang J, Cao X. Fever range temperature promotes TLR4 expression and signaling in dendritic cells. Life Sci 2007;80:307–13
- Zhao W, An H, Zhou J, Xu H, Yu Y, Cao X. Hyperthermia differentially regulates TLR4 and TLR2-mediated innate immune response. Immunol Lett 2007;108:137–42
- Ahlers O, Hildebrandt B, Dieing A, Deja M, Bohnke T, Wust P, et al. Stress induced changes in lymphocyte subpopulations and associated cytokines during whole body hyperthermia of 41.8−42.2 °C. Eur J Appl Physiol 2005;95:298–306
- Tarner IH, Muller-Ladner U, Uhlemann C, Lange U. The effect of mild whole-body hyperthermia on systemic levels of TNF-alpha, IL-1beta, and IL-6 in patients with ankylosing spondylitis. Clin Rheumatol 2009;28:397–402