Abstract
Advances in transcranial MRI-guided focused ultrasound have renewed interest in lesioning procedures in functional neurosurgery with a potential role in the treatment of neurological conditions such as chronic pain, brain tumours, movement disorders and psychiatric diseases. While the use of transcranial MRI-guided focused ultrasound represents a new innovation in neurosurgery, ultrasound has been used in neurosurgery for almost 60 years. This paper reviews the major historical milestones that have led to modern transcranial focused ultrasound and discusses current and evolving applications of ultrasound in the brain.
Introduction
Advances in transcranial MRI-guided focused ultrasound have renewed interest in lesioning procedures in functional neurosurgery with a potential role in the treatment of neurological conditions such as chronic pain, brain tumours, movement disorders and psychiatric diseases. This paper reviews the major historical milestones that have led to modern transcranial focused ultrasound and discusses current and evolving applications of ultrasound in the brain.
Historical use of ultrasound in the brain
William and Francis Fry from the Bioacoustics Laboratory at the University of Illinois were the first to apply ultrasound for therapeutic uses in the brain during the 1950s. This was a rapidly evolving era of stereotactic neurosurgery, and neurosurgeons used a variety of methods to achieve discrete, highly localised treatments including cryogenic methods, radiofrequency heating, and chemical infusions to treat severely disabling movement disorders [Citation1–4]. In their pioneering work, Fry and colleagues used high intensity focused ultrasound (HIFU) to produce discrete lesions in feline and primate brain without disturbing neighbouring tissue [Citation5,Citation6]. These studies laid the foundation for focused ultrasound lesioning in the human brain. The Fry brothers partnered with a neurosurgeon, Russell Meyers of the State Hospital of Iowa, to translate focused ultrasound technology into the treatment of patients suffering from debilitating Parkinson’s disease (PD) and choreoathetosis. They lesioned the pallidofugal tracts with focused ultrasound to treat severe tremor and rigidity of PD [Citation7] and the choreoathetotic movements in cerebral palsy and thalamic pain [Citation8]. Although symptom relief from these procedures was partial, these studies were among the first applications of focused ultrasound in the treatment of human diseases of the brain. Even though deep brain targets could be lesioned without surgically entering the brain, focused ultrasound required a craniotomy to produce an acoustic window, which hindered its widespread acceptance in neurosurgery.
A few human clinical studies utilised developing ultrasound technology with a craniotomy. Capitalising on the less invasive nature of focused ultrasound, Lindstrom [Citation9] performed frontal lobotomies by only sonicating the prefrontal lobe for patients with intractable pain secondary to advanced metastatic cancer. Here, 25 patients were treated with focused ultrasound – through a 1 to 1.5-inch-wide (2.54 cm to 3.81 cm wide) trephination – with a frequency of 1000 kHz from 2 to 14 min to an area of the brain just anterior to the frontal horns of the lateral ventricles. All patients experienced an initial relief of pain, but this was short lived, with only two patients pain free at 12 months. Post mortem histological analysis showed white matter necrosis in nine patients and no changes in the other 16. There was no correlation between necrosis and pain relief.
Fry also collaborated with Robert Heimburger, from the University of Indiana, to perform ultrasound ablations for malignant gliomas. A computer-controlled focused ultrasound apparatus was used after craniotomy to more precisely target selected brain lesions, in this instance for the treatment of glioblastoma [Citation10]. The clinical outcomes with glioblastoma were mixed, although two patients in particular had what could be considered positive outcomes with survival of 17 and 5 years following treatment [Citation11].
Transcranial focused ultrasound
One of the critical challenges in delivery of ultrasound to the brain is that the skull reflected or absorbed a substantial portion (>90%) of the energy delivered [Citation12] – hence the need for craniotomy in all of the early focused ultrasound trials.
Interest in focused ultrasound lesioning of the brain has been renewed with recent technological advances in ultrasound transducer design [Citation13] along with developments in computed tomography which have allowed for prediction of ultrasound distortion by the human skull and subsequent phase correction algorithms to correct for ultrasound distortion [Citation14,Citation15]. Such developments have made transcranial-focused ultrasound possible where in the past a craniotomy was required. Additional advances in high resolution MRI and MR thermography have allowed for precise targeting and monitoring during sonication [Citation16]. Prior work has further determined the optimal frequency for transcranial-focused ultrasound with lower frequencies exhibiting a higher transcranial transmission rate than higher frequencies [Citation17].
The first clinical trial with this contemporary MRI-guided focused ultrasound transducer system was still performed through a craniotomy window. Ram et al. [Citation18] treated three patients with recurrent glioblastoma. Brief sonications reaching peak MRI voxel temperatures between 60° and 90 °C were delivered to tumour volumes of 9 cm3, 25 cm3, and 5 cm3, respectively. Two of the three patients had a favourable response to focused ultrasound with resolution of pre-operative tumour contrast enhancement, and treatment failure in the third patient was attributed possibly to energy absorption from a previously implanted dural substitute that could harbour tiny air bubbles. Importantly, this small study provided histological evidence of malignant brain tumour tissue ablation in humans by high intensity focused ultrasound.
Current brain applications of focused ultrasound
Brain tumours
The first truly transcranial MR-guided focused ultrasound treatments through the intact human skull were by McDannold et al. [Citation19] targeting deep-seated gliomas. In this study, three patients with recurrent and refractory glioblastoma were targeted with MR-guided focused ultrasound using an operating frequency of 650 kHz. In this preliminary study, the authors measured the temperature of each sonication with MR thermometry, but only achieved peak voxel temperatures from 42 °C to 51 °C. The acoustic energy delivered in these treatments did not result in tissue ablation. The authors speculated that longer and/or more sonications could have been performed, but they wanted to be conservative with these initial treatments which were intended to establish the feasibility of transcranial MR-guided focused ultrasound for patients with cerebral malignancy.
Neuropathic pain
Martin and colleagues [Citation20] used a next-generation, transcranial MR-guided focused ultrasound system with 1024 elements at 650 kHz to target the centrolateral thalamus in the treatment of therapy-resistant neuropathic pain. This was the first demonstration of human brain tissue ablation with acoustic energy delivered through the intact skull. Nine patients suffered from a wide array of pain syndromes including phantom limb pain status post-amputation, schwannoma, radiculopathy, brachial plexus injury, post-thalamic infarct pain syndromes, and idiopathic trigeminal neuralgia. Bilateral centrolateral thalamotomies without adverse clinical effects were created with peak temperatures ranging from 51–60 °C. In the immediate post-procedure period, the authors reported a mean pain relief of 68% (range 30–100%). Jeanmonod et al. [Citation21] reported longer-term clinical outcomes with an average improvement in pain of 49% at 3 months and 57% at 1 year. A single intracerebral haemorrhage occurred at the Central Lateral nucleus of Thalamus (CLT) target resulting in dysmetria and neglect. Interestingly, this patient had the highest maximal temperature achieved (64 °C) with sonication, while another with the same degree of heating had no complication.
Essential tremor
Essential tremor, the most common movement disorder, is characterised by a postural and/or kinetic tremor. Patients with severe essential tremor are often unable to perform simple activities of daily living, leading to a lower perceived health status and severe impairment in quality of life [Citation22,Citation23]. In a recent pilot study by Elias et al. [Citation24], 15 patients with severe, medication-refractory, essential tremor were treated with unilateral MR-guided focused ultrasound targeted to the ventral intermediate thalamus. These patients experienced a 75% reduction in contralateral hand tremor, and an 85% reduction in disability and improved quality of life measurements at 1 year. Common transient adverse events included paresthesias of the mouth and/or fingers, ataxia or feelings of unsteadiness, and headaches. The most serious side effect involved a single patient with a dysesthesia of his treated index finger, while three other patients had mild but persistent paresthesias.
Chang et al. also performed unilateral Vim thalamotomy with MR-guided focused ultrasound in 11 patients with severe essential tremor [Citation25]. At 6-month follow-up, eight of the patients exhibited improved tremor. Treatment failure in the three other patients was attributed to an inability to achieve sufficient thermal dose at the target. Insufficient heating of the thalamic target was attributed to varying skull characteristics impeding the efficient transmission of transcranial acoustic energy. Lipsman et al. also recently reported results using MR guided focused ultrasound for essential tremor [Citation26]. In their series of four patients, all patients had improvement in tremor at 3-month follow-up and one patient experienced persistent paresthesias of the thumb and index finger.
The development of transcranial MRI-guided focused ultrasound has rekindled an interest in stereotactic lesioning. Deep brain stimulation remains the primary surgical option for the treatment of movement disorders as it can be implemented as an adjustable, reversible therapy for bilateral symptomatology. While a MR-guided focused ultrasound thalamotomy represents an ablative procedure that is currently restricted to the treatment of unilateral symptoms, it does offer the attractive option of avoiding craniotomy and the morbidity inherent in an implanted device. An international multicentre, randomised controlled trial is currently underway to further investigate the efficacy and safety of unilateral MR-guided focused ultrasound thalamotomy for essential tremor.
Parkinson’s disease
PD is a severely debilitating, progressive, neurodegenerative disease characterised by tremor, rigidity, akinesia, and postural instability. Like essential tremor, current surgical treatments for PD primarily involve deep brain stimulation, targeting the subthalamic nucleus or globus pallidus internus. The primary outflow of these basal ganglia nuclei is the pallidothalamic tract (PTT), which includes the fasciculus lenticularis and ansa lenticularis. Recently Magara et al. [Citation27] used unilateral transcranial MR-guided focused ultrasound to target the pallidothalamic tract in 13 patients with PD. The average temperature achieved at the target was 56.2 °C (range 52–59 °C). For the first four patients, peak energy was applied only once to the PTT target with an average lesion volume of 83 mm3. The next nine patients were treated four times at target with peak energies, and their average lesion size was 172 mm3. While lesion size appeared similar on immediate post-procedural MRI, lesions in the former group were not apparent at 3 months. Clinical outcomes were determined with the Unified Parkinson’s Disease Rating Scale (UPDRS). Among the first four patients (peak energy sonication applied only once), UPDRS improvement was modest at 7.6%. However, the nine patients who received four peak energy sonications improved by 60.9%, similar to the magnitude of symptom relief with deep brain stimulation surgery. This work suggests maximal intensity and number of sonications plays a role in the development of therapeutic lesions. While current work has focused on the tremor aspect of PD, the next step in the treatment of PD will be the treatment of non-tremulous motor symptoms by targeting the globus pallidus internus (GPi) and subthalamic nucleus (STN) – two standard PD target of deep brain stimulation.
Vascular neurosurgery
Stroke is one of the leading causes of death and disability in the USA and other developed countries. Intracerebral (extravascular) haemorrhagic (ICH) stroke represents approximately 10% of all strokes and has mortality rates ranging from 40–60%. Current surgical treatments for haemorrhagic stroke are largely unsuccessful in improving mortality or quality of life. Harnoff et al. [Citation28] hypothesised that focused ultrasound could be used to less invasively lyse intracerebral hematoma and hasten recovery. In an in vivo experiment, focused ultrasound was used to sonothrombolyse an artificial intracerebral haematoma in a large brain model. Swine were trephined and fresh blood (3 mL) was introduced into the brain parenchyma to simulate a lobar intracerebral haemorrhage. The animal’s head was subsequently placed in an MR-guided focused ultrasound unit and sonicated through the bone window with a 5-s pulse with a frequency of 230 kHz and a power of 700 W. Limited data is available regarding the temperature achieved in the sonications, however the authors do state the clot liquefaction occurred without a significant temperature increase. A second treatment arm used a porcine brain ICH model with a cadaveric human skull cap between the intracerebral haemorrhage and the ultrasound transducer to simulate transcranial delivery. As in the treatment arm without the human skull cap, sonication was able to generate clot liquefaction. Treatment parameters were the same as in the porcine model without the intervening human skull cap with the only difference being that to achieve transcranial sonication a power of 3000 W was utilised. Sonications were performed in times ranging from 2–24 h from intracerebral blood injection. These animal studies demonstrate the feasibility of transcranial focused ultrasound therapy for the treatment of ICH.
Building upon existing animal models, Monteith et al. [Citation29] studied intracerebral clot lysis with focused ultrasound in human cadaver preparations. In this study of 10 cadavers, ICH was simulated by injecting 40 mL of fresh venous blood into deep cerebral structures (basal ganglia and thalamus). Clot lysis was achieved in 4/5 cadaveric specimens using transcranial focused ultrasound at a frequency of 230 kHz and high energy sonications (3950 W for 30 s). The single failure was attributed to dissolved gas in the specimen secondary to decay. In two of the treated cadavers, the liquefied clot was aspirated with MRI guidance to simulate clinical feasibility.
These studies provide early preclinical evidence to demonstrate the feasibility of using transcranial focused ultrasound for intracerebral clot lysis for haemorrhagic stroke. Similar preclinical studies are being investigated for intraventricular haemorrhage in premature neonates. Active human clinical trials are not yet underway.
There is also an interest in applying focused ultrasound treatment for acute (intravascular) ischaemic stroke. Early work in the field of intravascular sonothrombolysis shows promising results in both in vitro and in vivo models, but these studies have not yet been performed transcranially [Citation30]. Holscher and colleagues [Citation31] have used a rabbit carotid artery model and ex vivo human skull to simulate human middle cerebral artery occlusion. Ex vivo experiments were additionally performed with blood clot generated in a polyethylene test tube, placed behind human cranium. The authors concluded that minimal clot fragmentation occurs at power levels lower than 400 W, and that less sonothrombolysis occurs in the in vivo rabbit occlusion than the ex vivo experimental model. Burgess et al. [Citation32] further advanced the field through their in vivo experiments treating thrombosed middle cerebral arteries in a rabbit model. Here there were three separate treatment groups: one group with sonications using 275 W of acoustic power (n = 3), one group with sonications using 415 W of acoustic power (n = 4), and another group with sonications using 550 W of acoustic power (n = 7). Post-sonication MRI and angiograms were performed which showed no recanalisation occurred in the group with 275 W of acoustic power. Of the remaining groups, half of the animals treated with 415 W demonstrated recanalisation and in the final group treated with 550 W, five of seven animals demonstrated recanalisation. Histological analysis of the treated middle cerebral artery was further performed to assess for any potential vascular injury induced by sonication. Animals treated with 415 W demonstrated no histological changes suggestive of tissue damage or haemorrhage post-sonication. Of the five animals with reperfusion in the 550-W group, only one animal exhibited contrast extravasation around the treated middle cerebral artery. Here the authors showed a relation between increased acoustic power and incidence of arterial recanalisation. Increased acoustic power is not, however, without consequence, as demonstrated by the occurrence of contrast extravasation in the 550-W group, possibly suggestive of reperfusion injury. More investigation will be needed to establish the ideal acoustic power for intravascular sonothrombolysis.
Figure 1. Current and future targets for MR-guided focused ultrasound. Ventral intermediate nucleus of the thalamus (VIM) for treatment of essential tremor. Pallidothalamic tract (PTT) for treatment of Parkinson’s disease (PD). Central lateral nucleus of thalamus (CL) for treatment of chronic pain. Anterior limb of internal capsule (ALIC) for treatment of OCD. Globus pallidus internus (GPi) for possible treatment of PD. Subthalamic nucleus (STN) for possible treatment of PD.
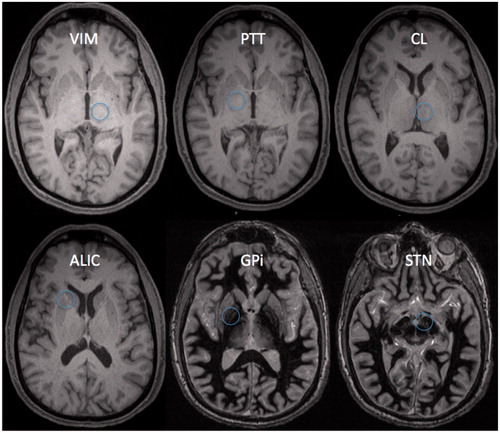
Table1. Clinical trials of focused ultrasound for movement disorders.
Trigeminal neuralgia
Trigeminal neuralgia is characterised by severe episodic attacks of lancinating, facial pain. Many of the surgical treatments for medication-resistant symptoms involve ablating the trigeminal nerve with RF heating, balloon compression, chemical gangliolysis, or stereotactic radiosurgery. Theoretically focused ultrasound could target the same cisternal portion of the trigeminal nerve as Gamma Knife radiosurgery; however, there are concerns for collateral heating of the skull base and adjacent, critical neural structures. Monteith et al. [Citation33] investigated the feasibility of focused ultrasound treatments for trigeminal neuralgia in a cadaveric study. Six trigeminal nerves from four cadavers were treated with an average increase in temperature of 10 °C (range 6–18 °C). The mid-cisternal segment of the trigeminal nerve and the root entry zone adjacent to the brainstem were targeted. Average temperature increases of the internal acoustic canal (IAC), which houses the vestibulocochlear nerve, were 16.7 °C when targeting the mid-cisternal trigeminal segment and 16.3 °C when targeting the Root Entry Zone (REZ). Using element blocking techniques to shield the petrous bone from acoustic energy, average temperature increases in the IAC decreased to 5.7 °C (mid-cisternal trigeminal target) and 4.9 °C (REZ trigeminal target). Trigeminal heating was also reduced, however, when using petrous blocking techniques with peak temperature increases ranging from 7.3–7.8 °C. The authors here show that collateral heating of the skull base can occur during focused ultrasound treatment of the trigeminal nerve but that this heating can be mitigated by blocking techniques. Although this study is anatomically useful, the cadaver skull is likely a poor physiological model since tissue heating and cooling within the brain is largely dependent on blood and CSF flow. While temperature elevations in the trigeminal nerve were seen, more work will need to be done to determine whether or not these physiological factors significantly contribute to clinically significant treatments along the trigeminal nerve.
Additional applications: blood–brain barrier disruption
As research surrounding MR-guided focused ultrasound progresses, additional innovative uses of the technology are becoming apparent. The blood–brain barrier (BBB) is a physical barrier between blood vessels and the extracellular compartment of the brain, preventing molecules larger than 400–500 Da from passing from the blood stream to brain tissue [Citation34]. While this physical barrier prevents harmful toxins from reaching the brain, it also prevents effective delivery of therapeutic agents for treatment of central nervous system (CNS) disease. Numerous strategies to penetrate the BBB have been posited, which include direct catheter penetration, convection-enhanced delivery [Citation35], and alterations in BBB permeability via intra-arterial infusions of hypertonic solutions [Citation36,Citation37]. To date these techniques have been either invasive or lacking in specificity, causing diffuse disruption in the BBB, limiting widespread use. This in theory could lead to adverse effects for areas of the brain outside the treatment target.
The concept of BBB disruption using focused ultrasound dates back to initial studies in the 1950s where several groups noted BBB disruption as evidenced by extravasation of dye from cerebral blood vessels without histological changes seen in the blood vessels [Citation38,Citation39]. Notably, these studies were conducted with HIFU for lesional procedures in the brain. Since then, multiple groups [Citation40–Citation42] have demonstrated the feasibility of focal and transient BBB disruption without permanent tissue destruction using focused ultrasound and intravenous injection of microbubbles. The non-invasive nature of MR-guided focused ultrasound and its ability to precisely alter BBB permeability in a focal manner represents significant improvements on prior attempts to disrupt the BBB. The mechanism through which focused ultrasound and microbubbles disrupts the BBB is thought to be secondary to mechanical effects of ultrasound rather than its thermal effects. It is thought that low intensity focused ultrasound of the vascular system with gas-filled microbubbles induces a stable state in which the microbubbles oscillate within the ultrasound field. This state has been termed ‘stable cavitation’. Mechanical oscillation of the microbubbles against the blood vessel wall leads to stretching of the endothelial walls, allowing for transient BBB disruption [Citation34,Citation43,Citation44].
This has potentially important implications for the treatment of CNS diseases and malignancies where therapeutic agents could be ‘leaked’ into the brain’s extracellular space. While initial study in the field of BBB disruption primarily dealt with chemotherapeutic delivery to the CNS [Citation45–47], it appears that alterations in BBB permeability may lend itself to immune-mediated treatment of neurodegenerative diseases. Jordao et al. [Citation48] treated mice with MRI-guided focused ultrasound followed by intravenous administration of anti-Aβ antibodies. Compared to non-focused ultrasound treated mice, those treated with ultrasound demonstrated reduced Aβ plaques. This therapeutic strategy with focused ultrasound disruption of the BBB could have implications for neurodegenerative conditions such as Alzheimer’s disease and PD. Currently, focused ultrasound-mediated BBB disruption remains investigational with research aimed at better understanding the use of microbubbles, repetitive sonications in the case of chemotherapy administration, and tissue penetration of therapeutics reliant on a diffusion gradient.
Obsessive–compulsive disorder
Preliminary investigations are also underway in the neurosurgical treatment of psychiatric disease. Gamma Knife lesioning of the anterior limb of the internal capsule (ALIC) has been suggested to be a successful treatment for medically refractory obsessive–compulsive disorder (OCD) [Citation49–51]. Chang et al. are currently investigating the feasibility and safety of focused ultrasound treatments for OCD [Citation52]. In a series of six patients, focused ultrasound anterior capsulotomies were performed bilaterally using an operating frequency of 650 kHz. The authors repetitively treated the anterior capsule 14.6 times with a mean temperature of 53.6 °C. At final follow-up, average lesion size at 6-month follow-up was 51.5 mm3, with lesions present in five out of six patients. Additionally, the authors compared these radiographic results of focused ultrasound lesions in a white matter tract (ALIC) to their thalamic lesions for essential tremor. They noted a difference in the appearance of the lesions on MRI with thalamotomies being clearly visible during and immediately following sonication, while focused ultrasound anterior capsulotomies were not as visible in the early peri-procedural period. The authors posit that white matter tract lesions may evolve from demyelination during the later subacute and chronic periods of injury. This is in contradistinction to lesioning of thalamus – a grey matter – structure in which thermal lesioning leads to more direct damage.
Neuromodulation
One of the most exciting potential applications of transcranial focused ultrasound is neuromodulation for non-invasive brain mapping. Early studies investigating the effects of ultrasound on living tissues demonstrated that acoustic energy can influence the activity of electrically excitable tissues including muscle and peripheral nerve. In the central nervous system, HIFU has been used to reversibly inhibit neuronal activity by thermal mechanisms below the temperature threshold for tissue ablation. Fry et al. sonicated the lateral geniculate nucleus in a feline model with low doses of HIFU that temporarily suppressed visual evoked responses in the absence of tissue ablation [Citation5,Citation53]. These studies were among the first to demonstrate that ultrasound could induce reversible changes to intact neural circuits. More recently, low intensity focused ultrasound (LIFU) has been used to transiently activate and depress neuronal function through non-thermal mechanisms. Several groups using rodent models have reported that brief, pulsed LIFU can activate somatomotor cortical circuits resulting in a variety of dramatic motor behaviours [Citation54–58]. LIFU neuromodulation has also been used to modulate cortical responses in the frontal eye fields of non-human primates [Citation59] and the somatosensory cortex of humans [Citation60]. Despite the exciting recent advances in LIFU neuromodulation, its mechanism of action remains unknown. However, one of the leading hypotheses is that LIFU changes neuronal membrane capacitance by 1) mechanically altering voltage-sensitive ion channels [Citation61], or 2) altering membrane permeability by stable cavitation or acoustic streaming, which eventually leads to discrete bursts of action potentials in the targeted tissue [Citation62].
Neuromodulation with LIFU offers several theoretical advantages over other current neuromodulation and brain-mapping technologies. Transcranial LIFU is non-invasive. It offers a high degree of spatial resolution and can be used to target superficial and deep cerebral structures. Despite these theoretical advantages, few studies have been conducted in large animal models or humans to clearly demonstrate the full capabilities of neuromodulation with low intensity ultrasound.
Conclusion
Focused ultrasound has evolved significantly in the past 60 years. Advances in imaging and transducer technology have allowed more accurate and less invasive treatments. Such advances have opened the door for clinical trials in neurological disorders and for research with other conditions such as haemorrhagic and ischaemic stroke, and drug delivery. Lastly, a potentially powerful use of transcranial-focused ultrasound would be for non-invasive brain mapping.
Declaration of interest
The authors report no conflicts of interest. The authors alone are responsible for the content and writing of the paper.
References
- Cooper IS. Neurosurgical alleviation of intention tremor of multiple sclerosis and cerebellar disease. N Engl J Med 1960;236:441–4
- Cooper IS. Chemopallidectomy: An investigative technique in geriatric Parkinsonians. Science 1955;121:217–18
- Narabayashi H, Shimazu H, Fujita Y, Shikiba A, Nagao T, Nagahata M. Procaine-oil-wax pallidotomy for double athetosis and spastic states in infantile cerebral palsy: Report of 80 cases. Neurology 1960;10:61–9
- Sweet WH, Mark VH, Hamlin H. Radiofrequency lesions in the central nervous system of man and cat: Including case reports of eight bulbar pain-tract interruptions. J Neurosurg 1960;17:213–25
- Fry WJ, Barnard JW, Fry FJ, Brennan JF. Ultrasonically produced localized selective lesions in the central nervous system. Am J Phys Med 1955;34:413–23
- Fry WJ, Mosberg WH Jr, Barnard JW, Fry FJ. Production of focal destructive lesions in the central nervous system with ultrasound. J Neurosurg 1954;11:471–8
- Meyers R, Fry WJ, Fry FJ, Dreyer LL, Schultz DF, Noyes RF. Early experiences with ultrasonic irradiation of the pallidofugal and nigral complexes in hyperkinetic and hypertonic disorders. J Neurosurg 1959;16:32–54
- Meyers R, Fry FJ, Fry WJ, Eggleton RC, Schultz DF. Determination of topologic human brain representations and modifications of signs and symptoms of some neurologic disorders by the use of high level ultrasound. Neurology 1960;10:271–7
- Nelson E, Lindstrom PA, Haymaker W. Pathological effects of ultrasound on the human brain. A study of 25 cases in which ultrasonic irradiation was used as a lobotomy procedure. J Neuropathol Exp Neurol 1959;18:489–508
- Heimburger RF. Ultrasound augmentation of central nervous system tumor therapy. Indiana Med 1985;78:469–76
- Heimburger RF, Heimburger DC. Reflections on a career in neurosurgery. Surg Neurol Int 2013;4:89
- Pinton G, Aubry JF, Bossy E, Muller M, Pernot M, Tanter M. Attenuation, scattering, and absorptions of ultrasound in the skull bone. Med Phys 2012;39:299–307
- Hynynen K, Clement GT, McDannold N, Vykhodtseva N, King R, White PJ, et al. 500-element ultrasound phased array system for noninvasive focal surgery of the brain: A preliminary rabbit study with ex vivo human skulls. Magn Reson Med 2004;52:100–7
- Clement GT, Hynynen K. A noninvasive method for focusing ultrasound through the human skull. Phys Med Biol 2002;47:1219–36
- Clement GT, Hynynen K. Correlation of ultrasound phase with physical skull properties. Ultrasound Med Biol 2002;28:617–24
- Ishihara Y, Calderon A, Watanabe H, Okamoto K, Suzuki Y, Kuroda K, et al. A precise and fast temperature mapping using water proton chemical shift. Magn Reson Med 1995;34:814–23
- Sun J, Hynynen K. The potential of transskull ultrasound therapy and surgery using the maximum available skull surface area. J Acoust Soc Am 1999;105:2519–27
- Ram Z, Cohen ZR, Harnof S, Tal S, Faibel M, Nass D, et al. Magnetic resonance imaging-guided, high intensity focused ultrasound for brain tumor therapy. Neurosurgery 2006;59:949–55
- McDannold N, Clement GT, Black P, Jolesz F, Hynynen K. Transcranial magnetic resonance imaging-guided focused ultrasound surgery of brain tumors: Initial findings in 3 patients. Neurosurgery 2010;66:323–32
- Martin E, Jeanmonod D, Morel A, Zadicario E, Werner B. High-intensity focused ultrasound for noninvasive functional neurosurgery. Ann Neurol 2009;66:858–61
- Jeanmonod D, Werner B, Morel A, Michels L, Zadicario E, Schiff G, et al. Transcranial magnetic resonance imaging-guided focused ultrasound: Non-invasive central lateral thalamotomy for chronic neuropathic pain. Neurosurg Focus 2012;32:E1
- Woods SP, Scott JC, Fields JA, Poquette A, Troster AL. Executive dysfunction and neuropsychiatric symptoms predict lower health status in essential tremor. Cog Behav Neurol 2008;21:28–33
- Lorenz D, Schwieger D, Moises H, Deuschl G. Quality of life and personality in essential tremor patients. Mov Disord 2006;21:1114–18
- Elias WJ, Huss D, Voss T, Loomba J, Khaled M, Zadicario E, et al. A pilot study of focused ultrasound thalamotomy for essential tremor. N Engl J Med 2013;369:640–8
- Chang WS, Jung HJ, Kweon EJ, Zadicario E, Rachmilevitch I, Chang JW. Unilateral magnetic resonance guided focused ultrasound thalamotomy for essential tremor: Practice and clinicoradiologic outcomes. J Neurol Neurosurg Psychiatry 2014 May Pii: jnnp-2014-307642
- Lipsman N, Schwartz ML, Huang Y, Lee L, Sankar T, Chapman M, et al. MR-guided focused ultrasound thalamotomy for essential tremor: A proof-of-concept study. Lancet Neurol 2013;12:462–8
- Magara A, Buhler R, Moser D, Kowalski M, Pourtehrani R, Jeanmonod D. First experience with MR-guided focused ultrasound in the treatment of Parkinson’s disease. J Ther Ultrasound 2014;2:11
- Harnoff S, Zibly Z, Hananel A, Monteith S, Grinfeld J, Schiff G, et al. Potential of magnetic resonance-guided focused ultrasound for intracerebral hemorrhage: An in vivo feasibility study. J Stroke Cerebrovasc Dis 2014;26:1585–91
- Monteith SJ, Harnof S, Medel R, Popp B, Wintermark M, Lopes MB, et al. Minimally invasive treatment of intracerebral hemorrhage with magnetic resonance-guided focused ultrasound. J Neurosurg 2013;118:1035–45
- Wright C, Hynynen K, Goertz D. In vitro and in vivo high-intensity focused ultrasound thrombolysis. Invest Radiol 2012;47:217–25
- Holscher T, Ahadi G, Fisher D, Zadicario E, Voie A. MR-guided focused ultrasound for acute stroke: A rabbit model. Stroke 2013;44:S58–60
- Burgess A, Huang Y, Waspe A, Ganguly M, Goertz D, Hynynen K. High-intensity focused ultrasound (HIFU) for dissolution of clots in a rabbit model of embolic stroke. PLoS One 2012;7:e42311
- Monteith SJ, Medel R, Kassel NF, Wintermark M, Eames M, Snell J, et al. Transcranial magnetic resonance-guided focused ultrasound surgery for trigeminal neuralgia: A cadaveric and laboratory feasibility study. J Neurosurg 2013;118:319–29
- Vykhodtseva N, McDannold N, Hynynen K. Progress and problems in the application of focused ultrasound for blood–brain barrier disruption. Ultrasonics 2008;48:279–96
- Bobo RH, Laske DW, Akbasak A, Morrison PF, Dedrick RL, Oldfield EH. Convection-enhanced delivery of macromolecules in the brain. Proc Natl Acad Sci USA 1994;91:2076–80
- Neuwelt EA, Frenkel EP, Diehl JT, Maravilla KR, Vu LH, Clark WK, et al. Osmotic blood–brain barrier disruption: A new means of increasing chemotherapeutic agent delivery. Trans Am Neurol Assoc 1979;104:225–60
- Neuwelt EA, Balaban E, Diehl J, Hill S, Frenkel E. Successful treatment of primary central nervous system lymphomas with chemotherapy after osmotic blood–brain barrier opening. Neurosurgery 1983;12:662–71
- Barnard JW, Fry WJ, Fry FJ, Krumins RF. Effects of high intensity ultrasound on the central nervous system of the cat. J Comp Neurol 1955;103:459–84
- Bakay L, Hueter TF, Ballantine Jr HT, Sosa D. Ultrasonically produced changes in the blood–brain barrier. AMA Arch Neurol Psychiatry 1956;76:457–67
- Wei KC, Tsai HC, Lu YJ, Yang HW, Hua MY, Wu MF, et al. Neuronavigation-guided focused ultrasound-induced blood–brain barrier opening: A preliminary study in swine. Am J Neuroradiol 2013;34:115–20
- Kinoshita M, McDannold N, Jolesz F, Hynynen K. Targeted delivery of antibodies through the blood–brain barrier by MRI-guided focused ultrasound. Biochem Biophys Res Commun 2006;340:1085–90
- Hynynen K, McDannold N, Vykhodtseva N, Jolesz F. Noninvasive MRI-guided focal opening of blood–brain barrier in rabbits. Radiology 2001;220:640–6
- Colen RR, Jolesz F, eds. MR-Guided Focused Ultrasound of the Brain. Berlin: Springer, 2012
- Cho EE, Drazic J, Ganguly M, Stefanovic B, Hynynen K. Two-photon fluorescence microscopy study of cerebrovascular dynamics in ultrasound-induced blood–brain barrier opening. J Cereb Blood Flow Metab 2011;31:1852–62
- Kinoshita M, McDannold N, Jolesz FA, Hynynen K. Noninvasive localized delivery of Herceptin to the mouse brain by MRI-guided focused ultrasound-induced blood–brain barrier disruption. Proc Natl Acad Sci USA 2006;103:11719–23
- Wei KC, Chu PC, Wang HY, Huang CY, Chen PY, Tsai HC, et al. Focused ultrasound-induced blood–brain barrier opening to enhance temozolomide delivery for glioblastoma treatment: A preclinical study. PLoS One 2013;8:e58995
- Treat LH, McDannold N, Zhang Y, Vykhodtseva N, Hynynen K. Improved anti-tumor effect of liposomal doxorubicin after targeted blood–brain barrier disruption by MRI-guided focused ultrasound in rat gliomas. Ultrasound Med Biol 2012;38:1716–25
- Jordao J, Ayala-Grosso C, Marham K, Huang Y, Chopra R, McLaurin J, et al. Antibodies targeted to the brain with image-guided focused ultrasound reduced amyloid-β plaque load in the TgCRND8 mouse model of Alzheimer’s disease. PLoS One 2010;11:e10549
- Sheehan JP, Patterson G, Schlesinger D, Xu Z. γ knife surgery anterior capsulotomy for severe and refractory obsessive–compulsive disorder. J Neurosurg 2013;119:1112–18
- Kondziolka D, Flickinger JC, Hudak R. Results following gamma knife radiosurgical anterior capsulotomies for obsessive–compulsive disorder. Neurosurgery 2011;68:28–32
- Lopes AC, Greenber BD, Canteras MM, Batistuzzo MC, Hoexter MQ, Gentil AF, et al. Gamma ventral capsulotomy for obsessive–compulsive disorder: A randomized clinical trial. JAMA Psychiatry 2014;71:1066–76
- Jung HH, Chang WS, Rachmitevitch I, Tlusty T, Zadicario E, Chang JW. Different magnetic resonance imaging patterns after transcranial magnetic resonance-guided focused ultrasound of the ventral intermediate nucleus of the thalamus and anterior limb of the internal capsule in patients with essential tremor or obsessive–compulsive disorder. J Neurosurg 2014;24:1–7
- Fry FJ, Ades HW, Fry WJ. Production of reversible changes in the central Nervous system by ultrasound. Science 1958;127:83–4
- King RL, Brown JR, Pauly KB. Localization of ultrasound-induced in vivo neurostimulation in the mouse model. Ultrasound Med Biol 2014;40:1512–22
- King RL, Brown JR, Newsome WT, Pauly KB. Effective parameters for ultrasound-induced in vivo neurostimulation. Ultrasound Med Biol 2013;39:312–31
- Younan Y, Deffieux T, Larrat B, Fink M, Tanter M, Aubry JF. Influence of the pressure field distribution in transcranial ultrasonic neurostimulation. Med Phys 2013;40:082902
- Tufail Y, Matyushov A, Baldwin N, Tauchmann ML, Georges J, Yoshihiro A, et al. Transcranial pulsed ultrasound stimulates intact brain circuits. Neuron 2010;66:681–94
- Yoo SS, Bystritsky A, Lee JH, Zhang Y, Fischer K, Min BK. Focused ultrasound modulates region-specific brain activity. Neuroimage 2011;56:1267–75
- Deffieux T, Younan Y, Wattiez N, Tanter M, Pouget Aubry JF. Low-intensity focused ultrasound modulates monkey visuomotor behavior. Curr Biol 2013;23:2430–3
- Legon W, Sato TF, Opitz A, Mueller J, Barbour A, Williams A, et al. Transcortical focused ultrasound modulates the activity of primary somatosensory cortex in humans. Nat Neurosci 2014;17:322–9
- Tyler WJ, Tufail Y, Finsterwald M, Tauchmann ML, Olson EJ, Majestic C. Remote excitation of neuronal circuits using low-intensity, low-frequency ultrasound. PLoS One 2008;3:e3511
- Krasovitski B, Frenkel V, Shoham S, Kimmel E. Intramembrane cavitation as a unifying mechanism for ultrasound-induced bioeffects. Proc Natl Acad Sci USA 2011;108:3258–63