Abstract
Background Chronic gastrointestinal (GI) morbidity occurs in ≥50% of patients after external beam radiotherapy (EBRT) for carcinoma of prostate (CaP). This prospective, longitudinal study examines which baseline measurements of: 1) homocysteine and micronutrients in plasma; 2) chromosome damage/misrepair biomarkers; and 3) anal and rectal dose volume metrics predict GI morbidity after EBRT.
Patients and methods In total, 106 patients with CaP had evaluations of GI symptoms (modified LENT-SOMA questionnaires) before EBRT and at one month, one, two and three years after its completion. Other variables measured before EBRT were: 1) plasma concentrations of homocysteine and micronutrients including caroteinoids and selenium; 2) chromosome damage/DNA misrepair (micronuclei/nucleoplasmic bridge) indices; and 3) mean anal and rectal wall doses and volumes of anal and rectal walls receiving ≥40 Gy and ≥60 Gy. Univariate and multivariate analyzes examined the relationships among: 1) plasma levels of homocysteine and micronutrients; 2) indices of chromosome damage/DNA misrepair; and 3) mean anal and rectal wall doses and volumes of anal and rectal walls receiving ≥40 Gy and ≥60 Gy and total GI symptom scores from one month to three years after EBRT.
Results Increased frequency and urgency of defecation, rectal mucous discharge and bleeding after EBRT resulted in sustained rises in total GI symptom scores above baseline at three years. On univariate analysis, total GI symptom scores were significantly associated with: 1) plasma selenium and α tocopherol; 2) micronuclei indices of DNA damage; 3) mean anal and rectal wall doses; and 4) volumes of anal and rectal wall receiving ≥40 Gy and ≥60 Gy (p = 0.08–<0.001). On multivariate analysis, only volume of anal wall receiving ≥40 Gy was significant for increased GI symptoms after EBRT (p < 0.001).
Conclusion The volume of anal wall receiving ≥40 Gy predicts chronic GI morbidity after EBRT for CaP.
External beam radiotherapy (EBRT) is the preferred treatment especially for older men with carcinoma of the prostate (CaP) [Citation1]. Despite advanced EBRT techniques including intensity modulated radiotherapy (IMRT), acute radiation proctitis, characterized by increased frequency and urgency of defecation, fecal incontinence and rectal bleeding still occurs in 73% of patients during IMRT for CaP [Citation2]. The prevalence of chronic radiation proctitis (CRP), defined as persistence of anorectal symptoms ≥3 months after EBRT, ranges between 5% and 65% [Citation2]. Studies reporting lower rates of CRP have used Radiation Therapy Oncology Group (RTOG) Gastrointestinal (GI) toxicity scoring system which does not include the evaluation of the more commonly occurring anorectal symptoms of fecal urgency and incontinence. As these symptoms also have a greater adverse impact on quality of life than rectal bleeding, use of the RTOG GI toxicity system under-estimates radiation morbidity [Citation3,Citation4]. Higher CRP prevalence rates of 50–65% following EBRT for CaP using advanced techniques including IMRT occur when patient-orientated questionnaires are included, such as the Late Effect Normal Tissue-Subjective Objective Management Analytic (LENT-SOMA) and Expanded Prostate Cancer Index Composite Bowel domain (EPICB) bother scales [Citation3–5].
The volume of rectum receiving ≥60 Gy is the only dose volume histogram (DVH) parameter consistently associated with the risk of RTOG Grade ≥2 CRP [Citation6]. Different definitions of the anatomy of the rectum, particularly with respect to its length and the finding that anal DVH parameters correlate better with anorectal symptoms other than rectal bleeding have led to the proposal that anal DVH parameters should be evaluated separately from the rectum [Citation5–7].
Despite wide variations in radiation sensitivity, the same radiation dose is prescribed for similar risk CaP [Citation8]. The Cytokinesis Block Micronucleus Cytome (CBMNCYT) assay, which evaluates spontaneous DNA damage and DNA damage induced following ex vivo irradiation in peripheral blood lymphocytes, is used to measure radiation sensitivity and to study the effects of nutritional factors on DNA damage and repair [Citation9–12]. The micronucleus (MN) index of DNA damage in the CBMNCYT assay following ex vivo irradiation have been reported to predict for RTOG grades 2 and 3 GI and genito-urinary (GU) morbidity approximately three years after EBRT for CaP in a previous study [Citation8]. Dietary micronutrients, such as β-carotene and vitamin E and above average intakes of fruits and vegetables, have been reported to reduce spontaneous DNA damage and may also reduce DNA damage induced by ionizing radiation through their actions as antioxidants or co-factors of DNA repair enzymes [Citation10–12].
However, the previous study of radiation sensitivity based on the CBMNCYT assay [Citation8] did not account for the potential confounding effects of dietary micronutrients and radiation dose volume metrics on chronic GI and GU morbidity.
The aims of this prospective, longitudinal study were to determine which of baseline measurements of: 1) homocysteine and dietary micronutrients including carotenoids, vitamin E and selenium in plasma; 2) indices of chromosome damage/misrepair in the CBMNCYT assay; and 3) anal and rectal radiation dose volume parameters predict GI morbidity after EBRT for CaP.
The significance of this prospective, longitudinal study includes the possibilities of: 1) improved treatment planning approaches for EBRT of CaP; and 2) dietary interventional studies to lessen radiation-induced chronic GI morbidity
Material and methods
The study population was derived among patients referred to the Radiation Oncology Department, Royal Adelaide Hospital for radical EBRT for localized CaP (UICC TNM Stage T1-T3, N0 M0) between 21 July 2004 and 10 May 2007, who met the eligibility criteria of: 1) suitability for EBRT ± hormonal manipulation; and 2) patient consent based on the written protocol approved by the institutional and laboratory Research Ethics Committees.
Risk categorization based on National Comprehensive Cancer Center (NCCN) criteria [Citation13] defined as target(s) of irradiation the prostate only for low-risk CaP, and the prostate and seminal vesicles for intermediate- or high-risk disease using three-dimensional (3D) computed tomography (CT) scans of the pelvis. Patients were provided with written instructions to empty their bowels on the morning of their CT planning pelvic scans and then to start drinking 500 ml of water slowly 1.5 hours before the time of their appointment.
For the intermediate- and high-risk disease patients, two planning target volumes (PTV), PTV 1 and PTV 2 were derived by applying anisotrophic (1.5 cm anteriorly, superiorly and inferiorly, 1.2 cm posteriorly and laterally) margins around the seminal vesicles plus prostate and the prostate only, respectively, using the automatic expansion device of the 3D planning (ADAC Pinnacle) system. For the low-risk disease patients, the same anisotrophic margins were applied around the prostate to derive one PTV only.
3D conformal radiotherapy (CRT) based on multi-leaf collimation of the radiation beams for the irradiated target volumes was used to treat all patients. The aim was to deliver a total radiation dose to the ICRU reference point of the prostate of 74.4 Gy for intermediate- and high-risk disease (1.8 Gy × 28 for PTV1 plus 2 Gy × 12 for PTV2) and 70 Gy (2 Gy × 35) to the prostate only PTV for low-risk disease.
The majority (see Results section for percentage) of patients with high-risk disease, also received hormonal therapy (androgen deprivation therapy in the form of either one of the two luteinizing hormone releasing hormone agonists, goserlin acetate or leuprorelin acetate depot injections) as well as internal and external iliac nodal irradiation.
The dose range to prostate achieved for the whole patient population was 66–74.4 Gy in 33–40 increments over 6.6–7.6 weeks as five patients failed to meet the pre-specified dose constraint of V 70 Gy outer rectal wall (contoured separately from the anal canal as discussed under DVHs of rectal and anal walls below).
Experimental protocol
Before EBRT, 1) plasma concentrations of homocysteine and dietary micronutrients, 2) indices of chromosome damage/DNA misrepair measured by the CBMNCYT assay, and 3) rectal and anal wall dose volume metrics were determined for each patient.
Individual and total GI symptom scores, using a modified version of the LENT-SOMA scales of GI morbidity reported to correlate with a validated health-related quality of life instrument following EBRT for CaP [Citation14], were also evaluated before EBRT, and at one month, one, two and three years after its completion.
Homocysteine and dietary micronutrient measurements
Plasma concentrations of: 1) homocysteine, B12 and folate; 2) zinc and selenium; and 3) carotenoids were determined by: 1) the ARCHITECT folate assay [Citation15]; 2) inductively coupled plasma optical emission spectroscopy (ICPOES) [Citation16]; and 3) High Performance Liquid Chromatography (HPLC) [Citation17], respectively.
Cytokinesis block micronucleus cytome assay
The CBMNCYT assay was used to measure the MN and nucleoplasmic bridge (NPB) frequency in peripheral blood lymphocytes. The MN and NPB biomarkers in the CBMNCYT assay have been used successfully to study radiation sensitivity phenotyping and the effects of micronutrient deficiency on genome integrity. MN and NPB originate from acentric chromosome fragments and dicentric chromosomes, respectively, and thus provide a reliable measure of chromosome DNA damage and DNA misrepair, respectively, in peripheral blood lymphocytes [Citation9].
The CBMNCYT assay was performed using 500 μl of a specimen of fresh whole blood suspended in 4.5 ml of Roswell Memorial Park Institute (RPMI)-1640 culture medium (Thermo Trace, Australia) supplemented with 10% fetal calf serum (FCS) (Thermo Trace, Australia) and phytohemagglutinin at 37°C. Cells were cytokinesis blocked using cytochalasin-B after 44 hours of culture and harvested 28 hours later using a previously published protocol [Citation9].
Spontaneous DNA damage in the CBMNCYT assay was first measured by determining MN and NPB frequency. Radiation-induced DNA damage in the CBMNCYT assay was measured after exposure of the whole blood suspension to 3 Gy γ-rays from a 137Cs source (Cis Bio IBL 437 C Blood Product Irradiator, dose rate 5.34 Gy/min) and then determining MN and NPB frequency.
Dose volume histograms of rectal and anal walls
Derivation of DVH parameters for the rectal and anal walls involved contouring both structures as solid organs in their entire extent. As the rectum normally contains gas and is not solid like the anal canal, it was defined as the ring structure between the outer and inner rectal walls. The outer wall of the rectum from the recto-sigmoid junction above to the anorectal junction below was first contoured. The inner wall of the rectum was then derived by contracting the outer wall of the rectum by 5 mm as previously described [Citation7]. The 3D (ADAC Pinnacle) planning system was finally used to assign a ring structure between the outer and inner wall of the rectum as a region of interest.
Gastrointestinal radiation morbidity measurements
Individual GI symptom scores were based on a five (0–4) point modified LENT-SOMA GI toxicity scale [Citation3]. The total GI symptom scores (0–24) were derived by summation of the individual symptom scores [Citation3].
As it was not possible to determine which individual GI symptom had the most clinical significance in patients after EBRT, the effect of total GI symptoms on activities of daily living (ADL) of the patients after EBRT was also derived. The latter was based on a four (0–3) point categorical scale, higher scores reflecting worsening impact on ADL as in a previous study [Citation3].
Data analysis
The means and medians of plasma homocysteine and micronutrient concentrations were calculated using individual values measured for each patient. The individual values were the calculated means of duplicate measurements of homocysteine and micronutrients for each patient.
MN and NPB frequency was determined on a minimum 1000 binucleated lymphocytes using previously described scoring criteria [Citation9]. Scoring MN frequency in a minimum of 1000 binucleated lymphocytes provides the required statistical power to detect individual differences in radiation sensitivity with high probability and has been widely accepted as the standard CBMNCYT protocol for radiation biology studies [Citation9,Citation18].
The medians of the mean anal (D mean anal) and rectal (D mean rectal) ring doses and volumes of anal wall and rectal ring receiving ≥40 Gy (V 40 Gy anal) and ≥60 Gy (V 60 Gy rectal), respectively, were calculated.
The medians of the individual and total GI symptom scores, using the modified LENT-SOMA toxicity scales before EBRT and at one month, one, two and three years after completion of EBRT, were determined. The median of the effect of total GI symptoms on ADL of scores after EBRT was also calculated.
Statistical analysis
Individual and total LENT-SOMA GI symptoms scores before EBRT and at the pre-determined intervals after its completion were examined by Friedman repeated analysis of variance (ANOVA). χ2-tests were used to compare the percentage of patients with and without increases in individual and total GI symptom scores and the percentage of patients with D mean anal <40 Gy versus D mean anal >40 Gy, V 40 Gy anal ≤65% versus V 40 Gy anal >65% and percentage of patients with V 60 Gy rectal ≤40% versus V 60 Gy rectal >40% [Citation5]. Linear regression examined the relationship between total GI symptom scores and the effect of GI symptoms on ADL scores after EBRT.
A two-sided p-value of ≤0.05 was considered significant in all analyses.
To adjust for correlations between the error terms of the repeated measures and to allow for modeling of both continuous and dichotomized (only one predictor variable, before EBRT total GI symptom score < versus > median value of 1, was dichotomized) variables, generalized estimating equations (GEE) were used to analyze the best joint predictors of the outcome variable of increased total GI symptom scores from one month to three years after EBRT (see below for justification of this as a valid measure of chronic GI morbidity). Due to the large number of predictor variables, the three groups of variables were separately analyzed, the final model combining significant group predictor variables. The groups were: 1) plasma homocysteine and dietary micronutrient levels; 2) spontaneous and radiation-induced MN and NPB indices of the CBMNCYT assay; and 3) D mean anal, D mean rectal, V 40 Gy anal and V 60 Gy rectal (correlations of group predictor variables were tested in the GEE model and no evidence of ill conditioning was found. In particular, most correlations between the radiation dose metrics were of the order of ≤0.2, e.g. r = 0.13 between V 40 Gy anal and mean anal dose suggesting minimal overlap between the dose metrics). In each group, univariate analysis was first used to determine which of the variables were significantly associated (p ≤ 0.10) with increased total GI symptom scores. Multivariate analysis was undertaken by including all the significant predictor variables from the univariate analysis, followed by backwards elimination until all remaining predictor variables within the group were statistically significant (p ≤ 0.05), forward elimination of the variables producing the same results. The final overall model of best fit was then found by combining the statistically significant predictor variables from the three groups of variables, again using backwards elimination, until all the remaining variables were statistically significant (p ≤ 0.05). Notably, all GEE models were adjusted for the baseline (before EBRT) total GI symptom scores for the dependent variable and for time as RT as a nominal variable when univariate analysis was performed in the development of the models. The time variable gives an indication of the pattern of response of total GI symptom scores from one month to three years after EBRT. There appeared to be an increase in GI symptoms at one year and two years compared with one month and total GI symptoms decreased at three years compared with one month, thus justifying the outcome variable of increased total GI symptom scores from one month to three years after EBRT as a valid measure of chronic GI morbidity. It is important to note that the outcome variable of total GI symptom scores after EBRT is an interval (range 0–24) and not a dichotomous variable (only one predictor variable was dichotomized in the development of the multivariate model as specified above).
Stata 13 software package was used for all statistical analyses.
Results
A total of 106 patients, [median age 72 (range 49–84) years, body mass index 27.3 (range 17.7–41.7)] who met the eligibility criteria formed the study population.
Of the 106 patients, 29, 46 and 31 were categorized to have low-, intermediate- and high-risk CaP, respectively, based on National Comprehensive Cancer Center (NCCN) criteria. Twenty-one of the 31 patients with high-risk disease received hormonal therapy (see Methods and Materials for details) as well as EBRT.
The means (± standard error) and medians (range) of the plasma concentrations of homocysteine and dietary micronutrients before EBRT are summarized below and detailed in Supplementary Table I (available online at http://www.informahealthcare.com).
For 1) homocysteine and 2) selenium, the values in μmol/l were 12 ± 1 and 10 (5–84) and 116 (±2) and 115 (72–158), respectively.
For 3) Vitamin B12, the values in ρmol/l were 277 ± 16 and 235 (44–1450).
For 4) folate the values in nmol/l were 18 (±1) and 16 (3–45).
For 5) zinc, 6) lutein, 7) retinol, 8) α-tocopherol, 9) lycopene, 10) α-carotene, 11) β-carotene the values in μg/ml were 5) 0.8 (±0.01) and 0.7 (0.5–1.2), 6) 0.18 (±0.01) and 0.16 (0.03–0.55) 7) 0.66 (±0.02) and 0.63 (0.41–1.08), 8) 13.3 (±0.4) and 12.6 (7.1–37.5), 9) 0.18 (±0.01) and 0.17 (0.01–0.66), 10) 0.05 (±0.00) and 0.03 (0.00–0.27), 11) 0.19 (±0.01) and 0.14 (0.02–0.70), respectively.
The means (±standard error) and medians (range) of the spontaneous and ex vivo irradiation MN and NPB frequencies in the CBMNCYT assay data before EBRT are summarized below and detailed in Supplementary Table II (available online at http://www.informahealthcare.com).
For 1) spontaneous MN frequencies, and 2) ex vivo irradiation MN frequencies (minus spontaneous frequencies), the values in MN/1000 binucleated cells are 1) 12 (±0.6) and 10 (2–44) and 2) 620 (±12) and 619 (233–1037), respectively.
For 3) spontaneous NPB frequencies, and 4) ex vivo irradiation NPB frequencies (minus spontaneous frequencies), the values in NPB/1000 binucleated cells are 3) 4 (±0.3) and 4 (0–12) and 4) 146 (±4) and 143 (0.2–252), respectively.
The medians (range) of the baseline (before EBRT) planning volume, D mean, V ≥40 Gy, V ≥60 Gy of the outer rectal wall, rectal ring and anal wall are summarized below and detailed in Supplementary Table III (available online at http://www.informahealthcare.com).
For the planning volume, the values in cm2 are 62 (19–252) for outer rectal wall, 30 (14–60) for rectal ring and 38 (12–64) for anal wall.
For D mean, the values in Gy are 51 (31–65) for outer rectal wall, 51 (34–64) for rectal ring and 43 (15–62) for anal wall.
For V ≥40 Gy, the values in percent are 64 (26–100) for outer rectal wall, 60 (9–100) for rectal ring and 46 (3–94) for anal wall.
For V ≥60 Gy, the values in percent are 40 (14–81) for outer rectal wall, 41 (17–74) for rectal ring and 26 (1–63) for anal wall.
Total GI symptom scores persisting above baseline values at all the pre-determined times after EBRT resulted from increased stool frequency, urgency of defecation, rectal mucous discharge and rectal bleeding scores ().
Table I. Modified LENT-SOMA gastrointestinal (GI) symptom scores before EBRT and at 1 month, 1 year, 2 years and 3 years after completion.
At three years, scores of: 1) stool frequency; 2) diarrhea; 3) rectal pain; 4) mucous discharge (anal incontinence); 5) urgency of defecation; 6) rectal bleeding; and 7) total GI symptoms were persistently increased above baseline in: 1) 23%; 2) 20%; 3) 22%; 4) 26%; 5) 40%; 6) 41%; and 7) 72% of the patients, respectively. Whilst χ2 comparisons of percentage of patients with and without increases of individual symptom scores, such as mucous discharge (anal incontinence) and rectal bleeding at three years and the measured dose volume parameters at baseline (before EBRT, dichotomized as detailed in statistical analysis), χ2-tests percentage of patients with and without increases in total GI symptom scores at three years were significant for D mean anal <40 Gy versus D mean anal >40 Gy and for V 40 Gy anal ≤65% versus V 40 Gy anal >65% (p < 0.05 for both, data not shown).
Total GI symptom scores and the effect of GI symptoms on ADL were directly related at each time point after EBRT (r = 0.32–0.70, p < 0.001–0.0001, for three-year data).
Figure 1. Relationship between total GI symptom scores and scores of activities of daily living (ADL) in patients 3 years after radiation therapy. Data based on observations in 84 patients, the numbers in parentheses representing ties in the data points.
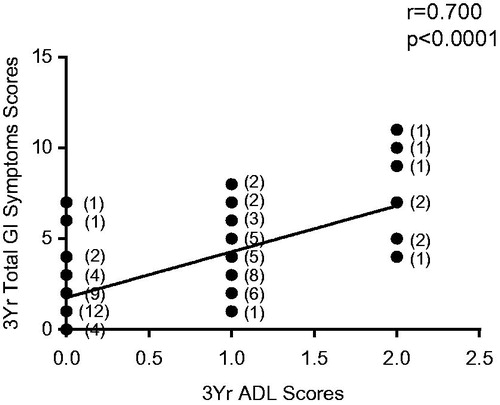
Univariate analysis
Direct associations of significance (defined as p ≤ 0.10 for univariate analysis as detailed in statistical analysis section) existed between the predictor variables of D mean anal, D mean rectal, V 40 Gy anal, V 60 Gy rectal, spontaneous and ex vivo irradiation MN frequency, spontaneous and ex vivo irradiation NPB frequency, plasma levels of homocysteine, Vitamin B12, folate, zinc, selenium, lutein, retinol, α tocopherol, lycopene, α-carotene, β-carotene, and the outcome variable of increased GI symptoms from one month to three years after EBRT (p = 0.96–<0.001, ).
Table II. Univariate and multivariate predictor variables of increased total GI symptom score from 1 month to 3 years after EBRT.
Multivariate analysis
On multivariate analysis, only V 40 Gy anal predicted for increased GI symptom scores following EBRT (p < 0.001, ) after, adjusting for before EBRT total GI symptom score and time since EBRT as a nominal variable.
Discussion
This prospective longitudinal study is the first to examine a range of nutritional and DNA damage biomarkers together with several anal and rectal DVH parameters to determine which predictor variable(s) is of independent prognostic significance for chronic GI morbidity after EBRT for CaP.
Previous studies have examined anal and/or rectal DVH parameters and patient-risk factors including radiosensitive phenotype which may be predictive of GI morbidity after EBRT for CaP in isolation [Citation5–8]. With the possible exception of the consistent association of V ≥60 Gy rectum and RTOG Grade ≥2 chronic radiation proctitis, there is no conclusive anal radiation dose metric to provide a definite basis for radiation treatment planning.
Although reports that dietary micronutrients and above average intakes of fruits and vegetables may reduce DNA damage induced by ionizing radiation through their actions as antioxidants or co-factors of DNA repair enzymes [Citation10–12], there have hitherto been no studies of their impact on chronic radiation-induced GI morbidity. The likely explanation for the absence of such studies is the theoretical concern among radiation oncologists that antioxidants may diminish the therapeutic efficacy of RT although a recent study reported that supplements of β-carotene did not to result in worse outcomes after EBRT for CaP compared with placebo [Citation19].
In the present study, plasma selenium and α-tocopherol levels were associated with chronically increased GI symptoms after EBRT on univariate but not multivariate analysis. The wide range of concentrations of homocysteine, vitamin B12, folate, selenium and carotenoids, such as lycopene, found in the plasma of patients in our study indicate large variation in intake and possible B vitamin deficiency.
The CBMNCYT biomarker data also show a wide range in spontaneous and radiation-induced MN and NPB frequency indicating substantial variation in genome integrity and radiation sensitivity.
Our finding of the association of spontaneous (0 Gy) MN with worsening GI symptoms on univariate analysis differs from the report that radiation-induced (3 Gy ex vivo) but not spontaneous MN predicted increased chronic GI and GU morbidity after EBRT in a previous study of radiation sensitivity based on the CBMNCYT assay [Citation8]. A likely explanation for the discordance in findings is that the confounding effects of nutritional factors on the expression of the radiosensitive phenotype were not examined in the previous study [Citation8]. For example, it is likely that the association of GI symptoms with spontaneous MN frequency in our study may reflect carotenoid and α-tocopherol intake as well as zinc, selenium, folate and vitamin B12 status as lower levels of DNA damage in lymphocytes have been reported with increased intake of retinol, β-carotene, α-tocopherol, folate and higher plasma carotenoid, homocysteine and lower zinc, selenium, folate and vitamin B12 concentrations [Citation20–22].
We acknowledge that the in vitro radiation challenge model used in the CBMNCYT assay in not replicating the in vivo conditions, particularly with respect to tissue type and dose fractionation clinically constitutes one of the limitations of the study. Three Gy γ-rays was used to test variation in ex vivo radiation sensitivity in this study because MN frequency after ex vivo radiation doses of between 2 Gy and 4 Gy γ-rays, in particular, were reported to be significantly different between patients with normal and abnormal radiosensitivity (measured as RTOG grades 0 or 1 versus RTOG grades 2 or 3 chronic GI and GU morbidity) in a previous study [Citation8]. Although the dose rate of γ-rays used in this study is greater than the 0.9 Gy/min in the previous study [Citation8], it is outside of the well recognized steep dose effect range of (0.01 and 1 Gy/min) for cell survival data. Furthermore, the 5.43 Gy/min in this study more closely approximates the dose rate of the linear accelerators used in EBRT clinically. The use of peripheral blood lymphocytes is thus a practical surrogate model assuming that normal tissue sensitivity is dictated by intrinsic genetic defects in DNA strand break repair that would be evident across tissues. The peripheral blood lymphocyte model could theoretically be improved to test effects of radiation under the same in vivo conditions which apply in EBRT of patients. However, with respect to dose fractionation, it would only be practical to use just two dose fractions which should be enough to test adaptive capacity of cells to an initial radiation dose and also possibly to reveal another aspect of the phenotype of resistance or sensitivity to radiation lethality.
Unlike the previous study of radiation sensitivity based on the CBMNCYT assay [Citation8], our study also examined the influence of DVH parameters on radiation-induced GI morbidity. Although the range of volumes of outer rectal and anal wall of 19–252 cm3 and 12–64 cm3 in our study are at variance with the 37–193 cm3 and 7–22 cm3, respectively, in another study [Citation23], the apparent discrepancies can be attributed to different definitions of the anal canal. In our study, the 3–4 cm length of the anal canal is defined from the anorectal junction to the anal verge instead of the standard 2 cm length from the anal verge definition previously reported [Citation23]. D mean for outer rectal (31–65 Gy) and anal wall (15–62 Gy) in our study closely corresponds with the calculated 32–64 Gy and 10–62 Gy parameters in yet another study of the influence of radiation dose metrics on GI morbidity [Citation24].
The association between all anal and rectal DVH parameters examined and increased total GI symptom scores on univariate analysis in this study is consistent with the data from previous studies [Citation5–7,Citation25]. However, the finding that only V 40 Gy anal independently predicted for increased GI symptoms from one month to three years after EBRT in our study differs from a previous report that D mean anal >40 Gy increased the risk of fecal incontinence [Citation5]. There are a number of possible explanations for the discordant findings between our study and that of the previous study [Citation5]. These include differences in the design, definition and analysis of the outcome variables between the previous study [Citation5] and our study. Whilst Alsadius et al. [Citation5] examined the relationship between the prevalence of fecal incontinence at a varying point of 2–15 years after completion of primary or post-operative EBRT for CaP and mean anal dose determined retrospectively from the treatment plans of the patients, the findings in our prospective longitudinal study were based on univariate and multivariate analyses of variables which predict for increased total GI symptom scores in patients from one month and three years after EBRT for CaP. Of these differences, a plausible explanation for the discordant findings in radiation dose metrics between the previous study [Citation5] and our study lies in the outcome measures of chronic GI morbidity in the two studies. Increased total GI symptoms in our study includes anorectal symptoms other than fecal incontinence and the finding that a 1% increase in volume of the anal wall receiving ≥40 Gy results in 0.32 rise in total GI symptom score from one month to three years after EBRT in our multivariate model suggests the predominance of a volume effect. In contrast, the large increase in the prevalence of fecal incontinence between patients receiving D mean dose ≥40 Gy versus <40 Gy suggests a greater dose effect in the previous study by Alsadius et al. [Citation5].
A third smaller study of radiation dose metrics only and GI morbidity based on 65 patients who had completed EBRT for localized CaP 2–4 years earlier found correlations between the 25–42 Gy dose interval for rectum and defecation urgency/diarrhea and 45–55 Gy interval for anal sphincter region and fecal leakage but was inconclusive in defining threshold volumes and doses for increased GI morbidity after EBRT for CaP [Citation25]. Whilst the greater sample size of 106 in this study is still modest, the multivariate analysis is based on 424 observations, albeit not independent ones. However, our modeling strategy was carefully designed to avoid over-fitting and all models were adjusted for baseline total GI symptom score and time since EBRT as a nominal variable.
The relationships between dose metrics and chronic GI morbidity in this study like others are based on the assumption that the dose structures defined on the basis of a single planning scan applies throughout the 7–7.4 week’s course of radiation treatment. The finding that V 40 Gy anal independently predicted for increased GI symptoms from one month to three years after EBRT in our study is therefore not conclusive.
However, the data in our study supports: 1) previous recommendations that the DVHs of the anal wall should be derived separately from the rectal wall; and 2) minimizing the volume and dose received by the rectum and anal canal, in particular V ≥40 Gy anal wall and D mean anal <40 Gy in order to reduce all GI symptoms which contribute to chronic GI morbidity after EBRT for CaP.
ACTA_Oncologica_Manuscript_Supplementary_Material.doc
Download MS Word (42.5 KB)Acknowledgments
The authors gratefully acknowledged research support from the Royal Adelaide Hospital Special Purposes Fund (EY) and CRC for Diagnostics (MF). The study has been presented in part at Digestive Disease Week, Los Angeles 2006, Washington DC 2007 and San Diego 2008 and at the Neuro-gastroenterology and Motility Joint International Meeting, Chicago 2009 and Boston 2010.
Declaration of interest
The authors report no conflicts of interest. The authors alone are responsible for the content and writing of the paper.
References
- Jani AB, Hellman S. Early prostate cancer: clinical decision-making. Lancet 2003;361:1045–53.
- Smeenk RJ, Teh BS, Butler EB, Vanlin EN, Kaanders JH. Is there a role for endorectal balloons in prostate radiotherapy? A systematic review. Radiother Oncol 2010;95:277–82.
- Yeoh EK, Holloway RJ, Fraser RJ, Botten R, Di Matteo AC, Butters JF, et al. Hypofractionated versus conventionally fractionated radiation therapy for prostate carcinoma; updated results of a Phase III randomised trial. Int J Radiat Oncol Biol Phys 2006;66:1072–83.
- Krol R, Smeenk RJ, van Lin EN, Hopman WP. Impact of late anorectal dysfunction on quality of life after pelvic radiotherapy. Int J Colorectal Dis 2013;28:519–26.
- Alsadius D, Hedelin M, Lundstedt D, Pettersson N, Wilderang U, Steineck G. Mean absorbed dose to the anal-sphincter region and fecal leakage among irradiated prostate cancer survivors. Int J Radiat Oncol Biol Phys 2012;84:e181–85.
- Michalski JM, Gay HG, Jackson A, Tucker SL, Deasy QL. Radiation dose-volume effects in radiation-induced rectal injury. Int J Radiat Oncol Biol Phys 2010;76:S123–29.
- Smeenk RJ, van Lin EN, van Kollenberg P, Kunze-Busch M, Kaanders JH. Anal wall sparing effect of an endorectal balloon in 3D conformal and intensity-modulated prostate radiotherapy. Radiother Oncol 2009;93:131–6.
- Lee TK, Allison RR, O’brien KF, Johnke RM, Christie KI, Naves JL, et al. Lymphocyte radiosensitivity correlated with pelvic radiotherapy morbidity. Int J Radiat Oncol Biol Phys 2003;57:222–9.
- Fenech M. Cytokinesis-block micronucleus cytome assay. Nat Protocol 2007;2:1084–104.
- Umegaki K, Ikegami S, Inoue IK, Ichikawa T, Kobayashi S, Soeno N, et al. Beta-carotene prevents x-ray induction of micronuclei in human lymphocytes. Am J Clin Nutr 1994;59:409–12.
- Smith MJ, Inserra PF, Watson RR, Wise JA, O’neill KL. Supplementation with fruit and vegetable extracts may decrease DNA damage in the peripheral lymphocytes of an elderly population. Nutr Res 1999;19:1507–18.
- Greenrod W, Stockley CS, Burcham P, Abbey M, Fenech M. Moderate acute intake of de-alcoholized red wine, but not alcohol, is protective against radiation-induced DNA damage ex vivo – results of a comparative in vivo intervention study in younger men. Mutat. Res 2005;591:290–301.
- Lee I, Sandler H. Hormone therapy and radiotherapy for intermediate risk prostate cancer. Semin Radiat Oncol 2008;18:7–14.
- Livsey J, Routledge J, Burns M, Swindell R, Davidson SE, Cowan RA, et al. Scoring of treatment-related late effects in prostate cancer. Radiother Oncol 2002;65:109–21.
- Hubl W, Zogbaum M, Boyd JC, Savory J, Schubert M, Meyer D, et al. Evaluation of analytical methods and workflow performance of the Architect ci8200 integrated serum/plasma analyzer system. Clin Chim Acta 2005;357:43–54.
- Bazzi A, Nriagu JO, Linder AM. Determination of toxic and essential elements in children's blood with inductively coupled plasma-mass spectrometry. J Environ Monit 2008;10:1226–32.
- Noakes M, Clifton P, Ntanios F, Sharpnel W, Record S, Mcinerney J. An increase in dietary carotenoids when consuming plant sterols or stanols is effective in maintaining plasma carotenoid concentrations. Am J Clin Nutr 2002;75:79–86.
- Fenech M. The lymphocyte cytokinesis-block micronucleus cytome assay and its application in radiation biodosimetry. Health Phys 2010;98:234–43.
- Margalit DN, Kasperzyk JL, Martin NE, Sesso HD, Gaziano JM, Stampfer MJ, et al. Beta-carotene antioxidant use during radiation therapy and prostate cancer outcome in the Physicians' Health Study. Int J Radiat Oncol Biol Phys 2012;83:28–32.
- Fenech MF, Dreosti IE, Rinald JR. Folate, Vitamin B12, homocysteine status and chromosome damage rate in lymphocytes of older men. Carcinogenesis 1997;18:1329–36.
- Thomas P, Wu J, Dhillon V, Fenech M. Effect of dietary intervention on human micronucleus frequency in lymphocytes and buccal cells. Mutagenesis 2011;26:69–76.
- Fenech M, Baghurst P, Luderer W, Turner J, Record S, Ceppi M, et al. Low intake of calcium, folate, nicotinic acid, vitamin E, retinol, beta-carotene and high intake of pantothenic acid, biotin and riboflavin are significantly associated with increased genome instability-results from a dietary intake and micronucleus index survey in South Australia. Carcinogenesis 2005;26:991–9.
- Fokdal L, Honore H, Hoyer M, von der Masse H. Dose-volume histograms associated to long-term colorectal functions in patients receiving pelvic radiotherapy. Radiother Oncol 2005;74:203–10.
- Peeters STH, Lebesque JV, Heemsbergen WD, van Putten WLJ, Slot A, Dielwart MFH, et al. Localized volume effects for late rectal and anal toxicity after radiotherapy for prostate cancer. Int J Radiat Oncol Biol Phys 2006;64:1151–61.
- Al-Abany M, Helgason AR, Cronqvist AK, Lind B, Mavroidis P, Wersall P, et al. Toward a definition of a threshold for harmless doses to the anal sphincter region and the rectum. Int J Radiat Oncol Biol Phys 2005;61:1035–44.