Abstract
Background: To clarify if early reduction in standard uptake value (SUV) could predict metabolic response, radiologic response and overall survival (OS) in patients with metastatic colorectal cancer receiving third-line treatment.
Material and methods: Patients were regardless of KRAS status, included in this phase II trial. They were treated with the monoclonal antibody, cetuximab, and the chemotherapeutic drug, irinotecan, every second week. A F18-fluorodeoxy glucose positron emission tomography/computed tomography (FDG-PET/CT) was scheduled before the first and second treatment, respectively, and then after every fourth treatment. Early metabolic response after one treatment and best overall metabolic response was calculated according to EORTC criteria (responders: ≥15% decrease in ∑SUVmax) and PERCIST (responders: ≥30% decrease in SULpeak). Best overall radiologic response was calculated according to RECIST 1.0.
Results: By EORTC criteria, early metabolic response predicted partial metabolic response (PMR) with a high positive predictive value (PPV) of 0.875 and a high negative predictive value (NPV) of 0.714. Partial radiologic response was predicted with a low PPV of 0.368 but a high NPV of 1.0. By PERCIST, PMR was predicted with a high PPV of 0.826 and an intermediate NPV of 0.667 and partial radiologic response was predicted with a low PPV of 0.5 but a high NPV of 1.0. Median OS was nearly the same with the two criteria sets; 14.1 months for early metabolic responders and 9.9 months for non-responders using EORTC criteria and 13.5 and 10.1 months, respectively, using PERCIST.
Conclusions: With both EORTC criteria and PERCIST, early reduction in FDG uptake was predictive of a later partial metabolic and partial radiologic response to treatment. It was also predictive of significantly longer survival of early metabolic responders compared to non-responders. However, the sensitivities and specificities were not high enough to support clinical routine use.
The ability to predict whether a treatment will be beneficial to a patient is attracting growing interest for two main reasons: first, the ability to spare patients adverse effects from ineffective treatment and second, to spend the limited funds available for increasingly expensive cancer treatments most rationally.
F18-fluorodeoxy glucose positron emission tomography/computed tomography (FDG-PET/CT) has the ability to detect tumor changes induced by chemotherapy and targeted therapy earlier than CT [Citation1–5]. Perhaps FDG-PET/CT also has the ability to visualize the changes that targeted treatment induces in tumors more convincing than CT does [Citation3,Citation6,Citation7]. FDG-PET/CT could therefore theoretically be useful in predicting treatment response to chemotherapy, targeted treatment or a combination of the two. So far, most studies have investigated the utility of treatment response prediction with FDG-PET/CT in the preoperative setting, with chemo- or chemoradiation therapy being predominant. The utility has not been investigated in the metastatic setting to the same extent [Citation1]. A possible explanation for this could be the more unambiguous and dichotomous responses resulting from preoperative treatment: either a presence or an absence of residual tumor masses. The findings have been promising for the use of an initial drop in tumor FDG uptake as a predictive marker for response, and in some cases also as a predictive marker for survival [Citation1,Citation5,Citation8], however, not to a degree calling for use in routine clinical practice. Also, the cutoff values, separating responders from non-responders, vary between studies, challenging comparability of results [Citation5,Citation7,Citation9,Citation10].
In the present study, we investigated the value of early metabolic response, estimated using an FDG-PET/CT scan after one treatment, as a predictive marker for best overall metabolic and best overall radiologic response (BOMR and BOR), and of overall survival (OS) in patients with metastatic colorectal cancer (mCRC). The patients were treated with a combination of the monoclonal antibody, cetuximab, and the chemotherapeutic drug, irinotecan. To estimate early metabolic response we applied two sets of internationally recognized FDG-PET/CT-based response assessment methods: the EORTC criteria [Citation11] and PERCIST [Citation12]. To estimate BOMR we also applied EORTC criteria and PERCIST. To estimate BOR we applied RECIST 1.0 [Citation13].
Material and methods
Patients
From November 2006 to October 2008, patients with mCRC being treated at Copenhagen University Hospital Herlev (Herlev) and University Hospital Odense (Odense) were offered inclusion in a phase II trial prospectively and regardless of KRAS status. They were treated with a combination of a monoclonal antibody; cetuximab (Erbitux®, Merck, 500 mg/m2), and a chemotherapeutic drug; irinotecan (Irinotecan, Fresenius Kabi Oncology, 180 mg/m2), every second week as third-line palliative treatment.
All patients were FDG-PET/CT scanned before the first treatment (baseline) and just before the second treatment (early scan). Hereafter, the patients at Herlev continued to be FDG-PET/CT scanned after every fourth treatment while the patients at Odense were CT scanned with the same frequency. Treatment was continued until progression was recognized on CT according to RECIST 1.0. If the criteria for progression according to RECIST 1.0 had been met, the treatment was terminated. This also applied if the criteria for progression according to EORTC criteria and/or PERCIST had not been met at the same point in time. To avoid therapy-induced stunning the FDG-PET/CT scans were scheduled to be performed between 10 and 14 days after last treatment [Citation12,Citation14].
As the patients included at Odense were FDG-PET/CT scanned only at baseline and after the first treatment, they were not included in the analyses of prediction of BOMR, but were included in the analyses of prediction of BOR and in the OS analyses.
The protocol was approved by the Danish Regional Research Ethics Committee, the Danish Medicines Agency and the Data Protection Agency (EudraCT no. 2006-001961-40). Oral and written informed consent was obtained from all patients before inclusion in the trial.
FDG-PET/CT scans
All patients were scanned from the base of the skull to mid-thigh. At Herlev, the patients were scanned on two different scanners: Philips Gemini Dual Slice PET/CT (GeminiDS) or Philips Gemini TruFlight™ (TF) 16 slice PET/CT (GeminiTF) (Philips Medical Systems, Cleveland, Ohio, USA). At Odense, the patients were scanned on a General Electric (GE) Discovery STE 16 slice PET/CT scanner (GE Healthcare, Milwaukee, Wisconsin, USA). All scans performed at Odense were sent to Herlev. All scans were examined with Philips Extended Brilliance Workspace Nuclear Medicine software version 2.0, Tumor Tracking by the same observer, drawing all regions of interest (ROIs) [Citation12].
Both centers used 18F-FDG as tracer. It was injected intravenously with a median activity of 372 ± 25 MBq and the median uptake time from tracer injection to onset of PET imaging was 65 ± 11 minutes. Patients fasted for at least five hours before imaging but were allowed to drink water. At Herlev, blood glucose (bg) level was measured immediately before scan start and all patients with bg levels ≥8 mmol/l (144 mg/dl) were excluded. At Odense, blood glucose levels were not measured. Patients with NIDDM abstained from oral anti-diabetic medicine for a minimum of 24 hours before scanning, whereas patients with IDDM had breakfast and insulin followed by a four-hour fast before scanning.
The spiral, multi-detector (two or 16 slices pr. rotation) CT scans were standard diagnostic contrast enhanced computed tomography (ceCT) examinations with oral and intravenous contrast, covering the thorax, abdomen and pelvis. At Herlev, iodinated contrast (Omnipaque 350 GE Healthcare, Oslo, Norway or Optiray 320 Covidien, Neustadt/Donau, Germany or Iomeron 350 Bracco, Milan, Italy) was administered both orally: 20 ml in 500 ml bottled water (4% solution) half an hour before scanning, and intravenously: 100 ml with an injection flow of 5 ml/s immediately before scanning. At Odense, iodinated contrast (E-Z-CAT 720 suspension bariumsulfate, E-Z-EM, USA) was administered orally: 225 ml dissolved in 900 ml bottled water half an hour before scanning, and iopromid was administered intravenously (Ultravist 370 mg/ml, Bayer Schering Pharma, Germany): 0.8 ml/kg with an injection flow of 2.5 ml/s immediately before scanning.
Response evaluation
The treatment-induced metabolic tumor changes, both early metabolic response and BOMR, were quantified according to both EORTC criteria [Citation11] and PERCIST [Citation12]. Treatment-induced radiologic tumor changes; BOR, were quantified according to RECIST 1.0 [Citation13].
FDG-PET/CT response evaluation, EORTC criteria
Software normalization with body surface area (bsa) was applied. The EORTC criteria hold no definition of minimum uptake value of a target lesion or instructions on number of target lesions to measure. Hence, we selected up to seven target lesions at baseline and measured the same lesions on the early scan and on every subsequent follow-up scan. Whether to measure SUVmax or mean is optional. We registered SUVmax in each target lesion. As recommended, lesions with the highest 18F-FDG uptake, representing viable tumor tissue, were chosen as target lesions. The target lesions represented as many involved organ systems as possible. SUVmax from all target lesions were summed on each scan, giving ∑SUVmax. The change in ∑SUVmax between baseline and the early scan was calculated. The EORTC criteria define partial metabolic response (PMR) after one cycle of chemotherapy as a drop in SUV of 15–25%. We therefore applied the cutoff value of ≥15% to the early scan to establish early metabolic response.
To register the patients’ BOMR during treatment, the chosen baseline lesions were measured on all follow-up scans. Response was calculated according to EORTC criteria (BOMR-EORTC):
Complete metabolic response (CMR): complete resolution of 18F-FDG uptake within all lesions making them indistinguishable from the surrounding tissue. PMR: reduction of ∑SUVmax of ≥25% after more than one treatment cycle. Progressive metabolic disease (PMD): increase of ≥25% in ∑SUVmax or a new 18F-FDG avid lesion. Stable metabolic disease (SMD): in between PMR and PMD [Citation11].
The two early metabolic response groups – responders (≥15% drop in ∑SUVmax after one treatment) and non-responders (<15% drop in ∑SUVmax after one treatment) – were then correlated with BOMR-EORTC.
FDG-PET/CT response evaluation, PERCIST
Software normalization of SUV with lean body mass (lbm) was applied. Background areas were drawn in the liver. If the disease had spread to the liver, it was drawn in the descending thoracic aorta, as defined in PERCIST. With the available software, it was not possible to extend the aorta ROI from 1 to 2 cm in the z-axis and it was therefore drawn as a spherical 1-cm diameter ROI. The background cutoff values were calculated as: 1.5 × liver ROI SULmean + [2 × standard deviation of liver ROI] or as: 2.0 × aorta ROI SULmean, as outlined in PERCIST.
The lesion with highest FDG uptake was located and a 1.2-cm diameter spherical ROI was drawn in the part of the lesion showing the highest FDG uptake. The ROI was placed to include the “hottest” pixel and at the same time give the highest SULmean value. SULmean of this ROI was = SULpeak. On each scan, the lesion with the highest FDG uptake was chosen for measurement, even though this was a different lesion than the one measured at baseline, as long as the lesion had been present at baseline. The changes in FDG uptake during treatment were then calculated. If a patient’s baseline SULpeak did not exceed the background value, the patient was not eligible for response evaluation as defined in PERCIST.
We applied PERCIST to the baseline scan, the early scan and all subsequent follow-up FDG-PET/CT scans. This gave us early metabolic response and BOMR according to PERCIST (BOMR-PERCIST). PERCIST holds no early response definition. PERCIST response definitions are:
CMR: complete resolution of 18F-FDG uptake within all lesions to ≤ mean liver activity and indistinguishable from background blood-pool levels. PMR: reduction of ≥30% in SULpeak and an absolute drop of 0.8 SULpeak units as well. PMD: increase of ≥30% in SULpeak and an absolute increase of 0.8 SULpeak units as well, or a new 18F-FDG avid lesion. SMD: in between PMR and PMD.
The two early metabolic response groups, responders (≥30% drop in SUVpeak after one treatment) and non-responders (<30% drop in SUVpeak after one treatment) were then correlated with BOMR-PERCIST.
CT response evaluation, RECIST 1.0
RECIST 1.0 was applied to the baseline scan and all subsequent scans, except for the early scan (just before second treatment). Target lesions, up to five per organ and 10 in total, were chosen on the baseline scan, their longest diameter measured and summed. On each subsequent scan the target lesions were again measured and summed. Non-target lesions were registered at baseline and described on each subsequent scan.
RECIST 1.0 response definitions are:
Complete response (CR): disappearance of all target and non-target lesions. Partial response (PR): reduction of ≥30% in sum of largest diameter of target lesions taking as reference the baseline sum longest diameter. Progressive disease (PD): increase of ≥20% in sum of largest diameter of target lesions taking as reference the smallest sum longest diameter recorded since treatment start or appearance of new measurable or non-measurable lesions or unequivocal progression of existing non-target lesions. SD: in between PR and PD. BOR achieved by each patient during treatment was registered.
KRAS analysis
Patients were included in the trial regardless of their KRAS status. After protocol closure, all formalin-fixed paraffin-embedded tumor tissue samples from archival specimens, collected at the time of diagnosis, were analyzed for KRAS mutations in codon 12 and 13 using TheraScreen® KRAS mutation kit (DxS Ltd, Manchester, UK) identifying seven somatic KRAS mutations. The patients were classified as KRAS mutated (mut) if one of the seven mutations were present and as KRAS wild-type (wt) if none of the seven mutations were present in their tumor tissue sample [Citation15,Citation16].
Statistical analysis
To test whether there was an association between an initial drop in tumor metabolism and BOMR/BOR, Fisher’s exact test was used. For the association between early changes in FDG uptake and PMR/PR, sensitivity, specificity, positive predictive value (PPV) and negative predictive value (NPV) were calculated for BOMR-EORTC, BOMR-PERCIST and BOR-RECIST 1.0. ROC curves were performed accordingly. Kaplan-Meier method was used for drawing survival curves with log-rank test for p-value calculation. For the association between early changes in FDG uptake and OS, hazard ratio (HR) was calculated and tested for influence by performance status (PS), KRAS status and carcinoembryonic antigen (CEA) at baseline by multivariable Cox regression analysis. Prior to the multivariable analysis, univariate analyses with 10 relevant variables (gender, age, PS, number of metastatic sites, first-line treatment, second-line treatment, time to protocol treatment, KRAS status, BRAF status and CEA at baseline) were performed. p-Values below 0.05 were considered significant and all p-values were two-sided. All statistical analyses were performed with the statistical program R version 3.2.0 (R Foundation for Statistical Computing, Vienna, Austria). OS was defined as the time from trial registration of a patient until death of any course. By January first 2015 all patients had died.
Results
Eligible patients
At Herlev, 150 patients were included. At Odense, 27 patients were included (). For different reasons, presented in , 111 patients were excluded leaving 66 patients eligible for response evaluation and correlation of BOMR/BOR with OS.
For the correlation between early changes in FDG uptake and BOMR, the 53 patients included at Herlev were eligible, as they had been FDG-PET/CT scanned throughout the course of their treatment. For the correlation between early changes in FDG uptake and BOR as well as OS, all 66 patients from both inclusion sites were eligible. Patient characteristics are presented in .
Table 1. Patient characteristics.
Prediction of partial metabolic and partial radiologic response as best overall metabolic and best overall radiologic response
With both EORTC criteria and PERCIST, patients with the defined reductions in SUV at their early FDG-PET/CT scan had a significantly different BOMR distribution with higher PMR rate, lower SMD rate and no patients with PMD (EORTC: p = 0.0001, PERCIST: p = 0.0006) compared to the patients without the defined reductions (). Distribution according to BOR was also significantly different between early metabolic responders and non-responders (EORTC: p < 0.0001, PERCIST: p < 0.0001) as shown in .
Figure 2. Distribution of best overall metabolic response (BOMR) in patients with early metabolic response and without according to EORTC criteria and PERCIST. EMRs: early metabolic responders; non-EMRs: early metabolic non-responders; PMD: progressive metabolic disease; PMR: partial metabolic response; SMD: stable metabolic disease.
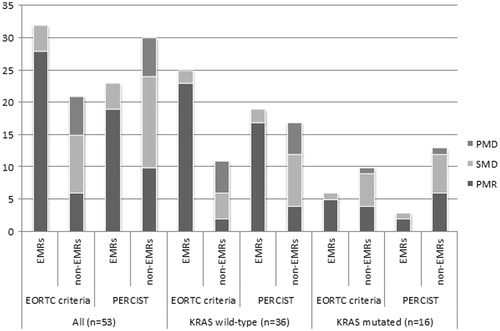
Figure 3. Distribution of best overall radiologic response (BOR) in patients with early metabolic response and without according to EORTC criteria and PERCIST. EMRs: early metabolic responders; non-EMRs: early metabolic non-responders; PD: progressive disease; PR: partial response; SD: stable disease. Two patients were KRAS indefinable.
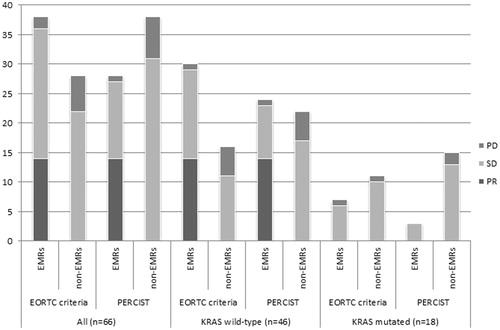
With EORTC criteria, the sensitivity of prediction of PMR was 0.824, the specificity was 0.789, the PPV was 0.875 and the NPV was 0.714, all high values ( and ). The sensitivity to predict PR was high; 1.0, the specificity was low; 0.538, the PPV was also low; 0.368 and the NPV high; 1.0 ( and ).
Figure 4. ROC curves for prediction of partial metabolic response as best overall metabolic response (BOMR) with EORTC criteria and PERCIST and for prediction of partial response as best overall radiological response with RECIST 1.0. BOMR: best overall metabolic response; BOR: best overall radiological response; PMR: partial metabolic response; PR: partial response.
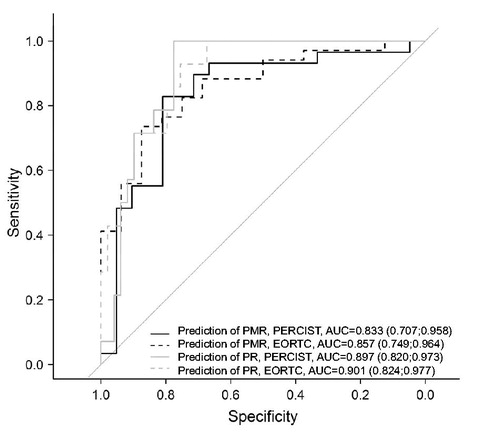
Table 2. The ability of an early metabolic response, defined as an initial drop in SUV according to EORTC criteria and PERCIST, to predict partial metabolic response as best overall metabolic response (BOMR).
Table 3. The ability of an early metabolic response, defined as an initial drop in SUV according to EORTC criteria and PERCIST, to predict partial response as best overall radiological response (BOR).
With PERCIST, the sensitivity to predict PMR was intermediate; 0.655, the specificity was high; 0.833, the PPV was also high; 0.826 and the NPV intermediate; 0.667 ( and ). The sensitivity and specificity to predict PR were both high; 1.0 and 0.731, respectively, the PPV was low; 0.5 and the NPV was also high; 1.0 ( and ).
In the subgroup of the KRAS wt patients, results were similar to those for the whole patient group described above. Please see .
In the subgroup of patients with KRAS mutations, the same analyses gave outcomes different from the whole patient group and the group of KRAS wt patients. The BOMR distributions between early metabolic responders and non-responders differed insignificantly by both EORTC criteria and PERCIST (p = 0.4 and p = 1.0, respectively, ) and by RECIST 1.0 (EORTC: p = 1.0 and PERCIST: p = 1.0, ). The sensitivity, specificity, PPV and NPV are presented in . One patient’s KRAS status was indefinable.
Prediction of overall survival
In the univariate analyses only PS was significant (p = 0.002). KRAS status and CEA at baseline both had a p-value of 0.07. Gender, age, number of metastatic sites, first-line treatment, second-line treatment, time to protocol treatment and BRAF status all came out non-significant with p-values higher than 0.2.
With EORTC criteria, early metabolic non-responders had a relative hazard of dying that was 1.8 times higher [95% confidence interval (CI) 1.1–3.0] than early metabolic responders. The median survival of early metabolic responders versus non-responders was 14.1 months (95% CI 10.4–20.8) and 9.9 months (95% CI 7.0–13.0), respectively. This difference was significant (p = 0.02) ().
Figure 5. Overall survival in the group of early metabolic responders and in the group of early metabolic non-responders according to both EORTC criteria and PERCIST. CI: 95% confidence interval; EMRs: early metabolic responders; mo: months; non-EMRs: early metabolic non-responders; OS: overall survival.
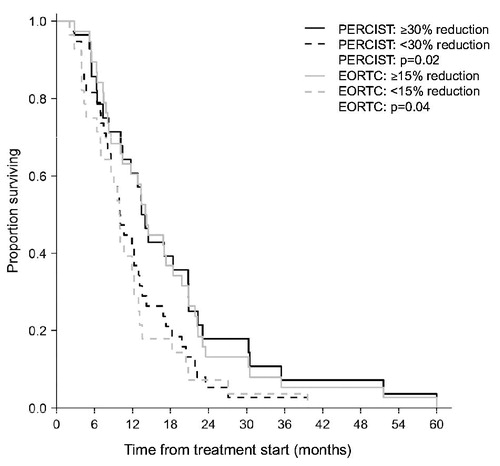
The same analysis with PERCIST resulted in an HR of 1.7 (95% CI 1.0–2.8) and a significant difference (p = 0.04) in median survival of 13.5 (95% CI 10.4–20.8) and 10.1 months (95% CI 8.6–13.4), respectively (). Correcting for PS, KRAS status and CEA at baseline changed the HR with EORTC criteria to 2.5 (95% CI 1.4–4.4, p = 0.002) and with PERCIST to 1.9 (95% CI 1.0–3.4, p = 0.04).
Discussion
We assessed early treatment response by FDG-PET/CT in patients with mCRC after one treatment with cetuximab and irinotecan, using two internationally recognized sets of criteria: EORTC criteria and PERCIST. Early metabolic response was correlated to the patients’ FDG-PET/CT-based BOMR, their CT-based BOR, and OS.
We found that an initial reduction in FDG uptake predicted metabolic response by EORTC criteria with a high sensitivity and by PERCIST with a high specificity. In spite of the cutoff difference, the two sets of criteria gave similar and relatively high PPVs for the prediction of metabolic response, indicating that they had equal ability to identify the patients who would achieve a PMR during treatment. To a lesser extent, with a bit lower NPVs, the two sets of criteria had equal ability to identify the patients who would not achieve a PMR. Regarding radiologic response, it was predicted with high sensitivity and also, the NPV among the early metabolic non-responders was high, correctly identifying the patients who would not achieve a partial radiologic response during treatment. Yet, early reduction in FDG uptake was not, with moderate specificity and especially low PPVs, a clear-cut predictor of long-term radiologic response to the treatment. The difference in survival between patients with an early metabolic response and patients without was notable, also after correcting for PS, KRAS status and CEA at baseline.
A few studies have investigated the utility of early FDG-PET/CT for prediction of response [Citation17,Citation18] and survival [Citation8,Citation17–19] in patients with mCRC. Bystrom et al. [Citation17] investigated the correlation between early decrease in SUV (EORTC criteria) and radiologic response in first-line palliative treatment. They found a sensitivity of 0.77, a specificity of 0.76, a PPV of 0.71 and an NPV of 0.81 of an early PET/CT performed after two cycles of chemotherapy. All four parameters were high and showed higher specificity and higher PPV than in our results, suggesting that early evaluation more optimally could be performed after two treatment cycles than after one. In line with our findings, the difference in OS between early metabolic responders and non-responders was 3.4 (24.9 vs. 21.5) months. Hendlisz et al. [Citation18] investigated the correlation between early decrease in SUV (EORTC criteria) and radiologic response in all lines of palliative treatment. They found a sensitivity of 1.0, a specificity of 0.57, a PPV of 0.43 and an NPV of 1.0 of an early PET/CT performed after one cycle of chemotherapy, as in our study. These findings resembles ours, however, they found a far stronger association between initial SUV decrease and OS of 27 versus 11 months between early metabolic responders and non-responders. Dimitrakopoulou-Strauss et al. [Citation19] also found a correlation between longer OS and initial SUV decrease, using SUVmean values. These findings, along with ours, support the idea that an early reduction in tumor metabolism is correlated to better response and longer survival. However, the results vary. This could be due to the study-specific differences in patient population, in the choice of SUV measurement and in the time-point of the early scan. Thus, the degree of correlation remains unclear.
Also, a large number of patients in our study did not achieve PR but SD as best response during treatment. Durable SD is, from a clinical perspective, an acceptable outcome. However, the patients who achieved SD as best response were, in our study, both early metabolic responders and non-responders. Classifying the patients with SD as responders would result in far lower values of especially specificity and NPV and thereby reduce the value of an early FDG-PET/CT as a predictive marker of long-term treatment response. How to distinguish this patient group from the ones who will not benefit from the treatment remains a challenge.
In the group of KRAS wt patients, the results for prediction of PMR showed the same tendency as in the whole patient group, but with slightly higher values. This was to be expected as the majority (69%) of the cohort was KRAS wt, and because KRAS wt patients have a far higher response rate than patients with KRAS mutations to treatment with cetuximab [Citation20,Citation21]. Finally, the absolute number of patients with KRAS mutations was relatively low, resulting in low statistical reliability.
Predicting PMR results in relatively high and more similar values of sensitivity, specificity, NPV and PPV than for prediction of PR. This is not surprising with the imaging modality unchanged from early to overall response evaluation and with noteworthy differences in distribution between metabolic and radiologic overall response earlier demonstrated [Citation22,Citation23]. However, it is not standard practice to evaluate treatment response of patients with mCRC with FDG-PET/CT instead of CT – the evaluation modality of choice.
In our population of patients with metastatic disease, progression-free survival (PFS) would have been informative. However, the patients were treated until progression was verified radiologically on the CT part of the FDG-PET/CT scan. In a large part of the patients this was not coincident with metabolically verified progression on the FDG-PET part of the scan. This insufficiency of the FDG-PET/CT-based PFS is a limitation of this study. However, the fact that all patients received the same treatment and all in third-line, strengthens the present study. It also enhances the comparability of the trial that we applied standardized pre-scan preparation of the patients and applied internationally recognized criteria to evaluate the response.
It has been suggested, that FDG-PET/CT could provide a favorable and useful visualization of the tumor changes induced by both chemotherapy and targeted therapy like the treatment regimen in this trial [Citation6,Citation7,Citation24]. Our findings support this suggestion. Still, differences in response evaluation methods, in time-points of assessing changes in FDG uptake, in cutoff values and in processing data challenge the comparison of trial results, and thereby the clinical utility of the outcomes [Citation1,Citation6,Citation10,Citation25].
Conclusions
We found, that in patients with mCRC, receiving third-line palliative treatment with irinotecan and cetuximab, an early reduction in FDG uptake was predictive of a later partial metabolic and partial radiologic treatment response. It was also predictive of significantly longer survival of early responders compared to early non-responders. However, our data and resulting sensitivities and specificities are not strong and unambiguous enough to support implementation of an early FDG-PET/CT scan for response prediction in daily clinical practice.
Acknowledgments
We would like to thank Kambiz Avoghlian, Theis Hansen and Jonas Stig Hermansen for their technical support with the scanner software and Tobias Wirenfeldt Klausen for his statistical support.
Disclosure statement
The authors report no conflicts of interest. The authors alone are responsible for the content and writing of the paper.
References
- De Maeseneer DJ, Lambert B, Surmont V, et al. 18-Fluorodeoxyglucose positron emission tomography as a tool for response prediction in solid tumours. Acta Clin Belg 2010;65:291–9.
- Michalski MH, Chen X. Molecular imaging in cancer treatment. Eur J Nucl Med Mol Imaging 2011;38:358–77.
- Van den Abbeele AD. The lessons of GIST–PET and PET/CT: a new paradigm for imaging. Oncologist 2008;13:8–13.
- Weber WA, Wieder H. Monitoring chemotherapy and radiotherapy of solid tumors. Eur J Nucl Med Mol Imaging 2006;33:27–37.
- Weber WA. Positron emission tomography as an imaging biomarker. J Clin Oncol 2006 10;24:3282–92.
- Desar IM, van Herpen CM, van Laarhoven HW, et al. Beyond RECIST: molecular and functional imaging techniques for evaluation of response to targeted therapy. Cancer Treat Rev 2009;35:309–21.
- Milano A, Perri F, Ciarmiello A, et al Targeted-therapy and imaging response: a new paradigm for clinical evaluation? Rev Recent Clin Trials 2011;6:259–65.
- de Geus-Oei LF, Vriens D, van Laarhoven HW, et al Monitoring and predicting response to therapy with 18F-FDG PET in colorectal cancer: a systematic review. J Nucl Med 2009;50:43S–54S.
- Allen-Auerbach M, Weber WA. Measuring response with FDG-PET: methodological aspects. Oncologist 2009;14:369–77.
- Weber WA. Assessing tumor response to therapy. J Nucl Med 2009;50:1S–10S.
- Young H, Baum R, Cremerius U, et al. Measurement of clinical and subclinical tumour response using [18F]-fluorodeoxyglucose and positron emission tomography: review and 1999 EORTC recommendations. Eur J Cancer 1999;35:1773–82.
- Wahl RL, Jacene H, Kasamon Y, et al. From RECIST to PERCIST: evolving considerations for PET response criteria in solid tumors. J Nucl Med 2009;50:122S–50S.
- Therasse P, Arbuck SG, Eisenhauer EA, et al. New guidelines to evaluate the response to treatment in solid tumors. European organization for research and treatment of cancer, National cancer institute of the United States, National cancer institute of Canada. J Natl Cancer Inst 2000;92:205–16.
- Shankar LK, Hoffman JM, Bacharach S, et al. Consensus recommendations for the use of 18F-FDG PET as an indicator of therapeutic response in patients in National Cancer Institute Trials. J Nucl Med 2006;47:1059–66.
- Cross J. DxS Ltd. Pharmacogenomics 2008;9:463–7.
- Kotoula V, Charalambous E, Biesmans B, et al. Targeted KRAS mutation assessment on patient tumor histologic material in real time diagnostics. PLoS One 2009;4:e7746.
- Bystrom P, Berglund A, Garske U, et al. Early prediction of response to first-line chemotherapy by sequential [18F]-2-fluoro-2-deoxy-D-glucose positron emission tomography in patients with advanced colorectal cancer. Ann Oncol 2009;20:1057–61.
- Hendlisz A, Golfinopoulos V, Garcia C, et al. Serial FDG-PET/CT for early outcome prediction in patients with metastatic colorectal cancer undergoing chemotherapy. Ann Oncol 2012;23:1687–93.
- Dimitrakopoulou-Strauss A, Strauss LG, Burger C, et al. Prognostic aspects of 18F-FDG PET kinetics in patients with metastatic colorectal carcinoma receiving FOLFOX chemotherapy. J Nucl Med 2004;45:1480–7.
- Saif MW, Shah M. K-ras mutations in colorectal cancer: a practice changing discovery. Clin Adv Hematol Oncol 2009;7:45–53, 64.
- Karapetis CS, Khambata-Ford S, Jonker DJ, et al. K-ras mutations and benefit from cetuximab in advanced colorectal cancer. N Engl J Med 2008;359:1757–65.
- Skougaard K, Johannesen HH, Nielsen D, et al. CT versus FDG-PET/CT response evaluation in patients with metastatic colorectal cancer treated with irinotecan and cetuximab. Cancer Med 2014;3:1294–301.
- Monteil J, Mahmoudi N, Leobon S, et al. Chemotherapy response evaluation in metastatic colorectal cancer with FDG PET/CT and CT scans. Anticancer Res 2009;29:2563–8.
- Krystal GW, Alesi E, Tatum JL. Early FDG/PET Scanning as a pharmacodynamic marker of anti-EGFR antibody activity in colorectal cancer. Mol Cancer Ther 2012;11:1385–8.
- Sargent DJ, Rubinstein L, Schwartz L, et al. Validation of novel imaging methodologies for use as cancer clinical trial end-points. Eur J Cancer 2009;45:290–9.